Abstract
Ovarian hormones, particularly oestrogen and progesterone, undergo major fluctuations across the female lifespan. These hormone transition periods, such as the transition from pregnancy to postpartum, as well as the transition into menopause (perimenopause), are also known to be times of elevated susceptibility to depression. This study reviews how these transition periods likely influence neurochemical changes in the brain that result in disease vulnerability. While there are known associations between oestrogen/progesterone and different monoaminergic systems, the interactions and their potential implications for mood disorders are relatively unknown. Positron Emission Tomography (PET) allows for the in-vivo quantification of such neurochemical changes, and, thus, can provide valuable insight into how both subtle and dramatic shifts in hormones contribute to the elevated rates of depression during pre-menstrual, post-partum, and perimenopausal periods in a woman’s life. As one better understands how to address the challenges of PET studies involving highly vulnerable populations, such as women who have recently given birth, one will gain the insight necessary to design and individualize treatment and therapy. Understanding the precise time-line in younger women when dramatic fluctuations in the hormonal milieu may contribute to brain changes may present a powerful opportunity to intervene before a vulnerable state develops into a diseased state in later life.
Introduction
Depression is ranked by the World Health Organization as the largest contributor to disability worldwide, and it is predicted to be the leading cause of disease burden by 2030 (World Health Organization, Citation2008). Unfortunately, due to a lack of knowledge of the specific underlying neuropathophysiological mechanisms, treatment decisions for depression are guided primarily by observable symptoms rather than the actual pathology specific to an individual. Several recent NIMH initiatives, including the Research Domain Criteria (RDoc) project framework, reflect the need for psychiatry to move beyond the mere observation of symptoms and to configure how genomic, enviromic, endophenomic, and phenomic domains can be tested at the individual level to inform the most indicated course of anti-depressive treatment (Cuthbert, Citation2014). One important potential source of such individual variance is biological sex. Sex differences in mood disorders are currently of peak interest in the field of neuroscience. In fact, the National Institute of Health now requires consideration of sex as a biological variable in grant applications (National Institutes of Health, Citation2015). This requirement is, in part, driven by the prominent epidemiological sexual dimorphism observed in numerous neuropsychiatric disorders, such as major depressive disorder (MDD), which women are twice as likely to develop as men (Kessler, Citation2003; Weissman et al., Citation1993).
Recent work not only suggests that differences between men and women influence mood disorder aetiology, but individual differences among women further create particular windows of vulnerability to depression. The prevalence of depression in women is most prominent during the reproductive years (Soares & Zitek, Citation2008), and especially during times such as post-partum (Mehta et al., Citation2014; O’hara, Stuart, Gorman, & Wenzel, Citation2000; Sohr-Preston & Scaramella, Citation2006) and perimenopause (Freeman, Sammel, Boorman, & Zhang, Citation2014; Freeman, Sammel, Lin, & Nelson, Citation2006; Rekkas et al., Citation2014), which are periods defined by extreme fluctuations in ovarian hormones. This evidence strongly suggests that hormone changes across the individual mean, especially dramatic decreases in oestrogen, may be a risk factor for depression. While several studies have shown an association between changes in mood and ovarian hormone levels in women, we lack a detailed understanding of the specific mechanisms underlying the relationship between mood and hormonal transitions.
We require methodological incorporation from the fields of cognitive science, genetics, and neuroimaging in order to clarify the biological contributors to sex differences in mood disorders, as well as to explain windows of heightened vulnerability to mood disorders across the lives of women. Among the broad field of rapidly developing neuroimaging techniques, Positron Emission Tomography (PET) stands out as it allows for the in vivo quantification of a specific neurochemical target at a specific molecular site (Konarski, McIntyre, Soczynska, Bottas, & Kennedy, Citation2006). PET may allow for the early identification of biochemical markers of mood disorders before structural brain changes take place, providing an opportunity for earlier and more specific diagnosis, intervention, and treatment (Chen, Liu, Li, Zhang, & Tian, Citation2011). Radioligand PET studies provide information at the biochemical level about binding affinity, receptor-density parameters, neurotransmission activity, and location of different transmitter pathways. Moreover, these studies can give insight into medication dosing by quantifying receptor binding following drug administration, thereby measuring drug occupancy and confirming that the drug is reaching and affecting the target site.
Numerous PET studies examined regional cerebral blood flow, cerebral glucose metabolism rate, and cerebral oxygen metabolism rate in patients with depression, and found these measures changed, especially in the prefrontal cortex and limbic structures, which are regions involved in emotional regulation and memory formation. In general, cerebral blood flow/metabolism is decreased in the prefrontal cortex (Baxter et al., Citation1989; Bench, Friston, Brown, Frackowiak, & Dolan, Citation1993; Buchsbaum et al., Citation1997; Hosokawa, Momose, & Kasai, Citation2009; Kennedy et al., Citation2001; Kimbrell et al., Citation2002) and increased in the amygdala (Drevets et al., Citation2002). The abnormal hyper- and hypo-metabolism in these regions, respectively, are also correlated with the severity of depression (Baxter et al., Citation1985, Citation1989; Drevets et al., Citation2002: Ketter et al., Citation2001; Kimbrell et al., Citation2002). Additionally, there are reports of decreased blood flow and metabolism in the sub-genual prefrontal cortex (Brooks et al., Citation2009; Drevets et al., Citation1997), and increases in the hippocampus, cerebellum, anterior cingulate gyrus, and basal ganglia in depressed patients. These findings support that depressed patients have functional deficits in the frontal lobes, limbic system, basal ganglia, and cerebellum.
The neuroplasticity hypothesis of depression suggests that there is reduced neurogenesis and plasticity in response to external or internal environmental stimuli, and that antidepressants may serve to improve neuroplasticity (Serafini, Citation2012; Wainwright & Galea, Citation2013). According to the monoamine hypothesis, depression is caused by a deficit in monoamine levels in the central nervous system (Delgado, Citation2000). There is currently no other imaging technique as promising as PET radioligand studies for directly analysing specific neurotransmitter abnormalities in the central nervous system and their biological consequences in humans in vivo. These theories are not likely exclusive, but rather complementary, supporting the need for gathering neurochemical information via PET in conjunction with network and connectivity data, with the ultimate aim to identify the appropriate molecular target.
Most PET depression studies have focused on disruptions in the serotonin and dopamine systems, both of which are neurotransmitters widely implicated in mood disorders. Serotonin dysfunction in depressed patients has been shown in serotonin synthesis, serotonin transporter binding potential (5-HTT BP), and 5-HT1A and 5-HT2A receptors BP. The findings have been mixed, some reporting increases or decreases in transporter BP, increases or decreases in 5-HT1A BP, and increases or decreases in 5-HT2A BP. While the data are controversial, there is consistency with respect to implicated brain regions. Dopamine system dysfunction has also been highly implicated in depression (Drevets et al., Citation2001; Dunlop & Nemeroff, Citation2007; Nestler & Carlezon, Citation2006; Willner, Hale, & Argyropoulos, Citation2005). Primary rewards are associated with striatal dopamine increases, and deficiencies are thought to underlie the loss of pleasure and motivation rewarding in depression (Whitton, Treadway, & Pizzagalli, Citation2015). However, the findings are discrepant in imaging studies. Monoamine oxidase A (MAO-A) is an enzyme that metabolizes monoamine neurotransmitters, such as serotonin and dopamine (Youdim, Edmondson, & Tipton, Citation2006). Thus, it has been suggested that elevated MAO-A density could be the primary monoamine-lowering process during depression (Meyer et al., Citation2006), as excessive removal of these monoamines is associated with a lower mood (Hasler et al., Citation2008). Indeed, depressed patients show exaggerated MAO-A binding rates as compared to healthy controls (Meyer et al., Citation2009). These studies are a promising beginning to a better understanding of the underlying mechanisms of neurochemical dysfunctions in mood disorders via the use of radioligands.
This review will explore how and why PET is a tool that uniquely allows us to explore the causes and consequences of mood disorders across the lifespan of a woman. Specifically, we will report the major findings of PET studies that have explored sex differences in mood disorders, as well as differences within women driven by hormonal changes. We will discuss recent innovative approaches to model hormonal transition periods in human in vivo PET research and extend the discussion of this experimental work to a focused review on how periods of endogenous and physiological hormonal transitions, such as the menstrual cycle, perimenopause, and post-partum, can serve both as a time of vulnerability and opportunity in understanding the transition from health to a disease state. We will further explore how a deeper understanding of this can aid us in facing challenges in neuroimaging research, such as establishing safety protocols in post-partum patients who are breastfeeding.
If we better understand how sex and hormonal changes influence mood, we can identify windows of increased vulnerability to depression and can better optimize/tailor treatment to the individual patient. A deeper understanding of neurotransmitter dysfunction, particularly in relationship to endogenous hormonal changes across the female lifespan, can help us design, test, and personalize drug treatment for women. Beyond the clinical implications, this could also have important social/practical implications such as increased understanding of and support during the many female-specific life events that are currently misunderstood and often stigmatized simply due to a faulty grasp of the biological origins linked to these events. because we do not fully understand their biological origins yet. A neurobiological approach also challenges many of the stereotypes, which often unfortunately continue to be associated with female-specific periods across the lifespan, such as the pre-menstrual phase, menopausal transition, and post-partum blues, as we view these periods as more than just individual psychological conflicts and rather as products of a complex interaction of hormonal and neurochemical transitions that are also psychologically influenced by certain psychological and societal expectations. Thus, to add to the important discussion of psychological factors, we require more research into the complex interactions between hormones and brain functional and neurochemical activity. This review will discuss ways of overcoming practical challenges in this topic.
If we can overcome these challenges and address this stigma, we can identify windows of increased vulnerability, prevent/intervene during the transition from depression-vulnerable state to a diseased state, and optimize therapy and drug treatment during specific times of hormonal fluctuations.
Evidence for sex differences
There is extensive literature on the heightened risk of developing depression in women than in men, with the lifetime risk of major depression at 21% for women vs only 13% in men (Kessler et al., Citation1994). Beyond that, symptom severity and duration are higher in women, as well as rates of associated weight gain, anxiety, and physical manifestations of the disease (Angst & Dobler-Mikola, Citation1984; Frank, Carpenter, & Kupfer, Citation1988; Kornstein et al., Citation2000; Young, Scheftner, Fawcett, & Klerman, Citation1990). While differences are demonstrated across all age groups, the gap in depression risk between the sexes is widest during the reproductive years (Soares & Zitek, Citation2008).
PET can be used to visualize in vivo neurochemical changes and metabolism in the midst of a depressive episode. While sex differences in this area are often only reported as secondary findings, there is increasing attention within this field. There have been sex-related PET findings in glucose metabolism, in which women showed enhanced activity in the left amygdala and men in the right amygdala related to emotionally influenced memory (Cahill et al., Citation2001). Radioligand neuroreceptor studies have also shown that depression-relevant neurotransmitters exhibit sex differences in healthy controls. For example, healthy women have higher 5-HT1A receptor BP (Costes et al., Citation2005; Jovanovic et al., Citation2008; Parsey et al., Citation2002), and the 5-HT1A receptor demonstrates decreased BP with age in men, but not in women (Meltzer et al., Citation2001). Furthermore, women show lower 5-HTT BP in cortical and subcortical brain regions, as compared with healthy men (Costes et al., Citation2005; Jovanovic et al., Citation2008; Parsey et al., Citation2002). However, other studies (Staley et al., Citation2001) found greater availability of 5-HTT in females. Discrepancies have also been reported in serotonin synthesis. Women have decreased serotonin synthesis compared to males after tryptophan was depleted (Nishizawa et al., Citation1997). Women show lower α-[11C]methyl-L-tryptophan brain trapping constant (an index of serotonin synthesis) in a number of cerebral cortical regions compared with men (Sakai Citation2006). In another study, women showed elevated levels of serotonin, as well as the serotonin metabolite 5-hydroxyindoleacetic acid, compared with men (Young, Gauthier, Anderson, & Purdy, Citation1980). For the dopamine system, women showed a higher concentration of synaptic dopamine in the striatum than men, and age decreases these levels in men more than in women (Laakso et al., Citation2002). Women also show less dopamine release in the ventral striatum, anterior putamen, and anterior and posterior caudate nuclei in response to amphetamines than do men (Munro et al., Citation2006).
Given that it has been proposed that individuals with depression have abnormal neurotransmission, the findings in healthy subjects do not necessarily reflect what is happening in the brains of depressed men and women. Sex differences in depressed populations, however, have not been the primary focus of clinical PET studies. Videbech et al. (Citation2001) reported significant sex differences in cerebral blood flow in the parietal lobe (and a trend for sex differences in the temporal lobe) for the entire sample of MDD patients and healthy controls (n = 89, 42 patients including 12 males, 47 controls including 16 males). When regressing out the effects of sex and age, the research revealed increased blood flow to the hippocampus as the main finding in major depression, but no statistically significant correlations were found between any region and the Hamilton Depression Scale (HDS). Videbech et al. (Citation2002) later re-investigated this secondary finding, and reported that sex displayed a noteworthy interaction for hippocampal blood flow in major depressive disorder (MDD): a positive correlation between symptom severity (HDS score) and blood flow in the hippocampus in female MDD-patients, but the reverse relationship in males. Their study was not designed to investigate sex differences, however, and so they warned against over-interpreting the results due to the low male population (n = 12 compared to female n = 30) included. Hosokawa et al. (Citation2009) uniquely looked at an all-female population, and found a decrease in glucose metabolism in the right temporal gyrus, right insula, and left posterior cingulate gyrus in depressed women.
There have also been sex differences reported in monoaminergic systems in depressed patients. Rosa-Neto et al. (Citation2004) found a lower α-[11C]methyl-L-tryptophan brain trapping constant (an index of serotonin synthesis) in the bilateral anterior cingulate cortex (ACC) of MDD women compared to female healthy controls, and lower levels in the left ACC in MDD males compared to male healthy controls as well. Their lab later directly compared male to female samples and found that depressed women have higher serotonin synthesis in multiple regions of the prefrontal cortex and limbic system involved with mood regulation, as compared with depressed men (Frey, Skelin, Sakai, Nishikawa, & Diksic, Citation2010). Monoamine tryptophan depletion results in a temporary decrease in serotonin transmission, and this increased depressive symptoms in females significantly more than in males (Moreno, McGahuey, Freeman, & Delgado, Citation2006). There have been reports, however, of no effect of sex on 5-HTT BP (Meyer et al., Citation2004) or 5-HT1A receptor BP (Parsey, Olvet, et al., Citation2006; Parsey, Oquendo, et al., Citation2006).
While these are promising findings, it is noticeable that the primary aim of most of these studies was not to investigate sex differences. For the minority of studies that did make it their primary focus, the reported results have been conflicting. A likely reason for these discrepancies may be the heterogeneity of the female sample, in particular the challenges to monitor the details of reproductive status. If ovarian hormones are highly implicated in depression aetiology, as well as known to influence key monoaminergic systems, women with different hormonal profiles may exhibit very different responses. However, hormonal levels were not measured in the previously mentioned studies. This may lead to false negatives or false positives, depending on the hormonal profile of the female cohort. If all women were in the follicular phase or all in the luteal phase, they would have specific hormonal profiles (described in next section) that may bias the results. More likely, the women were in different phases of their menstrual cycle, and there may be a masked effect on results. The potential likelihood is especially high when sample sizes are relatively small, as is often the case in studies not specifically designed to compare males and females, but rather have a combined group. Since depression has become such a serious problem in health today, identifying biomarkers/biological contributors is of crucial importance to better understand the influence of hormones in depression across the lives of women.
Ovarian hormones
Oestrogen is the ovarian hormone that exhibits the most dramatic endogenous changes across the female lifespan. Oestrogen levels rise during puberty, and then remain high but unstable throughout the reproductive years. The physiological changes that occur to indicate the transition into menopause are known as perimenopause. Women typically exhibit initial changes beginning in their late 30s and early 40s, and then changes continue, on average, until the late 40s (Mitchell, Woods, & Mariella, Citation2000). Perimenopause is characterized by dramatic fluctuations and decreases in oestrogen, followed by chronic low oestrogen levels during the post-menopause years. Progesterone, the other main ovarian hormone, also rises in level during puberty and remains relatively stable until perimenopause, when the level gradually but continuously decreases. For a more in-depth review of changes in ovarian hormones across the female lifespan, please see Barth, Villringer, and Sacher (Citation2015).
These ovarian hormones have known effects on neurotransmitter functions, mainly through the alteration of the responsiveness of post-synaptic receptors and/or the pre-synaptic release of neurotransmitters. shows a schematic of oestrogen and progesterone receptors in the glutamatergic, GABAergic, dopaminergic, and serotoninergic systems. Briefly, oestrogen facilitates glutamate transmission (Adams, Fink, Janssen, Shah, & Morrison, Citation2004; Gazzaley, Weiland, McEwen, & Morrison, Citation1996; Smith & Woolley, Citation2004), which plays a significant role in synaptic plasticity, learning, and memory. Progesterone has been shown to do the opposite, instead inhibiting the excitatory glutamate response (Hausmann & Güntürkün, Citation2000). Oestrogen suppresses GABA inhibitory inputs (Murphy, Cole, Greenberger, & Segal, Citation1998), and progesterone exhibits excitatory effects through its action on GABAA receptors (van Wingen et al., Citation2008). Both the serotonergic and dopaminergic systems are influenced by oestrogen. In rodents, oestrogen appears to promote dopamine activity and release in the striatum, thereby enhancing the dopamine turnover rate (Di Paolo, Falardeau, & Morissette, Citation1988; Lévesque, Gagnon, & Di Paolo, Citation1989; Shimizu & Bray, Citation1993; Xiao & Becker, Citation1994). In rodents and non-human primates, oestrogen administration enhances serotonergic tone by increasing serotonin synthesis (Bethea, Mirkes, Shively, & Adams, Citation2000), decreasing degradation (Gundlah, Lu, & Bethea, Citation2002), and decreasing inhibitory feedback to serotonergic neurons in the dorsal raphe nucleus (Henderson & Bethea, Citation2008; Lu & Bethea, Citation2002). If these ovarian hormones impact the neurochemical systems involved in emotional and cognitive functioning, which are also known to be altered in depression, it is critical that we examine how hormones and these neurotransmitters interact to influence mood—a complex question that PET imaging is uniquely qualified to answer.
Figure 1. Localization of oestrogen receptors, progesterone receptors, and monoaminergic pathways in the female brain (adapted with copyright permission granted from Barth et al., Citation2015). Co-expression of ovarian hormone receptors in the CNS alongside neurotransmitter localization. The glutamatergic (pink), GABAergic (green), dopaminergic (blue), and serotonergic (yellow) pathways are displayed separately for clearer representation. For a detailed description of ovarian hormone receptor distribution in each pathway, please see Barth et al. (Citation2015). (A) Glutamatergic pathways in the central nervous system. (B) GABAergic pathways in the central nervous system. (C) Dopaminergic pathways in the central nervous system. (D) Serotoninergic pathways in the central nervous system.
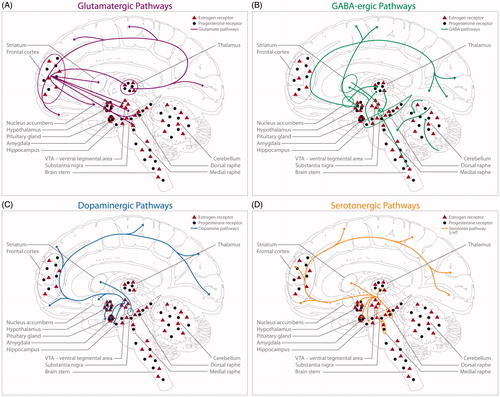
Hormonal transitions
Periods of vulnerability
Epidemiological evidence suggests the highest vulnerability towards depressed mood during life phases when ovarian steroid hormones fluctuate or decline rapidly (Deecher, Andree, Sloan, & Schechter, Citation2008), such as post-partum (Gavin et al., Citation2005; Le Strat, Dubertret, & Le Foll, Citation2011) and during menopausal transition (Freeman et al., Citation2014) (). During pregnancy, there is a large increase in both oestrogen and progesterone, which is followed by a rapid, dramatic drop in both hormones following delivery. Post-partum depression (PPD) is defined as a major depressive episode starting within 4 weeks of delivery, and markedly affects 13% of women within the first 3 months of giving birth. With its high prevalence rate, PPD is ranked as the most common medical complication of child-bearing (Sit & Wisner, Citation2009); and with its extremely adverse effect on maternal–infant bonding and infant development (O’Higgins, Roberts, Glover, & Taylor, Citation2013; Poobalan et al., Citation2007; Weikum, Oberlander, Hensch, & Werker, Citation2012), it is a critical concern.
Figure 2. Hormonal transition periods and mood disorder findings in PET in the reproductive years. Hormones: oestrogen and progesterone rise during puberty and undergo subtle, cyclical variations during the menstrual cycle. Oestrogen and progesterone rapidly increase and maintain high levels during pregnancy, followed by a dramatic withdrawal following delivery in the post-partum period. Both hormones decrease during perimenopause, but oestrogen is markedly unpredictable and erratic in its fluctuations. Depression: depression associated with hormonal fluctuations during the pre-menstrual phase of the menstrual cycle, following delivery in the post-partum period, and in the transition into menopause. PET Findings: summary of PET imaging studies that focused on women suffering from hormone-mediated depression.
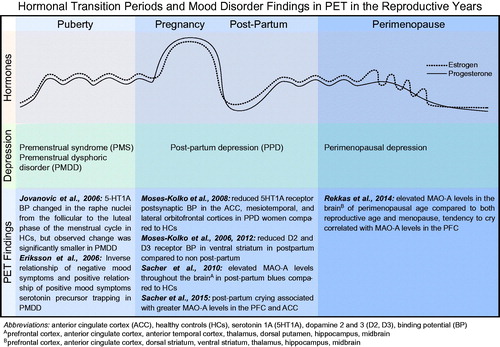
As the symptoms often appear within the first month post-partum, PPD pathophysiology is likely linked to the hormonal disruptions occurring after delivery. Evidence supports that it is not only the absolute concentration of ovarian hormone levels that mediates PPD, but also subsequent neurochemical alterations influenced by the dramatic hormonal changes. Women may develop PPD in part due to imbalanced monoamine levels and activity following the massive withdrawal of oestrogen and progesterone.
Moses-Kolko et al. (Citation2008) evaluated 5HT1A receptor BP in women experiencing PPD. They concluded that, even though reproductive hormone levels did not differ between groups, post-synaptic BP in the depressed women was significantly reduced compared to in healthy controls, especially in the anterior cingulate, mesial-temporal, and lateral orbitofrontal cortices. Their group also investigated D2 receptor BP, due to known alterations in the dopaminergic system in healthy, maternal animal studies. They found reduced D2 receptor BP in the ventral striatum in post-partum compared to non-post-partum women, and reduced BP in unipolar depression patients compared to healthy controls (Moses-Kolko et al., Citation2006). They later (Moses-Kolko et al., Citation2012) looked for depression-by-post-partum interaction effects on D2 and D3 receptor binding, but they failed to find that combined depressed and post-partum status produced additive lowering of D2 and D3 receptor BP. The authors suggest that the lower BP may not be specific to depression and post-partum status, but rather a shared consequence of stress experienced by both depressed patients and recent mothers. However, they also report that the anatomical extent of striatal D2 and D3 receptor reductions differed between depressed and post-partum women: while both post-partum status and depression were associated with 7–8% lower BP in the whole striatum and ventral striatum, only depression showed a trend (p = 0.06) for a 5% reduction in BP in the dorsal striatum. The sub-regions of the striatum participate in a wide range of motivational and associative brain functions, with findings linking the ventral striatum to initial rewarding effects of a drug, and dorsal regions more to consequent habitual behaviour (Koob, Everitt, & Robbins, Citation2013; Stewart & Paulus, Citation2013). Thus, there remains the possibility of mechanistic differences in hormone-mediated dopamine activity that is unique to post-partum status, in which dorsal striatum alterations are a specific feature of prolonged depression, and not to post-partum-related depression. Furthermore, because of the hormonal transition and behavioural adaptations unique to post-partum women, the authors suggest that other experimental designs could more precisely distinguish dopaminergic alterations that are exclusive to depression vs post-partum depression. Regardless, Moses-Kolko et al. (Citation2006, Citation2012) succeeded in showing post-partum modifications of the striatal dopamine system, which may contribute to the high risk of depression following delivery. In addition, a recent paper (Atzil et al., Citation2017) found that maternal behaviour is associated with increased dopamine responses in women to their infants, and stronger intrinsic connectivity within the nucleus accumbens, amygdala, and medial pre-frontal cortex (an intrinsic network that supports social functioning). Moreover, stronger network connectivity is associated with increased dopamine responses within the network.
While the previously mentioned studies are exceptional pioneering studies, post-partum studies are uniquely challenging in terms of recruitment and ability to schedule a PET scan immediately after childbirth, and, thus, their participants were scanned ranging 4–13 weeks after delivery. Since oestrogen levels decrease 100–1000-fold during the first 3–4 days after delivery, there may be a particular neurochemical dysregulation associated with this acute window. Indeed, 70% of new mothers experience ‘post-partum blues’ within a week of delivery, which is a syndrome characterized by anxiety, insomnia, poor appetite, irritability, and overall depressed mood. Beyond this, women with post-partum blues have a 4-fold risk of developing PPD, and greater severity of post-partum blues is associated with a greater risk for PPD. Sacher et al. (Citation2010) compared healthy non-post-partum to women who had given birth within 4–6 days (). They looked at MAO-A, due to its previously described role in monoamine depletion. Additionally, lower oestrogen levels are associated with greater MAO-A levels and activity. They found that the MAO-A distribution volume was significantly elevated throughout the pre-frontal cortex, anterior cingulate cortex, anterior temporal cortex, thalamus, dorsal putamen, hippocampus, and midbrain. They also found that, on the day when symptoms are most severe, day 5, MAO-A levels were significantly greater than on day 4 and day 6. Later, Sacher et al. (Citation2015) also found that both PPD and greater disposition to post-partum crying were associated with greater MAO-A density in the pre-frontal cortex and anterior cingulate cortex. This work provides great insight into the consequences following a major oestrogen decline, finding significant MAO-A alterations in a broad range of structures that may be implicated in depression in the post-partum period.
Figure 3. Elevated brain monoamine oxidase A binding in the early post-partum period (adapted with copyright permission granted from Sacher et al., Citation2010). Using post-partum endogenous hormone fluctuations as a neurobiological model for depression. (A) Oestrogen levels decrease 100–1000-fold within the first 3–4 days after delivery, and continue to decline at a moderate rate afterwards. (B) Monoamine oxidase A (MAO-A) levels are significantly increased during the post-partum period, especially in the early period. There is a peak in MAO-A levels on day 5 post-partum. (C) Approximately 70% of post-partum women experience mood symptoms such as sadness, anxiety, and irritability. Mood is lowest on day 5 post-partum.
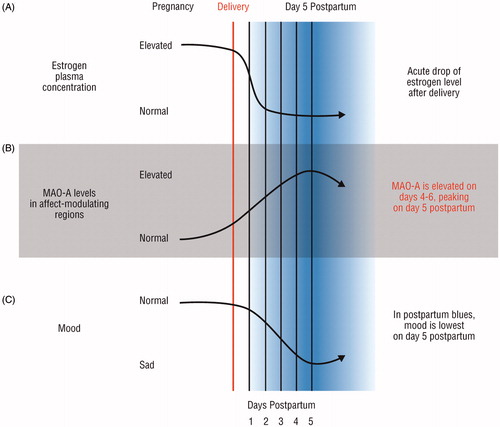
Similar to the dramatic decrease of oestrogen in the post-partum period, perimenopause is also defined by oestrogen decline and associated risk of depression with the magnitude by which oestradiol levels fluctuate around a woman’s own mean as the strongest predictor of a depressive episode during the menopausal transition (Freeman et al., Citation2006, Citation2014). While there are currently no PET studies specifically looking at a clinically-depressed perimenopausal cohort, Rekkas et al. (Citation2014) examined MAO-A levels in perimenopausal women, and found that MAO-A is uniquely elevated in this age group compared to women in either reproductive age or post-menopause across a broad range of brain regions: the prefrontal cortex, anterior cingulate cortex, dorsal striatum, ventral striatum, thalamus, hippocampus, and midbrain. A tendency to cry was positively correlated with MAO-A levels in the pre-frontal cortex. This was the first study in perimenopausal women to report a change of a central monoamine that is highly involved in major depression, and calls for further research to assess MAO-A dysfunction as a target to prevent mood disorders.
Other endogenous hormonal fluctuations periods as critical time-windows: from heightened vulnerability to opportunities for intervention
Beyond these large hormonal shifts across the female lifespan, there are also more acute fluctuations that pre-dispose women to depressive symptoms. There are cyclical fluctuations that naturally occur across the menstrual cycle on a monthly basis throughout the reproductive years. The follicular phase occurs between the onset of menses and ovulation, characterized by rising oestrogen and low levels of progesterone. Shortly before ovulation, oestrogen levels significantly surge. The luteal phase is the time after ovulation and before the next onset of menses. During the luteal phase, progesterone levels slowly rise, with a mid-luteal peak in parallel to a second blunted peak in oestrogen. Both ovarian hormones rapidly decrease at the end of the luteal phase near the onset of the next menses. These cyclical fluctuations can be parallelled by cyclical change in mood and behaviour, which up to 80% of women report to be affected by (Bäckström et al., Citation2014). Thus, the menstrual cycle provides a naturalistic model for studying hormone-mediated changes in neurochemical mechanisms, and how this interaction influences depression aetiology.
PET allows us to go beyond the impact of hormones on healthy brain structural and functional connectivity by allowing the quantification of the hormone-mediated neurochemical changes that may underlie the heightened vulnerability of women for depression. The central serotonergic (5HT) system is one of the candidate systems that may mediate the abnormal mood and behavioural response to ovarian steroids. A large body of pre-clinical and clinical evidence supports crosstalk between oestrogens, in particular oestradiol, and key features of the serotonin system. The serotonin transporter (5-HTT) is the primary mechanism for clearing extracellular serotonin and, thus, regulates synaptic serotonin. Nevertheless, the contribution from 5-HTT across phases of sex-steroid hormone fluctuation and the timing of such serotonergic integration of ovarian steroid hormone information is far from clear and sparsely studied in humans. A recent study elegantly evaluated the interaction between mood and cerebral 5-HTT during hormonal fluctuation by using a gonadotropin-releasing hormone agonist (GnRHa) to induce a biphasic ovarian hormone response, with an initial increase in oestrogen levels and then subsequent decrease in healthy women (Frokjaer et al., Citation2015). Frokjaer et al. (Citation2015) found that the pharmacologically induced fluctuations in hormone levels triggered sub-clinical depressive responses in healthy women without any previous history of depressed mood. Symptom severity was positively correlated with magnitude of oestrogen decline and neocortical serotonin transporter increases. In contrast, Jovanovic, Karlsson, Cerin, Halldin, and Nordström (Citation2009) reported no 5-HTT binding difference in the high-oestrogen follicular phase vs low-oestrogen luteal phase of healthy females, although they did find a trend for increased 5-HTT binding in the ralphe nuclei in the high-oestrogen follicular phase, which is notable given their small sample size (n = 8). As individuals with depression may have abnormal neurotransmission, the findings in healthy subjects do not necessarily reflect what is happening in the brains of women with clinical-level pathology. Indeed, Jovanovic et al. (Citation2006) did report 5-HT1A BP changes in the raphe nuclei from the follicular to the luteal phase of the menstrual cycle in HCs, but observed change was significantly smaller in women with pre-menstrual dysphoric disorder (PMDD) (). PMDD is a DSM-5 recognized condition occurring specifically in the pre-menstrual period with symptom severity equivalent to that of a major depressive episode. Eriksson et al. (Citation2006) found strong associations between mood and serotonin precursor trapping in PMDD patients: negative mood symptoms inversely correlated with serotonin precursor trapping, while positive mood symptoms positively correlated with serotonin precursor trapping. A theory suggests that women who are vulnerable to pre-menstrual mood changes do not have abnormal levels of hormones or some type of hormonal dysregulation, but rather a particular sensitivity to normal cyclical hormonal changes (Bäckström et al., Citation2003). This theory, in conjunction with the stated findings, suggests that there is an irregularity in the oestrogen-mediated serotonin mechanisms in the PMDD population that makes them more vulnerable to depressed mood. This is supported by previous findings showing different responses in PMDD patients to the serotonin-releasing drug dl-fenfluramine (Fitzgerald et al., Citation1997) and serotonergic agent m-chlorophenylpiperazine (Su, Schmidt, Danaceau, Murphy, & Rubinow, Citation1997), suggesting serotoninergic dysfunction in PMDD. This all supports a need for further investigation of hormone-mediated monoamine systems in women with depression during reproductive years.
Starting the study of these mechanisms in health during the reproductive years, with a longitudinal follow-up later in mid- and late-life, may also provide important insight into potential consequences for risk across the ageing process (late menopausal transition, post-menopause, and late life). By systematically characterizing menstrual-cycle associated neurochemical dynamics in younger populations, we will better understand how these interactions might influence the transition from vulnerability for depression into a diseased state, during times across the menstrual cycle, pregnancy to post-partum depression, and reproductive years into perimenopausal, post-menopausal, and late life depression. These findings also have the chance to inform therapeutic approaches by identifying susceptible time windows across the menstrual cycle for pharmacological interventions, such as SSRI administration or intake of dietary precursors for targeting the serotonergic system (Dowlati et al., Citation2017).
Challenges in research
As the clinical and conceptually driven application of PET imaging continues to build on rapid advancements in tracer-development (Sabri, Seibyl, Rowe, & Barthel, Citation2015) and the integration of simultaneous MR-imaging (Aiello et al., Citation2015; Atzil et al., Citation2017), new challenges and concerns arise as research extends into more complex patient populations. For example, using PET in a post-partum cohort is a rather recent development and requires careful consideration of additional factors, such as the potential radiotracer effect on breastmilk. While there are relatively few PET studies examining this population, they have shown that it is possible to do so in a safe manner. For example, Moses-Kolko et al. (Citation2006, Citation2008, Citation2012) and Sacher et al. (Citation2010, Citation2015) measured and confirmed undetectable radioactivity levels in breastmilk within 250 min after injection of carbon 11-labelled tracers with a radio-active half-life of 20 min. In addition, these studies all found robust findings in monoamine abnormalities in post-partum women, which have major implications on vulnerability for PPD in recent mothers. Thus, the additional costs and consideration that make studies in these vulnerable populations possible is a worthy, enlightening endeavour. This section will focus on suggestions on what concerns must be addressed in study design in the hopes of facilitating and encouraging further research.
The first suggestion is to reach out to other disciplines and invest in a multi-professional team. Sacher et al. (Citation2010, Citation2015) employed a paediatric ICU nurse for their post-partum studies, who not only contributed critical professional knowledge, but also made the recent mothers more comfortable and confident to participate — a key component to the feasibility of participant recruitment. The nurse advised and provided the mothers with infant care and soothing strategies. They also employed a lactation consultant, who encouraged the use of a special artificial nipple to avoid nipple confusion in the infants. Thus, the infants could be fed previously pumped breastmilk with a bottle while the mothers underwent scanning as well as after the scan, while the mother’s breastmilk was tested for radioactivity. The multidisciplinary team also collectively brainstormed all logistical planning that made study participation more practicable and achievable immediately after childbirth, such as providing safe transport and a special infant car-seat throughout the study. The findings in these studies, such as elevated MAO-A levels in PPD, are critical indications that we need to further investigate neurochemical mechanisms in post-partum women, and justify the value in additional investments such as a multidisciplinary staff, additional equipment, and transportation services.
The neuroscience field does not need to shy away from the challenges associated with working in highly vulnerable populations. As previously described, consideration of menstrual cycle status is highly informative in mood disorders that are likely to be mediated by ovarian hormones. However, there is a lack of adequate menstrual cycle monitoring strategies in most current literature. Self-reports are likely an inadequate way to determine current cycle phase, as less than half of women correctly report their last menstruation when recall is 3 or more weeks after bleeding cessation (Wegienka & Baird, Citation2005). While it is demanding to monitor menstrual cycle status, a recent study (Barth et al., Citation2016) conveys the exciting and worthwhile potential of implementing rigorous monitoring techniques. Barth et al. (Citation2016) studied the in-vivo dynamics of hippocampal structural changes across the menstrual cycle. They acquired 30 diffusion weighted imaging scans in a single healthy subject across two menstrual cycles, as well as daily blood samples for serum hormone levels and urine ovulation tests to detect the lutropin hormone surge. They found that structural hippocampal changes parallel fluctuations in endogenous ovarian hormone levels rapidly across the menstrual cycle. Specifically, they observed a positive correlation between daily oestrogen level and microstructural integrity in the bilateral hippocampus with zero time lag, and the same oestrogen levels were negatively correlated with radial diffusivity. This paper extended previous papers that show menstrual cycle associated fluctuations in functional connectivity, particularly in the hippocampus (Arélin et al., Citation2015; Lisofsky et al., Citation2015), by measuring on a daily basis. These in vivo findings from human neuroimaging studies further support the extensive evidence from animal research for an immediate subsequent effect of acute changes in oestrogen on brain plasticity across the menstrual cycle. This line of work strongly suggests that acute monitoring in patients who show hormone-related mood disorders is both feasible and relevant to the individual patient trajectory for illness, treatment, and relapse-risk.
These lines of work delineate the impressive effect of the menstrual cycle on brain region dynamics involved in the pathophysiology of depression and call for integration with PET imaging to fully understand the interplay between ovarian hormones, functional and structural connectivity, and neurochemical mechanisms. To better investigate this effect, we suggest a combination of following strategies for comprehensive cycle monitoring: (1) daily recording of body temperature to assess the reproductive phase as marked by an increase in core body temperature up to 1 degree, (2) assessment of ovarian hormone serum blood levels at each time point to measure fluctuation dynamics and menstrual cycle phase, (3) confirmation of ovulation by lutropin surge in urine, and (4) vaginal ultrasounds to confirm and determine time of ovulation by measuring follicle size. Applying these methods in combination does require time and financial investment, but doing so will provide critical information for staging the menstrual cycle phase of each individual participant.
In summary, there is now strong scientific evidence for the influence of ovarian hormone fluctuations on brain connectivity, neurochemical pathways, and depression psychophysiology. The female populations that most critically require scientific attention often first appear too complicated and ‘messy’ to investigate, and thus are often systematically excluded from scientific research. Here, we have only begun to describe the existing resources that make these studies feasible, and encourage further discussion on how to address populations who most direly need scientific understanding.
Outlook
In summary, PET is a tool providing us with unique insight into how ovarian hormones trigger neuroplastic changes through their interactions with neurotransmitter systems and how these interactions can influence the transition from a potential individual vulnerability for depression to a diseased state. This is especially important because there is no current standard approach based on neurobiological evidence for preventing or treating major depression onset in post-partum or during the menopause transition, which takes the unique hormonal environment during which symptoms occur into consideration.
We require more targets specifically dedicated to the ovarian hormone receptors in the CNS. [18F]-FES is a PET tracer that is used in clinical practice to image the oestrogen receptor (ER) in breast cancer and the most widely studied ER (Lin et al., Citation2017; Venema et al., Citation2017), but its potential suitability for imaging of ER in the human brain has not yet been systematically investigated. The ER consists of two isoforms, ERα and ERβ, which have distinct biologic functions. Whereas activation of ERα stimulates cell proliferation and cell survival, ERβ promotes apoptosis. While selective imaging of ERα can be done effectively with [18F]FES, synthesis and evaluation of a potential ERβ-selective PET tracer: 2-18F-fluoro-6-(6-hydroxynaphthalen-2-yl)pyridin-3-ol (18F-FHNP) has recently been introduced (Antunes, van Waarde, et al., Citation2017; Antunes, Willemsen, et al., Citation2017).
Two newer approaches to get at aromatase imaging are 11C-cetrozole and [N-methyl-11C-vorozole]. Aromatase converts androgens to oestrogens, thus allowing us to control local oestrogen synthesis. In addition, it is challenging to measure local concentrations of oestrogen in the brain, and so tracers for this enzyme provide insight onto the dynamics. Ovarian uptake of 11C-vorozole did vary across the menstrual cycle in pre-menopausal women; however, regional brain uptake did not (Biegon et al., Citation2015). The group did observe significant menstrual cycle-dependent changes in modelled kinetic parameters in female baboon brains (Pareto et al., Citation2013) and found significant differences between men and women (Biegon et al., Citation2015). It remains to be tested in a larger sample and applying accurate menstrual cycle staging whether 11C-vorozole brain uptake is subject to endogenous shifts in steroid hormone environment in humans. 11C-cetrozole shows a promising signal-to-noise ratio in the amygdala, hypothalamus, and nucleus accumbens in the brain of non-human primates (Takahashi et al., Citation2014), but remains to be tested in humans.
As depression is becoming more and more a ‘multimodal’ condition, we require a multimodal solution. Integration of PET with other techniques and research methods would vastly improve identification of depression-related brain abnormalities. The MR-PET Hybrid scanner is an exceptional example, allowing both structural and functional brain mechanisms to be examined. This is especially important if one is looking at ovarian hormones in the menstrual cycle, as ovulation lasts ∼24 h, thus providing only a short and dynamic time-window, which, using simultaneous scanning protocol, seems much more feasible to map.
As there is likely an interaction between hormonal changes, neurochemical processes, and individual vulnerability, PET research may also be combined with genetic risk investigation in specific hormonal phases. One study in PMDD women found preliminary evidence in an emotion processing task where there was an interaction between the less functional variant of the brain derived neurotropic factor polymorphism: brain derived neurotropic factor (BDNF) Val66Met and decreased fronto-cingulate activity during the luteal phase. In healthy women, the COMT Val158Met genotype has been shown to play a modulatory role on the association between oestradiol and pre-frontal cortex activity during memory tasks in healthy women (Jacobs & D’Esposito, Citation2011).
While PET is too expensive and invasive to be part of any routine diagnostic workup, it plays a significant role in informing conceptual treatment and medication decisions, and it can, thus, help in avoiding a more invasive treatment strategy in certain clinical populations. For example, PET studies that found MAO-A levels to be dysregulated in vulnerable populations, such as post-partum, suggest therapeutically targeting or compensating elevated MAO-A levels. In more sensitive populations, such as breastfeeding mothers post-partum, this could be as non-invasive as administering dietary amino acids that are precursors for the monoamines that are depleted as a result of MAO-A (Dowlati et al., Citation2017). In severe but less sensitive cases, such as during severe perimenopausal depressive episode with a previous MDD history, this could include inhibiting MAO-A or increasing multiple monoamines with anti-depressive augmentation strategies.
Since dose-occupancy studies are integral in pharmacotherapy, PET ligand studies are integral for guiding medication dosing, and can identify specific treatment needs for hormonal transition periods. For example, oestradiol treatment in depressed perimenopausal women has shown an antidepressant effect comparable to that observed with classical anti-depressant treatment. However, the same hormonal intervention did not effectively treat depression in post-menopausal women, suggesting that a different mechanism occurs during these different hormonal profiles in the life span.
Conclusion
Ovarian hormones, particularly oestrogen and progesterone, impact the neurochemical systems involved in emotional and cognitive functioning that are known to be altered in depression. However, we still lack an in-depth understanding of how hormones and neurochemical processes interact in the context of depression in patient populations. Neurotransmitter dysfunction, in relationship to endogenous hormonal changes across the female lifespan, can help us design, test, and personalize drug treatment for women. Studying PMDD, PPD, and perimenopausal depression provides a unique opportunity to gain insight into how sex hormones influence mood-regulation. The menstrual cycle can serve as a model of different vulnerability states, and it may shed light on the mechanisms of transition from a high-risk to a diseased state. Widespread hormonal contraceptive use needs to be urgently considered as a modulating factor of the delicate hormonal balance in the brain, as increasing evidence suggests depressive symptoms as a potential side-effect of hormonal contraceptive use (Skovlund, Mørch, & Lidegaard, Citation2017). It is clear that the unique quantitative information PET imaging can provide during hormonal transition periods across the female lifespan has important implications for the field of mood disorders, and it may have the potential to transform individual care in female mental health.
Disclosure statement
The authors report no conflicts of interest. The authors alone are responsible for the content and writing of the paper.
References
- Adams, M.M., Fink, S.E., Janssen, W.G., Shah, R.A., & Morrison, J.H. (2004). Estrogen modulates synaptic N‐methyl‐D‐aspartate receptor subunit distribution in the aged hippocampus. Journal of Comparative Neurology, 474, 419–426.doi:10.1002/cne.20148
- Aiello, M., Salvatore, E., Cachia, A., Pappatà, S., Cavaliere, C., Prinster, A., … Quarantelli, M. (2015). Relationship between simultaneously acquired resting-state regional cerebral glucose metabolism and functional MRI: A PET/MR hybrid scanner study. Neuroimage, 113, 111–121. doi:10.1016/j.neuroimage.2015.03.017
- Angst, J., & Dobler-Mikola, A. (1984). Do the diagnostic criteria determine the sex ratio in depression? Journal of Affective Disorders, 7, 189–198.doi:10.1016/0165-0327(84)90040-5
- Antunes, I.F., van Waarde, A., Dierckx, R.A., de Vries, E.G., Hospers, G.A., & de Vries, E.F. (2017). Synthesis and evaluation of the estrogen receptor β–selective radioligand 2-18F-fluoro-6-(6-Hydroxynaphthalen-2-yl) pyridin-3-ol: Comparison with 16α-18F-Fluoro-17β-Estradiol. Journal of Nuclear Medicine, 58, 554–559. doi:10.2967/jnumed.116.180158
- Antunes, I.F., Willemsen, A.T., Sijbesma, J.W., Boerema, A.S., van Waarde, A., Glaudemans, A.W., … de Vries, E.F. (2017). In vivo quantification of ER-β expression by pharmacokinetic modeling: Studies with 18F-FHNP PET. Journal of Nuclear Medicine, 58, 1743–1748. doi:10.2967/jnumed.117.192666
- Arélin, K., Mueller, K., Barth, C., Rekkas, P.V., Kratzsch, J., Burmann, I. … Sacher, J. (2015). Progesterone mediates brain functional connectivity changes during the menstrual cycle: A pilot resting state MRI study. Frontiers in Neuroscience, 9, 44. doi:10.3389/fnins.2015.00044
- Atzil, S., Touroutoglou, A., Rudy, T., Salcedo, S., Feldman, R., Hooker, J.M., … Barrett, L.F. (2017). Dopamine in the medial amygdala network mediates human bonding. Proceedings of the National Academy of Sciences, 114, 2361–2366. doi:10.1073/pnas.1612233114
- Bäckström, T., Andreen, L., Birzniece, V., Björn, I., Johansson, I.M., Nordenstam-Haghjo, M., … Zhu, D. (2003). The role of hormones and hormonal treatments in premenstrual syndrome. CNS Drugs, 17, 325–342. doi:10.2165/00023210-200317050-00003
- Bäckström, T., Bixo, M., Johansson, M., Nyberg, S., Ossewaarde, L., Ragagnin, G., … van Wingen, G. (2014). Allopregnanolone and mood disorders. Progress in Neurobiology, 113, 88–94. doi:10.1016/j.pneurobio.2013.07.005
- Barth, C., Steele, C.J., Mueller, K., Rekkas, V.P., Arélin, K., Pampel, A., … Sacher, J. (2016). In-vivo dynamics of the human hippocampus across the menstrual cycle. Scientific Reports, 6. doi:10.1038/srep32833
- Barth, C., Villringer, A., & Sacher, J. (2015). Sex hormones affect neurotransmitters and shape the adult female brain during hormonal transition periods. Frontiers in Neuroscience, 9. doi:10.3389/fnins.2015.00037
- Baxter, L.R., Phelps, M.E., Mazziotta, J.C., Schwartz, J.M., Gerner, R.H., Selin, C.E., & Sumida, R.M. (1985). Cerebral metabolic rates for glucose in mood disorders: Studies with positron emission tomography and fluorodeoxyglucose F 18. Archives of General Psychiatry, 42, 441–447.doi:10.1001/archpsyc.1985.01790280019002
- Baxter, L.R., Schwartz, J.M., Phelps, M.E., Mazziotta, J.C., Guze, B.H., Selin, C.E., … Sumida, R.M. (1989). Reduction of prefrontal cortex glucose metabolism common to three types of depression. Archives of General Psychiatry, 46, 243–250. doi:10.1001/archpsyc.1989.01810030049007
- Bench, C.J., Friston, K.J., Brown, R.G., Frackowiak, R.S.J., & Dolan, R.J. (1993). Regional cerebral blood flow in depression measured by positron emission tomography: the relationship with clinical dimensions. Psychological Medicine, 23, 579–590.doi:10.1017/S0033291700025368
- Bethea, C.L., Mirkes, S.J., Shively, C.A., & Adams, M.R. (2000). Steroid regulation of tryptophan hydroxylase protein in the dorsal raphe of macaques. Biological Psychiatry, 47, 562–576.doi:10.1016/S0006-3223(99)00156-0
- Biegon, A., Alexoff, D.L., Kim, S.W., Logan, J., Pareto, D., Schlyer, D., … Fowler, J.S. (2015). Aromatase imaging with [N-methyl-11C] vorozole PET in healthy men and women. Journal of Nuclear Medicine, 56, 580–585. doi:10.2967/jnumed.114.150383
- Brooks, J.O., Wang, P.W., Bonner, J.C., Rosen, A.C., Hoblyn, J.C., Hill, S.J., & Ketter, T.A. (2009). Decreased prefrontal, anterior cingulate, insula, and ventral striatal metabolism in medication-free depressed outpatients with bipolar disorder. Journal of Psychiatric Research, 43, 181–188. doi:10.1016/j.jpsychires.2008.04.015
- Buchsbaum, M.S., Joseph, W., Siegel, B.V., Hackett, E., Trenary, M., Abel, L., & Reynolds, C. (1997). Effect of sertraline on regional metabolic rate in patients with affective disorder. Biological Psychiatry, 41, 15–22.doi:10.1016/S0006-3223(96)00097-2
- Cahill, L., Haier, R.J., White, N.S., Fallon, J., Kilpatrick, L., Lawrence, C., … Alkire, M.T. (2001). Sex-related difference in amygdala activity during emotionally influenced memory storage. Neurobiology of Learning and Memory, 75, 1–9. doi:10.1006/nlme.2000.3999
- Chen, Q., Liu, W., Li, H., Zhang, H., & Tian, M. (2011). Molecular imaging in patients with mood disorders: a review of PET findings. European Journal of Nuclear Medicine and Molecular Imaging, 38, 1367–1380.doi:10.1007/s00259-011-1779-z
- Costes, N., Merlet, I., Ostrowsky, K., Faillenot, I., Lavenne, F., Zimmer, L., … Le Bars, D. (2005). A 18F-MPPF PET normative database of 5-HT1A receptor binding in men and women over aging. Journal of Nuclear Medicine, 46, 1980–1989.Retrieved from http://jnm.snmjournals.org/content/46/12/1980.full
- Cuthbert, B.N. (2014). The RDoC framework: Facilitating transition from ICD/DSM to dimensional approaches that integrate neuroscience and psychopathology. World Psychiatry, 13, 28–35. Retrieved from http://doi.org/10.1002/wps.20087
- Deecher, D., Andree, T.H., Sloan, D., & Schechter, L.E. (2008). From menarche to menopause: Exploring the underlying biology of depression in women experiencing hormonal changes. Psychoneuroendocrinology, 33, 3–17.doi:10.1016/j.psyneuen.2007.10.006
- Delgado, P.L. (2000). Depression: The case for a monoamine deficiency. The Journal of Clinical Psychiatry, 61(Suppl 6), 7–11.Retrieved from https://www.ncbi.nlm.nih.gov/pubmed/10775018
- Di Paolo, T., Falardeau, P., & Morissette, M. (1988). Striatal D-2 dopamine agonist binding sites fluctuate during the rat estrous cycle. Life Sciences, 43, 665–672.doi:10.1016/0024-3205(88)90137-3
- Dowlati, Y., Ravindran, A.V., Segal, Z.V., Stewart, D.E., Steiner, M., & Meyer, J.H. (2017). Selective dietary supplementation in early postpartum is associated with high resilience against depressed mood. Proceedings of the National Academy of Sciences, 114, 3509–3514.doi:10.1073/pnas.1611965114
- Drevets, W.C., Gautier, C., Price, J.C., Kupfer, D.J., Kinahan, P.E., Grace, A.A., … Mathis, C.A. (2001). Amphetamine-induced dopamine release in human ventral striatum correlates with euphoria. Biological Psychiatry, 49, 81–96. doi:10.1016/S0006-3223(00)01038-6
- Drevets, W.C., Price, J.L., Bardgett, M.E., Reich, T., Todd, R.D., & Raichle, M.E. (2002). Glucose metabolism in the amygdala in depression: Relationship to diagnostic subtype and plasma cortisol levels. Pharmacology Biochemistry and Behavior, 71, 431–447.doi:10.1016/S0091-3057(01)00687-6
- Drevets, W.C., Price, J.L., Simpson, J.R., Jr., Todd, R.D., Reich, T., Vannier, M., & Raichle, M.E. (1997). Subgenual prefrontal cortex abnormalities in mood disorders. Nature, 386, 824. doi:10.1038/386824a0
- Dunlop, B.W., & Nemeroff, C.B. (2007). The role of dopamine in the pathophysiology of depression. Archives of general psychiatry, 64, 327–337.doi:10.1001/archpsyc.64.3.327
- Eriksson, O., Wall, A., Marteinsdottir, I., Ågren, H., Hartvig, P., Blomqvist, G., … Naessén, T. (2006). Mood changes correlate to changes in brain serotonin precursor trapping in women with premenstrual dysphoria. Psychiatry Research: Neuroimaging, 146, 107–116. doi:10.1016/j.pscychresns.2005.02.012
- FitzGerald, M., Malone, K.M., Li, S., Harrison, W.M., McBride, P.A., Endicott, J., … Mann, J.J. (1997). Blunted serotonin response to fenfluramine challenge in premenstrual dysphoric disorder. American Journal of Psychiatry, 154, 556–558. doi:10.1176/ajp.154.4.556
- Frank, E., Carpenter, L.L., & Kupfer, D.J. (1988). Sex differences in recurrent depression: are there any that are significant?. The American Journal of Psychiatry, 145, 41. doi:10.1176/ajp.145.1.41
- Freeman, E.W., Sammel, M.D., Boorman, D.W., & Zhang, R. (2014). Longitudinal pattern of depressive symptoms around natural menopause. JAMA Psychiatry, 71, 36–43.doi:10.1001/jamapsychiatry.2013.2819
- Freeman, E.W., Sammel, M.D., Lin, H., & Nelson, D.B. (2006). Associations of hormones and menopausal status with depressed mood in women with no history of depression. Archives of General Psychiatry, 63, 375–382.doi:10.1001/archpsyc.63.4.375
- Frey, B.N., Skelin, I., Sakai, Y., Nishikawa, M., & Diksic, M. (2010). Gender differences in α-[11 C] MTrp brain trapping, an index of serotonin synthesis, in medication-free individuals with major depressive disorder: A positron emission tomography study. Psychiatry Research: Neuroimaging, 183, 157–166. doi:10.1016/j.pscychresns.2010.05.005
- Frokjaer, V.G., Pinborg, A., Holst, K.K., Overgaard, A., Henningsson, S., Heede, M., … Stenbaek, D.S. (2015). Role of serotonin transporter changes in depressive responses to sex-steroid hormone manipulation: A positron emission tomography study. Biological Psychiatry, 78, 534–543. doi:10.1016/j.biopsych.2015.04.015
- Gavin, N.I., Gaynes, B.N., Lohr, K.N., Meltzer-Brody, S., Gartlehner, G., & Swinson, T. (2005). Perinatal depression: a systematic review of prevalence and incidence. Obstetrics & Gynecology, 106, 1071–1083.doi:10.1097/01.AOG.0000183597.31630.db
- Gazzaley, A.H., Weiland, N.G., McEwen, B.S., & Morrison, J.H. (1996). Differential regulation of NMDAR1 mRNA and protein by estradiol in the rat hippocampus. Journal of Neuroscience, 16, 6830–6838. Retrieved from http://www.jneurosci.org/content/16/21/6830.short
- Gundlah, C., Lu, N.Z., & Bethea, C.L. (2002). Ovarian steroid regulation of monoamine oxidase-A and -B mRNAs in the macaque dorsal raphe and hypothalamic nuclei. Psychopharmacology (Berlin), 160, 271–282.doi:10.1007/s00213-001-0959-0
- Hasler, G., Fromm, S., Carlson, P.J., Luckenbaugh, D.A., Waldeck, T., Geraci, M., … Drevets, W.C. (2008). Neural response to catecholamine depletion in unmedicated subjects with major depressive disorder in remission and healthy subjects. Archives of General Psychiatry, 65, 521–531. doi:10.1001/archpsyc.65.5.521
- Hausmann, M., & Güntürkün, O. (2000). Steroid fluctuations modify functional cerebral asymmetries: The hypothesis of progesterone-mediated interhemispheric decoupling. Neuropsychologia, 38, 1362–1374. doi:10.1016/S0028-3932(00)00045-2
- Henderson, J.A., & Bethea, C.L. (2008). Differential effects of ovarian steroids and raloxifene on serotonin 1A and 2C receptor protein expression in macaques. Endocrine, 33, 285–293.doi:10.1007/s12020-008-9087-5
- Hosokawa, T., Momose, T., & Kasai, K. (2009). Brain glucose metabolism difference between bipolar and unipolar mood disorders in depressed and euthymic states. Progress in Neuro-Psychopharmacology and Biological Psychiatry, 33, 243–250.doi:10.1016/j.pnpbp.2008.11.014
- Jacobs, E., & D’esposito, M. (2011). Estrogen shapes dopamine-dependent cognitive processes: Implications for women’s health. Journal of Neuroscience, 31, 5286–5293. doi:10.1523/JNEUROSCI.6394-10.2011
- Jovanovic, H., Cerin, Å., Karlsson, P., Lundberg, J., Halldin, C., & Nordström, A.L. (2006). A PET study of 5-HT 1A receptors at different phases of the menstrual cycle in women with premenstrual dysphoria. Psychiatry Research: Neuroimaging, 148, 185–193. doi:10.1016/j.pscychresns.2006.05.002
- Jovanovic, H., Karlsson, P., Cerin, Å., Halldin, C., & Nordström, A.L. (2009). 5-HT 1A receptor and 5-HTT binding during the menstrual cycle in healthy women examined with [11 C] WAY100635 and [11 C] MADAM PET. Psychiatry Research: Neuroimaging, 172, 31–37. doi:10.1016/j.pscychresns.2008.07.002
- Jovanovic, H., Lundberg, J., Karlsson, P., Cerin, Å., Saijo, T., Varrone, A., … Nordström, A.L. (2008). Sex differences in the serotonin 1A receptor and serotonin transporter binding in the human brain measured by PET. Neuroimage, 39, 1408–1419. doi:10.1016/j.neuroimage.2007.10.016
- Kennedy, S.H., Evans, K.R., Krüger, S., Mayberg, H.S., Meyer, J.H., McCann, S., … Vaccarino, F.J. (2001). Changes in regional brain glucose metabolism measured with positron emission tomography after paroxetine treatment of major depression. American Journal of Psychiatry, 158, 899–905. doi:10.1176/appi.ajp.158.6.899
- Kessler, R.C. (2003). Epidemiology of women and depression. Journal of Affective Disorders, 74, 5–13.doi:10.1016/S0165-0327(02)00426-3
- Kessler, R.C., McGonagle, K.A., Zhao, S., Nelson, C.B., Hughes, M., Eshleman, S., … Kendler, K.S. (1994). Lifetime and 12-month prevalence of DSM-III-R psychiatric disorders in the United States: results from the National Comorbidity Survey. Archives of General Psychiatry, 51, 8–19. doi:10.1001/archpsyc.1994.03950010008002
- Ketter, T.A., Kimbrell, T.A., George, M.S., Dunn, R.T., Speer, A.M., Benson, B.E., … Post, R.M. (2001). Effects of mood and subtype on cerebral glucose metabolism in treatment-resistant bipolar disorder. Biological Psychiatry, 49, 97–109. doi:10.1016/S0006-3223(00)00975-6
- Kimbrell, T.A., Ketter, T.A., George, M.S., Little, J.T., Benson, B.E., Willis, M.W., … Post, R.M. (2002). Regional cerebral glucose utilization in patients with a range of severities of unipolar depression. Biological Psychiatry, 51, 237–252. doi:10.1016/S0006-3223(01)01216-1
- Konarski, J.Z., McIntyre, R.S., Soczynska, J.K., Bottas, A., & Kennedy, S.H. (2006). Clinical translation of neuroimaging research in mood disorders. Psychiatry (Edgmont), 3, 46. Retrieved from https://www.ncbi.nlm.nih.gov/pmc/articles/PMC2990553/
- Koob, G.F., Everitt, B.J., & Robbins, T.W. (2013). Reward, motivation, and addiction. In Fundamental Neuroscience. 3rd ed. Amsterdam: Academic Press; 987–1016.
- Kornstein, S.G., Schatzberg, A.F., Thase, M.E., Yonkers, K.A., McCullough, J.P., Keitner, G.I., … Davis, S.M. (2000). Gender differences in chronic major and double depression. Journal of Affective Disorders, 60, 1–11. doi:10.1016/S0165-0327(99)00158-5
- Laakso, A., Vilkman, H., Örgen Bergman, J., Haaparanta, M., Solin, O., Syvälahti, E., … Hietala, J. (2002). Sex differences in striatal presynaptic dopamine synthesis capacity in healthy subjects. Biological Psychiatry, 52, 759–763. doi:10.1016/S0006-3223(02)01369-0
- Le Strat, Y., Dubertret, C., & Le Foll, B. (2011). Prevalence and correlates of major depressive episode in pregnant and postpartum women in the United States. Journal of Affective Disorders, 135, 128–138.doi:10.1016/j.jad.2011.07.004
- Lévesque, D., Gagnon, S., & Di Paolo, T. (1989). Striatal D 1 dopamine receptor density fluctuates during the rat estrous cycle. Neuroscience Letters, 98, 345–350. doi:10.1016/0304-3940(89)90426-6
- Lin, F.I., Gonzalez, E.M., Kummar, S., Do, K., Shih, J., Adler, S., … Bhattacharyya, S. (2017). Utility of 18F-fluoroestradiol (18F-FES) PET/CT imaging as a pharmacodynamic marker in patients with refractory estrogen receptor-positive solid tumors receiving Z-endoxifen therapy. European Journal of Nuclear Medicine and Molecular Imaging, 44, 500–508. doi:10.1007/s00259-016-3561-8
- Lisofsky, N., Mårtensson, J., Eckert, A., Lindenberger, U., Gallinat, J., & Kühn, S. (2015). Hippocampal volume and functional connectivity changes during the female menstrual cycle. Neuroimage, 118, 154–162. doi:10.1016/j.neuroimage.2015.06.012
- Lu, N.Z., & Bethea, C.L. (2002). Ovarian steroid regulation of 5-HT1A receptor binding and G protein activation in female monkeys. Neuropsychopharmacology, 27, 12–24.doi:10.1016/S0893-133X(01)00423-7
- Mehta, D., Newport, D.J., Frishman, G., Kraus, L., Rex-Haffner, M., Ritchie, J.C., … Stowe, Z.N. (2014). Early predictive biomarkers for postpartum depression point to a role for estrogen receptor signaling. Psychological Medicine, 44, 2309–2322. doi:10.1017/S0033291713003231
- Meltzer, C.C., Drevets, W.C., Price, J.C., Mathis, C.A., Lopresti, B., Greer, P.J., … Reynolds, C.F. (2001). Gender-specific aging effects on the serotonin 1A receptor. Brain Research, 895, 9–17. doi:10.1016/S0006-8993(00)03211-X
- Meyer, J.H., Ginovart, N., Boovariwala, A., Sagrati, S., Hussey, D., Garcia, A., … Houle, S. (2006). Elevated monoamine oxidase a levels in the brain: An explanation for the monoamine imbalance of major depression. Archives of General Psychiatry, 63, 1209–1216. doi:10.1001/archpsyc.63.11.1209
- Meyer, J.H., Houle, S., Sagrati, S., Carella, A., Hussey, D.F., Ginovart, N., … Wilson, A.A. (2004). Brain serotonin transporter binding potential measured with carbon 11–labeled DASB positron emission tomography: Effects of major depressive episodes and severity of dysfunctional attitudes. Archives of General Psychiatry, 61, 1271–1279. doi:10.1001/archpsyc.61.12.1271
- Meyer, J.H., Wilson, A.A., Sagrati, S., Miler, L., Rusjan, P., Bloomfield, P.M., … Houle, S. (2009). Brain monoamine oxidase A binding in major depressive disorder: Relationship to selective serotonin reuptake inhibitor treatment, recovery, and recurrence. Archives of General Psychiatry, 66, 1304–1312. doi:10.1001/archgenpsychiatry.2009.156
- Mitchell, E.S., Woods, N.F., & Mariella, A. (2000). Three stages of the menopausal transition from the Seattle Midlife Women’s Health Study: Toward a more precise definition. Menopause, 7, 334–349. Retrieved from https://www.ncbi.nlm.nih.gov/pubmed/10993033
- Moreno, F.A., McGahuey, C.A., Freeman, M.P., & Delgado, P.L. (2006). Sex differences in depressive response during monoamine depletions in remitted depressive subjects. The Journal of Clinical Psychiatry, 67, 1618–1623.doi:10.4088/JCP.v67n1019
- Moses-Kolko, E.L., Price, J.C., Wisner, K.L., Hanusa, B.H., Meltzer, C.C., Berga, S.L., … Drevets, W.C. (2012). Postpartum and depression status are associated with lower [11C] raclopride BPND in reproductive-age women. Neuropsychopharmacology, 37, 1422. doi:10.1038/npp.2011.328
- Moses-Kolko, E.L., Wisner, K.L., di Scalea, T.L., Kaye, W.H., Price, J.C., Grace, A.A., … Becker, C. (2006). Reduced ventral striatal D2/D3 receptor binding in depressed reproductive-aged women: A [C-11] raclopride positron emission tomography study. In Neuropsychopharmacology (Vol.31, pp. S87–S88). London, UK: Nature Publishing Group.
- Moses-Kolko, E.L., Wisner, K.L., Price, J.C., Berga, S.L., Drevets, W.C., Hanusa, B.H., … Meltzer, C.C. (2008). Serotonin 1A receptor reductions in postpartum depression: A positron emission tomography study. Fertility and Sterility, 89, 685–692. doi:10.1016/j.fertnstert.2007.03.059
- Munro, C.A., McCaul, M.E., Wong, D.F., Oswald, L.M., Zhou, Y., Brasic, J., … Wand, G.S. (2006). Sex differences in striatal dopamine release in healthy adults. Biological Psychiatry, 59, 966–974. doi:10.1016/j.biopsych.2006.01.008
- Murphy, D.D., Cole, N.B., Greenberger, V., & Segal, M. (1998). Estradiol increases dendritic spine density by reducing GABA neurotransmission in hippocampal neurons. Journal of Neuroscience, 18, 2550–2559. Retrieved from https://www.ncbi.nlm.nih.gov/pubmed/9502814
- National Institutes of Health. (2015). Consideration of Sex as a Biological Variable in NIH-funded Research. Retrieved from https://grants.nih.gov/grants/guide/notice-files/NOT-OD-15-102.html
- Nestler, E.J., & Carlezon, W.A. (2006). The mesolimbic dopamine reward circuit in depression. Biological Psychiatry, 59, 1151–1159.doi:10.1016/j.biopsych.2005.09.018
- Nishizawa, S., Benkelfat, C., Young, S.N., Leyton, M., Mzengeza, S.D., De Montigny, C., … Diksic, M. (1997). Differences between males and females in rates of serotonin synthesis in human brain. Proceedings of the National Academy of Sciences, 94, 5308–5313. Retrieved from https://www.ncbi.nlm.nih.gov/pmc/articles/PMC24674/
- O’hara, M.W., Stuart, S., Gorman, L.L., & Wenzel, A. (2000). Efficacy of interpersonal psychotherapy for postpartum depression. Archives of General Psychiatry, 57, 1039–1045. doi:10.1001/archpsyc.57.11.1039
- O’Higgins, M., Roberts, I.S.J., Glover, V., & Taylor, A. (2013). Mother-child bonding at 1 year; associations with symptoms of postnatal depression and bonding in the first few weeks. Archives of Women’s Mental Health, 16, 381–389. doi:10.1007/s00737-013-0354-y
- Pareto, D., Biegon, A., Alexoff, D., Carter, P., Shea, C., Muench, L., … Logan, J. (2013). In vivo imaging of brain aromatase in female baboons: [11C] vorozole kinetics and effect of the menstrual cycle. Molecular Imaging, 12, 7290–2013. doi:10.2310/7290.2013.00068
- Parsey, R.V., Olvet, D.M., Oquendo, M.A., Yung-Yu, H., Ogden, R.T., & Mann, J.J. (2006). Higher 5-HT1A receptor binding potential during a major depressive episode predicts poor treatment response: Preliminary data from a naturalistic study. Neuropsychopharmacology, 31, 1745. doi:10.1038/sj.npp.1300992
- Parsey, R.V., Oquendo, M.A., Ogden, R.T., Olvet, D.M., Simpson, N., Huang, Y.Y., … Mann, J.J. (2006). Altered serotonin 1A binding in major depression: a [carbonyl-C-11] WAY100635 positron emission tomography study. Biological Psychiatry, 59, 106–113. doi:10.1016/j.biopsych.2005.06.016
- Parsey, R.V., Oquendo, M.A., Simpson, N.R., Ogden, R.T., Van Heertum, R., Arango, V., & Mann, J.J. (2002). Effects of sex, age, and aggressive traits in man on brain serotonin 5-HT 1A receptor binding potential measured by PET using [C-11] WAY-100635. Brain Research, 954, 173–182.doi:10.1016/S0006-8993(02)03243-2
- Poobalan, A.S., Aucott, L.S., Ross, L., Smith, W.C.S., Helms, P.J., & Williams, J.H. (2007). Effects of treating postnatal depression on mother–infant interaction and child development. The British Journal of Psychiatry, 191, 378–386.doi:10.1192/bjp.bp.106.032789
- Rekkas, P.V., Wilson, A.A., Lee, V.W.H., Yogalingam, P., Sacher, J., Rusjan, P., … Chiuccariello, L. (2014). Greater monoamine oxidase A binding in perimenopausal age as measured with carbon 11–labeled harmine positron emission tomography. JAMA Psychiatry, 71, 873–879. doi:10.1001/jamapsychiatry.2014.250
- Rosa-Neto, P., Diksic, M., Okazawa, H., Leyton, M., Ghadirian, N., Mzengeza, S., … Benkelfat, C. (2004). Measurement of brain regional α-[11C] Methyl-L-tryptophan trapping as a measure of serotonin synthesis in medication-freepatients with major depression. Archives of General Psychiatry, 61, 556–563. doi:10.1001/archpsyc.61.6.556
- Sabri, O., Seibyl, J., Rowe, C., & Barthel, H. (2015). Beta-amyloid imaging with florbetaben. Clinical and translational imaging, 3, 13–26. doi:10.2967/jnumed.117.193649
- Sacher, J., Rekkas, P.V., Wilson, A.A., Houle, S., Romano, L., Hamidi, J., … Meyer, J.H. (2015). Relationship of monoamine oxidase-A distribution volume to postpartum depression and postpartum crying. Neuropsychopharmacology, 40, 429. doi:10.1038/npp.2014.190
- Sacher, J., Wilson, A.A., Houle, S., Rusjan, P., Hassan, S., Bloomfield, P.M., … Meyer, J.H. (2010). Elevated brain monoamine oxidase A binding in the early postpartum period. Archives of General Psychiatry, 67, 468–474. doi:10.1001/archgenpsychiatry.2010.32
- Sakai, Y., Nishikawa, M., Leyton, M., Benkelfat, C., Young, S.N., & Diksic, M. (2006). Cortical trapping of α-[11 C] methyl-L-tryptophan, an index of serotonin synthesis, is lower in females than males. Neuroimage, 33, 815–824. doi:10.1016/j.neuroimage.2006.08.004
- Serafini, G. (2012). Neuroplasticity and major depression, the role of modern antidepressant drugs. World Journal of Psychiatry, 2, 49. doi:10.5498/wjp.v2.i3.49
- Shimizu, H., & Bray, G.A. (1993). Effects of castration, estrogen replacement and estrus cycle on monoamine metabolism in the nucleus accumbens, measured by microdialysis. Brain Research, 621, 200–206.doi:10.1016/0006-8993(93)90107-X
- Sit, D.K., & Wisner, K.L. (2009). The identification of postpartum depression. Clinical obstetrics and gynecology, 52, 456. doi:10.1097/GRF.0b013e3181b5a57c
- Skovlund, C.W., Mørch, L.S., & Lidegaard, Ø. (2017). Hormonal contraception and its association with Depression-Reply. Jama Psychiatry, 74, 302–303. doi:10.1001/jamapsychiatry.2016.3865
- Smith, S.S., & Woolley, C.S. (2004). Cellular and molecular effects of steroid hormones on CNS excitability. Cleveland Clinic Journal of Medicine, 71, S4. doi:10.3949/ccjm.71.Suppl_2.S4
- Soares, C.N., & Zitek, B. (2008). Reproductive hormone sensitivity and risk for depression across the female life cycle: A continuum of vulnerability?. Journal of Psychiatry & Neuroscience: JPN, 33, 331. Retrieved from https://www.ncbi.nlm.nih.gov/pmc/articles/PMC2440795/
- Sohr-Preston, S.L., & Scaramella, L.V. (2006). Implications of timing of maternal depressive symptoms for early cognitive and language development. Clinical Child and Family Psychology Review, 9, 65–83.doi:10.1007/s10567-006-0004-2
- Staley, J.K., Krishnan-Sarin, S., Zoghbi, S., Tamagnan, G., Fujita, M., … Innis, R.B. (2001). Sex differences in [123I] β‐CIT SPECT measures of dopamine and serotonin transporter availability in healthy smokers and nonsmokers. Synapse, 41, 275–284. doi:10.1002/syn.1084
- Stewart, J.L., & Paulus, M.P. (2013). Neural correlates of craving for psychoactive drugs. Principles of Addiction: Comprehensive Addictive Behaviors and Disorders, 1, 453.
- Su, T.P., Schmidt, P.J., Danaceau, M., Murphy, D.L., & Rubinow, D.R. (1997). Effect of menstrual cycle phase on neuroendocrine and behavioral responses to the serotonin agonist m-chlorophenylpiperazine in women with premenstrual syndrome and controls. The Journal of Clinical Endocrinology & Metabolism, 82, 1220–1228.doi:10.1210/jc.82.4.1220
- Takahashi, K., Hosoya, T., Onoe, K., Doi, H., Nagata, H., Hiramatsu, T., … Suzuki, M. (2014). 11C-cetrozole: An improved C-11C-methylated PET probe for aromatase imaging in the brain. Journal of Nuclear Medicine, 55, 852–857. doi:10.2967/jnumed.113.131474
- Van Wingen, G.A., Van Broekhoven, F., Verkes, R.J., Petersson, K.M., Bäckström, T., Buitelaar, J.K., & Fernandez, G. (2008). Progesterone selectively increases amygdala reactivity in women. Molecular Psychiatry, 13, 325. doi:10.1038/sj.mp.4002030
- Venema, C.M., Mammatas, L.H., van Kruchten, M., Apollonio, G., Schroder, C.P., Glaudemans, A.W., … De Vries, E. (2017). Androgen receptor and estrogen receptor imaging in patients with metastatic breast cancer. Journal of Nuclear Medicine. Advance online publication. doi:10.2967/jnumed.117.193649
- Videbech, P., Ravnkilde, B., Pedersen, A.R., Egander, A., Landbo, B., Rasmussen, N.A., … Rosenberg, R. (2001). The Danish PET/depression project: PET findings in patients with major depression. Psychological Medicine, 31, 1147–1158. doi:10.1017/S0033291701004469
- Videbech, P., Ravnkilde, B., Pedersen, T.H., Hartvig, H., Egander, A., Clemmensen, K., … Rosenberg, R. (2002). The Danish PET/depression project: Clinical symptoms and cerebral blood flow. A regions‐of‐interest analysis. Acta Psychiatrica Scandinavica, 106, 35–44. doi:10.1034/j.1600-0447.2002.02245.x
- Wainwright, S.R., & Galea, L.A. (2013). The neural plasticity theory of depression: assessing the roles of adult neurogenesis and PSA-NCAM within the hippocampus. Neural Plasticity, 2013, 805497. doi:10.1155/2013/805497
- Wegienka, G., & Baird, D.D. (2005). A comparison of recalled date of last menstrual period with prospectively recorded dates. Journal of Women’s Health, 14, 248–252. doi:10.1089/jwh.2005.14.248
- Weikum, W.M., Oberlander, T.F., Hensch, T.K., & Werker, J.F. (2012). Prenatal exposure to antidepressants and depressed maternal mood alter trajectory of infant speech perception. Proceedings of the National Academy of Sciences, 109(Suppl 2), 17221–17227.doi:10.1073/pnas.1121263109
- Weissman, M.M., Bland, R., Joyce, P.R., Newman, S., Wells, J.E., & Wittchen, H.U. (1993). Sex differences in rates of depression: Cross-national perspectives. Journal of Affective Disorders, 29, 77–84.doi:10.1016/0165-0327(93)90025-F
- Whitton, A.E., Treadway, M.T., & Pizzagalli, D.A. (2015). Reward processing dysfunction in major depression, bipolar disorder and schizophrenia. Current Opinion in Psychiatry, 28, 7. doi:10.1097/YCO.0000000000000122
- Willner, P., Hale, A.S., & Argyropoulos, S. (2005). Dopaminergic mechanism of antidepressant action in depressed patients. Journal of Affective Disorders, 86, 37–45.doi:10.1016/j.jad.2004.12.010
- World Health Organization. (2008). The global burden of disease: 2004 update. 1st ed. Geneva: World Health Organization.
- Xiao, L., & Becker, J.B. (1994). Quantitative microdialysis determination of extracellular striatal dopamine concentration in male and female rats: Effects of estrous cycle and gonadectomy. Neuroscience Letters, 180, 155–158.doi:10.1016/0304-3940(94)90510-X
- Youdim, M.B., Edmondson, D., & Tipton, K.F. (2006). The therapeutic potential of monoamine oxidase inhibitors. Nature Reviews. Neuroscience, 7, 295. doi:10.1038/nrn1883
- Young, M.A., Scheftner, W.A., Fawcett, J., & Klerman, G.L. (1990). Gender differences in the clinical features of unipolar major depressive disorder. Journal of Nervous and Mental Disease, 178, 200–203.doi:10.1097/00005053-199003000-00008
- Young, S.N., Gauthier, S.E.R.G.E., Anderson, G.M., & Purdy, W.C. (1980). Tryptophan, 5-hydroxyindoleacetic acid and indoleacetic acid in human cerebrospinal fluid: Interrelationships and the influence of age, sex, epilepsy and anticonvulsant drugs. Journal of Neurology, Neurosurgery & Psychiatry, 43, 438–445.doi:10.1136/jnnp.43.5.438