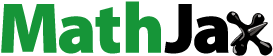
ABSTRACT
The simple and rapid identification of meat via DNA-based methods is still subject to the conditions of complex and time-consuming preprocessing. It usually takes 3–4 h to obtain DNA sample using conventionally available DNA extraction kits. The existing approaches are cumbersome, require large equipment, and are not suitable for field operation. This study developed a swelling microneedle patch, constituted by micron-sized copolymers of methyl vinyl ether and maleic acid (PMVE/MA). The microneedle patch was applied to the meat sample for 1 min before being pulled out and rinsed with Tris-EDTA (TE) buffer to obtain DNA, which could be used for polymerase chain reaction (PCR) without purification. The DNA extracted by the microneedle patch could meet the needs for amplification and identification of meat samples. The developed approach which combines the patch-based extraction method and conventional PCR amplification is quick, simple, and reliable for DNA extraction and identification of meat samples.
1. Introduction
In the food industry, people’s diet structure and level are gradually changing, and the demand for meat is further increasing (Beibei Wang, Citation2012; Kesmen et al., Citation2009). The consumer market for meat such as mutton is also expanding, but prices have risen due to the current epidemic and other factors (Dayu Liu, Citation2019). Therefore, it is not uncommon for some businesses or enterprises to use relatively cheap duck, pork, chicken, etc. as mutton or make adulterated mutton to maximise profits (Ballin et al., Citation2009; Jingxin Zhang, Citation2015; Li & Guan, Citation2019; Xu et al., Citation2016). The spread of fake mutton not only disturbs the market order but also damages the rights and interests of farmers and consumers (Xiaofang Wu, Citation2021). Hence, simple and rapid methods to extract DNA for meat adulterant detection will aid in resolving the current problem.
Since the advent of instrumental analysis technology, loop-mediated isothermal amplification (LAMP), polymerase chain reaction (PCR) and recombinase polymerase amplification (RPA) techniques have been used to identify meat products (Kang et al., Citation2022). LAMP has high primer requirements, and each reaction needs to design four special primers, which is difficult to be generalised to daily detection applications (Notomi et al., Citation2000), the price of RPA is relatively high and the terminal detection is relatively complicated (Dayu Liu, Citation2019), PCR has become the method of choice for meat species identification in medicine inspecting institutes and other testing facilities (Kumar et al., Citation2015). The test results have high timeliness and precision, due to their traits of strong specificity and high sensitivity (Nehal et al., Citation2021; Nicoloso et al., Citation2013). However, despite the above advantages of PCR detection methods, their application in the rapid and simple detection of meat foods is still constrained by complex and time-consuming pretreatment (Cao et al., Citation2018). It typically takes 3–4 h to obtain a DNA sample for analysis, using conventionally available DNA extraction kits (Li et al., Citation2021; Wang, Shen, et al., Citation2021; Xiujuan Wang, Citation2015). Currently, no optimal method is available for directly extracting DNA for the detection and identification of adulterated mutton. Developing an alternative technique for rapid DNA extraction can significantly reduce the total time required for DNA-based identification methods, which will also be useful in detecting fake mutton quickly. Therefore, developing a quick, practical process for extraction is critical to ensuring food safety in local and global markets. These markets require not only qualitative species identification but also quantitative detection of components in meat products.
Hydrogel microneedle patches consist of a series of micron-sized needles arranged in an orderly manner on the gel basal layer for rapid collection of desired biomarkers from biological tissues by in-situ expansion-driven capillary force (Chang et al., Citation2017; Mukerjee et al., Citation2003; Wang et al., Citation2005). The method of extracting skin interstitial fluid samples based on hydrogel microneedle patches can be further used for the detection of target DNA and miRNA (Al et al., Citation2019; Kim et al., Citation2022; Li et al., Citation2022; Qiao et al., Citation2022). In addition, the study by Paul et al. found that microneedle patches can rapidly extract pathogen DNA within 1 min, enabling direct molecular diagnosis of plant diseases in the field (Paul et al., Citation2019, Citation2020, Citation2021). According to the study of Li et al., rapid and on-site detection of allergen DNA in food can be achieved through the rapid extraction of microneedle patches combined with the RPA method (Li et al., Citation2021). Kight et al. successfully captured and detected Borrelia burgdorferi directly from the skin using PVA microneedle patches (Kight et al., Citation2022). Microneedle patches offer several benefits in the rapid collection of biomarkers from biological tissues without the need for bulky and complex laboratory equipment (Chang et al., Citation2017). To the best of our knowledge, there has not been a report on the use of microneedle patches to extract DNA from meat for identification.
The present study introduces a rapid DNA extraction method based on copolymers of methyl vinyl ether and maleic acid (PMVE/MA) microneedle patch, combined with PCR amplification technology, for the rapid qualitative and quantitative detection of meat foods (). The DNA extraction ability of the PMVE/MA microneedle patch was compared with DNA extracted using commercial DNA kits from meat samples such as mutton, pork, duck, and chicken. By simply pressing and peeling off the microneedle patch on the surface of the meat sample, the DNA was extracted. It was then rinsed out of the microneedle patch and subjected to PCR analysis. The results showed that the microneedle patch could successfully extract meat DNA with good quality and was suitable for rapid DNA-based identification methods for meat food safety.
2. Materials and methods
2.1. Materials
Copolymers of methyl vinyl ether and maleic acid (PMVE/MA, molecular weight 1080 kDa), poly (vinyl alcohol) (PVA, molecular weight 61 kDa) and sodium bicarbonate (NaHCO3) were purchased from Sigma. (St. Louis, MO, USA). Polyethylene glycol 10000 (PEG-10000), Carbopol® 974P, and SYBR Green I were purchased from Solarbio Biotech Co. LTD (Beijing, China). Fresh mutton, pork, chicken, duck, and mutton rolls were purchased from local supermarkets. (Bengbu, China). Commercial animal tissue DNA kits and fluorescent PCR detection kits for animal-sourced DNA – (mutton, pork, duck, and chicken) were purchased from Simgen. (Hangzhou, China). Twist AmpTM Basic RPA kit was purchased from Twist Amp. (Cambridge, UK), dNTPs from Beyotime, (Shanghai, China) and the primers used in RPA were purchased from Titan. (Shanghai, China).
2.2. Preparation of microneedle patches
The specific method used 20% w/w PMVE/MA and 7.5% w/w PEG-10000, followed by the addition of 3% w/w NaHCO3 to form a hydrogel solution. Then, 0.2 mL was added to the 10 × 10 polydimethylsiloxane (PDMS) mould, and the whole mould was filled by centrifugation at 3500 rpm for 15 min. Then the mould was dried at room temperature for 48 h, and the cross-linking was induced by an esterification reaction at 80°C for 24 h (Paul et al., Citation2004). The microneedle patch was then carefully removed from the mould and stored in a closed drying dish for future use (). Following the procedure outlined above, microneedle patches with varying concentrations (1%, 1.5%, 2%, and 3%) of NaHCO3, Carbopol® 974P, and other excipients in PMVE/MA were prepared to compare their performance (Sabri et al., Citation2022). PVA microneedle patches were prepared in accordance with previous reports (Li et al., Citation2021; Zhu et al., Citation2020).
2.3. Swelling properties of microneedle patches
Specific swelling experiments were performed as follows: First, the microneedle patches were weighed in the dry state (M0). The microneedle patches were then immersed in 5 mL phosphate-buffered saline (PBS) solution (pH = 7.4) for 1 min. The weight of the microneedle patch (Mt) was recorded after the liquid on its surface was carefully removed with filter paper. The weight change of the microneedle patch before and after swelling was calculated (ΔM = Mt–M0). Similarly, the microneedle patch was removed after soaking at different time intervals (2, 3, 4, 5, 10, 60, 120, 300, and 360 min), and the weight change was calculated as described above. The swelling ability of the microneedle patch prepared with varying concentrations of NaHCO3 solutions was also investigated. Following the prior study, the swelling ability of the PMVE/MA microneedle patch was examined while substituting 3% Na2CO3 with 3% NaHCO3 (Donnelly et al., Citation2014).
The swelling rate of the microneedle patch can be calculated by Equation (1):
(1)
(1)
2.4. Mechanical properties of microneedle patches
The microneedle patch was pressed into the surface of the sample for 1 min before being removed. The morphological changes of the microneedle patch as well as its penetration efficiency on the surface of the sample to be tested were observed.
2.5. Sample preparation and DNA extraction
Without any pretreatment, the microneedle patch was used to directly extract DNA from the tested meat samples. In general, the volume of the sample to be tested should be larger than the microneedle patch. The DNA was extracted by pressing the microneedle patch on the meat sample for 1 min, then stripping and rinsing with 100 μL Tris-EDTA (TE) buffer (pH = 8.0) to collect the adsorbed DNA. The UV spectra of DNA samples were measured by an Ultra Micro UV Visible Spectrophotometer (Thermo Fisher, USA).
Specific operational steps were followed for DNA extraction using commercial kits. Briefly, meat samples weighing ≤25 mg were cut and minced to a homogenate before being transferred to a 1.5 mL centrifuge tube. Then 20 μL proteinase K storage solution was added, followed by the addition of 180 μL preheated AT Buffer (Protein digester) at 56°C. The homogenate was dispersed by vortexing for several seconds before being placed in a 56°C water bath for 10–30 min, during which the tissue was dissolved by vortexing several times. After that, 200 μL of SL Buffer (Nucleic acid binding solution) was added and vortexed for 15 s for mixing. The centrifuge tube was placed in a water bath at 70°C for 10 min. 200 μL absolute ethanol was added and thoroughly mixed by vortexing for about 15 s. The solution in the above steps was sucked and added to the nucleic acid purification column and centrifuged at 12,000 rpm for 20 s. The filtrate was discarded, and 500 μL of WA Buffer (Deproteinized solution) was added to the nucleic acid purification column followed by centrifugation at 12,000 rpm for 30 s. Again, the filtrate was discarded and 600 μL of WB Buffer (Desalting solution) was added to the nucleic acid purification column, followed by centrifugation at 12,000 rpm for 30 s. The filtrate was discarded, and the nucleic acid purification column was subjected to centrifugation at 14,000 rpm for 1 min. Subsequently, 100–200 μL of TE Buffer incubated at 56°C was added to the purification column and allowed to rest for 1 min at room temperature followed by centrifugation at 12,000 rpm for 30 s to elute the DNA. The UV spectra of DNA samples were measured by an Ultra Micro UV Visible Spectrophotometer (Thermo Fisher, USA).
2.6. qRT-PCR assay
The primers used in this experiment were mixed in the PCR main reaction solution provided by the manufacturer. The PCR reaction volume was 40 μL, which was constituted of 34.6 μL PCR main reaction solution, 0.4 μL Taq enzyme, and 5 μL DNA template (obtained from the microneedle patch or kit extraction solution). PCR reactions were carried out on a real-time system (Roche Light Cycler 480), and fluorescence signals were collected for each cycle after the extension phase. Cycle threshold (Ct) values were used to reflect the initial content of the target DNA. The PCR amplification products were subjected to gel electrophoresis (1.5% agarose) for subsequent analysis.
2.7. RPA assay
Cao screened five primer sequences that could be used for both PCR and RPA (Cao, Citation2017). Table S1 enlists the primer sequences used. The RPA method was performed following the manual instructions provided by the Twist Amp Basic kit. Briefly, 25 μL 2 × Reaction Buffer, 5 μL 10 × Basic E-mix, 2.4 μL each primer (10 μM), 2.5 μL 20 × Core Reaction Mix were added to each reaction tube, followed by 9.2 μL dNTPs, and water. The reaction mixture was shaken well and briefly centrifuged. To ensure that all systems reacted simultaneously, 2.5 µL of 280 mM MgOAc and 1 µL template were added, mixed thoroughly (6 times inverted), and then the reaction was started immediately at 37°C for 30 min. The RPA conditions were kept the same, and the negative control was blank microneedle patches washed with TE buffer but with no DNA extracted from the meat samples. The RPA products were purified and loaded on 1.5% agarose gels, wherein, 2 μL 400 × SYBR Green I fluorescent dye was added to the system for visualisation and the colour development results were observed under the irradiation of an ultraviolet lamp.
3. Results and discussion
3.1. Performance evaluation of microneedle patch
The patch constituted a 10 × 10 microneedle array with a height of 850 μm for each needle. A single microneedle patch needle has a radius of 150 μm at its base and 5 μm at its tip (D). A morphological diagram of the differently fabricated microneedle patches is shown in A–C.
Figure 3. Morphological diagram of the differently fabricated microneedle patches. (A) PVA microneedle patch, (B) PMVE/MA microneedle patch with 3% NaHCO3 incorporation, (C) PMVE/MA microneedle patch with Carbopol® 974P incorporation, (D) Needle morphology of the PMVE/MA microneedle patch with 3% NaHCO3 incorporation.
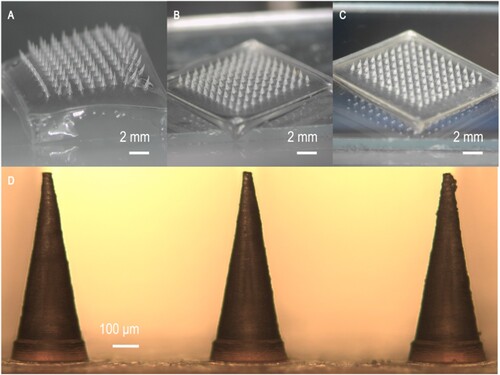
The results in show the swelling percentage of each swelling microneedle patch in PBS (pH = 7.4). The swelling percentage of the microneedle patch prepared by 20% w/w PMVE/MA, 7.5% w/w PEG, and 3% w/w NaHCO3 is 908% ± 20% after 1 h (A). Additionally, within the first 10 min, the swelling percentage of the microneedle patch can reach 445% ± 19% (C). In contrast, the swelling percentage of the microneedle patch with Carbopol® 974P and with 1% w/w NaHCO3 is only 257% ± 20% and 353% ± 14% respectively (A and B). Ester-based crosslinking is reduced after the addition of NaHCO3 excipient. Nonetheless, after cross-linking, the PMVE/MA gel can quickly absorb water and expand in a short time, absorbing 100% of its weight within a minute or so (Donnelly et al., Citation2014). The PMVE/MA microneedle patches with 3% NaHCO3 were chosen for further experiments due to their relatively high swelling ability. This is a cost-effective and efficient absorbent polymer with strong chemical resistance, mechanical strength, and good biocompatibility (Chang et al., Citation2017; Eltayib et al., Citation2016; Raj et al., Citation2010). One of the main forces that aggregate intracellular DNA molecules around the tip of the microneedle is thought to be expansion-driven capillary flow (Paul et al., Citation2019). Furthermore, the microneedle patch can be easily created in an ordinary laboratory without the special requirement for a clean room. The microneedle patch remained intact after infiltrating the meat sample, indicating that the microneedle patch had good mechanical properties ().
Figure 4. Swelling ability of the differently fabricated microneedle patches. (A) Swelling rate of PMVE/MA microneedle patches supplemented with different concentrations of NaHCO3 (n = 3), (B) Swelling rate of PMVE/MA microneedle patches supplemented with Carbopol® 974P (n = 3), (C) Swelling ability of PMVE/MA microneedle patches with 3% NaHCO3 for 10 min (n = 3).
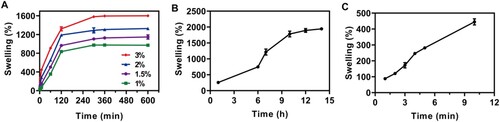
3.2. Evaluation of DNA extraction capability of microneedle patch
In this experiment, mutton, pork, chicken, and duck meat were used as model samples to assess the DNA extraction ability of PMVE/MA microneedle patches. The characteristic absorbance of the DNA extracted from the microneedle patch and commercial kit was observed at 260 nm (). The characteristic UV absorption peaks of DNA, proteins, and polysaccharides are found at 260, 280, and 230 nm, respectively. Therefore, the ratios of A260/A280 and A260/A230 are frequently used as quick indicators of DNA purity against proteins and polysaccharides, respectively (Varma et al., Citation2007).
Figure 6. The UV absorption spectra of the samples extracted by the commercial kit and microneedle patches from (A) Mutton, (B) Pork, (C) Duck, (D) Chicken (n = 3).
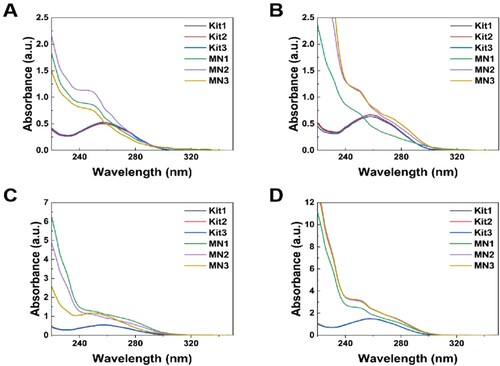
For the mutton, pork, duck, and chicken samples, the total amount of DNA extracted by the kit and the microneedle patch were 5026.67 ± 122.57 and 2688.00 ± 460.63 ng, 6520.00 ± 155.78 and 3024.00 ± 765.93 ng, 5338.60 ± 68.19 and 4471.11 ± 350.78 ng, 14,774.87 ± 60.37 and 9735.90 ± 1019.30 ng respectively. The study and subsequent electrophoresis experiments demonstrated that the microneedle patch requires a smaller sample volume than a commercial kit to extract DNA, without affecting the subsequent PCR experiments. Even though the total amount of DNA extracted with the commercial kit was higher, the microneedle patch required less sample extraction and extracted significantly more DNA per milligram of sample than the commercial kit (). According to the existing literature reports. Meat tissue contains a large amount of interstitial fluid that contains a variety of biomarkers (e.g. protein, cells, nucleic acids) (Xiao et al., Citation2023). The tip of the microneedle patch we prepared is only 5 μm and can easily Pierce the cell wall of the sample, and through the swelling ability of the microneedle patch, it is easier to collect DNA inside the cell (Paul et al., Citation2019).
Figure 7. The sample amount (mg) and DNA per mg (ng/mg) extracted from each meat by using two different methods. (A) Mutton, (B) Pork, (C) Duck, (D) Chicken (n = 3).
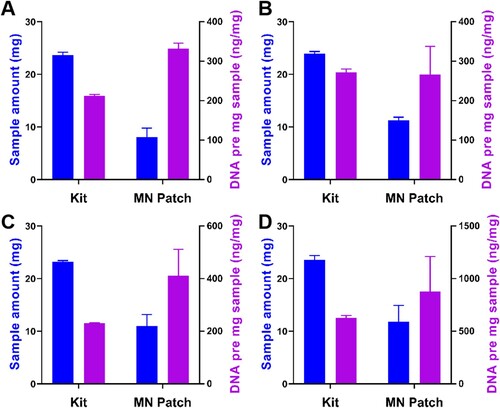
The DNA extracted using microneedle patches does not require a laborious purification process similar to that of commercial kits (Guha et al., Citation2018; Kim et al., Citation2009). Therefore, the DNA extracted by the two methods was subjected to qRT-PCR analysis to evaluate whether the DNA extracted by microneedle patches was of adequate quality for direct amplification. The amplified products were electrophoresed in agarose gel, and the results showed that the corresponding characteristic bands of mutton, pork, duck, and chicken were found in the DNA samples extracted using a microneedle patch or a commercial kit. The mutton was 98 bp, the pork was 70 bp, the duck was 85 bp, and the chicken was 90 bp (A–D). The above results indicate that the microneedle patch had DNA extraction ability as adequate as the commercial kit. The quality of DNA extracted from a microneedle patch can be directly amplified by PCR. In addition, the Ct value denotes the number of cycles experienced when the fluorescence signal in each PCR reaction tube reaches the set threshold value. There is a linear relationship between the Ct value of each template and the logarithm of the template’s initial copy number. The more the initial copy number, the smaller the Ct value, and vice versa (Higuchi et al., Citation1993). For these four kinds of meat samples, the difference in the Ct value of DNA extracted by the microneedle patch and kit is about 10–15 cycles (E–H). It is observed that there is a significant difference between the DNA extracted by the microneedle patch and the kit. It might be because the absolute content of DNA extracted by the microneedle patch is relatively small compared with the kit. This was verified in the subsequent quantitative experiments. Nevertheless, this difference will not have a significant impact on the judgment of the results. Therefore, the microneedle patch-based DNA extraction can be used as an alternative to commercial DNA extraction kits because it greatly simplifies the routine operation procedures (such as tissue dissolution, multi-step reagent purification, and centrifugation, etc.), shortens operation time and improves the sample identification efficiency (Wang et al., Citation2011; Yalcinkaya et al., Citation2017). Furthermore, it has been reported that the compounds commonly used in DNA extraction procedures (such as alcohols, detergents, and lysozyme) have potential denaturing adverse effects. The microneedle-based extraction methods do not require the involvement of organic solvents, implying that the microneedle patch-based approach can be used as a relatively mild alternative to DNA extraction (Rossen et al., Citation1992).
Figure 8. Qualitative and quantitative analysis of mutton, pork, duck, and chicken DNA. Gel electrophoresis plots showing the amplified bands of (A) mutton (B) pork (C) duck (D) chicken. Lanes 1–3: DNA extracted from the microneedle patch, lane 4: positive control, lane 5: DNA extracted from the commercial kit, (A) left M: 15,000 bp DNA ladder, right M: 500 bp DNA ladder, (B-D) M: 500 bp DNA ladder. (E-H) Ct values in qRT-PCR for genes of the (E) mutton, (F) pork, (G) duck, (H) chicken.
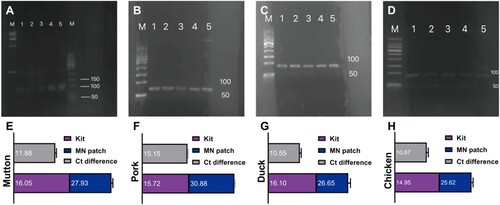
3.3. Application of microneedle patch method in practical detection
Four kinds of meat DNA samples were continuously diluted in the concentration range of 10 ng/μL–1 pg/μL to quantitatively compare the DNA extracted by the two methods. The linear relationship between the logarithm of DNA concentration and cycle threshold was obtained, which was used as the calibration curve for the determination of DNA concentration (). For further quantitative analysis, the PCR method was used. The actual four meat sources (mutton, pork, duck, chicken) DNA concentration () measured by the microneedle patch extraction method and commercial kit extraction method was compared. The concentration of DNA extracted by the microneedle patch was slightly lower than that of the conventional kit method, resulting in a slightly longer amplification reaction time and higher Ct value. The threshold of the microneedle patch extraction method was approximately 10 cycles higher than that of the conventional method. To further validate the feasibility of the microneedle patch DNA extraction method in daily life, the two types of mutton rolls were randomly purchased from the local supermarket. The first is labelled as containing only mutton, and the second is labelled as consisting of mutton and pork. Following the outlined procedures, the two methods of DNA extraction, and subsequent qRT-PCR experiments were performed. It should be noted that to prevent the purchase of lamb rolls containing meat outside the ingredients list, the four kits for qRT-PCR experiments were employed on mutton, pork, duck, and chicken. Only mutton ingredients were detected in the first mutton roll, and the Ct values measured by the two methods were brought into the mutton concentration calibration curve, indicating that the purity of this mutton roll was high (A). The two methods of DNA extraction were used to detect the presence of mutton and pork in the second mutton roll. By measuring the Ct value, a large proportion of pork was ascertained in this second mutton roll (B). The results obtained only by the microneedle extraction method were enough to draw the above conclusions.
Figure 9. Standard curve for Ct values measured by qRT-PCR for (A) mutton, (B) pork, (C) duck, (D) chicken.
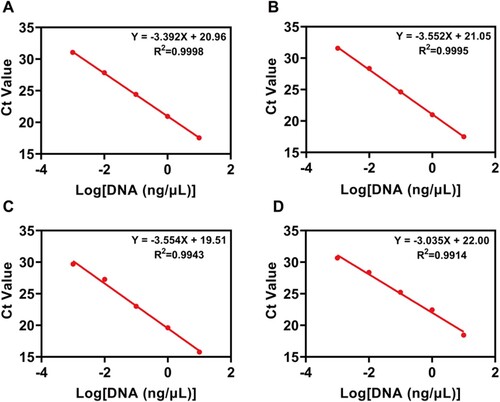
Figure 10. Application of the microneedle patches and commercial kit extraction methods for analysing commercial samples (A-B) Comparison of Ct values of the two detection methods in commercial lamb roll samples.
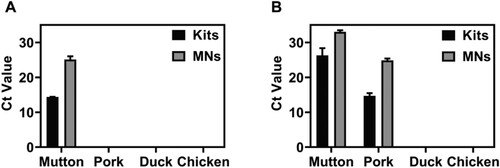
Table 1. Ct value and DNA concentration obtained by two extraction methods (n = 3).
In summary, by pressing the microneedle patch on the meat sample for one minute, the microneedle patch can obtain a sufficient amount of DNA without purification. This avoids the complex and time-consuming shortcomings of traditional kit extraction methods (Petya et al., Citation2013) and greatly improves work efficiency. Next, the combination of the extraction method based on a microneedle patch and PCR amplification detection method can realise the identification of adulterated meat, and a rapid, simple and reliable method for DNA extraction and identification of meat samples is established.
3.4. Exploration on future research direction of microneedle patch method
Additionally, even though PCR is a routine nucleic acid identification method, it still necessitates a professional operator to run sophisticated tools and equipment, as well as an hour or more of processing time and analysis. The RPA, as a new nucleic acid detection technology that can carry out the reaction at 37∼42°C, is gradually replacing PCR due to its advantages of being fast, sensitive, and accurate (Chandu et al., Citation2016; Li et al., Citation2019). Li et al. developed a rapid field test for food allergen genes using microneedle extraction and RPA technologies (Li et al., Citation2021). Wang et al. combined RPA with lateral flow strips (LFS) to achieve the rapid detection of Candida albicans (Wang, Ge, et al., Citation2021). Similarly, in the present study, the rapid identification of meat was carried out by combining microneedle technology with RPA. Figure S1 shows the RPA amplification of DNA samples based on the microneedle patch extraction method. The observed mutton genetic characteristic bands (David et al., Citation2018), confirm that the microneedle patch extracted DNA samples can be subjected to RPA amplification. SYBR Green I fluorescent dye was added to the purified RPA products for visual analysis to further verify the feasibility of this method in field detection (Piepenburg et al., Citation2006; Wang et al., Citation2017). Despite the ideal fluorescence in the sample tube, the blank control also exhibits weak fluorescence interference, which affects the determination of the results. This could be due to the dimer in the RPA reaction process affecting the subsequent colour development experiment. Future studies will involve appropriate modification of the primer to achieve the prospect of effective on-site visual detection.
4. Conclusions
In summary, a PMVE/MA-based microneedle patch was fabricated for real-time DNA extraction from four kinds of meat samples i.e. mutton, pork, duck, and chicken. It was demonstrated that the microneedle patch exhibits good extraction performance on insertion into meat tissue samples. It simply requires a pressing and peeling operation to collect high-quality DNA without purification, which could be analysed by advanced detection techniques such as PCR or RPA.
This technique shortens the sample processing time from several hours in conventional extraction methods to 2∼3 min, which improves work efficiency, reduces unnecessary consumption of manpower and material resources, and allows for the rapid identification of components in food and meat products. It has great application potential as it can also be rapidly executed in a more diverse market without bringing the merchandise into the laboratory. Moreover, the use of the RPA amplification method in conjunction with microneedle patch extraction technology can achieve fast species identification. In the future, the detection accuracy of microneedle patches might be improved and integrated with other DNA-based detection methods to address other additional types of food safety problems.
Associated content
Gel electrophoresis patterns of mutton DNA were identified by combining microneedle extraction with RPA; Primers were tested in this study.
Abbreviations used
PMVE/MA, copolymers of methyl vinyl ether and maleic acid; PVA, poly (vinyl alcohol); PCR, polymerase chain reaction; RPA, recombinase polymerase amplification; PEG, Polyethylene glycol; PDMS, polydimethylsiloxane; PBS, phosphate-buffered saline; qRT-PCR, quantitative real-time PCR; LFS, lateral flow strips.
Supplemental Material
Download MS Word (93.1 KB)Acknowledgements
The authors would like to thank all the reviewers who participated in the review, as well as the MJ Editor for providing English editing services during the preparation of this manuscript.
Disclosure statement
No potential conflict of interest was reported by the author(s).
Additional information
Funding
References
- Al, S. D., Chang, J., Bennett, N. R., Topouzi, H., Higgins, C. A., Irvine, D. J., & Ladame, S. (2019). Hydrogel-coated microneedle arrays for minimally invasive sampling and sensing of specific circulating nucleic acids from skin interstitial fluid. ACS Nano, 13(8), 9620–9628. https://doi.org/10.1021/acsnano.9b04783
- Ballin, N. Z., Vogensen, F. K., & Karlsson, A. H. (2009). Species determination – can we detect and quantify meat adulteration? Meat Science, 83(2), 165–174. https://doi.org/10.1016/j.meatsci.2009.06.003
- Beibei Wang, X. H. (2012). Supply and demand situation and prospect of mutton in China. Outlook for Agriculture, 8(10), 19–22.
- Cao. (2017). Study on visual identification methods for detection of 5 animal-derived components by PCR and RPA. Nanjing Agricultural University.
- Cao, Y., Zheng, K., Jiang, J., Wu, J., Shi, F., Song, X., & Jiang, Y. (2018). A novel method to detect meat adulteration by recombinase polymerase amplification and SYBR green I. Food Chemistry, 266, 73–78. https://doi.org/10.1016/j.foodchem.2018.05.115
- Chandu, D., Paul, S., Parker, M., Dudin, Y., King-Sitzes, J., Perez, T., Mittanck, D. W., Shah, M., Glenn, K. C., & Piepenburg, O. (2016). Development of a rapid point-of-use DNA test for the screening of genuity® roundup ready 2 yield® soybean in seed samples. Biomed Research International, 2016. https://doi.org/10.1155/2016/3145921
- Chang, H., Zheng, M., Yu, X., Than, A., Seeni, R. Z., Kang, R., Tian, J., Khanh, D. P., Liu, L., Chen, P., & Xu, C. (2017). A swellable microneedle patch to rapidly extract skin interstitial fluid for timely metabolic analysis. Advanced Materials, 29(37), 1702243. https://doi.org/10.1002/adma.201702243
- David, S., Blaise, B., Bruce, C. V. C., Jeffrey, S. C., Christophe, C., Diederik, D., Eesa, M., Fischer, U., Hausegger, K., Hirsch, J. A., Hussain, M. S., Jansen, O., Jayaraman, M. V., Khalessi, A. A., Kluck, B. W., Lavine, S., Meyers, P. M., Ramee, S., Rüfenacht, D. A., … Dierk, V. (2018). Multisociety consensus quality improvement revised consensus statement for endovascular therapy of acute ischemic stroke. International Journal of Stroke, 13(6), 612–632. https://doi.org/10.1177/1747493018778713
- Dayu Liu, L. X. W. W. (2019). Research progress and analysis and comparison of mutton forgery identification technology. Journal of Food Safety and quality Inspection, 10(1), 181–186.
- Donnelly, R. F., Mccrudden, M. T., Zaid, A. A., Larraneta, E., Mcalister, E., Courtenay, A. J., Kearney, M.-C., Singh, T. R. R., McCarthy, H. O., Kett, V. L., Caffarel-Salvador, E., Al-Zahrani, S., & Woolfson, A. D. (2014). Hydrogel-forming microneedles prepared from “super swelling” polymers combined with lyophilised wafers for transdermal drug delivery. PLoS One, 9(10), e111547. https://doi.org/10.1371/journal.pone.0111547
- Eltayib, E., Brady, A. J., Caffarel-Salvador, E., Gonzalez-Vazquez, P., Zaid, A. A., Mccarthy, H. O., McElnay, J. C., & Donnelly, R. F. (2016). Hydrogel-forming microneedle arrays: Potential for use in minimally-invasive lithium monitoring. European Journal of Pharmaceutics and Biopharmaceutics, 102, 123–131. https://doi.org/10.1016/j.ejpb.2016.03.009
- Guha, P., Das, A., Dutta, S., & Chaudhuri, T. K. (2018). A rapid and efficient DNA extraction protocol from fresh and frozen human blood samples. Journal of Clinical Laboratory Analysis, 32(1), 1. https://doi.org/10.1002/jcla.22181
- Higuchi, R., Fockler, C., Dollinger, G., & Watson, R. (1993). Kinetic PCR analysis: Real-time monitoring of DNA amplification reactions. Bio/technology (Nature Publishing Company), 11(3), 9. https://doi.org/10.1038/nbt0393-S9
- Jingxin Zhang, Y. G. Y. F. (2015). Identification of duck-derived components in livestock and poultry meat by PCR. Anhui Agricultural Sciences, 43(34), 202–203.
- Kang, T., Lu, J., Yu, T., Long, Y., & Liu, G. (2022). Advances in nucleic acid amplification techniques (NAATs): COVID-19 point-of-care diagnostics as an example. Biosensors & Bioelectronics, 206, 114109. https://doi.org/10.1016/j.bios.2022.114109
- Kesmen, Z., Gulluce, A., Sahin, F., & Yetim, H. (2009). Identification of meat species by TaqMan-based real-time PCR assay. Meat Science, 82(4), 444–449. https://doi.org/10.1016/j.meatsci.2009.02.019
- Kight, E., Alfaro, R., Gadila, S., Chang, S., Evans, D., Embers, M., & Haselton, F. (2022). Direct capture and early detection of lyme disease spirochete in skin with a microneedle patch. Biosensors (Basel), 12(10), 819. https://doi.org/10.3390/bios12100819
- Kim, J., Johnson, M., Hill, P., & Gale, B. K. (2009). Microfluidic sample preparation: Cell lysis and nucleic acid purification. Integrative Biology: Quantitative Biosciences from Nano to Macro, 1(10).
- Kim, Y. S., Kim, J., Na, W., Sung, G. H., Baek, S. K., Kim, Y. K., Kim, G. R., Hu, H.-J., & Park, J. H. (2022). Development of a microneedle swab for acquisition of genomic DNA from buccal cells. Frontiers in Bioengineering and Biotechnology, 10, 829648. https://doi.org/10.3389/fbioe.2022.829648
- Kumar, A., Kumar, R. R., Sharma, B. D., Gokulakrishnan, P., Mendiratta, S. K., & Sharma, D. (2015). Identification of species origin of meat and meat products on the DNA basis: A review. Critical Reviews in Food Science and Nutrition, 55(10), 1340–1351. https://doi.org/10.1080/10408398.2012.693978
- Li, H., Feng, J., Wang, Y., Liu, G., Chen, X., & Fu, L. (2021). Instant and multiple DNA extraction method by microneedle patch for rapid and on-site detection of food allergen-encoding genes. Journal of Agricultural and Food Chemistry, 69(24), 6879–6887. https://doi.org/10.1021/acs.jafc.1c01077
- Li, J., Lu, H., Wang, Y., Yang, S., Zhang, Y., Wei, W., Qiao, Y., Dai, W., Ge, R., & Dong, H. (2022). Interstitial fluid biomarkers’ minimally invasive monitoring using microneedle sensor arrays. Analytical Chemistry, 94(2), 968–974. https://doi.org/10.1021/acs.analchem.1c03827
- Li, J., Ma, B., Fang, J., Zhi, A., Chen, E., Xu, Y., Yu, X., Sun, C., & Zhang, M. (2019). Recombinase polymerase amplification (RPA) combined with lateral flow immunoassay for rapid detection of salmonella in food. Foods (basel, Switzerland), 9(1), 27. https://doi.org/10.3390/foods9010027
- Li, X., & Guan, Y. (2019). Specific identification of the adulterated components in beef or mutton meats using multiplex PCR. Journal of Aoac International, 102(4), 1181–1185. https://doi.org/10.5740/jaoacint.18-0338
- Mukerjee, E. V., Collins, S. D., Isseroff, R. R., & Smith, R. L. (2003). Microneedle array for transdermal biological fluid extraction and in situ analysis. Sensors & Actuators: A. Physical, 114(2), 267–275.
- Nehal, N., Choudhary, B., Nagpure, A., & Gupta, R. K. (2021). DNA barcoding: A modern age tool for detection of adulteration in food. Critical Reviews in Biotechnology, 41(5), 767–791. https://doi.org/10.1080/07388551.2021.1874279
- Nicoloso, L., Crepaldi, P., Mazza, R., Ajmone-Marsan, P., & Negrini, R. (2013). Recent advance in DNA-based traceability and authentication of livestock meat PDO and PGI products. Recent Patents on Food, Nutrition & Agriculture, 5(1), 9–18. https://doi.org/10.2174/2212798411305010004
- Notomi, T., Okayama, H., Masubuchi, H., Yonekawa, T., Watanabe, K., Amino, N., & Hase, T. (2000). Loop-mediated isothermal amplification of DNA. Nucleic Acids Research, 28(12), E63. https://doi.org/10.1093/nar/28.12.e63
- Paul, A. M., Ryan, A. D. W., Gavin, F. D., Agnieszka, Z., & John, H. P. (2004). Influence of plasticizer type and storage conditions on properties of poly(methyl vinyl ether-co-maleic anhydride) bioadhesive films. Journal of Applied Polymer Science, 91(3), 1576–1589.
- Paul, R., Ostermann, E., Chen, Y., Saville, A. C., Yang, Y., Gu, Z., Whitfield, A. E., Ristaino, J. B., & Wei, Q. (2021). Integrated microneedle-smartphone nucleic acid amplification platform for in-field diagnosis of plant diseases. Biosensors & Bioelectronics, 187, 113312. https://doi.org/10.1016/j.bios.2021.113312
- Paul, R., Ostermann, E., Gu, Z., Ristaino, J. B., & Wei, Q. (2020). DNA extraction from plant leaves using a microneedle patch. Current Protocols in Plant Biology, 5(1), e20104. https://doi.org/10.1002/cppb.20104
- Paul, R., Saville, A. C., Hansel, J. C., Ye, Y., Ball, C., Williams, A., Chang, X., Chen, G., Gu, Z., Ristaino, J. B., & Wei, Q. (2019). Extraction of plant DNA by microneedle patch for rapid detection of plant diseases. ACS Nano, 13(6), 6540–6549. https://doi.org/10.1021/acsnano.9b00193
- Petya, S., Marieta, T., Tzveta, G., Velitchka, G., & Angel, A. (2013). A modified CTAB method for DNA extraction from soybean and meat products. Biotechnology & Biotechnological Equipment, 27(3), 3803 –3810.
- Piepenburg, O., Williams, C. H., Stemple, D. L., & Armes, N. A. (2006). DNA detection using recombination proteins. Plos Biology, 4(7), 7. https://doi.org/10.1371/journal.pbio.0040204
- Qiao, Y., Du, J., Ge, R., Lu, H., Wu, C., Li, J., Yang, S., Zada, S., Dong, H., & Zhang, X. (2022). A sample and detection microneedle patch for psoriasis MicroRNA biomarker analysis in interstitial fluid. Analytical Chemistry, 94(14), 5538–5545. https://doi.org/10.1021/acs.analchem.1c04401
- Raj, S. T., Woolfson, A. D., & Donnelly, R. F. (2010). Investigation of solute permeation across hydrogels composed of poly(methyl vinyl ether-co-maleic acid) and poly(ethylene glycol). Journal of Pharmacy and Pharmacology, 62(7), 829–837. https://doi.org/10.1211/jpp.62.06.0003
- Rossen, L., Nørskov, P., Holmstrøm, K., & Rasmussen, O. F. (1992). Inhibition of PCR by components of food samples, microbial diagnostic assays and DNA-extraction solutions. International Journal of Food Microbiology, 17(1), 1. https://doi.org/10.1016/0168-1605(92)90017-W
- Sabri, A., Anjani, Q. K., Utomo, E., Ripolin, A., & Donnelly, R. F. (2022). Development and characterization of a dry reservoir-hydrogel-forming microneedles composite for minimally invasive delivery of cefazolin. International Journal of Pharmaceutics, 617, 121593. https://doi.org/10.1016/j.ijpharm.2022.121593
- Varma, A., Padh, H., & Shrivastava, N. (2007). Plant genomic DNA isolation: An art or a science. Biotechnology Journal, 2(3), 386–392. https://doi.org/10.1002/biot.200600195
- Wang, F., Ge, D., Wang, L., Li, N., Chen, H., Zhang, Z., Zhu, W., Wang, S., & Liang, W. (2021). Rapid and sensitive recombinase polymerase amplification combined with lateral flow strips for detecting Candida albicans. Analytical Biochemistry, 633, 114428. https://doi.org/10.1016/j.ab.2021.114428
- Wang, P. M., Cornwell, M., & Prausnitz, M. R. (2005). Minimally invasive extraction of dermal interstitial fluid for glucose monitoring using microneedles. Diabetes Technology & Therapeutics, 7(1), 131–141. https://doi.org/10.1089/dia.2005.7.131
- Wang, Q., Shen, X., Qiu, T., Wu, W., Li, L., Wang, Z., & Shou, H. (2021). Evaluation and application of an efficient plant DNA extraction protocol for laboratory and field testing. Journal of Zhejiang University-SCIENCE B, 22(2), 99–111. https://doi.org/10.1631/jzus.B2000465
- Wang, R., Zhang, F., Wang, L., Qian, W., Qian, C., Wu, J., & Ying, Y. (2017). Instant, visual, and instrument-free method for on-site screening of GTS 40-3-2 soybean based on body-heat triggered recombinase polymerase amplification. Analytical Chemistry, 89(8), 4413 –4418.
- Wang, T. Y., Wang, L., Zhang, J. H., & Dong, W. H. (2011). A simplified universal genomic DNA extraction protocol suitable for PCR. Genetics and Molecular Research, 10(1), 519–525. https://doi.org/10.4238/vol10-1gmr1055
- Xiao, B., Zhao, R., Wang, N., Zhang, J., Sun, X., Huang, F., & Chen, A. (2023). Integrating microneedle DNA extraction to hand-held microfluidic colorimetric LAMP chip system for meat adulteration detection. Food Chemistry, 411, 135508. https://doi.org/10.1016/j.foodchem.2023.135508
- Xiaofang Wu, H. Y. X. Z. (2021). Rapid detection of mutton-derived components by visual nucleic acid isothermal amplification. Chinese Journal of Health Inspection, 31(7), 817–819.
- Xiujuan Wang, R. X. C. Z. (2015). Optimization of extraction conditions of blood genomic DNA extraction kit. Disease Surveillance and Control, 9(12), 855–857.
- Xu, J., Zhao, W., Zhu, M., Wen, Y., Xie, T., He, X., Zhang, Y., Cao, S., Niu, L., Zhang, H., & Zhong, T. (2016). Molecular identification of adulteration in mutton based on mitochondrial 16S rRNA gene. Mitochondrial DNA Part A, DNA Mapping Sequencing, and Analysis, 27(1), 628–632. https://doi.org/10.3109/19401736.2014.908377
- Yalcinkaya, B., Yumbul, E., Mozioglu, E., & Akgoz, M. (2017). Comparison of DNA extraction methods for meat analysis. Food Chemistry, 221, 1253–1257. https://doi.org/10.1016/j.foodchem.2016.11.032
- Zhu, J., Zhou, X., Kim, H. J., Qu, M., Jiang, X., Lee, K., Ren, L., Wu, Q., Wang, C., Zhu, X., Tebon, P., Zhang, S., Lee, J., Ashammakhi, N., Ahadian, S., Dokmeci, M. R., Gu, Z., Sun, W., & Khademhosseini, A. (2020). Gelatin methacryloyl microneedle patches for minimally invasive extraction of skin interstitial fluid. Small, 16(16), e1905910. https://doi.org/10.1002/smll.201905910