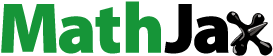
ABSTRACT
Immunonutrition or modulation of immune capacity through food and supplements has been gaining significant importance. Hydrolysed collagen has long been used as a functional ingredient, showing multiple physiological activities, including enhancement of immune functions. However, how collagen peptides may affect the immune system still needs further research. This study investigates bone collagen peptides (BCP) immunomodulatory activity on human monocytic THP-1 and human Jurkat T lymphocyte cell lines, using cytokine mRNA expressions as biomarkers. In vitro, gastrointestinal digestion and Caco-2 cell absorption allow obtaining digested and absorbed BCP fractions, respectively, which are tested on immune cells. Results show: (1) Immunostimulatory effect on M0 macrophages, but not on M1 macrophages (lipopolysaccharide (LPS)-activated), (2) Significant T lymphocyte proliferation after incubation with absorbed BCP fraction. (3) Significant increase of anti-inflammatory IL-10 cytokine biomarker. These results suggest that BCP could act as an immunonutrient, modulating the immune response and inflammatory processes.
Abbreviations: ATCC: American Type Culture Collection BCP: bone collagen peptide DMSO: dimethyl sulphoxide FBS: fetal bovine serum ECM: extracellular matrix GRAS: Generally recognized as safe; IFN: interferon IL: interleukin, LPS: lipopolysaccharide SEM: standard error of the mean TEER: transepithelial electrical resistance
1. Introduction
The immune system defends the human body against pathogens, toxic antigens, and tumour cells. Its response mechanisms take place through innate immunity and adaptive immunity. The first provides a quick response but lacks specificity and memory function. It comprises physical barriers and involves natural killer (NK) and phagocytic cells, such as neutrophils and macrophages. The second acts more slowly, but specifically, and retains a memory of its target. It involves B and T lymphocytes, and antigen-presenting cells.
For immune homeostasis or during the inflammatory response, both macrophages and T lymphocytes play a key role in the initiation, maintenance, and resolution of the threat. To do this, immune cells produce cytokines: small proteins that act as mediators in the immune response, haematopoiesis, and inflammation process, a mechanism of innate immunity whose purpose is to isolate and destroy the harmful agent, as well as repair the damaged tissue or organ. Macrophages, derived from monocytes, are found in blood and distributed throughout all body tissues to intervene against foreign substances or pathogens. They are effector cells of the immune system and the main actors in the innate immune response. Once activated, macrophages release different cytokines (IL-6, IL-1β) that promote the inflammatory response to neutralize the pathogen and make other cells of the immune (IL-8) system migrate to infection (Gauthier & Chen, Citation2022; Pollard, Citation2009). On the other hand, the balance between the subsets of T lymphocytes, and specifically CD4 +, both effectors (Th1, Th17, etc.) and T regulators play a crucial role in the control of inflammatory conditions. An important feature of T cell activation is the production of cytokines such as IL-2 that can cause T cell proliferation and differentiation (Luckheeram et al., Citation2012).
Global challenges, such as the high burden of known and new infections together with the recognized fact that immune capacity declines with age (immunosenescence), stress and malnutrition, have increased the concern to find out how the diet and individual nutrients can influence the immune system function. Collagen is the main structural protein of the extracellular matrix (ECM) of mammalian connective tissues and the most abundant in the human body, accounting for approximately 30% of all proteins. Edible collagen is extracted from collagen-rich animal tissues such as bovine and porcine skin and bones, and chicken and fish by-products. It can be found in foods in the form of gelatin, which is heat denatured collagen (MW: 20,000–100,000 Da), as well as in dietary supplements as hydrolysed collagen (also called hydrolysed gelatin or collagen peptides), made up of small peptides (MW: < 6000 Da) obtained by hydrolysis of gelatin with proteolytic enzymes. In addition to free amino acids, a considerable number of Hyp-containing oligopeptides have been detected in blood after hydrolysed collagen ingestion (Shigemura et al., Citation2014; Shigemura et al., Citation2018). Some of these peptides seem to exhibit biological activity (Chen et al., Citation2020; Fu et al., Citation2019), especially certain dipeptides (Koyama, Citation2016; Sato et al., Citation2020). This means that collagen peptides can stimulate physiological processes whose compliance could produce health beneficial effects on living organisms. Collagen peptides are also generated by the degradation of endogenous collagen at sites of tissue injury and inflammation. Interestingly, collagen peptides derived from orally administered hydrolysed collagen are deposited at the same sites (Koyama, Citation2016). Being identical, both endogenous and exogenous collagen peptides are thought to act as biological danger signals, recruiting immune cells and fibroblasts to trigger the healing process. Since collagen is a ubiquitous component of ECM, this mechanism seems to be a common protective and repair system throughout the body that can be reproduced and enhanced by ingestion of oral hydrolysed collagen (Sato et al., Citation2020).
First studies on hydrolysed collagen date back to the 80s, but during the last two decades, its number and diversity have grown notably. Clinical and in vivo studies have reported improvement in most prevalent degenerative diseases in joints and bones (Daneault et al., Citation2017; Porfírio & Fanaro, Citation2016), skin properties (de Miranda et al., Citation2021), pressure ulcer and wound healing (Nomoto & Iizaka, Citation2020), sarcopenia (Zdzieblik et al., Citation2015) and fibromialgia (Olson et al., Citation2016). Other studies have reported physiological effects as an antioxidant (Hong et al., Citation2019), antihypertensive (Kouguchi et al., Citation2013), and antidiabetic (Huang et al., Citation2014). In addition, several studies have reported immunomodulatory activity and immune improvements in different situations: immune capacity in immunosuppressive conditions (Koyama et al., Citation2015; Li et al., Citation2019; Si et al., Citation2020; Si et al., Citation2021; Yu et al., Citation2020), intestinal barrier and immune gut functions (Li et al., Citation2019; Song et al., Citation2019), skin damage (Chen et al., Citation2017; Fan et al., Citation2013; Subhan et al., Citation2017), inflammatory, allergic and stress conditions (Bakaeva et al., Citation2018; Hartog et al., Citation2013; Karnjanapratum et al., Citation2016; Nishikimi et al., Citation2018; Sae-leaw et al., Citation2016), tumour inhibition (Alemán et al., Citation2011; Liang et al., Citation2010), immunological properties of osteogenic differentiated stem cells, induced by collagen hydrolysate (Liu & Sun, Citation2019; Liu & Sun, Citation2020) and autoimmune and non-autoimmune diseases with a relevant inflammatory component as ulcerative colitis (Azuma et al., Citation2014; Daskalaki et al., Citation2021; Zhu et al., Citation2018), rheumatoid arthritis (Abramson et al., Citation2014; Mortarino et al., Citation2016), asthma (Zheng et al., Citation2019), atherosclerosis (Zhang et al., Citation2010) and chronic kidney disease (Zhu et al., Citation2020).
Neither native collagen (whole molecule present in animal tissues) nor gelatin (denatured collagen) develops bioactivity of hydrolysed collagen because bioactive peptides are inactive within their parent protein and only exhibit physiological effects once they are released by hydrolysis (Fan et al., Citation2013; Zhu et al., Citation2018). The bioactivity of hydrolysed collagen is exclusively exerted by the compounds derived from its intake that reach organs and tissues through the bloodstream (Fan et al., Citation2013; Larder et al., Citation2021; Zhu et al., Citation2018), whose composition depends mainly on the achievement of two previous stages: 1. Exogenous hydrolysis, which transforms gelatin into hydrolysed collagen. 2. Digestive hydrolysis, which yields a bioaccessible fraction, followed by intestinal absorption, which yields a bioavailable fraction. Therefore, to evaluate in vitro hydrolysed collagen's real bioactivity, its previous digestion and absorption should be considered. Taking all this information into account, the aim of this work is to investigate the effect of bone collagen peptides (BCP) on different immune cell lines, such as macrophages and T lymphocytes as representative cells of innate and adaptative immunity, respectively. Expressions of specific cytokines are used as biomarkers to evaluate the possible immunomodulatory activity of BCP. In addition, gastrointestinal digestion and intestinal absorption of BCP are simulated using in vitro methodologies, obtaining a digested or bioaccessible fraction and an absorbed or bioavailable fraction, respectively. This allows approaching in vivo systems and hypothesizing the underlying molecular mechanisms of BCP fractions that would be in contact with immune cells: the bioaccessible fraction in contact with gut immune cells and the bioavailable fraction in contact with blood immune cells.
2. Materials and methods
2.1. Bone collagen peptides
Enzymatically hydrolysed collagen COLPROPUR D® manufactured and provided by PROTEÏN, S.A. (Girona, Spain) was used for this study. The product is commercially available and consists of collagen peptides from porcine fresh bones (BCP), with a mean molecular weight of 3000–5000 Da and a high safety profile. It does not contain any additives, sweeteners, or flavours. No allergies or incompatibilities with drugs, foods, or dietary supplements have been observed.
Collagen hydrolysates received GRAS status (Generally recognized as safe) from the FDA (Citation2009). Additionally, the safety of the COLPROPUR D® production process was specifically evaluated and approved by EFSA (‘Opinion of the Scientific Panel’, Citation2005). COLPROPUR D® amino acid composition (Supplementary Materials Table 1) and molecular weight distribution (Supplementary Material Figure 1) was provided by the manufacturing company.
2.2. In vitro gastrointestinal digestion
Gastrointestinal digestion was simulated using AINIÁs in vitro Dynamic Gastrointestinal Digester equipment (ES 2 361 983 B1). This digester mimics the upper gastrointestinal tract of human adults. The digestive system consists of interconnected multicompartmental, computer-controlled equipment composed of a module that mimics gastric digestion, and another module simulating intestinal digestion. 10 g of COLPROPUR D®, which represent the recommended daily dose of the product, was added to the in vitro digestion procedure, obtaining the digested CLP sample. In addition, the same process using water (lack of sample) was done to obtain the digested control.
The dynamics of digestive content mixing, gastric emptying, and intestinal transit times, as well as gastric and intestinal pH values, were simulated in a computer-controlled process. In vitro, dynamic digestion was conducted following the method previously described by our group (Nieto et al., Citation2021) with few modifications. All the parameters for in vitro digestion were selected to reproduce the gastrointestinal conditions of a healthy human adult after intake of a solid meal. The whole transit times were 1 min, 2, and 6 h in the mouth, stomach, and intestine compartments, respectively. All the simulated digestive secretions (e.g. gastric juice with enzymes, electrolytes, pepsin, bile, pancreatin) were freshly prepared. Gastric and intestinal transit was simulated by opening and closing the peristaltic valves connecting the compartments.
The successive intestinal emptying samples were collected in a vessel cooled in an ice-bath (4°C) to stop the digestive enzyme activity, obtaining the accumulated intestinal digested. The whole accumulated intestinal digest was homogenized and heated in a water bath for 10 min at 90°C to inactivate the digestive proteases. The sample was then subjected to a centrifugation process to obtain the soluble fraction and the non-soluble fraction (precipitate), characterized by the non-soluble compounds and the insolubilized compounds during the digestion process, that represent the fraction of potentially non-absorbable digest which passed through the small intestine to reach the large intestine. The COLPROPUR D® in vitro digestion simulation was conducted in duplicates.
2.3. Cells
Human epithelial cell line Caco-2 obtained from the American Type Culture Collection (ATCC® HTB-37 ™, Manassas, VA, USA) was used to carry out intestinal transport studies. For its maintenance, cells were cultured in a growth medium containing Eagle Minimum Essential Medium (EMEM, ATCC) supplemented with 20% fetal bovine serum (FBS, Gibco-Thermo Fisher Scientific) and antibiotics (penicillin 100 U/mL, streptomycin 30 μg/mL, PAN-Biotech, Aidenbach, Germany), following ATCC recommendations. A biocompatibility test was carried out with the samples to determine the maximum biocompatible concentration with the cell model and then, the protocol for the intestinal absorption test was carried out.
Two cell lines were used for immune modulatory evaluation. Human monocytic cell line THP-1 (ATCC® TIB-202™) was used as macrophages. This cell line was maintained with RPMI-1640 (ATCC) supplemented with 10% FBS and 0.05 mM 2-mercaptoethanol following ATCC recommendations. Jurkat T lymphocyte cell line obtained from ATCC (ATCC® Clone E6-1 TIB-152) was used as lymphocytes since the effect of collagen on this cell line has been previously tested (Bijian et al., Citation2007). RPMI-1640 supplemented with 10% FBS was used for maintenance of the cell line, following ATCC recommendations. All cultures were grown at 37°C and 5% CO2.
2.4. Cell viability measurement
Samples used in the different cell models were the soluble fractions generated from the simulated gastrointestinal digestion of COLPROPUR D®. Blank adding water instead of the sample was generated as a control digest. These soluble fractions were filtered and frozen until used. Two replicates of each product obtained in the bioaccessibility studies were integrated to perform cellular assays. A cytotoxicity assay was done with serial dilutions of the samples in all three cell lines: Caco-2, THP-1, and Jurkat, using alamarBlue Cell Viability Reagent (Thermo Fisher Scientific, Waltham, MA, USA). After the exposure period (120 min), the reagent was added according to manufacturer instructions, and fluorescence was read at Ex 530 nm Em. 590 using Fluoroskan™ FL (Themo Fisher Scientific). DMSO at 20% was used as a positive cytotoxicity control. The percentage of cell viability relative to control cells (untreated) was determined as follows:
2.5. Transepithelial intestinal transport studies
Intestinal absorption through intestinal epithelial cells was assessed after optimizing culture conditions. Briefly, Caco-2 cells were seeded into the polyester membrane (0.4 µm of pore size; Corning, Somerville, MA, USA) inserts and cells were incubated at 37°C for 21 days for its differentiation (Hubatsch et al., Citation2007). The integrity of cell monolayers was determined by measuring transepithelial electrical resistance (TEER) using Millicell ERS-2 (Millipore Corporation, Burlington, MA, USA) before and after treatment. After incubation with digested COLPROPUR D® and digested control at 1/16 dilution for 120 min at 37 °C, the basal medium was collected obtaining the absorbed COLPROPUR D® and absorbed control. Both fractions were used for further treatments of macrophage and lymphocyte cell models. Only cell monolayers with TEER values higher than 300 Ω cm2 were considered.
2.6. Immunomodulatory studies with macrophages
THP-1 cells were treated with two different samples. Bioaccessible soluble fractions obtained after simulating its gastrointestinal digestion were diluted for its compatibility (at 1/4 dilution, aprox. 2,5 mg/mL of BCP) and exposed for 2 h, a short exposure time simulating the effect during intestine digestion. In addition, the bioavailable fraction obtained after simulating its intestinal transport at 1/16 dilution (aprox. 625 μg/mL of BCP) was directly in contact with cells for 24 h, a long exposure time simulating the potential systemic effect after absorption.
For immunomodulatory assays, these cells were treated with the samples in 2 different phenotypes. First, cells were treated with 200 nM of phorbol 12-myristate 13-acetate (PMA), for 72 h which differentiates THP-1 cells in M0 macrophages. These macrophages adherent cells after differentiation were treated with 100 ng/mL of bacterial lipopolysaccharide (LPS) from E.Coli (Sigma-Aldrich, Saint Louis, MO, USA) to obtain inflammatory M1 macrophages. Both M0 and M1 macrophages were in contact with the samples for each exposure time. Finally, cell lysates were obtained for further analysis.
2.7. Immunomodulatory studies with lymphocytes
For immunomodulation and cell proliferation assay, Jurkat cells were treated with 2 different samples. The bioaccessible soluble fractions obtained after simulating the gastrointestinal digestion were diluted for its compatibility (at 1/4 dilution, aprox. 2.5 mg/mL of BCP) and exposed for 2 h, a short exposure time simulating the effect during digestion in the intestine. Also, the bioavailable fraction obtained after simulating its intestinal transport at 1/16 dilution (aprox. 625 μg/mL of BCP) was directly in contact with cells for 24 h, a long exposure time simulating the potential systemic effect after absorption.
For the immunomodulatory assays, Jurkat cells were seeded in multi-well plates and after each exposure time, cells were washed, and cell lysates were obtained for further experiments. Regarding the proliferation assay, alamarBlue (Thermo Fisher Scientific) was used according to manufacturer instructions at different time points. After each exposure time (T0) and after 24 (T24) and 48 (T48) h of the exposure time.
2.8. RNA isolation and quantitative real-time polymerase chain reaction (qRT-PCR)
After different cell treatments, cellular RNA was isolated and purified using MAXWELL equipment (Promega, Madison, WI, USA). RNA quantity and quality were measured by Nanodrop (Thermo Fisher Scientific) before cDNA synthesis. cDNA was obtained from 1 μg of quality RNA using High-Capacity cDNA reverse transcription kit (Applied Biosystems, Foster City, CA, USA). Real-time PCR was performed with TAQMAN™ fast advanced master mix (Thermo Fisher Scientific) from cDNA using commercial primers of selected biomarkers: IL-6 (Hs00174131_m1), IL-1β (Hs01555410-m1), IL-10 (Hs00961622-m1) for THP-1 cells and IL-2 (Hs00174114-m1), IFN-γ (Hs00989291-m1) and IL-10 for Jurkat. All primers were obtained from Thermo Fisher Scientific. A housekeeping gene, β-actin was used. Quantification of gene expression was carried out in a relative way, therefore the magnitude of physiological changes in every biomarker gene was obtained in comparison with the reference gene β-actin. For calculations, the formula 2−ΔΔCTwas used.
2.9. Statistical analyses
Results were presented as mean ± standard error of the mean (SEM). Statistical significance between different conditions was assessed using the student's t-test with a Welch's correction applied in case of significantly different variances (F test) using GraphPad Prism software 8.3.1. A value of p ≤ 0.05 was considered significant.
3. Results
BCP immunomodulatory activity was examined in four situations resulting from the treatment of macrophages (M0 and M1 (LPS)-activated) and T lymphocytes with digested and absorbed BCP fractions. Results showed significant changes in biomarkers (mRNA cytokine expressions) compared to digestion and absorption blanks (samples resulting from performing digestion and absorption processes, but in the absence of BCP). Previously, the corresponding cell viability assays were carried out to determine the concentration of BCP samples to be tested.
3.1. Assessment of digested BCP fraction immunomodulation on macrophage
To study the effects of digested BCP fraction on macrophages, the biocompatibility was first evaluated. Using 1/4 dilution, no toxic effects on macrophages were observed in contact with both digestion blank and digested BCP fraction (aprox. 2.5 mg/mL), since it was the first dilution that showed no differences with the untreated control. Interestingly, at 1/2 dilution, digested BCP fraction (aprox. 5 mg/mL of BCP) showed biocompatibility while digestion blanks not, suggesting a protective effect. DMSO positive control showed a cytotoxic effect, as expected ((A)).
Figure 1. (A) Cell viability and (B) changes in mRNA cytokine expression of digested COLPROPUR D® samples (aprox. 2,5 mg/mL) in M0 and M1 macrophages after 2 h in contact. DMSO: dimethyl sulphoxide **p ≤ 0.01; *p ≤ 0.05. n = 5.
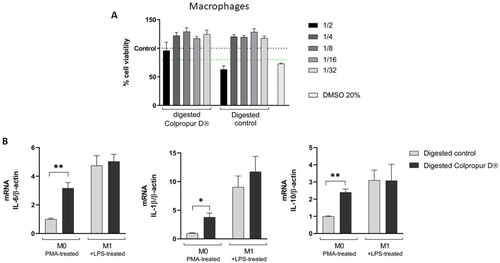
After 2 h of contact between M0 macrophages and digested BCP fraction, a significant increase in all cytokines (IL-6, IL-1β, and IL-10) biomarkers were detected compared to digestion blank. In contrast, biomarkers did not undergo significant changes in M1 macrophages ((B)).
3.2. Assessment of digested BCP fraction immunomodulation on T lymphocytes
To study the effects of digested BCP fraction on T lymphocytes, the biocompatibility was first evaluated. Using 1/4 dilution, no toxic effects were observed in contact with both digestion blank and digested BCP fraction (aprox. 2.5 mg/mL), since it was the first dilution that showed no differences with the untreated control. Interestingly, at 1/2 dilution, digested BCP fraction (aprox. 5 mg/mL of BCP) showed biocompatibility while digestion blanks not, suggesting a protective effect. DMSO positive control showed a cytotoxic effect, as expected ((A)).
Figure 2. (A) Cell viability and (B) changes in mRNA cytokine expression of digested COLPROPUR D® samples (aprox. 2.5 mg/mL) in Jurkat lymphocytes after 2 h in contact. DMSO: dimethyl sulphoxide ***p ≤ 0.001; *p ≤ 0.05. n = 6.
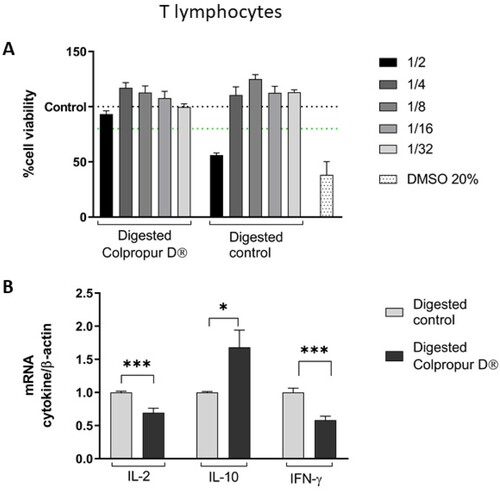
After 2 h of contact between T lymphocytes and digested BCP fraction, a significant increase in anti-inflammatory IL-10 cytokine biomarker and a significant reduction in pro-inflammatory IL-6 and IFN-γ cytokine biomarkers were detected, compared to digestion blank. ((B)).
3.3. Assessment of Caco-2 cell biocompatibility
Epithelial intestinal cells were in contact with serial dilutions of digested fractions and their cell viability was measured. Using 1/16 dilution, no toxic effects on Caco-2 were observed in contact with both digestion blank and digested BCP fraction (aprox. 625 µg/mL), since it was the first dilution that showed no differences with the untreated control. Interestingly, at 1/8 dilution, digested BCP fraction (aprox. 1.25 mg/mL of BCP) showed biocompatibility with Caco-2 cells while digestion blanks not, suggesting a protective effect. DMSO positive control showed a cytotoxic effect, as expected ().
Figure 3. (A) Cell viability of digested COLPROPUR D® samples (aprox. 2.5 mg/mL) in Caco-2 epithelial cells after 2 h in contact. DMSO: dimethyl sulphoxide. n = 6.
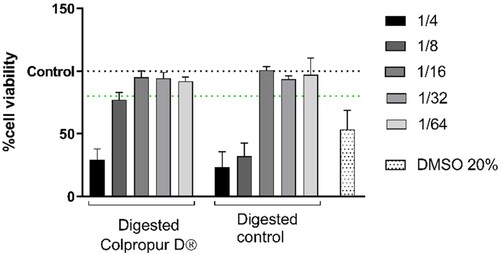
For subsequent assays, the absorbed BCP fraction obtained from 625 µg/mL of digested BCP and its equivalent control were used.
3.4. Assessment of absorbed BCP fraction immunomodulation on macrophage
After 24 h of contact between M0 macrophages and absorbed BCP fraction, an increase in all cytokine biomarkers was detected (significant in IL-1β and IL-10 biomarkers), compared to absorption blank. In contrast, biomarkers did not undergo significant changes in M1 macrophages ().
3.5 Assessment of absorbed BCP fraction immunomodulation on T lymphocytes
After 24 h of contact between T lymphocytes and absorbed BCP fraction, a significant increase in pro-inflammatory IL-2 cytokine biomarker and a significant reduction in pro-inflammatory IFN-γ cytokine biomarker were detected, compared to absorption control ((A)).
Figure 5. (A) Changes in mRNA cytokine expression and (B) cell proliferation of absorbed COLPROPUR D® samples in Jurkat lymphocytes after 24 h in contact. ***p ≤ 0.001; **p ≤ 0.01; *p ≤ 0.05. n = 6.
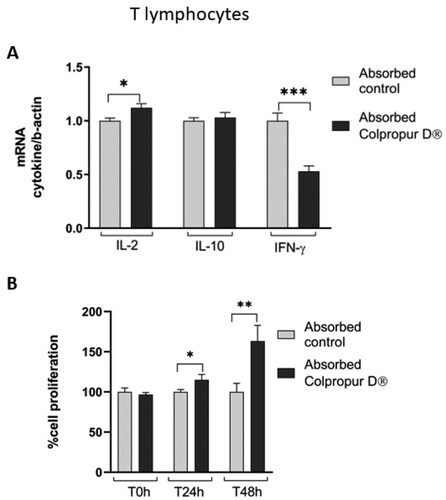
In addition, 24 h after finishing treatment, a significant increase in T-lymphocyte cell proliferation was detected, (even more significant at 48 h), suggesting activation of T-lymphocytes, which corresponds to the increase in pro-inflammatory IL-2 cytokine biomarker ((B)).
4. Discussion
The results of this study suggest that, after simulating the processes of digestion and absorption, BCP exerts immunomodulatory activity on human monocytic THP-1 and Jurkat T lymphocytes cell lines, as representative cells of innate and adaptive immunity, respectively. Monocytes are induced to differentiate into M0 macrophages and lipopolysaccharide (LPS)-stimulated M1 macrophages and both types of cells are tested. mRNA expressions of specific cytokines are evaluated, and different results are observed depending on the type of immune cell tested and whether it is treated with digested BCP fraction, which is thought to act at the intestinal level, or with absorbed BCP fraction, which is thought to pass into the blood, reach organs and tissues, and act at a systemic level.
Cytokines regulate cell-to-cell communication and play a crucial role in inflammatory and immune responses (Liu et al., Citation2021). In addition to lymphocytes and macrophages, non-immunological cells are also capable of producing cytokines. The knowledge of the role of cytokines has allowed cytokine profile diagnostic to have clinical utility (Dhar et al., Citation2021).
Some studies have observed that mRNA expression and secretion of cytokines respond to collagen peptides, suggesting that hydrolysed collagen could act as an immunonutrient: gelatin hydrolysate reduces pro-inflammatory IL-6 and IL-1β cytokine production in LPS-stimulated RAW 264.7 macrophage cells (Karnjanapratum et al., Citation2016; Sae-leaw et al., Citation2016); in a dextran sodium sulphate (DSS)-induced colitis mice model, gelatin degradation products (prolyl-hydroxyproline and glycine) and hydrolysed collagen from different sources prevent an increase in pro-inflammatory IL-6 and IL-1β cytokines in the colon and in peripheral blood (Daskalaki et al., Citation2021; Zhu et al., Citation2018); collagen peptides inhibit pro-inflammatory cytokine expressions (IL-1β and others) in human epidermal CoCl2-stimulated HaCaT keratinocytes, protecting them against cytotoxicity, oxidative stress and inflammatory responses (Subhan et al., Citation2017).
Other studies use cytokines as biomarkers to show that bone marrow mesenchymal stem cells (BMSCs) maintain their immunological properties after being induced to osteogenic differentiation by hydrolysed fish collagen (HFC- BMSCs). In this case, different results are obtained: on the one hand, both undifferentiated BMSCs and differentiated HFC-BMSCs can suppress pro-inflammatory IL 1β and IL 6 cytokine expression and secretion in a co-cultured model with RAW264.7 macrophages (Liu & Sun, Citation2020); on the other hand, pro-inflammatory IL-6 and anti-inflammatory IL-10 cytokine productions are higher in differentiated HFC-BMSCs cultures than in undifferentiated BMSCs cultures; also, peripheral blood mononuclear cells (i.e. lymphocytes, monocytes and other immune cells) produced higher amount of IL-6 and IL-10 when co-cultured with differentiated HFC- BMSCs than with undifferentiated BMSCs, suggesting that hydrolysed collagen could exert an immunostimulant effect; in contrast, both undifferentiated BMSCs and differentiated HFC-BMSCs reduce IL-1β production when co-culture with LPS-stimulated NR8383 macrophage cells, suggesting that the immunostimulant effect does not continue when the macrophages are already activated (Liu & Sun, Citation2019).
In line with the last cited study, in the present study, both digested and absorbed BCP fractions increase pro-inflammatory IL-6, IL-1β, and anti-inflammatory IL-10 cytokine expressions in M0 macrophages. In contrast, this immunostimulatory effect does not take place when macrophages are previously LPS-stimulated (M1 macrophages), suggesting that BCP could contribute to maintaining immune alertness that would not lead to an exacerbation of the immune response in the event of a pathogen or pathogen-associated molecular pattern appearance. In addition, M0 macrophages cell viability is markedly higher in contact with digested BCP fraction (1/2 dilution, approx. 5mg/mL) than with the digestion blank at the same dilution. These results suggest that BCP could produce an immunoprotective effect, together with an immunostimulatory effect, on M0 macrophages, which could be useful in inducing an effective immune response.
Some studies suggest that collagen peptides could modulate T-cell function and development. In an ovalbumin (OVA)-induced allergy model, collagen peptide administration inhibits mice ear swelling and modulates the balance of cytokine production in splenocytes toward T-helper type 1 (Th1) and regulatory T cells (Treg) (Nishikimi et al., Citation2018). In immunocompromised mice (simulated weightlessness condition), collagen peptide administration improves peripheral blood lymphocyte distribution and T lymphocyte proliferation (Si et al., Citation2020). A placebo-controlled double-blind study concludes that the ingestion of 10 g of collagen peptide for 8 weeks improves human immunological status, especially Scoring of Immunological Vigour (SIV) scores, and T lymphocytes number and their subsets (Koyama et al., Citation2015).
Consistent with these results, in the present study, T lymphocyte cell viability is markedly higher in contact with digested BCP fraction (1/2 dilution, approx. 5mg/mL) than with the digestion blank at the same dilution, as happens in the case of macrophages. Furthermore, the absorbed BCP fraction induces a significant increase in T lymphocyte cell proliferation and pro-inflammatory IL-2 cytokine expression. Both effects are interrelated since, as is known, IL-2 cytokine is produced by activated T lymphocytes and in turn, promotes their proliferation.
Several studies suggest that hydrolysed collagen modulates inflammatory responses, both locally and systemically (Abramson et al., Citation2014; Azuma et al., Citation2014; Chen et al., Citation2017; Daskalaki et al., Citation2021; Hartog et al., Citation2013; Mortarino et al., Citation2016; Subhan et al., Citation2017; Zhang et al., Citation2010; Zheng et al., Citation2019; Zhu et al., Citation2020). This anti-inflammatory effect seems to be due to different bioactivity of collagen peptides: on the one hand, the possibility to induce oral tolerance, a physiological mechanism that moderates immune auto-reactivity and could take place when collagen peptides interact with certain nodules of gut-associated lymphoid tissue (Peyer's patches), which could be useful for autoimmune diseases (Abramson et al., Citation2014; Mortarino et al., Citation2016); on the other hand, the possibility to inhibit pro-inflammatory cytokine production (Karnjanapratum et al., Citation2016; Liu & Sun, Citation2019; Liu & Sun, Citation2020; Sae-leaw et al., Citation2016; Subhan et al., Citation2017; Zhang et al., Citation2010; Zhu et al., Citation2018) and increase anti-inflammatory cytokine production, especially IL-10, that is known to be produced by Treg cells in the gut and to moderate allergic and inflammatory reactions (Daskalaki et al., Citation2021; Nishikimi et al., Citation2018). In fact, in a healthy gut, IL-10 production is essential for maintaining intestinal homeostasis, as it provides the necessary anti-inflammatory environment to enable microbial symbiosis (Daskalaki et al., Citation2021). It is known that intestinal resident macrophages promote the differentiation and maintenance of Treg cells in the intestine via IL-10 production, contributing directly and indirectly to the dampening of inflammation (Ochi et al., Citation2016).
Consistent with these studies, in the present study, IL-10 expression increases after the exposure of digested BCP fraction in both M0 macrophages and T lymphocytes, and after the exposure of absorbed BCP fraction in M0 macrophages, suggesting an anti-inflammatory effect, especially at the intestinal level. In addition, both digested and absorbed BCP fractions reduce pro-inflammatory IFN–γ cytokine expression in T lymphocytes. In line with this result, a decrease in IFN-γ serum level is detected in studies that postulate an increase in oral tolerance and a reduction in rheumatoid arthritis symptoms when hydrolysed collagen is administered (Abramson et al., Citation2014; Mortarino et al., Citation2016). These results suggest that BCP could have an anti-inflammatory effect potentially useful for the management of autoimmune disorders, allergies, and inflammation-mediated diseases.
Some limitations of this study should be considered. On the one hand, in vitro conditions do not reproduce or include all active mediators involved in the immune and inflammatory responses. On the other hand, an in vitro study cannot reproduce the cumulative effect that a daily intake can have on the bioactivity of the functional ingredient studied. In the case of BCP, it is designed to take 10 g daily, an amount used only once in this in vitro study for 2 or 24-hour treatments. Despite this, in vitro studies are widely used and necessary to find out molecular mechanisms that explain the role of specific cell types and elucidate the effects detected in vivo studies. In our study, only changes in mRNA cytokine expression have been evaluated, considering that these biomarkers constitute the first step to define the immunomodulatory activity of BCP. In fact, some studies show the correlation between mRNA and protein level of some of the cytokines analysed in our study (Liu & Sun, Citation2020; Subhan et al., Citation2017).
5. Conclusions
This study (summary in ) suggests that oral intake of BCP (COLPROPUR D®) could modulate the immune status of human macrophages and T-lymphocytes. In approach to an in vitro system, this immunomodulatory activity would take place both locally, exerted by digested or bioaccessible BCP fraction (that would act on the gut), and systemically, exerted by absorbed or bioavailable BCP fraction (that would pass into the bloodstream).
Table 1. Summary of BCP immunomodulatory activity.
The most remarkable findings are: (1) Immunostimulating effect on macrophages, suggested by the increase in IL-6, IL-1β, and IL-10 expressions in contact with both the bioaccessible and bioavailable BCP fraction, which does not occur when macrophages have been previously stimulated by an antigen. (2) Increase in cell proliferation of T lymphocytes in contact with bioavailable BCP fraction, together with an increase in cell viability of both macrophages and T lymphocytes in contact with bioavailable BCP fraction, which could denote an immunoprotective effect on immune cells. (3) Anti-inflammatory effect suggested by the increase in IL-10 expression on macrophages in contact with both the bioaccessible and bioavailable BCP fraction, and on T lymphocytes, in contact with the bioaccessible BCP fraction.
These results point to BCP as a possible immunonutrient, suggesting that it could favour an effective and non-exacerbated immune response, a protective effect on the immune cells population, and an anti-inflammatory effect.
Supplemental Material
Download MS Word (45 KB)Acknowledgements
The authors thank Teresa Tomás and Lorena Argudo for their support and assistance in the methodology. Also, Juan Pedro Alcántara and Esther Basés, who work at PROTEIN, S.A., for their contribution of knowledge about studied product nature and review of current scientific publications on oral hydrolysed collagen. The study was financially supported by PROTEIN SA, Spain.
Disclosure statement
No potential conflict of interest was reported by the author(s).
Additional information
Funding
References
- Abramson, D. B., Cabello, J., Bumaguin, G. E., Jamín, A., Vitelli, E. J., Zingoni, N., Sarrió, L., Feldman, S., & Cointry, G. R. (2014). Tolerancia oral en artritis experimental inducida por antígeno en conejos por administración de hidrolizado de cartílago articular. Inmunología, 33(4), 121–127. https://doi.org/10.1016/J.INMUNO.2014.06.003
- Alemán, A., Pérez-Santín, E., Bordenave-Juchereau, S., Arnaudin, I., Gómez-Guillén, M. C., & Montero, P. (2011). Squid gelatin hydrolysates with antihypertensive, anticancer and antioxidant activity. Food Research International, 44(4), 1044–1051. https://doi.org/10.1016/J.FOODRES.2011.03.010
- Azuma, K., Osaki, T., Tsuka, T., Imagawa, T., Okamoto, Y., & Minami, S. (2014). Effects of fish scale collagen peptide on an experimental ulcerative colitis mouse model. PharmaNutrition, 2(4), 161–168. https://doi.org/10.1016/J.PHANU.2014.10.001
- Bakaeva, Z., Ermakova, N., Mankaeva, O., Sveshnikov, D., Severin, A., Sinel’nikova, A., Starshinov, Y., Radysh, I., Torshin, V., Frolov, D., Kozlov I. G. (2018). Collagen hydrolysis products reduce the formation of stress-induced ulcers by regulating stress-associated activation of the neuroendocrine and immune systems. Bulletin of Experimental Biology and Medicine, 165(4), 449–452. https://doi.org/10.1007/S10517-018-4191-X
- Bijian, K., Zhang, L., & Shen, S. H. (2007). Collagen-mediated survival signaling is modulated by CD45 in Jurkat T cells. Molecular Immunology, 44(15), 3682–3690. https://doi.org/10.1016/j.molimm.2007.04.005
- Chen, M., Li, Y., & Huang, G. (2020). Potential health functions of collagen bioactive peptides: A review. American Journal of Biochemistry and Biotechnology, 16(4), 507–519. https://doi.org/10.3844/AJBBSP.2020.507.519
- Chen, Q., Hou, H., Wang, S., Zhao, X., & Li, B. (2017). Effects of early enteral nutrition supplemented with collagen peptides on post-burn inflammatory responses in a mouse model. Food & Function, 8(5), 1933–1941. https://doi.org/10.1039/C7FO00181A
- Daneault, A., Prawitt, J., Fabien Soulé, V., Coxam, V., & Wittrant, Y. (2017). Biological effect of hydrolyzed collagen on bone metabolism. Critical Reviews in Food Science and Nutrition, 57, 1922–1937. https://doi.org/10.1080/10408398.2015.1038377
- Daskalaki, M., Axarlis, K., Aspevik, T., Orfanakis, M., Kolliniati, L. I., Tzardi, M., Dermitzaki, E., Venihaki, M., Kousoulaki, K., Tsatsanis, C., et al. (2021). Fish sidestream-derived protein hydrolysates suppress DSS-induced colitis by modulating intestinal inflammation in mice. Marine Drugs, 19(6). https://doi.org/10.3390/MD19060312
- de Miranda, R. B., Weimer, P., & Rossi, R. C. (2021). Effects of hydrolyzed collagen supplementation on skin aging: A systematic review and meta-analysis. International Journal of Dermatology, 60(12), 1449–1461. https://doi.org/10.1111/IJD.15518
- Dhar, S. K., V, K., Damodar, S., Gujar, S., & Das, M. (2021). IL-6 and IL-10 as predictors of disease severity in COVID-19 patients: Results from meta-analysis and regression. Heliyon, 7. https://doi.org/10.1016/J.HELIYON.2021.E06155.
- Fan, J., Zhuang, Y., & Li, B. (2013). Effects of collagen and collagen hydrolysate from jellyfish umbrella on histological and immunity changes of mice photoaging. Nutrients, 5(1), 223–233. https://doi.org/10.3390/NU5010223
- FDA. (2009). Evaluation of the health aspects of gelatin as a food ingredient. https://ntrl.ntis.gov/NTRL/dashboard/searchResults/titleDetail/PB254527.xhtml
- Fu, Y., Therkildsen, M., Aluko, R., & Lametsch, R. (2019). Exploration of collagen recovered from animal by-products as a precursor of bioactive peptides: Successes and challenges. Critical Reviews in Food Science and Nutrition, 59(13), 2011–2027. https://doi.org/10.1080/10408398.2018.1436038
- Gauthier, T., & Chen, W. (2022). Modulation of macrophage immunometabolism: A new approach to fight infections. Frontiers in Immunology, 13. https://doi.org/10.3389/FIMMU.2022.780839
- Hartog, A., Cozijnsen, M., de Vrij, G., & Garssen, J. (2013). Collagen hydrolysate inhibits zymosan-induced inflammation. Experimental Biology and Medicine, 238(7), 798–802. https://doi.org/10.1177/1535370213480740
- Hong, G.-P., Min, S.-G., & Jo, Y.-J. (2019). Anti-oxidative and anti-aging activities of porcine by-product collagen hydrolysates produced by commercial proteases: Effect of hydrolysis and ultrafiltration. Molecules, 24. https://doi.org/10.3390/MOLECULES24061104
- Huang, S. L., Hung, C. C., Jao, C. L., Tung, Y. S., & Hsu, K. C. (2014). Porcine skin gelatin hydrolysate as a dipeptidyl peptidase IV inhibitor improves glycemic control in streptozotocin-induced diabetic rats. Journal of Functional Foods, 11, 235–242. https://doi.org/10.1016/J.JFF.2014.09.010
- Hubatsch, I., Ragnarsson, E. G. E., & Artursson, P. (2007). Determination of drug permeability and prediction of drug absorption in caco-2 monolayers. Nature Protocols, 2(9), 2111–2119. https://doi.org/10.1038/nprot.2007.303
- Karnjanapratum, S., O’Callaghan, Y. C., Benjakul, S., & O’Brien, N. (2016). Antioxidant, immunomodulatory and antiproliferative effects of gelatin hydrolysate from unicorn leatherjacket skin. Journal of the Science of Food and Agriculture, 96(9), 3220–3226. https://doi.org/10.1002/jsfa.7504
- Kouguchi, T., Ohmori, T., Shimizu, M., Takahata, Y., Maeyama, Y., Suzuki, T., Morimatsu, F., & Tanabe, S. (2013). effects of a chicken collagen hydrolysate on the circulation system in subjects with mild hypertension or high-normal blood pressure. Bioscience, Biotechnology, and Biochemistry, 77(4), 691–696. https://doi.org/10.1271/BBB.120718
- Koyama, Y.-I., Hayashida, O., Kuwaba, K., Takara, T., Kusubata, M., & Tsukada, Y. (2015). Supplemental ingestion of collagen peptide improves T-cell-related human immune status –placebo-controlled double-blind study–. Japanese Pharmacology & Therapeutics, 43(1), 51–56.
- Koyama, K. (2016). Effects of collagen ingestion and their biological significance. Journal of Nutrition & Food Sciences, 6. https://doi.org/10.4172/2155-9600.1000504
- Larder, C. E., Iskandar, M. M., & Kubow, S. (2021). Assessment of bioavailability after In vitro digestion and first pass metabolism of bioactive peptides from collagen hydrolysates. Current Issues in Molecular Biology, 43(3), 1592–1605. https://doi.org/10.3390/CIMB43030113
- Li, D. D., Li, W. J., Kong, S. Z., Li, S. D., Guo, J. Q., Guo, M. H., Cai, T. T., Li, N., Chen, R. Z., Luo, R. Q., et al. (2019). Protective effects of collagen polypeptide from tilapia skin against injuries to the liver and kidneys of mice induced by d-galactose. Biomedicine & Pharmacotherapy, 117, Article 109204. https://doi.org/10.1016/J.BIOPHA.2019.109204
- Li, Q., Wang, S., Poungchawanwong, S., & Hou, H. (2019). Effect of peptides from Alaska Pollock on intestinal mucosal immunity function and purification of active fragments. Nutrients, 11, Article 2517. https://doi.org/10.3390/NU11102517
- Liang, J., Pei, X. R., Wang, N., Zhang, Z. F., Wang, J. B., & Li, Y. (2010). Marine collagen peptides prepared from chum salmon (Oncorhynchus keta) skin extend the life span and inhibit spontaneous tumor incidence in sprague-dawley rats. Journal of Medicinal Food, 13(4), 757–770. https://doi.org/10.1089/JMF.2009.1279
- Liu, C., Chu, D., Kalantar-Zadeh, K., George, J., Young, H. A., & Liu, G. (2021). Cytokines: From clinical significance to quantification. Advanced Science (Weinheim, Baden-Württemberg, Germany), 8, Article 2004433. https://doi.org/10.1002/ADVS.202004433
- Liu, C., & Sun, J. (2019). Osteogenically differentiated mesenchymal stem cells induced by hydrolyzed fish collagen maintain their immunomodulatory effects. Life Sciences, 238, Article 116970. https://doi.org/10.1016/j.lfs.2019.116970
- Liu, C., & Sun, J. (2020). Modulation of the secretion of mesenchymal stem cell immunoregulatory factors by hydrolyzed fish collagen. Experimental and Therapeutic Medicine, 20, 375–384. https://doi.org/10.3892/etm.2020.8674
- Luckheeram, R., Zhou, R., Verma, A., & Xia, B. (2012). CD4 + T cells: Differentiation and functions. Clinical and Developmental Immunology, 2012, 1–12. https://doi.org/10.1155/2012/925135
- Mortarino, P. A., Goy, D. P., Abramson, D. B., Cabello, J., Bumaguin, G. E., Vitelli, E. J., Toledo, J., Sarrio, L., Pezzotto, S. M., Mardegan Issa, J. P., Cointry G.R., Feldman S. (2016). Emerging therapy in arthritis: Modulation of markers of the inflammatory process. Microscopy Research and Technique, 79(2), 89–97. https://doi.org/10.1002/jemt.22609
- Nieto, J., Soriano-Romaní, L., Tomás-Cobos, L., Sharma, L., & Budde, T. (2021). Improved in vitro bioavailability of a newly developed functionalized calcium carbonate salt as a food ingredient and its comparison with available commercial calcium salts. Food Chemistry, 348, Article 128740. https://doi.org/10.1016/J.FOODCHEM.2020.128740
- Nishikimi, A., Koyama, Y. I., Ishihara, S., Kobayashi, S., Tometsuka, C., Kusubata, M., Kuwaba, K., Hayashida, O., Hattori, S., & Katagiri, K. (2018). Collagen-derived peptides modulate CD4+ T-cell differentiation and suppress allergic responses in mice. Immunity, Inflammation and Disease, 6(2), 245–255. https://doi.org/10.1002/iid3.213
- Nomoto, T., & Iizaka, S. (2020). Effect of an oral nutrition supplement containing collagen peptides on stratum corneum hydration and skin elasticity in hospitalized older adults: A multicenter open-label randomized controlled study. Advances in Skin & Wound Care, 33(4), 186–191. https://doi.org/10.1097/01.ASW.0000655492.40898.55
- Ochi, T., Feng, Y., Kitamoto, S., Nagao-Kitamoto, H., Kuffa, P., Atarashi, K., Honda, K., Teitelbaum, D. H., & Kamada, N. (2016). Diet-dependent, microbiota-independent regulation of IL-10-producing lamina propria macrophages in the small intestine. Scientific Reports, 6(1). https://doi.org/10.1038/SREP27634
- Olson, G. B., Savage, S., & Olson, J. A. (2016). The effects of collagen hydrolysat on symptoms of chronic fibromyalgia and temporomandibular joint pain. The Journal of Craniomandibular & Sleep Practice, 18, 135–141. https://doi.org/10.1080/08869634.2000.11746125
- Opinion of the Scientific Panel on biological hazards (BIOHAZ) on the safety of collagen and a processing method for the production of collagen. (2005). EFSA Journal, 3. https://doi.org/10.2903/J.EFSA.2005.174
- Pollard, J. W. (2009). Trophic macrophages in development and disease. Nature Reviews Immunology, 9(4), 259–270. https://doi.org/10.1038/NRI2528
- Porfírio, E., & Fanaro, G. B. (2016). Collagen supplementation as a complementary therapy for the prevention and treatment of osteoporosis and osteoarthritis: A systematic review. Revista Brasileira de Geriatria e Gerontologia, 19(1), 153–164. https://doi.org/10.1590/1809-9823.2016.14145
- Sae-leaw, T., O’Callaghan, Y. C., Benjakul, S., & O’Brien, N. M. (2016). Antioxidant, immunomodulatory and antiproliferative effects of gelatin hydrolysates from seabass (lates calcarifer) skins. International Journal of Food Science & Technology, 51(7), 1545–1551. https://doi.org/10.1111/IJFS.13123
- Sato, K., Asai, T. T., & Jimi, S. (2020). Collagen-derived Di-peptide, prolylhydroxyproline (Pro-Hyp): A new low molecular weight growth-initiating factor for specific fibroblasts associated with wound healing. Frontiers in Cell and Developmental Biology, 8, Article 548975. https://doi.org/10.3389/FCELL.2020.548975
- Shigemura, Y., Kubomura, D., Sato, Y., & Sato, K. (2014). Dose-dependent changes in the levels of free and peptide forms of hydroxyproline in human plasma after collagen hydrolysate ingestion. Food Chemistry, 159, 328–332. https://doi.org/10.1016/J.FOODCHEM.2014.02.091
- Shigemura, Y., Suzuki, A., Kurokawa, M., Sato, Y., & Sato, K. (2018). Changes in composition and content of food-derived peptide in human blood after daily ingestion of collagen hydrolysate for 4 weeks. Journal of the Science of Food and Agriculture, 98(5), 1944–1950. https://doi.org/10.1002/JSFA.8677
- Si, S., Xu, B., Wu, Y., Qin, Y., Duan, R., & Song, S. (2020). [Collagen peptides improve lymphocyte distribution in peripheral blood and T lymphocyte proliferation in spleen of mice under the condition of simulated weightlessness]. Zhongguo shi yan xue ye xue za zhi, 28, 1001–1005. https://doi.org/10.19746/J.CNKI.ISSN.1009-2137.2020.03.047
- Si, S., Guo, Y., Xu, B., Qin, Y., & Song, S. (2021). Protective effects of collagen peptides on the dexamethasone-induced immunosuppression in mice. International Journal of Peptide Research and Therapeutics, 27(2), 1493–1499. https://doi.org/10.1007/S10989-021-10187-Y
- Song, W., Chen, Q., Wang, Y., Han, Y., Zhang, H., Li, B., & Yu, G. (2019). Identification and structure–activity relationship of intestinal epithelial barrier function protective collagen peptides from Alaska Pollock skin. Marine Drugs, 17(8), 450. https://doi.org/10.3390/MD17080450.
- Subhan, F., Kang, H. Y., Lim, Y., Ikram, M., Baek, S. Y., Jin, S., Jeong, Y. H., Kwak, J. Y., & Yoon, S. (2017). Fish scale collagen peptides protect against CoCl2/TNF- α-induced cytotoxicity and inflammation via inhibition of ROS, MAPK, and NF-κ B pathways in HaCaT cells. Oxidative Medicine and Cellular Longevity, 2017, 1–17. https://doi.org/10.1155/2017/9703609
- Yu, F., He, K., Dong, X., Zhang, Z., Wang, F., Tang, Y., Chen, Y., & Ding, G. (2020). Immunomodulatory activity of low molecular-weight peptides from nibea japonica skin in cyclophosphamide-induced immunosuppressed mice. Journal of Functional Foods, 68, Article 103888. https://doi.org/10.1016/J.JFF.2020.103888.
- Zdzieblik, D., Oesser, S., Baumstark, M. W., Gollhofer, A., & König, D. (2015). Collagen peptide supplementation in combination with resistance training improves body composition and increases muscle strength in elderly sarcopenic men: A randomised controlled trial. British Journal of Nutrition, 114(8), 1237–1245. https://doi.org/10.1017/S0007114515002810
- Zhang, Y., Kouguchi, T., Shimizu, K., Sato, M., Takahata, Y., & Morimatsu, F. (2010). Chicken collagen hydrolysate reduces proinflammatory cytokine production in C57BL/6.KOR-ApoEshl mice. Journal of Nutritional Science and Vitaminology, 56(3), 208–210. https://doi.org/10.3177/JNSV.56.208
- Zheng, J., Xie, W., Chen, Y., Qu, C., Xiao, Y., Kong, Y., Jin, G., Li, F., Jin, Q., Huang, X.-Z., Cheng, X., Jin, D. (2019). Dry cod skin collagen oligopeptides ameliorate ovalbumin-induced asthma in a mouse model via inhibition of NLRP3 inflammasome. International Journal of Clinical and Experimental Medicine, 12(8), 9548–9558.
- Zhu, S., Huang, M., Feng, G., Miao, Y., Wu, H., Zeng, M., & Lo, Y. M. (2018). Gelatin versus its two major degradation products, prolyl-hydroxyproline and glycine, as supportive therapy in experimental colitis in mice. Food Science & Nutrition, 6(4), 1023–1031. https://doi.org/10.1002/fsn3.639
- Zhu, S., Wu, L., Zhang, J., Miao, Y., Zhao, Y., Zeng, M., Li, D., & Wu, H. (2020). Collagen hydrolysate corrects anemia in Chronic kidney disease via anti-inflammatory renoprotection and HIF-2α-dependent erythropoietin and hepcidin regulation. Journal of Agricultural and Food Chemistry, 68(42), 11726–11734. https://doi.org/10.1021/ACS.JAFC.0C04459/SUPPL_FILE/JF0C04459_SI_001.PDF