Abstract
Constructed reed wetland microcosms (CRWs) in a lab of east China have been irrigated with bleaching wastewater per month for a reed growth season. The soil physicochemical properties, enzyme activities (i.e. urease, invertase, polyphenol oxidase, alkaline phosphatase and cellulase) and soil microbial diversity were assayed before and after the exposure experiment. Compared to the river water irrigated controls (CKs), bleaching wastewater application has no marked influence on soil pH, but significantly increased soil Na+, total halogen and absorbable organic halogen (AOX) contents, which induced the increasing of soil electrical conductivity. Furthermore, soil enzyme activities displayed significant variation (except for polyphenol oxidase). Bleaching wastewater irrigation decreased Sorenson’s pairwise similarity coefficient (Cs), which indicated the changes of the structure of bacterial and fungal communities. However, only the diversity of bacterial community was inhibited and has no effect on the diversity of fungal community, as evidenced by the calculated Shannon–Wiener index (H).
1. Introduction
Freshwater resources are limited and a growing number of watersheds are facing water scarcity because of insufficient local water resources and reduced water quality due to changes in climate, urbanization, increasing population, rapid development of agricultural and industrial activities.[Citation1–3] In addition to water scarcity, discharge of massive volumes of industrial wastewater has become another important issue that needs to be addressed.[Citation4,5] Thus, it has to be urgent need to assess the environmental safety of industrial wastewater reuse.
Land application has become one of the widely applied methods in wastewater treatment practice.[Citation6,7] Substitution of good quality water by wastewater, richer in nutrients, is a key conservation strategy contributing to agricultural production.[Citation8] On the other hand, hazardous materials in wastewater can be inevitably sequestered in soil, which may pose potential risks to ecological environment and humans.[Citation9,10] For example, it was revealed that papermaking wastewater irrigation resulted in soil salinization, which was mainly due to the high sodium content of the wastewater applied for irrigation.[Citation5] However, Xia et al. [Citation11] found that papermaking wastewater irrigation would not only increase soil fertility but also significantly decrease soil salinity. Accordingly, there is no final conclusion about the utilization of wastewater in agricultural irrigation.
Pulp and paper making industry is one of the water intensive industries.[Citation12] A large volume of bleaching effluent has been generated from paper making process (800–100 m3/ton paper production). Bleaching wastewater contains dissolved lignin, carbohydrate, and inorganic ions and chloride compounds, making process for reuse difficult and uneconomical.[Citation12] It seems to be economically feasible to reuse the effluent for agricultural irrigation. Therefore risk management and interim solutions are needed to prevent adverse environmental and health impacts from wastewater irrigation.[Citation13,14]
In this study, constructed reed wetlands (CRWs) with horizontal surface flow were firstly constructed in a sunroom, and then the effects of bleaching wastewater irrigation on soil physiochemical properties were investigated. Furthermore, the impacts of bleaching wastewater irrigation on soil enzyme activities (cellulase, invertase, polyphenol oxidase, urease, and alkaline phosphatase) and diversity of soil microbial communities were also investigated, which provided important perspectives on the administration of bleaching wastewater irrigation.
2. Materials and methods
2.1. CRWs and theirs operation
The CRW microcosms with horizontal surface flow were located near Yancheng Biosphere Reserve, China. Four parallel pilot-scale CRWs (ST-1, ST-2, CK-1 and CK-2), each with a chamber (1.5 m × 0.8 m × 1.1 m (H)) (Figure ) were constructed in a sunroom. Soil, collected from the Reserve, was filled to a depth of 80 cm, and then reeds were transplanted to the chambers at a density of 8 plants/m2. The soil used to construct the CRWs was silty sand with the following physicochemical properties: pH 7.6 (1:2.5 w/v in water), 1.37% organic matter, and 0.67 g total N kg−1. Each CRW has a water inlet and no outlet, which resulted in a relatively closed system. After two years of cultivation with river water, the CRWs were used for the exposure experiment.
Figure 1. Diagram of the CRWs.
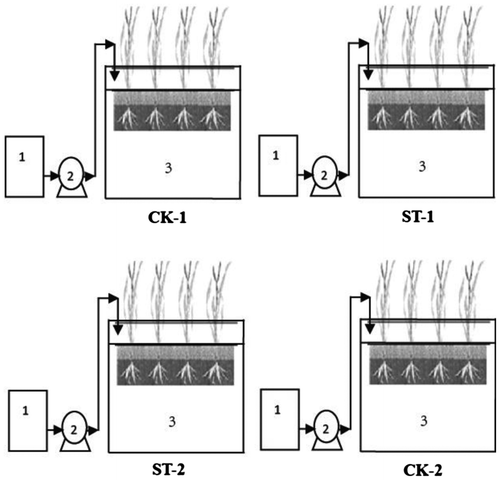
Soil samples were collected at three plots from each CRW in 8 April 2015, and then a volume of 70 L of bleaching wastewater was induced into ST-1 and ST-2 units per month. During the bleaching wastewater irrigation intervals, appropriate river water was added as there was no convincing show of ponding in the CRWs. The other two CRWs were selected as controls (CK-1 and CK-2), which were still irrigated with river water. One month before the harvest of reeds (17 October 2015), irrigation was stopped. Straight after the harvest, soil samples were collected at three plots in each CRW. The detailed sampling and preservation methods were described in our previous publication.[Citation5]
2.2. Analytic methods
Soil pH was measured in 1:2 of soil:water slurry using a glass electrode, as described by Datta et al. [Citation15] The soil organic matter and total nitrogen (TN) were assayed as described by Walkley [Citation16] and Page et al. [Citation17], respectively. Total phosphorus (TP) was determined by the NaOH fusion–Mo–Sb anti-spectrophotometric method. Soil electrical conductivity (EC) was determined in a 1:5 of soil:water slurry with the method described by Montoroi [Citation18].
The soil total halogen (TX) and absorbable organic halogen (AOX) were determined by coupling the AOX-analyzer with a multi X® 2500 (Analytik Jena, Germany). The detailed procedures of AOX assay were carried out as described by Oleksy-Frenzel et al. [Citation19].
The activities of urease (EC 3.5.1.5), invertase (EC 3.2.1.26), polyphenol oxidase (EC 1.10.3.1), alkaline phosphatase (AlkP; EC3.1.3.1) and cellulase (EC3.2.1.4) were assayed according to the methods described by Guan [Citation20]. Enzyme activities were determined in duplicate per sample and expressed by the dry weight equivalent soil. Soil moisture content was determined from the loss in weight after drying at 105 °C for 48 h.
Total DNA was extracted from 0.3 g soil according to our previous publication.[Citation21] The concentration of DNA was measured by Nanodrop (Eppendorf AG, Barkhausenweg, Hamburg, Germany), and then stored at 4 °C for further analysis. The PCR primers for V3 region of 16S and partial sequence (D1–D2 region) of 18SrDNA were synthesized according to the literatures.[Citation22,23]
PCR products were separated by denaturing gradient gel electrophoresis (DGGE) with a BioRad DCode Universal Mutation Detection System (BioRad, Richmond, CA, USA). Gel used in this study was 8% (w/v) polyacrylamide gel with a 40–60% urea-formamide denaturing gradient. Electrophoresis was carried out at 65 V, 60 °C for 16 h, and gel was stained about 30 min with SYBR Green I (1:10,000 v/v) (Molecular Probes, Eugene, UK).
The software Quantity One (Version 4.0.1, Bio-Rad, USA) was used to convert individual DGGE lanes to densitometric profiles. Shannon–Wiener index (H) was computed with the equation H = −ΣPi (lnPi), where Pi is the ratio of individual band density to the sum of all band densities. Sorenson’s pairwise similarity coefficient (Cs) was calculated with the equation Cs = 2j/(Nx + Ny), where Nx is the number of bands for lane x, Ny is the number of bands for lane y, and j represents the number of shared bands for both lane x and y.
2.3. Statistical analysis
All tests were performed in triplicate. The significant differences of measurements were performed by using one-way analysis of variance followed by least significant difference tests and p < 0.05 were considered to be statistically significant.
3. Results and discussion
3.1. Characteristics of bleaching wastewater
The chemical characteristics of the bleaching wastewater used in this study are given in Table . Bleaching wastewater was, on average, acerbic with a pH value of 6.6 ± 0.1. According the FAQ guidelines,[Citation24] the acceptable pH interval for irrigation water is from 6.5 to 8.4. The pH value of the wastewater was within the acceptable limits.
Table 1. Characteristics of bleaching wastewater used for CRWs irrigation.
Regarding major nutrient elements, the average content of the ammonia nitrogen (NH4+ –N) was 16.01 ± 3.02 mg/L, a total phosphorous (TP) content of 0.56 ± 0.16 mg/L, and a chemical and biochemical oxygen demand of 2228.5 ± 325.6 and 324.6 ± 85.5 mg/L, respectively. The AOX was 12.77 ± 2.21 mg/L. Values for COD, BOD, NH4+ –N, TP and AOX were above the maximum allowable limits for effluent discharge into water bodies and land as stipulated by the World Health Organization and the Ministry of Environment Protection of the People’s Republic of China. In conclusion, the bleaching wastewater presents a potential pollution risk.
3.2. Effects of bleaching wastewater irrigation on soil physicochemical properties
Compared to the river water irrigated controls, soil pH value was not affected by the period of bleaching wastewater irrigation, as there were no differences registered (Table ). However, bleaching wastewater irrigation significantly increased (p < 0.05) soil Na+, TX and AOX contents, while the value of EC was also remarkably enhanced (p < 0.05) (Table ). It was reported in the literature that the increase of EC value for soil irrigated with wastewater as compared to soil irrigated with potable water is attributed to the original high level TDS of the wastewater.[Citation25] Clearly, the high content of salt in bleaching wastewater resulted in the rising of EC, which might induce the soil salinity.
Table 2. Soil chemical parameters of bleaching wastewater irrigated CRWs (CKs) and river water irrigated controls (STs).
The initial soil organic matter, TN and TP contents of the bleaching wastewater irrigated CRWs (STs) were similar to the soils from the river water irrigated controls (CKs) (Table ). After the exposure experiment, though the above element contents showed an increasing tendency, only the TN content increased significantly (p < 0.01) (Table ). It seems that most of the organic matters were degraded, and also the soil has a strong ability to degrade organic matters. The accumulation of TN in bleaching wastewater irrigated STs may have resulted from the input of nitrogen element, mineralization and cycles of nitrogen element in the soil.[Citation26] On the other hand, balance of carbon, nitrogen and phosphorus is very important to the nutrient management and agricultural sustainability.[Citation27,28] In this study, whereas the content of nitrogen increased significantly in the STs after the exposure experiment, the presence of bleaching wastewater did not significantly affect the ratio of soil C/N and N/P. Apparently, the input of bleaching wastewater did not remarkably change the balance of soil main nutrient elements.
3.3. Effects of bleaching wastewater irrigation on soil biological properties
It is well known that soil hydrolases play important roles in nutrient cycling. For example, cellulase, invertase and polyphenol oxidase are involved in the carbon cycling; urease is related to organic nitrogen cycling; and alkaline phosphatase is related to phosphorus metabolism. The effects of soil pollution on soil enzyme activities are complex. The response of different enzymes to the same pollutant may vary greatly.[Citation29] In the current study, bleaching wastewater showed heterogeneous effects on soil enzymes. The data in Figure showed that, compared to the control CKs, cellulase and invertase activities were significantly increased, but alkaline phosphatase and urease activities were remarkably decreased in bleaching wastewater irrigated STs. For example, the invertase activity was increased to almost 131% of the control, but the urease activity was decreased to 75% of the control. These suggested that the nutrient cycling was modified in the STs since these enzymatic activities have been stimulated or inhibited during the period of bleaching wastewater irrigation. To polyphenol oxidase activity, there were no differences have been detected after the bleaching wastewater exposure.
Figure 2. Soil enzymatic activities of cellulase (a), invertase (b), alkaline phosphatase (c), urease (d), and polyphenol oxidase (e) in the CRWs before and after the bleaching wastewater exposure experiment.
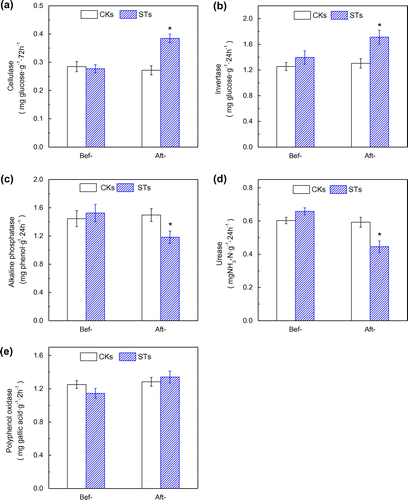
It was reported that the soil microbial community could be affected by pollution.[Citation21] In this study the variation of microbial community was measured by DGGE. From Figure (a) it can be seen that the initial Cs of bacterial community in the CKs was 2.367, and it was 2.372 in the STs. After exposure experiment, the H decreased to 1.749 and 1.541, respectively. It seems that the bleaching wastewater has some adverse influence on the bacterial diversity. The pairwise Cs between STs and CKs decreased from 73.8 to 58.7% (Figure (b)), which confirmed the variable effect of bleaching wastewater on bacterial community.
Figure 3. Sorenson’s pairwise similarity coefficient (Cs) of bacterial and fungal community (a), Shannon–Wiener index (H) of bacterial diversity (b) and fungal diversity (c) in the CRWs.
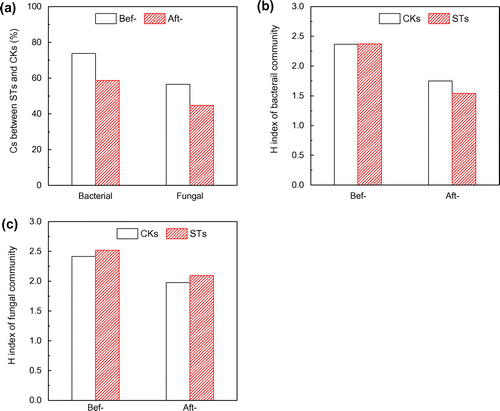
As shown in Figure (a), the initial H of STs and CKs were 2.520 and 2.414, which respectively decreased to 2.094 and 1.976 after the exposure experiment. However, there were no measurable differences observed between STs and CKs after the exposure experiment (p > 0.05). The bleaching wastewater irrigation has no significant effect on the fungal diversity. As for the Cs the index decreased from 56.5 to 44.7% (Figure (b)), which indicated that that bleaching wastewater changed the structure of fungal community.
4. Conclusion
Bleaching wastewater application has significantly increased soil Na+, TX and AOX contents, which induced the increasing of soil EC value. However, a growth season application has no significant influence on soil pH. Bleaching wastewater displayed significant variations of soil enzyme activities (except for polyphenol oxidase) and the structure of bacterial and fungal communities. However, the bleaching wastewater did not give intensive effect on the fungal Shannon–Wiener diversity, but remarkably decreased the bacteraial diversity. Thus, the application of bleaching wastewater disrupted the equilibrium of the CRWs.
Disclosure statement
No potential conflict of interest was reported by the authors.
Funding
This work was financially supported by the National Natural Science Foundation of China [grant number 21277115]; the Qinglan Program of Science and Technology Innovation Team of Jiangsu Province, China (2010).
References
- Fry LM, Mihelcic JR, Watkins DW. Water and nonwater-related challenges of achieving global sanitation coverage. Environ. Sci. Technol. 2008;42:4298–4304.10.1021/es7025856
- Jiang Y. China’s water scarcity. J. Environ. Manage. 2009;90:3185–3196.10.1016/j.jenvman.2009.04.016
- He H, Hu G, Sun C, et al. Trace analysis of persistent toxic substances in the main stream of Jiangsu section of the Yangtze River, China. Environ. Sci. Pollut. Res. 2011;18:638–648.10.1007/s11356-010-0414-z
- Asano T, Burton FL, Leverenz HL, et al. Water reuse: issues, technologies, and applications. Eng. Sanit. Ambient. 2008;13:247–248.
- Chen A, Liang H, Chen T, et al. Influence of long-term irrigation with treated papermaking wastewater on soil ecosystem of a full-scale managed reed wetland. J. Soils Sediments. 2016;16:1352–1359.10.1007/s11368-015-1161-z
- Paranychianakis NV, Angelakis AN, Leverenz H, et al. Treatment of wastewater with slow rate systems: a review of treatment processes and plant functions. Crit. Rev. Environ. Sci. Technol. 2006;36:187–259.10.1080/10643380500542756
- Vymazal J. The use constructed wetlands with horizontal sub-surface flow for various types of wastewater. Ecol. Eng. 2009;35:1–17.10.1016/j.ecoleng.2008.08.016
- Rosabal A, Morillo E, Undabeytia T, et al. Long-term impacts of wastewater irrigation on Cuban soils. Soil Sci. Soc. Am. J. 2007;71:1292–1298.10.2136/sssaj2006.0248
- Rutkowski T, Raschid-Sally L, Buechler S. Wastewater irrigation in the developing world – two case studies from the Kathmandu Valley in Nepal. Agric. Water Manage. 2007;88:83–91.10.1016/j.agwat.2006.08.012
- Christou A, Eliadou E, Michael C, et al. Assessment of long-term wastewater irrigation impacts on the soil geochemical properties and the bioaccumulation of heavy metals to the agricultural products. Environ. Monit. Assess. 2014;186:4857–4870.10.1007/s10661-014-3743-4
- Xia M, Liu Z, Lu Z. Soil remediation of degraded coastal saline wetlands by irrigation with paper mill effluent and plowing. J. For. Res. 2012;23:267–274.10.1007/s11676-012-0252-6
- Sridhar R, Sivakumar V, Immanuel VP, et al. Treatment of pulp and paper industry bleaching effluent by electrocoagulant process. J. Hazard. Mater. 2011;186:1495–1502.10.1016/j.jhazmat.2010.12.028
- IWMI. Recycling realities: managing health risks to make wastewater an asset. Water Policy Briefing 17. Colombo, Sri Lanka; 2006.
- WHO. Guidelines for the safe use of wastewater, excreta and grey water. Vol. 2, Wastewater use in agriculture. Geneva: World Health Organization; 2006.
- Datta SP, Rao AS, Ganeshamurthy AN. Effect of electrolytes coupled with variable stirring on soil pH. J. Ind. Soc. Soil Sci. 1997;45:185–187.
- Walkley A. A critical examination of a rapid method for determining organic carbon in soils – effect of variations in digestion conditions and of inorganic soil constituents. Soil Sci. 1947;63:251–264.10.1097/00010694-194704000-00001
- Page AL, Miller RH, Keeney DR. Methods of soil analysis. Part 2. Chemical and microbiological properties. 2nd ed. WI: ASA, SSSA, CSSA No. 9; 1982. p. 595–623.
- Montoroi JP. Electrical conductivity of soil solution and soil aqueous extracts. Case of a saline acid sulphate soil in lower casamance [field technique, saline soil] Etud. Gest. Sols. 1997;4:279–298.
- Oleksy-Frenzel J, Wischnack S, Jekel M. Application of ion-chromatography for the determination of the organic-group parameters AOCl, AOBr and AOI in water. Fresenius J. Anal. Chem. 2000;366:89–94.10.1007/s002160050016
- Guan SY. Soil enzyme and its research method. Beijing: China Agriculture Press; 1986.
- Chen A, Xiao B, Liang H, et al. Soil microbial community response to nitrobenzene exposure for a Spartina wetland. Soil Sediment Contam. Int. 2013;22:74–184.
- Muyzer G, Waal EC, Uitterlinden AG. Profiling of complex microbial populations by denaturing gradient gel electrophoresis analysis of polymerase chain reaction-amplified genes coding for 16S rRNA. App. Environ. Microbiol. 1993;59:695–700.
- Haruta S, Ueno S, Egawa I, et al. Succession of bacterial and fungal communities during a traditional pot fermentation of rice vinegar assessed by PCR-mediated denaturing gradient gel electrophoresis. Int. J. Food Microbiol. 2006;109:79–87.10.1016/j.ijfoodmicro.2006.01.015
- Pescod MB, Arar A. Treatment and use of sewage effluent for irrigation. London: Butterworths; 1998.
- Mohamed MJ, Mazahreh N. Changes in soil fertility parameters in response to irrigation of forage crops with secondary treated wastewater. Soil Sci. Plant Nutr. 2003;34:1281–1294.10.1081/CSS-120020444
- Yan J, Pan G. Effects of wastewater irrigation on soil enzyme activities and respiration from a managed wetland. Soil Sediment Contam. Int. 2010;19:204–216.
- Whitmore AP, Goulding KWT, Glendining MJ, et al. Nutrient management in support of environmental and agricultural sustainability. Sustainability. 2012;4:2513–2524.10.3390/su4102513
- Schlüter U, Colmsee C, Scholz U, et al. Adaptation of maize source leaf metabolism to stress related disturbances in carbon, nitrogen and phosphorus balance. BMC Genomics. 2013;14:3306–3310.
- He ZL, Yang XE, Baligar VC, et al. Microbiological and biochemical indexing systems for assessing acid soil quality. Adv. Agron. 2003;78:89–138.10.1016/S0065-2113(02)78003-6