Abstract
The effects of Cd on the growth and Cd uptaking in corn (Zea mays L.) were explored under different Cd stress. The results showed that no reduction in shoot and root dry matter yields were noted when the plants were grown at Cd supply levels ≤100 μmol l−1 nutrient solution. The Cd concentration in the shoots and roots of corn increased sharply with increasing external Cd supply levels, peaked at 50 μmol l−1, and then decreased slowly with further increasing Cd levels due to high Cd toxic effects on root growth. The concentrations of chlorophyll a, chlorophyll b and chlorophyll a + b in the leaf of corn decreased slowly with increasing external Cd supply levels. Proline concentrations of corn increased when the plants were grown under the external Cd influence. The lower concentration of Cd treatments did not influenced the growth of corn significantly, and increased the uptake of Cd, the higher levels of Cd supply caused significantly physiological resposes and decreased the Cd uptaking.
Cd contamination has been a greater emphasis towards the problems of Cd pollution with the development of modern industry and agriculture [Citation1]. Cd contamination of agricultural soil has been becoming a serious environmental concern. Cadmium is a non-essential metal for crops, can be extremely toxic and characteristically causing growth inhibition, chlorophyll breakdown, leaf chlorosis and browning of root, Excessive Cd uptake by plants effected essential physiological process, induced oxidating stress and caused damage in plants by increasing the production of reactive oxygen species and lipid peroxidation [Citation2–6]. Cd ecotoxicity induced low biomass of crops compensated by very high metal concentrations in the shoots and roots [Citation7–10]. Crops in agricultural soil take up Cd from the soil pore water, while the uptaking content was usually associated with the concentration of total Cd in soil solution [Citation11–14]. Cd accumulation in plants has been extensively studied, however, the mechanism by which Cd toxicity causes plant tissue injure are not yet fully understood.
Corn is a common and important agricultural crop worldwide, is the most plentiful sources of the agricultural resources. We aimed to investigate the effects of Cd on the growth and Cd uptake for corn (Zea mays L.).
Materials and methods
Plant culture
Seeds of corn (Zea mays L.) cultivar were offered by Gansu Academy of Agricultural Sciences. Seeds were germinated in a perlite medium and under intermittent mist until the development of the first true leaves. Plants were then grown in a greenhouse under natural light and temperature (average day, 24 °C; night, 22 °C) conditions. Seedlings were watered with deionized water or quarter-strength Hoagland’s No.1 solution. At the two-true-leaf stage roots were washed free of perlite, and selected plants were transferred to 2 l of quarter-strength modified Hoagland’s solution in aerated, polyethylene containers.
After pre-cultured for 12 days, plants were subjected to full-strength modified Hoagland’s solutions. The composition of the nutrient solution was (in mmol l−1): Ca(NO3)2·4H2O 2.0, KH2PO4 0.10, MgSO4·7H2O 0.50, KCl 0.10, K2SO4 0.70, and (in μmol l−1): H3BO3 10.00, MnSO4·H2O 0.50, ZnSO4·7H2O 0.50, CuSO4·5H2O 0.20, (NH4)6Mo7O24 0.01, Fe-EDTA 100. Cadmium (Cd) in the form of cadmium chloride (CdCl2) was supplemented in Hoagland nutrient solution at different concentrations, 12.5, 25, 50, 100, 200, 400, 800 μmol Cd l−1 according to the demand for testing Cd ecotoxicity from mild to severe stress. A randomly complete block design was used with each treatment replicated three times. The nutrient solution was aerated and replaced every four days, and the pH was maintained at 5.8 by daily adjustment with 0.1 mol l−1 HCl or 0.1 mol l−1 NaOH. Plants were grown under controlled glasshouse conditions at a temperature of 27 ± 3 °C, and relative humidity of 70 ± 3%.
Plant samples and analysis of cadmium
The experiment was terminated and the plants were harvested after they were grown in the metal treated nutrient solution for 21 days. At harvest, roots of intact plants were rinsed with tap water and then were immersed in 20 mmol l−1 Na2-EDTA (disodium ethylenediaminetetraacetate) for 15 min to remove Cd adhering to root surfaces. Roots were washed for 5 min with distilled water. The plants were divided into two samples for analyses: the fully mature leaves and the roots and were rinsed three times with deionized water. All tissues were dried at 80 °C for 48 h in a forced-air oven, and dry weight (DW) was determined. Dried leaf samples were ground by mortar and pestle, and other plant tissues were ground in a rotary (Wiley) mill to pass a 40-mesh (0.5 mm) screen. After grinding, portions of all plant tissues were ashed in a muffle furnace at 500 °C for 18 h, and the resulting ash was dissolved in 10 ml of concentrated HNO3 and diluted to a final volume of 50 ml with distilled water. These digested samples were the basis for the determination of Cd in plant tissues. The concentrations of Cd in the solutions were measured using inductively coupled plasma atomic emission spectrometry (ICP-AES).
Chlorophyll and carotenoid analyses
The pigments were extracted from fresh leaf discs in 80% acetone. The chlorophyll and carotenoid contents were determined by spectrophotometry according to the procedure described by Lichtenthaler (1987) and using the following equations for the determination of the concentration of total chlorophyll and carotenoid in the leaf pigments:
where Ca = chlorophyll a; Cb = chlorophyll b; Ca + b = total chlorophyll; Cx + c = carotenoid, Aλ = absorbance at λ (nm).
Proline analysis
Extraction and determination of free proline was carried out from freeze dried material (Bates et al., 1973). A sample of 0.1 g leaf material was homogenized in 10 ml of 3% 5-sulfosalysilic acid solution. The homogenate was filtered through Whatman No. 1 filter paper. Proline was analyzed by reacting 2 ml of the extract with 2 ml of glacial acetic acid and 2 ml of ninhydrin solution. The mixture was incubated in a boiling water bath for 1 h. After cooling, 4 ml toluene was added and vigorously shaken. The absorbance of the toluene phase was determined at 520 nm in a Cecil 5000 spectrophotometer.
Bioconcentration factor (BCF) and transportation index (Ti)
Cadmium uptake, depicted by a bioconcentration factor (BCF), provides an index of the ability of the plant to accumulate a particular metal with respect to its concentration in the growing substrate. It is calculated as follows:
The transportation index (Ti) gives the shoot/root cadmium concentration and depicts the ability of the plant to transfer the metal species from roots to shoots at different concentrations.
Data analysis
Analysis of variance (ANOVA) for the data was performed on all data sets. Least significant different (LSD) was used for multiple comparisons between treatment means. Statistical analysis was performed using STATISTICA software (Statsoft, 1993).
Results
Response of plant growth to different Cd levels
Plant growth of corn was normal at the Cd levels ≤200 μmol Cd l−1. However, visual Cd toxicity symptoms with necrosis and browned root tip were observed on the roots of corn grown for 7 days at external Cd level of 400 μmol Cd l−1. The toxicity symptoms became more severe with increasing Cd levels and exposure time. The leaves of corn wilted after the plants were grown for 7 days, and the old leaves began to fall off after grown for 14 days at 800 μmol Cd l−1.
Reduced shoot height was noticed when plants were grown at Cd levels ≥200 μmol l−1, whereas, shoot fresh and dry weights slightly increased when plants were grown at the Cd levels ≤100 μmol l−1 (Table ). However, shoot height and shoot dry matter yields decreased gradually with increasing Cd levels from 200 to 800 μmol l−1, as compared with the control (Table ). Similar response patterns to Cd supply levels were noted between the shoot height and the shoot dry weight. The maximum root length decreased with increasing Cd supply levels from 50 μmol l−1 and dramatically decreased at Cd levels higher than 400 μmol l−1 (Table ). The maximum root length grown at 800 μmol Cd l−1 was only about 48% of the control. There was no obvious decrease in root fresh and dry weights of corn when the plants were grown at the Cd levels ≤100 μmol l−1 (Table ).
Table 1. Effects of Cd supply levels on the growth of corn.
Cadmium concentration and accumulation at different Cd supply levels
The Cd concentration in the shoots and roots of corn increased sharply with increasing external Cd supply levels, peaked at 50 μmol l−1, and then decreased slowly with further increasing Cd levels (Figure ). The maximum Cd concentrations in the shoots and roots were 389.5 and 505.5 mg kg−1 (DW), respectively, when the plants were grown at 50 μmol Cd l−1 for 21 days. There was no significant (p 0.05) difference between Cd supply level of 400 and 800 μmol l−1 for root Cd concentration. The values of Cd concentration in the roots were higher than that of shoots at the Cd supply level ≤200 μmol l−1. These results indicate that corn has an extraordinary ability to accumulate Cd in the roots. In view of Cd concentrations in the shoots of corn, the plant could be considered as a Cd-hyperaccumulator, according to the current accepted shoot concentration that defines hyperaccumulation as 0.01% (w/w) for cadmium.
Figure 1. Cadmium concentrations in the shoots and roots of corn grown at different Cd supply levels. Data with the same letter represent statistically identical values (p 0.05).
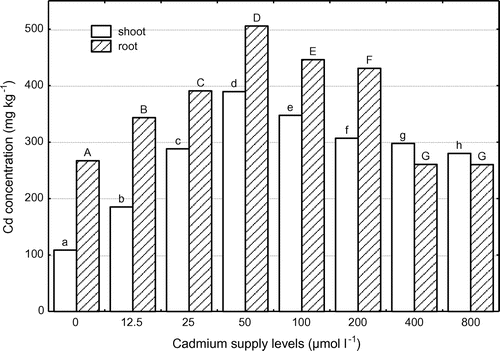
Cadmium uptake by the shoots and roots linearly increased with increasing Cd supply levels, peaked at the Cd level of 50 μmol l−1, and then dramatically decreased with further increasing external Cd levels (Figure ). The maximum amounts of Cd taken up by the shoots and roots were as high as 105.2 and 126.4 μg pot−1, respectively, grown at the external Cd level of 50 μmol l−1. The amounts of Cd accumulated in the roots were higher than that of shoots except at the Cd supply levels of 400 and 800 μmol l−1. High Cd (≥400 μmol l−1) treatments considerably reduced Cd accumulation in the roots due to its toxic effects on root growth.
Ti and BCF
The shoot/root cadmium concentration index (Ti) is shown for corn in Table . Maximum transport was observed at 400 μmol l−1 with the value of 1.14, followed by 1.08 at 800 μmol l−1. For other treatments it was 1, indicating that the root Cd concentration was always high. In the case of 0 and 12.5 μmol l−1, the large difference between root and shoot concentrations indicates an important restriction of the internal transport of Cd from roots towards shoots, resulting in higher root concentrations rather than translocation to shoots. The apoplastic barriers in roots played an important role in corn under Cd supply stress. Higher concentrations of Cd could result in the damage of root apoplastic barriers, which will be benefit for retarding the transport of Cd ion from roots to shoots.
Table 2. The transportation index (Ti) of Cd in plants grown for 21 days.
The BCF decreased with increasing Cd supply level, according to the equation as below:
where y is bioconcentration factor and x is cadmium supply level (μmol l−1). It differed according to the treatment. However, higher Cd in the plant in comparison to substrate has been reported at lower levels of substrate Cd (Robinson et al., 2000) (Figure ).
Contents of chlorophyll and carotenoid in corn at different Cd supply levels
The concentrations of chlorophyll a, chlorophyll b and chlorophyll a + b in the leaf of corn decreased slowly with increasing external Cd supply levels. At the treatment of 800 μmol l−1, the concentration values of chlorophyll a, chlorophyll b and chlorophyll a + b in the leaves were 33.03, 50.67 and 83.70 mg 100 g−1 FW, which decreased to 38.9, 46.0 and 42.9% of the control plants, respectively. There was no obvious change in the ratio chlorophyll a/chlorophyll b among eight treatments, which indicated that the influence of Cd on the concentration of chlorophyll a and chlorophyll b in corn leaf was similar (Table ). As compared with the control, the decrease in leaf chlorophyll a concentration was statistically significant (p 0.05) at Cd supply levels of 400 and 800 μmol l−1. Similarly, the content of carotenoid in the leaves of corn also decreased with increasing external Cd supply levels (Table ).
Table 3. Effect of cadmium on leaf chlorophyll and carotenoid contents of corn at different cadmium supply level for 21 days.
Proline concentration in corn at different Cd supply levels
Proline concentrations of corn increased linearly when the plants were grown at the Cd supply levels less than 50 μmol l−1. There was no significant difference between the treatments of 50 and 100 μmol l−1 (p 0.05). The maximum amount of proline concentration was as high as 26.58 μg g−1 FW at the external Cd level of 400 μmol l−1, and then decreased with further increasing Cd level (Figure ). The increased proline concentration indicated that corn could adapt to the external conditions via changing the concentration of proline.
Discussion
Cadmium is a toxic element without any known physiological function in plants. Stunting is a commonly observed growth response in a wide range of plants in metal induced soils. It may be due to specific toxicity of the metal to the plant metabolism, or antagonism with other nutrients in plants. It is possible that Cd-treated plants show stunting due to deficiency of an element such as phosphorous, which has been shown to form insoluble complexes with Cd [Citation7].
Free cadmium (Cd2+) is probably the most available form to plants. Soluble Cd can enter roots either by movement in the cell wall free space (apoplastic pathway) or by transport across the plasma membrane of root cells and movement through the cytoplasm (symplastic pathway). It may also travel across the cell and ultimately enter the translocation stream. Generally, toxic metals cause enzyme inactivation and damage to cells by acting as antimetabolites or forming precipitates with essential metabolites [Citation1,5,6,15]. Although roots absorb an appreciable fraction of Cd2+, it is translocated to other parts of the plant [Citation8]
The chlorophyll content is often used to assess the impact of environmental stresses in plants. The reason is that changes in pigment contents are linked to visual symptoms of plant illness and photosynthetic productivity [Citation16]. Heavy metals often inhibit metabolic processes by inhibiting the action of enzymes. Decreased chlorophyll contents associated with heavy metal stress may be the result of the inhibition of enzymes responsible for chlorophyll biosynthesis [Citation6]. In our studies, the contents of pigment contents (including carotenoid) in the leaves of corn all decreased slowly with increasing external Cd supply levels. As regards the effect of elevated concentration of heavy metal on the synthesis and accumulation of photosynthetic pigments, contradictory data have been reported: some researchers reported decreased pigment content, whereas others found it unchanged or even increased [Citation17]. Accumulation of free proline in response to heavy metal exposure seems to be widespread among plants. Proline increases the stress tolerance of plants through mechanisms such as osmoregulation, protection of enzymes against denaturing, and stabilization of protein synthesis [Citation6].
Conclusions
The amounts of Cd accumulated in the roots were higher than that of shoots at relatively low Cd supply levels (400 μmol l−1). High Cd (≥400 μmol l−1) treatments considerably reduced Cd accumulation in the roots due to its toxic effects on root growth. The concentrations of chlorophyll a, chlorophyll b and chlorophyll a + b in the leaf of corn decreased slowly with increasing external Cd supply levels. The influence of Cd on the concentration of chlorophyll a and chlorophyll b in corn leaf was similar. The increased proline concentration indicated that corn could adapt to the external conditions via changing the concentration of proline.
Funding
This research was supported by the National Natural Science Foundation [grant number 30970490].
Disclosure statement
No potential conflict of interest was reported by the authors.
References
- Ashraf MY, Roohi M, Iqbal Z, et al. Cadmium (Cd) and Lead (Pb) induced changes in growth, some biochemical attributes, and mineral accumulation in two cultivars of mung bean [Vigna radiate (L.) Wilczek]. Commun Soil Sci Plant Anal. 2016;47(4):405–413.
- Doganlar ZBP, Yurekli F. Interactions between cadmium and phytochelatin accumulation in two different sunflower cultivars. Fresen Environ Bull. 2009;18(3):304–310.
- Michel-López CY, Espadas y Gil F, Fuentes Ortíz G, et al. Bioaccumulation and effect of cadmium in the photosynthetic apparatus of Prosopis juliflora. Chem Spec Bioavailab. 2016;28:1–6.10.1080/09542299.2015.1129290
- Okem A, Moyo M, Stirk WA, et al. Investigating the effect of cadmium and aluminium on growth and stress-induced responses in the micropropagated medicinal plant Hypoxis hemerocallidea. Plant Biol. 2016;18:805–815.10.1111/plb.12480
- Schat H, Sharma SS, Vooijs R. Heavy metal-induced accumulation of free proline in a metal-tolerant and a nontolerant ecotype of Silene vulgaris. Physiol Plant. 1997;101:477–482.10.1111/ppl.1997.101.issue-3
- Vijendra PD, Huchappa KM, Lingappa R, et al. Physiological and biochemical changes in moth bean (Vigna aconitifolia L.) under cadmium stress. J Bot. 2016;1–13.10.1155/2016/6403938
- Gonçalves JF, Antes FG, Maldaner J, et al. Cadmium and mineral nutrient accumulation in potato plantlets grown under cadmium stress in two different experimental culture conditions. Plant Physiol Biochem. 2009;47:814–821.10.1016/j.plaphy.2009.04.002
- Jiang WS, Liu DH, Hou WQ. Hyperaccumulation of cadmium by roots, bulbs and shoots of garlic (Allium sativum L.). Biores Tech. 2001;76:9–13.10.1016/S0960-8524(00)00086-9
- Liu JG, Cai GL, Qian M, et al. Effect of Cd on the growth, dry matter accumulation and grain yield of different rice cultivars. J Sci Food Agric. 2007;87:1088–1095.10.1002/(ISSN)1097-0010
- Ozdener Y, Kutbay HG. Toxicity of copper, cadmium, nickel, lead and zinc on seed germination and seedling growth in Eruca sativa. Fresen Environ Bull. 2009;18(1):26–31.
- Gounden D, Kisten K, Moodley R, et al. Impact of spiked concentrations of Cd, Pb, As and Zn in growth medium on elemental uptake of Naturtium officinale (watercress). J Environ Sci Health B. 2016;51(1):1–7.10.1080/03601234.2015.1080477
- Kutrowska A, Małecka A, Piechalak A, et al. Effects of binary metal combinations on zinc, copper, cadmium and lead uptake and distribution in Brassica juncea. J Trace Elem Med Biol. 2017;44:32–39.10.1016/j.jtemb.2017.05.007
- Rahman M, Haq N, Williams ID. Phytoaccumulation of arsenic, cadmium and lead by Brassica juncea parents and their F1 hybrids. J Environ Prot. 2016;07:613–622.10.4236/jep.2016.75055
- Wang M, Zou JH, Duan XC, et al. Cadmium accumulation and its effects on metal uptake in maize (Zea mays L.). Bioresour Technol 2007; 98: 82–88.10.1016/j.biortech.2005.11.028
- Zaimoglu Z, Attilla P. The Uptake and translocation of hexavalent chromium and effects on growth and enzyme activity of Zea mays L.. J Food Agric Environ 10(3&4): 982–986.
- Anjum SA, Ashraf U, Khan I, et al. Chromium and aluminum phytotoxicity in maize: morpho-physiological responses and metal uptake. Clean. 2016;44(8):1075–1084.10.1002/clen.v44.8
- Sfaxi-Bousbih A, Chaoui A, El Ferjani EE. Coper affects affects the cotyledonary carbohydrate status during the germination of bean seed. Bio Trac Elem Res. 2010;137:110–116.10.1007/s12011-009-8556-x