Abstract
The mobility and bioavailability of lead (Pb) in seven military shooting range soils found in eastern and north eastern Botswana were studied using sequential extraction procedure. The different forms of Pb and their reactivity in the soil help explain their speciation, mobility and bioavailability in the environment. Mobility of Pb in the berm soils in all the seven shooting ranges was found to be over 90% implying high Pb lability. The bioavailability index of Pb was in the range 60–90%, an indication that most of the Pb can be available for plant uptake. Sequential extraction studies indicate that the partitioning of Pb was mostly confined to the carbonate compartment in all the shooting ranges. All the seven shooting ranges failed the Synthetic Precipitation Leaching Procedure (SPLP) with SPLP Pb concentrations exceeding United States Environmental Protection Agency (USEPA) 0.015 mg/kg critical level of hazardous waste, posing a pollution threat to surface and groundwater.
1. Introduction
Shooting ranges have been found to be the second largest anthropogenic contributor to environmental pollution from lead (Pb) deposition after the battery manufacturing industry [Citation1]. Owing to its numerous exceptional properties, Pb is still being used today in a wide range of applications; thereby increasing its concentration in our environment overtime [Citation2]. Current uses of lead include cable sheathing, pigments, chemicals, storage batteries, petrol, alloys and ammunition worldwide [Citation3,4]. All these Pb producing industries and activities such as Pb smelting plants, battery manufacturing plants, firing ranges and construction sites lead to exposure of humans and the environment at large to high amounts of Pb. In an attempt to reduce these detrimental effects of lead, some of its uses in gasoline, paint, solder and many other lead-containing products have been banned [Citation5].
This exposure to Pb poses a serious health risk and general wellbeing of people, animals and the environment. The danger to human beings, animals and plants posed by exposure to the bioavailable Pb is well documented [Citation6]. Elevated levels of Pb in adults have resulted in reproductive dysfunction in men and women [Citation7]. Low concentrations of 10 μg/dl have been found to cause brain and nervous system damage, slowed growth, headaches, hearing problems and impairment of vision in children [Citation8]. Other harmful health effects have been observed in human beings such as high blood pressure, digestive discomfort, neurological disorders and memory loss [Citation7]. The mobility and bioavailability of Pb in soils is dependent on many physical, chemical and biochemical factors such as physical weathering, oxidation-reduction, association-dissociation, precipitation-dissolution and sorption-desorption [Citation9]. Most studies have dealt with total concentration of Pb in soil which does not provide a good measure for the mobility and bioavailability of Pb failing to give a true picture of the potential environmental, vegetative and human health hazards. To better understand the risk posed by elevated levels of Pb in the environment, it is important to understand the different chemical species in which Pb exist in the shooting range soils (free metal, metal-organic complexes and salts). The different forms of heavy metals and their reactivity with the specific target constituents of the soils help ascertain for their solubility, mobility, bioavailability, fate and ecotoxicological importance in the environment [Citation10,11].
The method of sequential extraction was first coined by Tessier et al. [Citation12] and has been widely applied in trying to ascertain for the solubility, mobility and bioavailability of heavy metals in the environment [Citation12]. This method has been used frequently in the determination of chemical speciation of heavy metals in soils, water and sediments [Citation13,14]. The same method has also been applied to assessing the distribution of Pb in shooting range soils arising from Pb shots and bullets [Citation15–17]. The sequential extraction procedure describes the existence of heavy metals predominately in five different chemical species in the soil [Citation12]. The successive extraction procedures account for four well defined compartments and a residual. These include heavy metals as components of the water soluble and exchangeable (WE), organic-bound (OM), carbonate-bound (CB), Fe–Mn oxides bound (FM) and residual fractions (RS). The binding of heavy metals to the water-soluble and exchangeable fraction is considered weak as compared to other fractions with the binding more strongly in the residual fraction. Therefore, in terms of mobility, the heavy metals in the WE fraction are considered mobile while the fractions in the OM, CB, and FM fractions are considered mobilisable. The RS fraction on the other hand is referred to as the immobile fraction. The Fe–Mn oxides bound and organic-bound compartments are also referred to as the reducible and oxidizable fractions respectively [Citation18]. The residual fraction refers to the soil component with heavy metals embedded in the crystal lattice of minerals (detrital) [Citation19]. Under normal environmental conditions, heavy metals present in the RS fraction have restricted mobility and are unreachable by living organisms. It is also important to note the assumption that the bioavailability of heavy metals diminishes with each successive fractionation step [Citation19]. The bioavailability and non-bioavailability of Pb can be further divided into two types of soil fractions through which heavy metals in the shooting range soils can be confined to; (1) the inert fraction and (2) the labile fraction [Citation20]. The inert fraction is considered chemically inactive form of heavy metal compounds and is believed to be non-toxic whilst the labile fraction is considered hazardous and toxic. For example, shooting range soils in which Pb exists in residual fractions such as lead phosphate (Pb3(PO4)2, lead sulphate (PbSO4), lead sulphite (PbS) and lead chloride phosphate (Pb5(PO4)3Cl) make lead unreachable and therefore less potential risk to the biota. On the other hand the chemically active fraction of Pb-particulate matter such as lead nitrate (PbNO3), lead acetate (PbCOOCH3) and lead chloride (PbCl2) has the potential to be translocated into plants and contaminating both surface and underground water sources leading to their presence in the food chain. As a result the mobile toxic Pb metal can end up in plants, animals and human beings systems [Citation21,22]. The bioavailability of the chemical species in the active fraction is dependent on the soil physical and chemical properties such as pH, cation exchange capacity (CEC) and organic matter content [Citation17]. When Pb shots and bullets are deposited into shooting range soils, Pb would undergo series of chemical transformations. Some possible Pb chemical transformations in the soil include the following [Citation23]:
A preliminary investigation into assessment of extent of pollution from total Pb concentration in shooting range soils in the seven shooting ranges is reported in this study. To further understand the migration, partitioning and speciation of Pb in shooting range soils, fractionation studies were carried out. There were two main objectives of this study: (i) assessment of chemical partitioning and speciation of Pb in shooting range soil compartments in seven shooting ranges in the eastern and north eastern Botswana in order to determine the potential risk posed to the grazing fields, arable fields and communities nearby the shooting ranges; and (ii) determination of mobility and bioavailability of Pb in soil components by calculating the mobility and bioavailability factors in order to evaluate the potential risk of Pb from the soil fractions to be absorbed by plants.
2. Materials and methods
2.1. Features and descriptions of study sites
Seven shooting ranges located in the eastern and north eastern part of Botswana were used in this study (Figure 1(a) and (b)). The seven shooting ranges were; Selibe Phikwe pistol range (S/P Pistol), Selibe Phikwe range 1 (S/P R1) and Selibe Phikwe Range 2 (S/P R2) located in Selibe Phikwe, Matsiloje Range 1 (MAT R1) and Matsiloje Range 2 (MAT R2) located near Matsiloje village while Lebolobolo (LEBO) and Tshukudu (TSHU) ranges are both located in the Pandamatenga area. The S/P Pistol, S/P R1 and S/P R2 (GPS Coordinates: 21° 58′ 19.79′′ South, 27° 50′ 14.39′′ East, 21° 57′ 58.55′′ South, 27° 52′ 32.97′′ East and 21° 57′ 44.80′′ South, 27° 52′ 35.44′′ East respectively) sampling areas are situated near the town of Selibe Phikwe, The MAT R1 and MAT R2 with GPS coordinates: 21° 22′ 12.00′′South, 27° 52′ 12.00′′ East and 21° 22′ 12.25′′South, 27° 55′ 6.73′′ East respectively are found in the Matsiloje area. On the other hand the LEBO and TSHU shooting ranges at GPS coordinates 18° 31′ 57.01′′South, 25° 39′ 14.22′′East and 18° 31′ 27.72′′South, 25° 40′ 7.37′′East respectively are located in the Pandamatenga area. These are military shooting ranges used for shooting practices using military rifles of 0.50′′, 5.56 and 7.62 mm calibres. Background soil was collected at a distance of 200 m from the seven shooting ranges which served as a control sample.
All the seven shooting ranges studied have been in operation for over fifteen years as shown in Table . The soils in the shooting ranges investigated are mostly luvisols and vertisols (Table ). The haplic luvisols have a shallow topsoil cover with subsoil materials being mainly weathered and decomposed granites. The soils found in this area have been found to be less suitable for arable agriculture. The pellic vertisols on the other hand are fertile black cotton clay rich soils and these soils are mostly used for arable agriculture in the studied region [Citation24]. The chromic luvisols are moderately deep to very deep and slightly excessively drained, strong brown to dark red, sandy loams to clay loams. The soil type strongly affects the soil CEC. Sandy soils in comparison to clay soils have been found to have low CEC [Citation25].
Table 1. Shooting ranges years of establishment and the corresponding soil types found at the seven shooting ranges located in the eastern and northern Botswana.
2.2. Soil sampling
A soil recovery probe was used to collect 48 soil samples to a depth of 20 cm from the earth surface in the seven shooting ranges. Three sampling points were marked at the berm, target line, 50 m from target line and 100 m from target line (Figure ). The 50 and 100 m firing lines were chosen because they are positions from which weapons are frequently fired. Four soil samples were collected from each of these three circular sampling points (radius 150 cm) along a central transect as shown in Figure below. The soil samples were stored in butyrated zip-lock plastic bags and then transported to the Botswana Geoscience Institute (BGI) chemistry laboratory for analysis. Control soil samples were collected near shooting ranges at a distance of 200 m.
2.3. Laboratory soil sample preparation for analysis
Composite soil samples were made by mixing quadruplicate samples collected from the three sampling points at the berm, target line, 50 and 100 m position lines. After air drying the soil samples sieving was achieved using a 2 mm stainless steel sieve to remove spent bullets, rock pebbles and small tree barks. The soil samples were then divided into two portions. One portion was pulverised using a manually operated Retsch RS 200 Vibratory Disk Mill at 700 rpm for 3 min. The dry heating-block digestion procedure (United States Environmental Protection Agency [USEPA] Method 3050) was used to digest a series of 0.100 g of soil samples in 25 mL of 10% HNO3 for 16 h.
2.4. Physical and chemical analysis of soil samples
The soil pH, organic matter (OM), CEC and total Pb concentration were all evaluated using standard methods. A 20 g of the homogenised soil sample was added to 40 mL of deionized water and 0.01 M CaCl2 solution and a calibrated crimson basic 20 pH meter was used for pH measurements at 25 °C. The organic matter (OM) content was measured using the Walkley-Black procedure [Citation26]. Method 9081 was used to ascertain for the soil CEC.
2.5. The leaching test
The SPLP extraction technique is widely applied to evaluate the possible underground water contamination [Citation27,28]. The extraction fluid is used to simulate acid rain and this involves the use of acidified de-ionised water using a mixture of 60/40 H2SO4/HNO3 to achieve the pH of 4.2 ± 0.05. The SPLP Pb in the soils was extracted in the ratio of soil:solution of 1:20 and determined using the USEPA method 1312 [Citation29].
2.6. Fractionation studies
In this study, the mobility, availability and fate of Pb in the soil were evaluated using five sequential extraction processes adapted from Tessier et al. [Citation12]. The procedure was used to assess the different components of the berm soil Pb in the forms; water-soluble and exchangeable (WE); carbonate bound (CB); Fe–Mn oxides (FM); organic matter bound (OM) and the residual (RS) fractions [Citation12]. The accuracy of the sequential procedure was assessed using total metal Pb analysis.
2.7. Pb mobility and bioavailability potential
The absolute and relative content of fractions weakly bound to components may be used to evaluate the mobility and fate of Pb in shooting range soils. A method by Kabala and Singh [Citation30] for the calculation of mobility factor was used to ascertain for the relative index of metal mobility and fate [Citation30]. The mobility factor was calculated based on the following expression;
where, FWE = water soluble fraction, FCB = carbonate-bound fraction, FFM = Fe–Mn oxides fraction, FOM = organic-bound fraction, FRS = residual fraction.
The strength of binding of Pb to different soil components determines its bioavailability and the risk associated with its presence in the ecosystem. The risk assessment was carried out through calculating the bioavailability factor (BF). The bioavailability factor represents the potential of Pb from the shooting range soil matrix to enter the soil solution from which it can be adsorbed and accumulated in plants. The bioavailability factor is calculated as the ratio of the available Pb in a fraction to its total concentration as given in the following expression,
2.8. Total Pb concentration in shooting range soils
Total Pb concentration was analysed using inductively coupled plasma optical emission spectrometry (ICP-OES; Perkin Elmer, Optima7300DV) after digestion using USEPA Method 3050 with 1:1 nitric acid. Standard reference material of soil, NCS DC 73320 was obtained from the Botswana Geoscience Institute (BGI Chemistry Lab) and used for quality assurance after taking it through the same sample preparation procedure as the shooting range soil samples.
3. Result and discussion
3.1. Total Pb concentration of shooting range soils
High concentrations of total Pb contaminant were found in all the seven shooting ranges studied (Figure (a)–(d)). Both the berm and target line soils accumulated the highest concentrations of Pb with a decrease in subsurface Pb concentration moving away from the berm towards the firing lines (50 and 100 m). The largest densities of the spent bullets and shots were found in the berm soils, accounting for the highest concentrations of total Pb in the berm soils. A direct relationship between the high concentration of Pb and the accumulation of spent bullets and shots in the berm soils has been reported [Citation31]. Concentrations of total Pb of up to 21000 mg/kg were found in the shooting range soils. The highest total Pb concentrations of 20882 ± 5419 mg/kg were recorded in MAT R2 (established in 1985) shooting range. The lowest total Pb concentrations of 685 ± 219 mg/kg were measured at the S/P Pistol range. This concentration of total Pb at the S/P Pistol is still high relative to the USEPA set critical level of 400 mg/kg of Pb is soils [Citation23]. The low concentrations of total Pb in the S/P Pistol range can be attributed to the fact that pistol ammunition contains small content of Pb slug as compared to the rifles used in the other six shooting ranges. According to Bricka [Citation32] pistol ammunition contains less amount of Pb slug of about 52% as compared to assault rifles with over 80% Pb slug content [Citation32]. In addition pistol shooting practices are only carried out by military officers (officer cadets) who are few in numbers and thereby less accumulation of Pb shots in pistol shooting range soils. There are many reasons contributing to the elevated levels of Pb at MAT R2 shooting range. This shooting range has been in operation since 1985. This implies that Pb bullets and shots have been accumulating in this shooting range for more than 20 years. The TSHU shooting range is the oldest of all the shooting ranges studied, established in 1984. It accumulated total Pb concentrations of 14731 ± 7353 mg/kg. However, total Pb concentration at TSHU shooting range was less than that at MAT R2. There are several reasons for this difference, according to military personnel; intense shooting practices take place at MAT R2 than any other range studied. The MAT R2 is used mostly by the infantry units who are the foot soldiers and backbone of the Botswana Defence Force (BDF). These military personnel carry out their shooting practices frequently at this shooting range. The total Pb concentrations of other shooting ranges were in the in following decreasing order 13449 ± 2375, 10386 ± 2248, 6413 ± 253 and 2741 ± 1291 at MAT R1, S/P R2, LEBO and S/P R1 respectively. As expected the berm soils accumulated the highest concentrations of Pb as compared to soils found at the target line, 50 and 100 m away from the target line on the shooting range bed. The target line being closer to the berm accumulated higher concentrations of Pb than at 50 and 100 m firing lines (Figure (b)). The target line total Pb concentrations were highest for the S/P R2 (3169 ± 479 mg/kg) followed by S/P R1 (3169 ± 479 mg/kg) and the lowest at the S/P Pistol range as expected (180 ± 13 mg/kg). It was found out that the two ranges S/P R1 and S/P R2 are located at the foot of a hill with the hill acting as the berm. It was assumed this greatly increased the mobility of Pb due to rain runoff water travelling down the hill. Another important point to note is that the target line total Pb concentration at S/P R1 (2741 ± 1291 mg/kg) was higher than the berm concentration, an indication that most of Pb on the berm soils had migrated down to the foot of the berm. In addition to runoff water down the berm, the target line Pb concentrations are higher than at 50 and 100 m firing lines because of the physical abrasion of Pb bullet from friction between the bullet and target as the bullet pierced through the target. Most of the shooting ranges showed contamination less than the USEPA critical level of 400 mg/kg at the 50 and 100 m firing lines. This implies that most of the Pb pollution is restricted to the berm soils and the target line soils. However it should be noted that the total Pb concentrations at the 50 and 100 m firing lines are still higher than the USEPA maximum contaminant limit (MCL) of 15 μg/L in water and the Botswana and World Health Organisation MCL limit of 10 μg/L. This may still pose a contamination threat to both surface and underground water. Background soils were taken at a distance of 200 m from the shooting ranges. In all the seven shooting ranges studied, the background soil concentrations were in the range between 2.5 and 17 mg/kg (Figure ). The background soils showed Pb concentrations in the range of the earth’s crust of 15–20 mg/kg [Citation33]. This suggested that pollution of the soil from Pb was mainly confined within the shooting ranges, especially the berm. A certified reference material, NCS DC 73320, of known concentration of 20 mg/kg was used for quality control checks with a percent recovery of 89.7%. The accuracy of measurements was <3% RSD, an indication of accuracy of the analytical methodology. As shown below, Figure describes total Pb concentration in shooting range soils but does not give information on the partitioning of Pb within the different soil components and the various species of Pb that exist in the soil. The sequential extraction procedure would give such vital information.
Figure 3. Total Pb concentrations of composite soils taken from; (a) the berm; (b) target line; (c) 50 m from target line and (d) 100 m from target line at seven different shooting ranges found in the eastern and northern Botswana, Mean of n = 3; Standard error of the mean, δ = δ/√n, where δ = standard deviation.
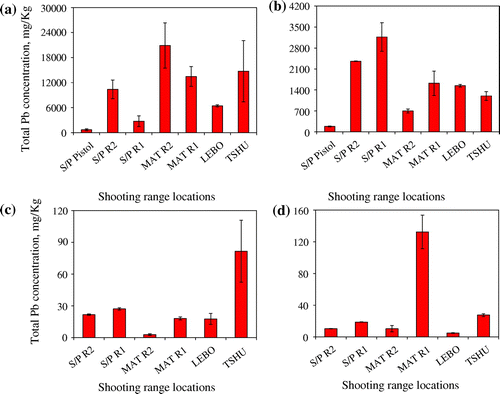
3.2. Sequential extraction and speciation of Pb in shooting range soils
The use of total Pb concentration as a criterion to assess its potential effects to the shooting range soils may imply that all its forms have equal impact to the environment. The retaining, chemical mobility, leaching and distribution of Pb in the different soil compartments were assessed using the sequential extraction procedure [Citation12]. As a result the sequential extraction procedure helps evaluate the speciation of Pb in every fraction. The use of sequential extraction gives detailed information about the origin, biological and physicochemical availability, mobilisation and transport of Pb in shooting range soils [Citation12]. The partitioning of Pb in the berm soils was studied in five different fractions being the water soluble and exchangeable (WE), organic-bound (OM), carbonate-bound (CB), Fe–Mn oxides bound (FM) and residual fractions (RS). It is important to note that the partitioning of Pb in the different soil fractions is largely dependent on the chemical and physical characteristics of the soils such as the soil pH, organic matter and CEC. In all the seven shooting ranges studied, Pb existed mostly in the carbonate fraction (CB), 36–53% (Figure ) followed by OB (2–41%)>FM (0–28%)>WE (1–27%)>RS (0–3%). The elevated levels of carbonate bound Pb in the berm soils was due mainly to the oxidation of metallic Pb bullets and shots and the conversion of the oxidised Pb (PbO) to Pb carbonates such as cerussites and hydrocerussites [Citation23]. The high prevalence of the carbonate bound fraction can be well explained in the following reactions;(1)
Figure 5. Sequential extraction of Pb into different chemical fractions found in soils from shooting ranges located in the eastern and nothern Botswana.
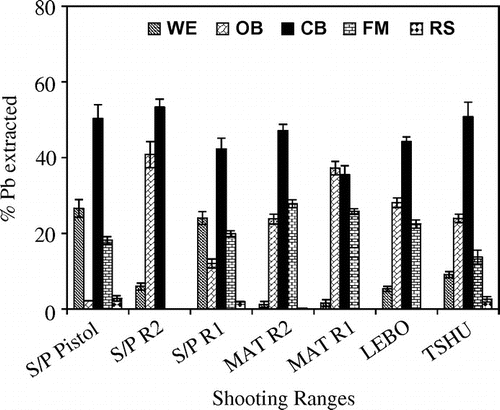
(2)
(3)
The pH of the soil plays an important role in the stability of the Pb fractions. At high alkaline pH the Pb oxides and carbonates become more stable and inert to chemical reactions and dissolutions. The shooting ranges that experienced the highest carbonate-bound Pb such as TSHU, S/P R2, MAT R2 and MAT R1 possessed soil pH in the range 7.55–8.69 (Table ). Our findings of the pH dependent existence of the carbonates have also been described elsewhere [Citation34]. It was found out that the Pb carbonates (cerussites) and Pb hydroxyl carbonates (hydrocerussites) were the most prevalent species at high pH in the shooting ranges studied. Other factors such as elevated levels of organic matter in the soil have been found to increase the weathering and transformation of Pb bullets and shots in the soil [Citation35]. Ma et al. [Citation17] were able to find that the removal of soil organic matter prevented the weathering and transformation of Pb shots and bullets into Pb-oxides and Pb-carbonates [Citation17]. The microbial organisms in soil rich in organic matter produce CO2 and make it available for the transformation of lead bullets and shots into Pb oxides and carbonates [Citation35]. The humic and fulvic acids present in the organic matter can bind with Pb on the surface of the Pb shots and bullets and thereby accelerating the weathering of the Pb shots and bullets by exposing new surfaces to weathering processes [Citation36]. All the seven shooting ranges studied contained organic matter in the range 0.31–2.13% OM. The levels of organic matter in the shooting range berm soils corresponded well with the partitioning of Pb in OM fraction. The amount of organic matter present at MAT R2 was 2.13% OM and this shooting range contained the highest organic matter than any other range in this study. Typical for such high content of organic matter, the MAT R2 accumulated the highest total Pb concentrations in the berm soils (Figure ). This implies that most of the Pb shots and bullets that accumulated into the MAT R2 berm soil weathered away and the metallic Pb in the bullets was transformed into more reactive Pb complexes in the soil thereby increasing the mobility and bioavailability of Pb in the soil. The application of the sequential extraction leaching procedure by Ashraf et al. (Citation37) was able to give critical information on the distribution of particulate metals such as Pb, Sb, Zn, Cr and As in the aquatic environment of an ex-mining catchment [Citation37]. They found that Pb was mostly restricted to the labile organic fraction and thereby increasing its bioavailability and uptake by plants. The soil CEC was also found to be an important factor in the weathering, transformation, mobility and bioavailability of Pb. The CEC of the soil determines the binding capacity of such soil. CEC is a measure of the amount of exchangeable cations that can be retained by a given mass of soil [Citation25]. Soils with higher CEC experience enhanced binding capacity of Pb leading to reduced Pb mobility and bioavailability [Citation23]. In addition to other factors such as pH and soil organic matter, MAT R2 berm soil had the second highest CEC of 21.87cmol/kg. The high CEC at MAT R2 implies that the soils at this range are able to retain high concentration of Pb. Cao et al. [Citation23] found total Pb concentration in berm soils of MPR shooting range as high as 48,400 mg/kg with the corresponding high CEC of 43.2 cmol/kg compared to the total Pb concentration of 12 710 mg/kg with corresponding CEC of only 8.51 cmol/kg at TRR shooting range [Citation23]. The type of soil affects the CECof the soil. Vertisol and luvisol soils normally have CEC values of ≥ 35 and ≥ 24 cmol/kg respectively (Table ). The soil CEC, pH and organic matter as mentioned earlier affect the mobility and bioavailability of Pb in the soil. It was found crucial therefore, to find a measure for these two factors through the determination of the mobility and bioavailability factors.
Table 2. Chemical properties of soils found in seven shooting ranges located in the eastern and northern Botswana.
3.3. Pb mobility and bioavailability
The mobility of Pb in shooting range soils describes its potential to become mobile and thereby posing a danger to the ecosystem. All the seven shooting ranges studied experienced the highest Pb mobility of over 90% (Figure ). The high Pb mobility signals the high Pb lability in the shooting range soils. The high mobility of Pb in the shooting ranges studied corroborates our sequential extraction findings in which most of the Pb was restricted to the carbonate fraction which can easily dissolve under favourable environmental conditions. Other factors such as pH, organic matter content and CEC affect the mobility of Pb. Soils high in organic matter have been found to greatly increase the mobility of Pb in the soil [Citation17]. The organic matter transforms Pb bullets and shots into organo-Pb complexes that become soluble under favourable conditions such as high pH [Citation38]. All the seven shooting ranges experienced pH values in the alkaline region. At high pH the solubilisation of soil organic matter becomes enhanced leading to the release of and mobilisation of Pb in the soil. The dissolution of Pb-organo complexes makes Pb more bioavailable. Studies have shown that the mobility of Pb can be greatly reduced in the absence of organic matter due to the impediment of transformation of metallic Pb into Pb-oxides and Pb-carbonates [Citation17]. Some of the shooting ranges such as S/P Pistol, S/P R2, S/P R1 and TSHU experienced low CEC (Table ). Accompanied by other favourable soil conditions such as high pH, low CEC increases the mobility of Pb in the soil as the binding of Pb to soil is greatly reduced (Figure ). Other factors such as soil pH and organic matter affect the CEC of the soil. Alkaline soils favour the exchange of Pb with other species such as H+ cations leading to the mobilisation of Pb in solution [Citation17]. All the seven shooting ranges experienced pH values in the alkaline region, a favourable condition for Pb mobilisation. It has been found that soils with high content of other metals present unfavourable conditions for Pb mobility [Citation39]. The toxicity and bioavailability of Pb in the soil is highly dependent on the chemical species that Pb exists in. The bioavailability index of Pb in the shooting range soils was evaluated. The bioavailability index was calculated in the range 35–87% for all the seven shooting ranges studied. More than 50% of Pb was found to be bioavailable in three of the seven shooting ranges. Pb in LEBO shooting range had the greatest chance of being accumulated in plants with bioavailability index of 87% and MAT R2 Pb was the least bioavailable at 35%. Aikpokpodion et al. [Citation40] were able to determine that the uptake of heavy metals by cocoa plants was greatly affected by the chemical speciation of the heavy metals in the soil [Citation40]. The concentration of Pb accumulated in the mollusks plant species was found to be higher than the concentrations specified by the Malaysian Food Act 1983 [Citation41]. The bioavailability of Pb to plants has also been found to have a negative impact on the morphology and growth of plants such as the tabacum [Citation42]. The accumulation of Pb in plants has been found to increase the production of reactive oxygen species such as oxo ions (O2−), hydroxides (OH−) and peroxides (H2O2) that are highly reactive anions and molecules leading to the disturbance of normal cellular metabolism. These chemical species have the potential of damaging plant proteins and nucleic acids through redox reactions [Citation42]. Immobilisation of Pb in the soil through addition of phosphate has been found to greatly reduce the mobility, bioavailability and bioaccessibility of Pb to plants. Sanderson et al. [Citation43] have shown that the bioavailability of Pb to plants in phosphate stabilized soils can be reduced by 20–40% through the conversion of Pb into the highly insoluble pyromorphite complex (Pb5(PO4)3Cl, Ksp = 10−25.05) [Citation43]. Soil amendments using mussel shells, cow bones and biochar have also seen decrease in exchangeable Pb in shooting range soil by 99% [Citation44]. The high mobility and bioavailability of Pb in the shooting range soils increase the possibility of surface and underground water contamination. An experiment was performed that evaluated the potential risk for underground water pollution. The synthetic precipitate leaching procedure (SPLP) was carried out.
3.4. Synthetic precipitation leaching procedure
The SPLP is used to simulate the leaching of heavy metals into the soils due to acid rain [Citation27]. The USEPA stipulates that any SPLP Pb concentration of higher than 0.015 mg/kg is indicative of possible contamination of underground water [Citation29]. All the seven shooting ranges studied failed the SPLP test. The TSHU shooting range experienced the highest SPLP Pb concentration of 267 ± 2.8 mg/kg and MAT R2 contained the lowest SPLP Pb concentration of 0.89 ± 0.32 mg/kg (Figure ). This implies that some of the Pb might have leached into the soil giving rise to possibility of underground water contamination. The leaching of Pb in shooting range soils can be reduced through application of Pb immobilisation techniques such as the use of Soybean stover-derived biochar [Citation45]. Biochar was able to reduce leaching of Pb by over 90% in soils treated with Soybean stover-derived biochar due to the formation of insoluble complexes such as chloropyromorphite and Pb-phosphate. Other procedures such as application of protonated teleosts biomass for removal of Pb from polluted environments have also been employed in trying to control Pb pollution [Citation46].
Figure 7. Synthetic precipitation leaching procedure (SPLP) Pb concentrations at seven different shooting ranges found in the eastern and northern Botswana (SPLP experiments simulate the effect of acid rain on chemical Pb transformation).
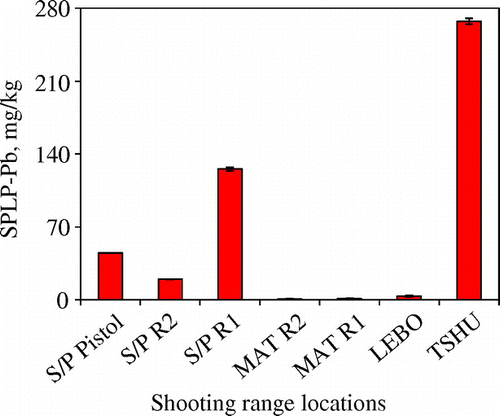
4. Conclusion
The fate and migration of Pb in the soil is dependent on the physical and chemical transformations Pb has to undergo in the soil. A detailed investigation on the various species of Pb that exist in shooting range soils help understand better the degree of contamination posed by Pb to plants, water and soil and the health hazards associated with such pollution. Instead of total Pb concentration determination in soil, Pb associated with the different soil compartments help ascertain for the mobility and bioavailability of Pb in the ecosystem. Sequential extraction procedure has been extensively applied to evaluating the association of heavy metals such as Pb with the various soil compartments giving rise to the labile and inert fractions. The fractionation procedure showed that most of the Pb in all the seven shooting ranges studied was confined to the carbonate fraction (CB). Some significant amount of Pb was also found to exist in the organic matter fraction. Both the carbonate bound and organic matter bound fractions belong to the labile fraction of the soil which is considered highly mobile and bioavailable. Plants growing near the shooting ranges are at risk from Pb poisoning and eventual death due to uptake of Pb by these plants as shown by the high bioavailability index (up to 87%). The mobility factors were well over 90% in all the shooting ranges studied. It is clear that the fate and toxicity of Pb is highly dependent on the particular chemical form in which it occurs in the ecosystem. Total Pb pollution in all the seven shooting ranges studied far exceeded the USEPA critical level of 400 mg/kg. Total Pb concentrations in the berm soils were in the range 600–20,000 mg/kg. MAT R2 experienced the highest pollution compared to other shooting ranges due to favourable environmental conditions such pH, organic matter and CEC. The elevated Pb pollution in these shooting ranges pose adverse health effects to human beings living in close proximity of the shooting ranges. Both surface and underground water run the risk of being contaminated since all the seven shooting ranges studied failed the SPLP. The SPLP Pb concentrations in all the seven shooting ranges exceeded the USEPA critical level of 0.015 mg/kg. To remedy soil pollution in shooting ranges, best shooting range management practices should be enforced. Remedial measures such as soil cleaning and immobilisation of Pb to reduce its solubility, mobility and bioavailability have been found to greatly reduce the impact from Pb in shooting range soils. A more detailed study involving investigation of Pb pollution in vegetation and water sources near shooting ranges need to be carried out to further assess the risk posed to the biota.
Funding
This work was supported by the Botswana International University of Science and Technology (BIUST), Research Initiation [grant number BIUST/ds/R&I/26/2016].
Disclosure statement
No conflict of interest was reported by the authors.
Acknowledgments
The authors would like to thank Botswana Defence Force (BDF) for availing their shooting ranges to be used in this study. We would also like to extend an appreciation to the Botswana Geoscience Institute (BGI) for their assistance with ICP-OES instrument for Pb analysis.
References
- Li Y, Zhu Y, Zhao S, Liu X. The weathering and transformation process of lead in China’s shooting ranges. Environ Sci: Processes Impacts. 2015;17:1620–1633.10.1039/C5EM00022J
- Scheuhammer AM. Historical perspective on the hazards of environmental lead from ammunition and fishing weights in Canada. In: Watson RT, Fuller M, Pokras M, Hunt WG, editors. Ingestion of leadfrom spent ammunition: implications for wildlife and humans. Boise, Idaho, USA: The PeregrineFund. DOI: 10.4080/iIsa2009.0105;2009. p. 61–67.
- Mao JS, Cao J, Graedel TE. Losses to the environment from the multilevel cycle of anthropogenic lead. Environ Pollut. 2009;157:2670–2677.10.1016/j.envpol.2009.05.003
- Rattner BA, Franson JC, Sheffield SR. Sources and implications of lead ammunition and fishing tackle on natural resources. Wildlife society technical review. Rev Lit Arts Am. 2008;6:1–62.
- US Environmental Protection Agency (USEPA). Region II, Best management practices for lead at outdoor shooting ranges. New York, RCRA Compliance Branch, 290 Broadway, 22nd Fl.;2005. ( EPA-902-B-01-001).
- Apostoli P, Cornelis R, Duffus J, et al. World Health Organisation (WHO). Elemental speciation in human health risk assessment. Geneva: Environmental Health Criteria 234; 2006. p. 1–256.
- Camobreco VJ, Richards BK, Steenhuis TS, et al. Movement of heavy metals through undisturbed and homogenized soil columns. Soil Sci. 1996;161:740–750.10.1097/00010694-199611000-00003
- Bruell R, Nikolaidis NP, Long RP. Evaluation of remedial alternatives of lead from shooting range soil. Environ Eng Sci. 1999;16:403–414.10.1089/ees.1999.16.403
- Violante A, Cozzolino V, Perelomov L, et al. Mobility and bioavailability of heavy metals and metalloids in soil environments. J Soil Sci Plant Nutr. 2010;10:268–292.
- Krishnamurti GSR, Naidu R. Speciation and phytoavailability of cadmium in selected surface soils of South Australia. Aust J Soil Res. 2000;38:991–1004.10.1071/SR99129
- Mana SC, Fatt NT, Ashraf MA. The fate and transport of arsenic species in the aquatic ecosystem: a case study on Bestari Jaya, Peninsular Malaysia. Environ Sci Pollut Res. 2016;23:1–9.
- Tessier A, Campbell PGC, Bisson M. Sequential extraction procedure for the speciation of particulate trace metals. Anal Chem. 1979;51:844–851.10.1021/ac50043a017
- Ashraf MA, Maah MJ, Yusoff I. Speciation of heavy metals in the sediments of former tin mining catchment. Iran J Sci Technol. 2012;36:163–180.
- Ashraf MA, Maah MJ, Yusoff I, et al. Speciation of heavy metals in the surface waters of a former tin mining catchment. Chem Speciation Bioavailability. 2012;24:1–12.10.3184/095422912X13259575370081
- Van Vleek B, Amarasiriwardena D, Xing B. Investigation of distribution of soil antimony using sequential extraction and antimony complexed to soil-derived humic acids molar mass fractions extracted from various depths in a shooting range soil. Microchem J. 2011;97:68–73.10.1016/j.microc.2010.05.015
- Chrastny V, Komarek M, Hajek T. Lead contamination of an agricultural soil in the vicinity of a shooting range. Environ Monit Assess. 2010;162(1-4):37–46.10.1007/s10661-009-0774-3
- Ma LQ, Hardison D Jr., Harris WG, et al. Effects of soil property and soil amendment on weathering of abraded metallic Pb in shooting ranges. Water Air Soil Pollut. 2007;178:297–307.10.1007/s11270-006-9198-7
- Vodyanitskii YN. Methods of sequential extraction of heavy metals from soils: new approaches and the mineralogical control (a review). Eurasian Soil Sci. 2006;39:1074–1083.10.1134/S106422930610005X
- Ma LQ, Rao GN. Chemical fractionation of cadmium, copper, nickel, and zinc in contaminated soils. J Environ Qual. 1997;26:259–264.10.2134/jeq1997.00472425002600010036x
- Rachou J, Sauve S. Evaluation of affinity constants of Cu, Cd, Ca and H for active soil surfaces for a solid phase-controlled soil ligand model. Environ Chem. 2008;5:150–160.10.1071/EN07093
- Chopin EIB, Black S, Hodson ME, et al. A preliminary investigation into mining and smelting impacts on trace element concentrations in the soil and vegetation around Tharsis, SW Spain. Mineral Mag. 2003;67:279–288.10.1180/0026461036720099
- Maramba NP, Reyes JP, Francisco-Rivera AT, et al. Environmental and human exposure assessment monitoring of communities near an abandoned mercury mine in the Philippines: a toxic legacy. J Environ Manage. 2006;81:135–145.10.1016/j.jenvman.2006.02.013
- Cao X, Ma LQ, Chen M, et al. Weathering of lead bullets and their environmental effects at outdoor shooting ranges. J Environ Qual. 2003;32:526–534.10.2134/jeq2003.5260
- Nachtergaele F, De Wit P. Soil map of the Republic of Botswana. Soil mapping and advisory services project; Gaborone;1990. ( FAO/BOT/85/011).
- Cheng SF, Huang CY, Lin MS. Sodium acetate method for determining CEC of cadmium-contaminated soil. Sustain Environ Res. 2012;22:85–89.
- Nelson DW, Sommers LE. Total carbon, organic carbon, and organic matter. In: Page AL, Miller RH, Keeney DR. editors. Methods of soil analysis, part 2 chemical and microbiological properties 9. Madison (WI): ASA; 1982. p. 539–577.
- Peddicord R. Synopsis of applications and limitations of TCLP and SPLP at outdoor shooting ranges. Newtown (CT): Facility development series No. 4, National shooting sports foundation; 1998. p. 1–12.
- Reid S, Cohen SZ. A new tool to predict lead mobility in shooting range soils: predicting SPLP results. The 16th Annual International Conference on Contaminated Soils, Sediments and Water; Amherst: University of Massachusetts; 2000.
- US Environmental Protection Agency (USEPA). Test methods for evaluation of solid waste. vol. IA, laboratory manual physical/chemical methods, SW 846, 40 CFR parts 403 and 503. 3rd ed. Washington (DC): US Government Printing Office; 1995.
- Kabala C, Singh BR. Fractionation and mobility of copper, lead, and zinc in soil profiles in the vicinity of a copper smelter. J Environ Qual. 2001;30:485–492.10.2134/jeq2001.302485x
- Mellor A, McCartney C. The effects of lead shot deposition on soils and crops at a clay pigeon shooting site in northern England. Soil Use Manage. 1994;10:124–129.10.1111/sum.1994.10.issue-3
- Bricka M. Soil treatments to limit lead mobility’. Fourth National Shooting Range Symposium; Policy Track: Environmental Issues; Vicksburg, Mississippi; 2000. p. 123–125.
- Abadin H, Ashizawa A, Stevens YW. Toxicological profile for lead. Atlanta (GA): US Public Health Service and Agency Toxic Substance and Disease; 2007. p. 582.
- Sporting Arms and Ammunition Manufacturers’ Institute, SAAMI. Lead mobility at shooting ranges. EA Engineering, Science, and Technology, Inc. 11019 McCormick Road, Hunt Valley (MD) 21031; Project No. 13081.01, Flintlock Ridge Office Center, 11 Mile Hill Road, Newtown, CT 06470; 1996. p. 22.
- Lin Z. Secondary mineral phases of metallic lead in soils of shooting ranges from Orebro county. Sweden. Environ Geol. 1996;27:370–375.10.1007/BF00766707
- Jorgensen SS, Willems M. The fate of lead in soils: the transformation of lead pellets in shooting range soils. Ambio. 1987;6:11–15.
- Ashraf MA, Ahmad M, Akib S, et al. Chemical species of metallic elements in the aquatic environment of an ex-mining catchment. Water Environ Res. 2014;86:717–728.10.2175/106143014X13975035525825
- McBride MB. Environmental chemistry of soils. Oxford: Oxford University Press; 1994.
- Kotoky P, Bora BJ, Baruah NK, et al. Chemical fractionation of heavy metals in soils around oil installations, Assam. Chem Spec Bioavailab. 2003;15:115–126.10.3184/095422903782775181
- Aikpokpodion PE, Lajide L, Aiyesanmi AF. Assessment of heavy metals mobility in selected contaminated cocoa soils in Ondo state, Nigeria. Global J Environ Res. 2012;6:30–35.
- Ismail THT, Adnan NAF, Samah MAA. The accumulation of Fe, Pb, Zn, Ni and Cd in Nerita lineata and Thais bitubercularis obtained from Tanjung Harapan and Teluk Kemang, Malaysia. J Clean WAS. 2017;1:6–16.
- Halim NIA, Phang IC. Salicylic acid mitigates Pb stress in Nicotiana Tabacum. G War Sains. 2017;1:16–19.
- Sanderson P, Naidu R, Bolan N. The effect of environmental conditions and soil physicochemistry on phosphate stabilisation of Pb in shooting range soils. J Environ Manage. 2016;170:123–130.10.1016/j.jenvman.2016.01.017
- Ahmad M, Lee SS, Lim JE, et al. Speciation and phytoavailability of lead and antimony in a small arms range soil amended with mussel shell, cow bone and biochar: EXAFS spectroscopy and chemical extractions. Chemosphere. 2014;95:433–441.10.1016/j.chemosphere.2013.09.077
- Moon DH, Park J, Chang Y, et al. Immobilization of lead in contaminated firing range soil using biochar. Environ Sci Pollut Res. 2013;20:8464–8471.10.1007/s11356-013-1964-7
- Ashraf MA, Maah MJ, Yusoff I. Removal of lead from synthetic solutions by protonated teleosts biomass. E-J Chem. 2012;9:345–353.10.1155/2012/769180