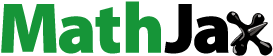
ABSTRACT
To develop a sensitive and specific ELISA for geosmin (GSM), the research of this study is focused on the design and synthesis of several GSM derivatives, which maintain the whole GSM structure as much as possible and contain a spacer arm with an active group at the end. To form the GSM backbone, tandem organic reaction strategies were used to replace the traditional Robinson cyclization reaction. Five GSM derivatives were synthesized and the formed GSM derivative-protein conjugates were used as the immunogens for the production of polyclonal antibodies against GSM, while four GSM derivatives were synthesized and the GSM derivative-protein conjugates were used as coating antigens for establishing ELISA. The relationship between the structures of GSM derivatives and the antibody properties was explored. Under optimal conditions, the LOD of the ELISA for GSM was found to be 0.16 ng mL−1, and the antiserum was able to specifically recognize the GSM backbone.
Introduction
Geosmin (GSM, ) is a small molecular compound resulting from the metabolites of filamentous bacteria (actinomycetes) and blue-green algae (cyanobacteria) overgrowing in the eutrophicated water body (Li et al., Citation2007; Lukassen et al., Citation2022; Zhang et al., Citation2016). GMS is one of the main earthy-musty odorants making the aquatic organisms and surface water strong smelly (Howgate, Citation2004; Lukassen et al., Citation2019). A high concentration of GSM in water bodies will not only decrease the taste and edible value of aquatic organisms and lead to a great economic loss for aquaculture but also has a negative effect on drinking-water safety (Bai et al., Citation2020; Doederer et al., Citation2019; Kim & Park, Citation2021). As the threshold value of GSM in fish and water bodies is very low (10 ng L−1) (Bai et al., Citation2013; Grimm et al., Citation2004; Smith et al., Citation2008), the development of sensitive and specific analytical methods for the detection of GSM is a great challenge.
Figure 1. Molecular structures of geosmin (a) and other four compounds used for testing specificity of the antibody (b–e).
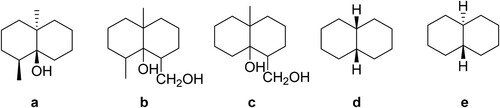
The common analytical method for GSM is gas chromatography (GC) with sample pretreatments such as solid phase extraction (SPE) or solid phase microextraction (SPME) (Bagheri & Salemi, Citation2006; Callejón et al., Citation2016; Cortada et al., Citation2011; Ma et al., Citation2007; Salemi et al., Citation2006). Although GC for GSM is reliable and sensitive, the disadvantages of GC, such as complicated performance, expensive instruments, high cost of testing, and the need for specialized technicians, etc., are also obvious. To reduce the detection cost and simplify the performance, it is required to develop a cheap, fast, sensitive and specific analytical method for the detection of GSM in aquatic organisms and water samples.
Based on the specific interaction between antibody and antigen, immunoassay, especially enzyme-linked immunosorbent assays (ELISA), has been widely used for the determination of small molecular compounds or macromolecules in clinical, environmental and food analytical fields (Cao et al., Citation2013; Jia et al., Citation2020; Liu et al., Citation2019; Wang et al., Citation2007; Wu et al., Citation2019). ELISA shows the properties of high sensitivity and specificity, simplicity, rapidity, high throughput and low cost of testing.
The first requirement to establish an ELISA for GSM is to produce the antibody against GSM. GSM is a small molecular compound called hapten, which is not able to stimulate the animal to produce the antibody against GSM. However, after being coupled to carrier proteins, the formed GSM-protein conjugates have sufficient immunogenicity, which can be used as immunogens for antibody production (Li et al., Citation2019; Xiong et al., Citation2020). Therefore, preparing GSM-protein conjugates is the key issue for the production of antibodies and the establishment of immunoassay. GSM contains a hydroxyl group, which may be used for linking to a carrier protein; however, the very high price of GSM (>100 US dollars/mg) makes it impossible to use GSM as the starting material for coupling to protein. In the last decades, researchers have made great efforts to synthesize different GSM derivatives from some cheap chemical substances as the starting materials (Chung et al., Citation1991). The synthesized GSM derivatives, containing a spacer arm with an active group at the end of the arm, were then coupled to carrier proteins. Nevertheless, the sensitivity and specificity of the antibodies that they produced were low, mainly because the GSM derivatives that they synthesized are analogues to GSM without maintaining the whole molecular structure of GSM.
To develop a cheap, rapid, sensitive and specific ELISA for GSM, the main research of in this study is focused on the design and synthesis of several GSM derivatives, which maintain the whole GSM structure as much as possible and contain a spacer arm with an active group at the end for coupling to a carrier protein. From normal chemical substances as the starting materials, the GSM backbone was constructed by tandem organic reaction strategies ( and ) instead of the traditional Robinson cyclization reaction (Figure S1) (Marshall & Hochstetler, Citation1966; Marshall & Hochstetler, Citation1968; Revial, Citation1989; Rieck et al., Citation1995). The synthetic methods that we proposed can directly construct functional groups on the GSM derivatives for linking while shortening the synthetic routing steps. The relationship between GSM derivative structures and antibody properties was explored.
Materials and methods
Reagents, solutions and apparatus
The chemically or analytically pure reagents used for synthesizing GSM derivatives (see Supporting Information) were purchased from Sigma-Aldrich (USA). Bovine serum albumin (BSA), ovalbumin (OVA), casein, 3,3,5,5-tetramethylbenzidine (TMB), N-hydroxysuccinimide (NHS), N,N′-dicyclohexylcarbodiimide (DCC), N,N-Dimethylformamide (DMF), geosmin solution at a concentration of 2 mg mL−1 (methanol solution), Freund’s complete and incomplete adjuvants were purchased from Sigma Co. (St. Louis, USA). The horseradish peroxidase-labelled goat anti-mouse IgG conjugate (HRP-GaMIgG) was purchased from Zhong Shan Golden Bridge Biological Technology Co. (Beijing, China).
The solutions used in this study are as follows: 0.01 mol L−1 phosphate buffer solution (PBS), pH 7.4; 0.05 mol L−1 Na2CO3-NaHCO3 buffer solution, pH 9.6; 1% casein as blocking solution; Substrate solution was prepared by adding 200 µL of 10 mg mL−1 TMB dissolved in DMSO, 3.5 µL of 30% H2O2 and 1 mL of acetate buffer to 20 mL of ultrapure water; Sulphuric acid (5%) was used to stop the enzymatic coloration.
Column chromatography silica gel is 300–400 mesh (Greagent, China). Avance NEO 300/400 NMR instrument (Brucker, Switzerland) for confirming the molecular structure was brought from Bruker Company using CDCl3, DMSO-d6 as the solvents (CIL, USA), and TMS as the internal standard. Small molecule masses were determined using a U3000-MSQPLUS liquid chromatography-mass spectrometer (ThermoFisher Scientific, Germany). The GSM-carrier protein conjugates were dried using an Alpha1-4LSCplus RC6 freeze dryer (Alpha, USA). Macromolecular mass was determined using UltrafleXtreme MALDI-TOF (Bruker, Switzerland). Pure water was prepared by Dura 12FV the deionized RO water supply system (The Lab Com, USA). Washing operations were performed using an ImmunoWash 1575 Microplate Washer (Bio-Rad, USA). Absorbance was measured using an A-5082 microplate reader (Tecan, Austria). ELISA was performed on 96-well microtiter plates (Corning, USA).
Synthesis of GSM derivatives
To synthesize GSM derivatives while maintaining the whole GSM molecular structure as much as possible, tandem organic reaction strategies ( and ) instead of the traditional Robinson cyclization reactions (Figure S1) were employed to form the GSM backbone. Using normal chemical reagents as the starting materials, nine GSM derivatives modified at different positions on the GSM backbone and containing different lengths of spacer arms with a carboxylic group (or amine group) at the end [ (6a, 6c); (7a, 7b, 7c, 7d, 9a, 9b,8)] were synthesized. Among them, in , 6a and 6c contains a shorter spacer arm (one-carbon-chain length) with a carboxylic group at the end, but modification positions of 6a and 6c are different. While in , all seven GSM derivatives contain a longer spacer arm but with different modification positions and different active groups at the end. The modification positions of 7a and 8 are the same, and the structures of the spacer arm are similar but with different active groups at the end (7a: carboxylic group; 8: amine group). On the other hand, the modification positions of the other five derivatives (7b, 7c, 7d, 9a, 9b) are the same with a carboxylic group at the end, but the structures of the spacer arm of these five derivatives are quite different. Compared to 7b, 7c, 7d, 9b, the structure of the spacer arm of 9a is very simple with a spacer arm of a four-atom length straight line. All the reaction processes for synthesizing the substances necessary for the modification [Figure S2 (2b, 2c)], intermediates [Figure S2 (3d, 4a, 4c, 4d, 5a, 5c)], the final reaction steps for nine GSM derivatives [ (6a, 6c); (7a, 7b, 7c, 7d, 8, 9a, 9b)], the NMR spectra (Figure S3) and NMR data for confirming the molecular structures were presented in Supporting Information (SI).
Synthesis of immunogens and coating antigens
Five GSM derivatives (6a, 6c, 7c, 7d, 9a) modified at different positions on the GSM backbone and containing different lengths of spacer arm with a carboxylic group at the end were selected for coupling to a carrier protein (BSA) (). The formed GSM derivative-BSA conjugates (P-A, P-B1–P-B4) were used as the immunogens for the production of polyclonal antibodies against GSM, while four GSM derivatives (6a, 7b, 7a, 8), modified at different positions on the GSM backbone and containing different lengths of spacer arms with a carboxylic group (or an amine group) at the end, were chosen for coupling to a carrier protein (OVA) (). It should be addressed that in the synthesis of 9b, due to very low synthetic yield, very small amount of 9b that we synthesized was used out in structure determination; therefore, no 9b was left for coupling to the carrier protein. The formed GSM derivative-OVA conjugates (P-C1, P-C2, P-C3, P-C4) were used as coating antigens for establishing indirect competitive ELISA. The syntheses of immunogens and coating antigens are detailed as follows.
Figure 4. Coupling of the GSM derivatives (6a, 6c, 7c, 7d, 9a) with BSA for the preparation of immunogens (P-A, P-B1, P-B2, P-B3, P-B4). N is the hapten/protein ratio in each GSM derivative-protein conjugate.
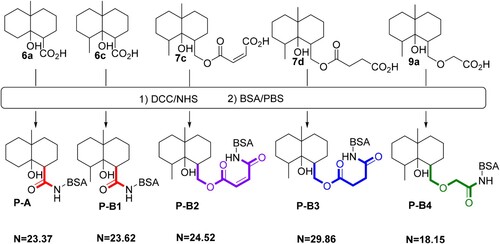
Figure 5. Coupling of the GSM derivatives (6a, 7b, 7a, 8) with OVA for the preparation of coating antigens (P-C1, P-C2, P-C3, P-C4). N is the hapten/protein ratio in each GSM derivative-protein conjugate.
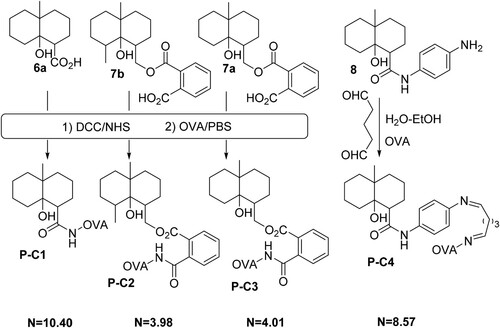
GSM derivatives, containing a carboxylic group at the end of the spacer arm (0.26 mmol dissolved in 1 mL of DMF), were added to 1 mL of 1,4-dioxane, followed by adding 171.67 mg of DCC and NHS. The reaction was carried out at 0–5°C for 1 h, and then raised to room temperature for 24 h. After the reaction, the mixture was centrifuged. 1 mL of the supernatant was added to the protein solution (1 μM BSA or OVA dissolved in 4 mL of 0.01 mol L−1 PBS, pH 7.4). The coupling reaction was performed at 4°C overnight. The solution was centrifuged at 4°C, and the supernatant was collected and dialyzed in 0.01 mol L−1 PBS, pH 7.4, for two to four days. After being lyophilized, GSM-BSA and GSM-OVA conjugates were obtained and stored at −20°C until use.
GSM derivatives, containing an amine group at the end of the spacer arm (16.2 mg of 8 dissolved in 3.5 mL of ethanol, supplemented with 2.5 mL of water), were added to 2.4 mL of the OVA solution (25 mg dissolved in 5 mL of water), followed by adding 84 μL of 25% pentane. The aqueous solution of dialdehyde reacted at 0–5°C for 2 h. After the reaction, the reaction temperature was raised to room temperature, and 424 μL of the stop solution (0.4 mmol of ethanolamine and 0.5 mmol of Na2CO3 dissolved in 1 mL of water) was added to terminate the reaction. The solution was centrifuged at 4°C, and the supernatant was collected and dialyzed in 0.01 mol L−1 PBS, pH 7.4, for two to four days. The solution was lyophilized, and GSM-OVA conjugate was stored at −20°C until use.
The molecular weights (MW) of BSA, OVA and GSM derivative-protein conjugates were determined by MALDI-TOF (Figures S4–S6). The linking radio of hapten/protein in GSM derivative-protein conjugates was calculated according to the equation: linking radio = (MW of conjugate – MW of protein)/(MW of GSM derivative – MW of water).
Production of polyclonal antibody
In this study, five GSM derivatives (6a, 6c, 7c, 7d, 9a) containing different lengths of spacer arm with a carboxylic group at the end were selected for coupling to BSA, and the formed GSM derivative-BSA conjugates (P-A, P-B1, P-B2, P-B3, P-B4) were used as the immunogens for the production of polyclonal antibodies against GSM (). Thirteen female BALB/C mice (six to eight weeks old, supplied by Experimental Animal Center of Soochow University) were subcutaneously immunized with five immunogens (). Among them, five mice were immunized with P-A, and the other eight mice were immunized with P-B1, P-B2, P-B3 and P-B4, . In the first immunization, the dose of the immunogen for each mouse was 100 µg of immunogen dissolved in physiological saline and emulsified with an equal volume of Freund’s complete adjuvant. In the next two to five booster immunizations, 100 μg of immunogen emulsified with the same volume of incomplete Freund’s adjuvant was given to each mouse at two to three weeks intervals. A small volume of blood was collected from the tail and evaluated by an indirect competitive ELISA. Bleeding of mice was noted 10 days after the last immunization and the collected antisera were stored at −20°C until use. The antisera at appropriate dilutions were used for the establishment of ELISA.
Table 1 . Thirteen mice immunized with five immunogens.
Animal welfare and experimental procedures were strictly carried out according to the recommendations in the Guide for the Care and Use of Laboratory Animals (23a) of the National Institutes of Health. All protocols were approved by the Institutional Animal Care and Use Committee (IACUC) of Soochow University. All efforts were made to minimize animal suffering and to reduce the number of animals used.
Procedures of ELISA
The procedures of ELISA assay were as follows. 200 μL/well of coating antigen (diluted with 0.05 mol L−1 of Na2CO3-NaHCO3 solution, pH 9.6) was added to a 96-well microtiter plate. The plate was kept at 4°C overnight. The plate was washed with a PBST buffer solution (0.01 M PBS buffer solution containing 0.01% Tween-20) three times and then blocked with 280 μL/well of 1% casein for 1 h at room temperature (r.t). The plate was washed with a PBST solution three times again, then 100 μL/well of mouse serum diluted with 0.01 mol L−1 PBS and 100 μL/well of GSM standard solutions (0 ng mL−1, 5000 ng mL−1) were added. The plate was slightly shaken for 1 h. After three times washing the plate, 200 μL/well of HRP-GaMIgG (1:3000 dilution in 0.01 mol L−1 PBS) was added to the plate and incubated for 1 h. Washing the plate again, 200 μL/well of TMB chromogenic solution was added to the plate. The plate was slightly shaken for 15 min for colour development. (6) 80 μL/well of 5% sulphuric acid was added to the plate to terminate the enzymatic reaction. (7) The plate was subjected to an ELISA reader for absorbance measurement.
Evaluation of antibody quality
In this study, inhibition ratio, e.g. (
: absorbance at 0 ng mL−1;
: absorbance difference between 0 ng mL−1 and 5000 ng mL−1), was employed to evaluate the antibody quality including the titer (e.g. suitable dilution of antiserum used in ELISA) and the immunoaffinity binding with GSM. The high value of
indicates the higher immunoaffinity binding of the antibody for GSM. For all antisera collected from 13 mice immunized with five immunogens, after evaluation, the antibody with the highest immunoaffinity binding for GSM was selected for constructing an ELISA standard curve.
Standard curve of the ELISA
GSM standard solutions (0, 0.2, 2.0, 20, 200, 2000 and 5000 ng mL−1) were applied to ELISA procedures, and the standard curve was constructed by plotting the inhibition ratio of against the logarithm of the concentration of GSM. The limit of detection (LOD) of the ELISA for GSM was calculated to form three times of standard deviation (SD) of the absorbances at zero concentration.
Results and discussion
Synthesis of GSM derivatives
To obtain a highly sensitive and specific antibody against the target analyte (hapten), in the synthesis of hapten derivative, the whole molecular structure of hapten should be maintained as much as possible. In addition, the location of the spacer arm attached to the hapten, the length of the spacer arm, the structure of the spacer arm, etc. should be carefully designed (Song et al., Citation2010). The location of the spacer arm attached to the hapten should not block the characteristic structure of the hapten to be exposed to the immune system of the animal. The length of the spacer arm should be appropriate. A shorter spacer arm can make the hapten be coupled to protein tightly, but the risk of the hapten being shielded by the neighbour structure in protein may increase. In contrast, a longer spacer arm can make the hapten be linked to protein flexibly, which may lead the hapten to be fallen into a cave of protein. The structure of the spacer arm should be simple. Complicated structure spacer arm in hapten derivative may result in a high possibility of the production of antibodies against spacer arm instead of hapten. However, in competitive immunoassay, if the hapten derivative synthesized is used for the preparation of coating antigen, to improve the sensitivity of the assay, the structure of the spacer arm in the hapten derivative should be different as much as possible from that used for the preparation of immunogen.
In this study, to synthesize GSM derivatives while maintaining the whole GSM molecular structure as much as possible, tandem organic reaction strategies ( and ) were employed to form the GSM backbone. Using normal chemical reagents as the starting materials, nine GSM derivatives, modified at different positions on the GSM backbone and containing different lengths of spacer arms with a carboxylic group (or amine group) at the end [ (6a, 6c); (7a, 7b, 7c, 7d, 9a, 9b, 8)], were synthesized. Among nine GSM derivatives, two GSM derivatives modified at different positions on the GSM backbone (6a, 6c) contain a short spacer arm with a carboxylic group at the end. While seven GSM derivatives (7a, 7b, 7c, 7d, 9a, 9b, 8) contain a longer spacer arm but with different modification positions and different active groups at the end.
As shown in , to form the GSM backbone, an intermediate (3a) was first synthesized. Under basic conditions, the starting material 1a (2-methylcyclohexanone) was changed to a carbanion and then reacted with halide 2a (the substances necessary for forming the GSM backbone) to form 3a. Under basic conditions, the ester carbonyl α-H in 3a has changed to a carbanion again and intramolecularly reacted with the ketone carbonyl to form 4a, which was then hydrolyzed to produce 6a. We also attempted to synthesize 6b and G18 but it was unsuccessful [(B, D); Figure S12].
We designed and successfully synthesized another GSM derivative (6c) containing a short spacer arm like that in 6a, but modified at different positions on the GSM backbone. As shown in (C), the starting substance was a cheap compound 1b. As the proton on the carbonyl α-H can be abstracted by an equivalent amount of stronger base LDA, 1b can react with 2a to form 3c, which can be further cyclized to form 4c by adding sodium hydride without isolation. However, the ring in 4c can be easily opened to form 3d in normal hydrolysis. Therefore, it is needed to reduce 4c to alcohol 5c by LiAlH4, and then 5c was oxidized to form 6c using PDC. Fortunately, through intermediate 5c, a new series of GSM derivatives (7b, 7c, 7d, 9a, 9b) can be produced ().
By the reduction of 4a, the intermediate (5a) can be obtained, which can be reacted with phthalic anhydride to form the GSM derivative of 7a (). In addition, by condensation of 6a with p-phenylenediamine, the GSM derivative (8) was also obtained ().
Preparation of immunogens and coating antigens
In this study, five GSM derivatives (6a, 6c, 7c, 7d, 9a) with different lengths of spacer arm attached to different positions of the GSM backbone were selected for the preparation of immunogens. The carboxylic group in GSM derivatives was activated by the DCC/NHS method and coupled to BSA to form five GSM-BSA conjugates (P-A, P-B1–P-B4, ), which were used as immunogens for antibody production.
Four GSM derivatives (6a, 7b, 7a, 8) were selected for coupling to OVA, and the formed GSM-OVA conjugates (P-C1, P-C2, P-C3, P-C4) were used as coating antigens for establishing competitive ELISA (). GSM derivatives (6a, 7b, 7a) contain a spacer arm with a carboxylic group at the end, thus they are coupled to OVA by the DCC/NHS method. While the GSM derivative (8) contains a spacer arm with an amine group at the end, thus it was coupled to OVA by the dialdehyde method. It was noted that, except for GSM derivatives (6a), due to the existence of the benzene ring, the structures of the spacer arms in GSM derivatives (7b, 7a, 8) are somewhat complicated compared to those in immunogens.
MALDI-TOF spectra of the GSM derivatives, BSA, OVA and GSM derivative-protein conjugates are presented in Figures S4–S6. The linking radios of hapten/protein in GSM derivative-protein conjugates were in the range of 4–30 ( and ), which indicates that GSM has been successfully coupled to carrier proteins.
Production of polyclonal antibody
GSM derivatives (6a, 6c, 7c, 7d, 9a) containing different lengths of a spacer arm with a carboxylic group at the end were selected for coupling to BSA, and the corresponding GSM derivative-BSA conjugates (P-A, P-B1, P-B2, P-B3, P-B4) were used as the immunogens to immunize 13 female BALB/C mice (). After five-time immunization, the sera from immunized mice (five mice immunized by P-A, other eight mice respectively immunized by P-B1, P-B2, P-B3, P-B4) were collected and kept at −20°C until use. The antisera were diluted with PBS at appropriate times and directly applied to ELISA procedures.
Evaluation of antibody quality
The polyclonal antisera from 13 immunized mice were collected and the quality of these antisera was primarily tested based on an indirect competitive ELISA procedure using checkerboard titration. Each coating antigen was diluted at different times (1:1000, 1:2000, 1:5000, 1:10,000) with coating buffers. The diluted coating antigen was added to a microtiter plate (200 μL/well) and kept at 4°C overnight. After the washing and blocking process, 100 μL/well of diluted antiserum (1:1000, 1:2000, 1:5000, 1:10,000) and 100 μL/well of GSM standard solution (0 ng mL−1 or 5000 ng mL−1) were added to the plate. After 1 h incubation at room temperature, the plate was washed and then 200 μL/well of GaMIgG-HRP diluted at 1:3000 was added to the plate. After 1 h incubation at room temperature and washing plate, 200 μL/well of the enzymatic substrate was added to the plate. After 20 min, the absorbances from the wells of the plate were measured. The inhibition ratio was used to evaluate the antibody quality.
Regarding the antisera (five sera from the mice immunized with P-A and two sera from the mice immunized with P-B1), it was found that the value was almost zero at any antibody/coating antigen combination, which indicates the poor quality of these antisera. In other words, these antisera display no specific immunoaffinity binding with GSM. The failure of the antibody production may mainly be due to the shorter spacer arm in GSM derivatives (6a, 6c) used for the preparation of immunogens (P-A, P-B1).
For other six antisera from the mice immunized with P-B2, P-B3 and P-B4, respectively, it was found that when P-C2 and P-C3 were used as the coating antigen, the value was in the range of 0.15–0.6, which demonstrates these antisera exhibit some extent specific immunoaffinity binding for GSM. However, the
value from the sera immunized with P-B3 and P-B4 was about three times higher than that from the sera immunized with P-B2. Higher specific immunoaffinity of the sera immunized with P-B3 and P-B4 may mainly be due to the simple structure and suitable length (four to six atom) of the spacer arm in GSM derivatives (7d, 9a) used for the preparation of immunogens (P-B3, P-B4), and the big difference between the spacer arm structure in immunogens and coating antigen. While lower specific immunoaffinity of the sera immunized with P-B2 may be relative to the structure of the spacer arm in GSM derivatives (7c, where the conjugation between carboxy group and C–C double bond existed) used for the preparation of immunogens (P-B2). In contrast, when P-C1 and P-C4 were used as the coating antigens, the
value was almost zero at any antibody/coating antigen combination, which proves that coating antigens also play an important role in the development of immunoassay.
Standard curve of the ELISA
As previously mentioned, the antisera immunized with P-B3 and P-B4 exhibit the high specific immunoaffinity binding for GSM. Thus, these two antisera were used for the construction of the ELISA standard curve. Further experiments confirmed that the sensitivity of the ELISA based on the serum of P-B4 and using P-C3 as coating antigen is a little higher than that of the ELISA based on the serum of P-B3. Under optimal experimental conditions (P-C3 as coating antigen at 1:2000 dilution; antiserum of P-B4 at 1:5000 dilution; GaMIgG-HRP at 1:3000 dilution), the standard curve of the ELISA for GSM is illustrated in . The LOD of the ELISA for GSM calculated from three times SD was found to be 0.16 ng mL−1, which was much lower than that in the published literature with the value of 1000 ng mL−1 (Chung et al., Citation1991).
Specificity of the antibody
Besides GSM, other four relative compounds [(b–e)] were selected for testing the specificity of the antibody (antiserum of P-B4). It was found that the value of the antiserum for the compounds in (b, c) was almost the same as that of the antiserum for GSM, and this
value was about four times higher than that of the antiserum for the compounds in (d, e). These results demonstrated that the antiserum of P-B4 can specifically recognize the GSM backbone, and the specificity achieved in this study was higher than that in published literature (Chung et al., Citation1991).
Conclusions
In this study, we designed and synthesized nine GSM derivatives that maintain the whole GSM molecular structure as much as possible using tandem organic reaction strategies instead of the traditional Robinson cyclization reaction. Among them, five GSM derivatives, in which the different length of the spacer arm was modified at different positions on the GSM backbone with a carboxylic group at the end, were used for the preparation of immunogens, while four GSM derivatives, in which the different length of spacer arm with different structure was modified at different positions on the GSM backbone with a carboxylic group or an amine group at the end, were used for the preparation of coating antigens. It is demonstrated that the length and structure of the spacer arm, the modification position, etc., will affect the antibody quality. In addition, coating antigens also play an important role in establishing ELISA. Under optimal conditions, the ELISA based on the antiserum of P-B4 (containing a four-atom length of spacer arm with a carboxylic group at the end) and coating antigen of P-C3 (in which the structure of spacer-arm is quite different compared to that in P-B4) exhibits the highest sensitivity and specificity for GSM. The results of the sensitivity and specificity obtained in this work were higher than those in the published literature.
Supplemental Material
Download MS Word (3.2 MB)Disclosure statement
No potential conflict of interest was reported by the author(s).
Additional information
Funding
References
- Bagheri, H., & Salemi, A. (2006). Headspace solvent microextraction as a simple and highly sensitive sample pretreatment technique for ultra trace determination of geosmin in aquatic media. Journal of Separation Science, 29(1), 57–65. https://doi.org/10.1002/jssc.200500182
- Bai, M. D., Huang, X. D., Zhong, Z. Q., Cao, M. J., & Gao, M. (2020). Comparison of OH and NaClO on geosmin degradation in the process of algae colonies inactivation at a drinking water treatment plant. Chemical Engineering Journal, 393(393), Article 123243. https://doi.org/10.1016/j.cej.2019.123243
- Bai, Z. W., Pilote, A., Sarker, P. K., Vandenberg, G., & Pawliszyn, J. (2013). In vivo solid-phase microextraction with in vitro calibration: Determination of off-flavor components in live fish. Analytical Chemistry, 85(4), 2328–2332. https://doi.org/10.1021/ac3033245
- Callejón, R. M., Ubeda, C., Ríos-Reina, R., Morales, M. L., & Troncoso, A. M. (2016). Recent developments in the analysis of musty odour compounds in water and wine: A review. Journal of Chromatography A, 1428, 72–85. https://doi.org/10.1016/j.chroma.2015.09.008
- Cao, B. Y., Yang, H., Song, J., Chang, H. F., Li, S. Q., & Deng, A. P. (2013). Sensitivity and specificity enhanced enzyme-linked immunosorbent assay by rational hapten modification and heterogeneous antibody/coating antigen combinations for the detection of melamine in milk, milk powder and feed samples. Talanta, 116(115), 173–180. https://doi.org/10.1016/j.talanta.2013.05.009
- Chung, S. Y., Vercellotti, J. R., Johnsen, P. B., & Klesius, P. H. (1991). Development of an enzyme-linked immunosorbent assay for geosmin. Journal of Agricultural and Food Chemistry, 39(4), 764–769. https://doi.org/10.1021/jf00004a030
- Cortada, C., Vidal, L., & Canals, A. (2011). Determination of geosmin and 2-methylisoborneol in water and wine samples by ultrasound-assisted dispersive liquid–liquid microextraction coupled to gas chromatography–mass spectrometry. Journal of Chromatography A, 1218(1), 17–22. https://doi.org/10.1016/j.chroma.2010.11.007
- Doederer, K., Gale, D., & Keller, J. (2019). Effective removal of MIB and geosmin using MBBR for drinking water treatment. Water Research, 149(149), 440–447. https://doi.org/10.1016/j.watres.2018.11.034
- Grimm, C. C., Lloyd, S. W., & Zimba, P. V. (2004). Instrumental versus sensory detection of off-flavors in farm-raised channel catfish. Aquaculture, 236(1–4), 309–319. https://doi.org/10.1016/j.aquaculture.2004.02.020
- Howgate, P. (2004). Tainting of farmed fish by geosmin and 2-methyl-iso-borneol: A review of sensory aspects and of uptake/depuration. Aquaculture, 234(1-4), 155–181. https://doi.org/10.1016/j.aquaculture.2003.09.032
- Jia, Q., Dahms, H.-U., & Wang, L. (2020). Detection of metallothionein proteins by enzyme-linked immunosorbent assay (ELISA). Current Pharmaceutical Biotechnology, 21(7), 544–554. https://doi.org/10.2174/1389201020666191127124629
- Kim, K. T., & Park, Y. G. (2021). Geosmin and 2-MIB removal by full-scale drinking water treatment processes in the Republic of Korea. Water, 13(5), 628. https://doi.org/10.3390/w13050628
- Li, L., Wan, N., Gan, N. Q., Xia, B. D., & Song, L. R. (2007). Annual dynamics and origins of the odorous compounds in the pilot experimental area of Lake Dianchi, China. Water Science and Technology, 55(5), 43–50. https://doi.org/10.2166/wst.2007.160
- Li, Q., Cui, Y., Liao, M., Feng, T., Tan, G., Wang, B., & Liu, S. (2019). A monoclonal antibody-based indirect competitive enzyme-linked immunosorbent assay for flubendiamide detection. Scientific Reports, 9(1), 1–10. https://doi.org/10.1038/s41598-019-38649-w
- Liu, C., Deng, D. D., Xu, D., Wu, K., Yang, H., Zhao, K., Li, J. G., & Deng, A. P. (2019). Development of a monoclonal antibody based-ELISA for the detection of chloramphenicol in shrimp, feed and milk samples and validation by LC-MS/MS coupled with immunoaffinity clean-up. Analytical Methods, 11(4), 507–516. https://doi.org/10.1039/C8AY02284D
- Lukassen, M. B., Jonge, N., Bjerregaard, S. M, Podduturi, R., Jørgensen, N. O. G., Petersen, M. A., David, G. S., Silva, R., & Nielsen, J. L. (2019). Microbial production of the off-flavor geosmin in tilapia production in Brazilian water reservoirs: Importance of bacteria in the intestine and other fish-associated environments. Frontiers in Microbiology, (10), 2447. https://doi.org/10.3389/fmicb.2019.02447
- Lukassen, M. B., Menanteau-Ledouble, S., Jonge, N., Schram, E., & Nielsen, J. L. (2022). Impact of water quality parameters on geosmin levels and geosmin producers in European recirculating aquaculture systems. Journal of Applied Microbiology, 132(3), 2475–2487. https://doi.org/10.1111/jam.15358
- Ma, X. Y., Gao, N. Y., Chen, B. B., Li, Q. S., Zhang, Q. L., & Gu, G. F. (2007). Detection of geosmin and 2-methylisoborneol by liquid-liquid extraction-gas chromatograph mass spectrum (LLE-GCMS) and solid phase extraction-gas chromatograph mass spectrum (SPE-GCMS). Frontiers of Environmental Science & Engineering in China, 1(3), 286–291. https://doi.org/10.1007/s11783-007-0048-7
- Marshall, J. A., & Hochstetler, A. R. (1966). Oxygenation of 1, 10-dimethyl-1 (9)-octalin. The Journal of Organic Chemistry, 31(4), 1020–1025. https://doi.org/10.1021/jo01342a008
- Marshall, J. A., & Hochstetler, A. R. (1968). Synthesis of (+-)-geosmin and the other 1, 10-dimethyl-9-decalol isomers. The Journal of Organic Chemistry, 33(6), 2593–2595. https://doi.org/10.1021/jo01270a120
- Revial, G. (1989). Asymmetric Michael-type alkylation of chiral imines. Enantioselective syntheses of (−)-geosmin and two other related natural terpenes, as well as enant-(+)-geosmin. Tetrahedron Letters, 30(31), 4121–4124. https://doi.org/10.1016/S0040-4039(00)99338-9
- Rieck, A., Bülow, N., & König, W. A. (1995). An epoxy-trinoreudesmane sesquiterpene from the liverwort Lophocolea bidentata. Phytochemistry, 40(3), 847–851. https://doi.org/10.1016/0031-9422(95)00355-B
- Salemi, A., Lacorte, S., Bagheri, H., & Barceló, D. (2006). Automated trace determination of earthy-musty odorous compounds in water samples by on-line purge-and-trap–gas chromatography–mass spectrometry. Journal of Chromatography A, 1136(2), 170–175. https://doi.org/10.1016/j.chroma.2006.09.087
- Smith, J. L., Boyer, G. L., & Zimba, P. V. (2008). A review of cyanobacterial odorous and bioactive metabolites: Impacts and management alternatives in aquaculture. Aquaculture, 280(1-4), 5–20. https://doi.org/10.1016/j.aquaculture.2008.05.007
- Song, J., Wand, R. M., Wand, Y. Q., Tang, Y. R., & Deng, A. P. (2010). Hapten design, modification and preparation of artificial antigens. Chinese Journal of Analytical Chemistry, 38(8), 1211–1218. https://doi.org/10.1016/S1872-2040(09)60063-3
- Wang, N., He, M., & Shi, H. C. (2007). Novel indirect enzyme-linked immunosorbent assay (ELISA) method to detect total E. coli in water environment. Analytica Chimica Acta, 590(2), 224–231. https://doi.org/10.1016/j.aca.2007.03.041
- Wu, L., Li, G. H., Xu, X., Zhu, L., Huang, R. M., & Chen, X. Q. (2019). Application of nano-ELISA in food analysis: Recent advances and challenges. Trends in Analytical Chemistry, 113(113), 140–156. https://doi.org/10.1016/j.trac.2019.02.002
- Xiong, Y., Leng, Y., Li, X., Huang, X., & Xiong, Y. (2020). Emerging strategies to enhance the sensitivity of competitive ELISA for detection of chemical contaminants in food samples. TrAC Trends in Analytical Chemistry, 126(126), 115861. https://doi.org/10.1016/j.trac.2020.115861
- Zhang, Y. C., Zhang, N., Xu, B. B., Kumirska, J., & Qi, F. (2016). Occurrence of earthy–musty taste and odors in the Taihu Lake, China: Spatial and seasonal patterns. RSC Advances, 6(83), 79723–79733. https://doi.org/10.1039/C6RA16733K