ABSTRACT
The transport and fractionation of copper (Cu) were investigated in three soils which were under continuous citrus production for over 30 years. The above soils received 50 kg Cu ha−1, and were leached with eight pore volumes of water (equivalent to a total of 91 cm of rainfall). Following the completion of leaching, the leaching columns were sectioned into 5 cm depth increments for analyses of soil Cu. Concentrations of different forms of soil Cu were analyzed using a sequential fractionation procedure. The leaching column study indicated as much as 98% of the applied Cu remained in the upper 5 cm of the soil despite leaching eight pore volumes of water. Copper recovered in eight pore volumes of leachate accounted for 0.18–2.87% of Cu applied. The organically bound and precipitated forms of Cu accounted for 33–87% and 6–44% of total soil Cu, respectively.
Introduction
A substantial portion of the soils in much of the Florida citrus belt has been under continuous citrus production for over 40 years. Since the early 1900s copper (Cu) has been applied: i) in rates ranging from 10–25 kg Cu ha−1 yr−1 as an amendment to control citrus dieback, and ii) 5–10 kg Cu ha−1 yr−1 as a common fungicide [Citation1]. The annual removal of Cu in harvested portion (fruit) of the trees is extremely low. A study shows that 40.8 Mg of oranges (on fresh weight basis, maximum potential production per hectare, equivalent to 1000 boxes per acre) contains only 0.023–0.031 kg of Cu [Citation2]. Therefore, on annual basis the depletion of accumulated Cu in soils by harvested portion of the grapefruit trees is insignificant. Accumulation of Cu in quantities exceeding 500 kg ha−1, in the top 15 cm soil, has been evident [Citation1,Citation3–Citation6]. Adrees et al. [Citation7], proposed the optimal range for soil Cu as 5–30 mg kg−1. The average concentration of Cu in soils across the United States was reported as 9 mg kg−1, but was less than 5 mg kg−1 for the highly weathered soils of the southeastern Coastal Plains [Citation8]. Ma et al. [Citation9] reported Cu concentrations less than 4.0 mg kg−1 for 24 Entisols with no history of cultivation. In contrast, Cu concentration can increase to as high as 539 mg kg−1 in soils with continuous citrus production for a number of years [Citation3]. Accumulation of Cu in soils has also been reported in apple orchards; up to 700 mg Cu kg−1 in Japan [Citation10] and in vineyards as high as 1280 mg Cu kg−1 in Germany [Citation11]. Ballabio et al. [Citation12], reported that 14.6% soil samples collected from vineyards in Europe were >100 mg kg−1.
Although Cu is required in trace amount for various metabolic processes in plants, at high concentrations Cu is toxic to plant growth. The Cu toxicity to plants includes decreasing photosynthesis [Citation13–Citation15], affecting nitrogen metabolism [Citation16] and reducing nutrient uptake [Citation16,Citation17]. Excess soil Cu decreased the uptake of Fe and causing Fe chlorosis in Florida sandy soils [Citation18].
Copper is the least mobile trace element in soils. However, Cu has potential to leach from surface horizon into a lower horizon in extreme sandy soils [Citation19–Citation21]. Most soils under citrus production in Florida are extremely sandy (>90–95% sand) with very little organic matter content (<0.5%). The mean annual rainfall in this region is about 1500 mm, with roughly 60% of the total annual rainfall received during June–September. The above factors may contribute to transport of Cu in the soil profile. South Florida Water Management District reported the elevated concentrations of copper and other metals in Indian River Lagoon sediments related to water flowering from drainage channels in agricultural area [Citation22].
The objective of this study was to examine the distribution, transport, and fractionation of Cu in three sandy soils under intensive crop production.
Materials and methods
Soil samples were collected from citrus groves in St. Lucie, Martin, and Polk Counties, Florida. These samples represented three soil series, namely Wabasso (sandy, siliceous, hyperthermic Alfic Haplaquods), Riviera (loamy, siliceous, hyperthermic Arenic Glossaqualf), and Candler (Hyperthermic, uncoated Typic Quartzipsamment). In the case of the Wabasso sand, samples were taken at A (surface horizon; 0–25 cm), E (sand horizon; 35–60 cm), and Bh (spodic horizon; 60–85 cm) horizons. Only the surface horizon was sampled for the Riviera (0–30 cm) and Candler (0–20 cm) series. Soil samples were air dried, ground, and sieved to pass through a 2-mm sieve. Particle size distribution was analyzed following a micropipette method. Soil pH was measured in a 1:1 (w/v) ratio of soil and water suspension. Organic carbon content was analyzed by the Walkley-Black method. The procedure reported by Shuman [Citation23] was used to measure the concentrations of total Fe, Mn, and Al. Selected properties of the soil samples are presented in .
Table 1. Selected properties of the soils used in this study.
Leaching study
Leaching columns (32.5 cm long and 7.5 cm inner diameter) were constructed using clear plexiglass columns. The plexiglass column was cut longitudinally into halves. The two halves were joined with silicon glue. A filter paper was placed on the bottom of the column which had a nylon mesh over a Plexiglass plate with several 5 mm diameter holes. The soil (1.6 kg per column) was packed to 25 cm high with a mean bulk density of 1.45 g cm−3. Soil columns were saturated using deionized water and excess water was allowed to drain for 24 h. A 2.5 mL stock solution (22 g Cu L−1) of CuSO4 was applied uniformly on the soil surface using a pipette. On a surface area basis, the amount of Cu applied on a Cu treated column was equivalent to 50 kg Cu ha−1. A filter paper was placed on the soil surface and deionized water was applied using a peristaltic pump at a rate of 1.5 ml min−1. The leachate was collected at each half pore volume (250 mL) for a total of eight pore volumes. The quantity of water applied to the soil column for each pore volume of leachate was equivalent to 11.38 cm of rainfall on the surface of the soil column. The total amount of water applied was 91.04 cm which is equivalent to 65% annual rainfall (139.7 cm) in the experimental region. The average rainfall for the leachate fractions was filtered through 0.45 µm filter paper and concentration of Cu was determined using inductively coupled plasma atomic emission spectroscopy (ICP-AES; Plasma 40 unit; Perkin-Elmer, Norwalk, CT). After completion of leaching of eight pore volumes of leachate, the plexiglass columns were split into two halves. The soil column was divided into five sections each of 5 cm height. The soil in each section was collected separately, air-dried, and extracted by 4 M HNO3 at 80ºC. The concentration of Cu was measured by ICPES. Each treatment was replicated three times.
Fractionation
Five sections of soil samples in each column were mixed after the completion of the above steps. The modified method of Sposito et al. [Citation24], was employed for fractionation. This procedure involved sequential extraction of the soil sample (2 g) with: 1) 0.5 M KNO3 (16 h); exchangeable Cu; 2) 0.5 M NaOH (16 h); organically bound Cu; 3) 0.05 M Na2 EDTA (6 h); precipitated Cu; and 4) 12.5 ml 4 M HNO3 at 80ºC (12 h); residual Cu. During each extraction, the soil suspension was shaken. After extraction, the suspension was centrifuged and the resulting supernatant solution was decanted and filtered. The concentrations of Cu in the above extractions were determined using ICP-AES. Analysis of variance was calculated using Statistical Analysis System (SAS) program.
Results and discussion
Cumulative leaching of Cu in eight pore volumes was significantly different (P = 0.001) among the soil horizon samples used in this study (). Copper recovered in eight pore volumes of leachate accounted for 0.2%, 0.4%, 0.5%, 2.4% and 2.9% of Cu applied, 50 kg Cu ha−1 for the Wabasso Bh, Wabasso Ap, Riviera Ap, Candler Ap, and Wabasso E horizon samples, respectively (). An increased proportion of Cu in the leachate in the case of the Wabasso E horizon soil may be due to its low organic matter content (1 g kg−1) as compared to the other soil horizon samples (7–47 g kg−1) (). Taylor et al.’s [Citation25] study on some contaminated forest soils showed only 1–2% of total Cu was leached.
Figure 1. Cumulative amount of Cu recovered in eight pore volumes of leachate from five soil horizon samples expressed as percentage of Cu applied (50 kg Cu ha−1). The vertical line on the top of each histogram represents the standard error of the mean.
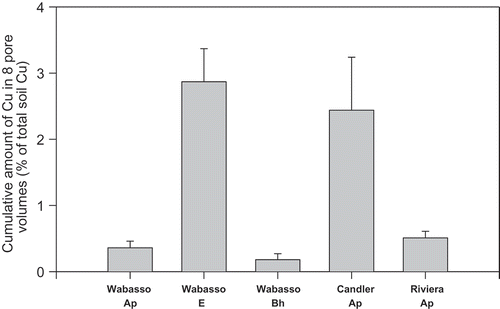
The peak concentrations of Cu in the leachate were 2.3, 1.6, 0.5, 0.4 and 0.3 mg L−1 for Wabasso E, Candler Ap, Riviera Ap, Wabasso Bh and Wabasso Ap soils, respectively ().
Figure 2. Elution curves of Cu for five soil horizon samples amended with 50 kg Cu ha−1 and leached continuously with eight pore volumes of water.
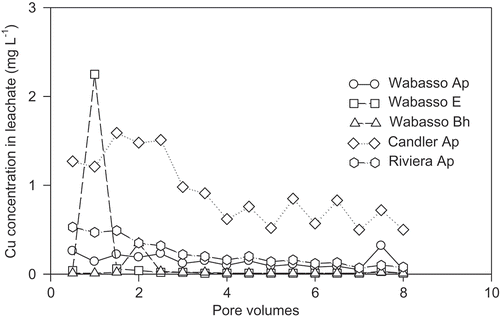
About 32–98% of total Cu (applied plus original Cu in soil) remained in the upper 5 cm of the soil column after leaching eight pore volumes of water in all soil horizon samples (.). Merry et al. [Citation26], reported over many years of Cu application as pesticide accumulation primarily in the surface soils (0–25 cm) of orchards (apple and pear trees) of Cu in concentrations up to 320 mg kg−1 with very little evidence of Cu transport accumulation below 25 cm in the soil profile. Labeled Cu applied to the soil surface was leached to a maximum depth of 5 cm in a coarse-textured soil which received 46 cm water additions [Citation27].
Figure 3. Distribution of soil Cu in soil columns following the addition of 50 kg Cu ha−1 and leaching with eight pore volumes of water. The error bar at each data point represents the standard error of the mean.
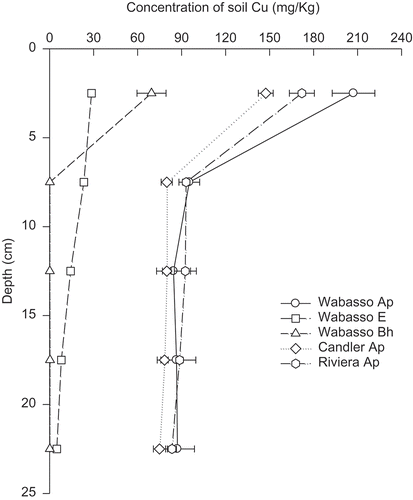
The Wabasso E soil horizon sample had the lowest amount of total Cu among all soils, with 36%, 30% and 34% of this Cu distributed in the 0–5, 5–10 and 10–25 cm depth layers (). Total Cu in the Wabasso E horizon sample without Cu amendment was only 0.20 mg kg−1. These results indicate that in the case of the Wabasso sand, if soil Cu is moved below the Ap horizon into the E horizon, the vertical transport of Cu could be rapid in the E horizon. However, the Bh horizon which is below the E horizon can restrict the downward movement of Cu. Wander [Citation20], conducted a study using five Florida sandy soils which have been under citrus production. At soil pH 5.5–6.0, despite the application of 18.5–27.1 kg Cu ha−1 Yr−1, 90% of the applied Cu remained in the top 30 cm soil. However, when the pH was lowered to 4.2–4.5, approximately 54% of applied Cu leached down to 90 cm.
Among the soil horizon samples evaluated in this study, the organically bound form and precipitated form of Cu accounted for 33–87% and 6–45% of total soil Cu (). The exchangeable form of Cu was 49% of the total Cu in the Wabasso E horizon sample, but less than 6.1% of the total in the rest of the soil horizon samples. Residual Cu was <5% of the total Cu. Copper fractionation study of Zhu and Alva [Citation3], using surface horizon samples from seven different soils from citrus groves also showed that organically bound form was the dominant form of Cu (40.7–73.4% of total soil Cu) while the precipitated form accounted only 17.1–43.8% of total soil Cu. A study by McLaren and Crawford [Citation28], using 24 soils showed that organically bound Cu accounted for 20–50% of the total Cu extracted by 0.1 M Sodium Pyrophosphate.
Figure 4. Proportion of total Cu in various forms in three sandy soils with addition of 50 kg Cu ha−1 and leached with eight pore volumes of water.
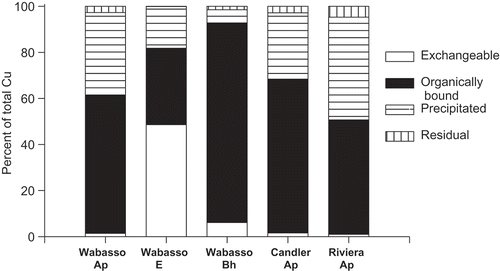
The effects of soil parameters on various forms of Cu were examined by correlation analysis (). There was a significant positive correlation (R2 = 0.99) between the soil pH and concentration of precipitated form of Cu. An increase in soil pH favors the precipitation of soluble forms of soil Cu [Citation29]. Both clay and organic carbon contents were highly correlated (positive) with organically bound form of Cu. Copper can form inner-sphere complexes with soil organic matter and while other metals such as Mn2+, Fe2+ and Co2+ are only bound by outer-sphere complexation [Citation30]. High correlations were also found between the residual Cu forms and concentrations of total Fe, Mn and Al (R = 0.85–0.90). This is probably due to the strong adsorption of Cu on Fe, Mn, Al oxides [Citation19]. McBride [Citation19], proposed that Cu is predominantly bound to either Fe-Mn oxide fraction as substitution of Cu for Fe and Mn in the oxide structure.
Table 2. Correlation coefficients between specific fractions of copper and some soil parameters.
In conclusion, this study shows that the major portion of soil Cu in soils in citrus orchids which received repeated Cu application was presented in organically bound and precipitate forms. Transport of soil Cu down the soil profile was insignificant in these soils. However, there was evidence of Cu leaching in these very sandy soils without the spodic horizon (hard pan). Soil pH, organic matter, total Fe, Mn, and Al concentrations are highly related to Cu fractions and mobility.
Correction Statement
This article has been republished with minor changes. These changes do not impact the academic content of the article.
Disclosure statement
No potential conflict of interest was reported by the authors.
Additional information
Funding
References
- Alva AK, Graham JH. The role of copper in citriculture. Adv Agron. 1991;1:145–170.
- Alva AK, Paramasivam S. An evaluation of nutrient removal by citrus fruit. Proc Fla State Hort Sci. 1998;111:126–128.
- Zhu B, Alva AK. Distribution of trace metals in some sandy soils under citrus production. Soil Sci Soc Am J. 1993;57:350–355.
- Reuther W, Smith PF, Specht AW. Accumulation of the major bases and heavy metals in Florida citrus soils in relation to phosphate fertilization. Soil Sci. 1952;73:375–381.
- Reuther W, Smith PF. Effects of high copper content of sandy soil on growth of citrus seedlings. Soil Sci. 1953;75:219–224.
- Alva AK. Copper contamination of sandy soils and effects on young Hamlin orange trees. Bull Environ Contam Toxicol. 1993;51:857–864.
- Adrees M, Ali S, Rizwan M, et al. The effect of excess copper on growth and physiology of important food crops: a review. Environ Sci Pollut Res. 2015;22:8148–8162.
- Kubota J. Copper status of U.S. soils and plants. In: Loneragan JF, Robson AD, Graham RD, editors. Copper in soils and plants. New York (NY): Academic Press; 1981. p. 363.
- Ma LQ, Tan F, Harris W. Concentrations and distributions of eleven metals in Florida soils. J Environ Qual. 1997;26:769–775.
- Mochizuki T, Chiba S, Haneda S, et al. Ecological study on the apple orchard soils contaminated by inorganic agricultural chemicals. 1. Effects of the contents of residual copper, lead and arsenate on the soil macrofauna of the apple orchards in Tsugaru District of Aomori Prefecture. J Sci Soil Manure. 1975;46:45–50.
- Gartel W. Investigation of copper contents in Weinbergs and Rebschul soils. Weinberg u. Keller; 1957; 4:221.
- Ballabio C, Panagos P, Lugato E, et al. Copper distribution in European topsoils: an assessment based on LUCAS soil survey. Sci Total Environ. 2018;636:282–298.
- Stiborova M, Doubravova M, Brezinova A. Effect of heavy metal ions on growth and biochemical characteristics of photosynthesis of barley (Hordeum vulgare L.). Photosynthetica. 1986;20:418–425.
- Shioi Y, Tamai H, Sasa T. Effects of copper (herbicides) on photosynthetic electron transport systems in spinach chloroplasts. Plant Cell Physiol. 1978;19:203–209.
- Lidon FC, Henriques FS. Effects of copper on the ascorbate, diamine and o-diphenol oxidases activities of rice leaves. Phyton. 1991;52:97–104.
- Lidon FC, Henriques FS. Copper toxicity in rice: diagnostic criteria and effect on tissue Mn and Fe. Soil Sci. 1991;154:130–135.
- Wallace A. Relationships among nitrogen, silicon, and heavy metal uptake by plants. Soil Sci. 1989;147:457–460.
- Alva AK, Chen EQ. Effects of external copper concentrations on uptake of trace elements by citrus seedlings. Soil Sci. 1995;159:59–64.
- McBride, M.B. Forms and distribution of copper in solid and solution phases of soil. In Loneragan JF, Roberson AD, Graham RD, editors. Copper in soils and plants. Sydney: Academic Press Australia; 1981. p. 25–45.
- Wander IW. The total manganese, copper and zinc content of soils used for citrus production in Florida. Soil Sci Soc Fla Proc. 1954;14:27–33.
- Dragicevic I, Eich-Greatorex S, Sogn TA, et al. Fate of copper, nickel and zinc after biogas digestate application to three different soil types. Environ Sci Pollut Res. 2017;24:13095–13106.
- South Florida Water Management District (SFWMD). Ecosummary. West Palm Beach (FL): Southeast District Assessment and Monitoring Program; 1998.
- Shuman LM. Fractionation method for soil microelements. Soil Sci. 1985;140:11–22.
- Sposito G, Lund LJ, Chang AC. Trace metal chemistry in arid-zone field soils amended with sewage sludge: i. fractionation of Ni, Cu, Zn, Cd, and Pb in solid phases1. Soil Sci Soc Am J. 1982;46:260–264.
- Taylor JM, Epstein E, Burger WD, et al. Chemical and Biological Phenomena Observed with Sewage Sludges in Simulated Soil Trenches. J Environ Qual. 1978;7:477–482.
- Merry RH, Tiller KG, Alston AM. The accumulation of copper in Australian orchard soils. In: Loneragem JF, Robson AD, Graham RD, editors. Copper in soils and plants. New York (NY): Academic Press; 1981. p. 364.
- Jones GB, Belling GB. The movement of copper, molybdenum, and selenium in soils as indicated by radioactive isotopes. Aust J Agric Res. 1967;18:733–740.
- McLaren RG, Crawford DV. Studies on soil copper: 1. The fractionation of copper in soils. J Soil Sci. 1973;24:172–181.
- Lindsay WL. Inorganic equilibria affecting micronutrients in soils. In: Mortvedt JJ, Cox FR, Shuman LW, et al., editors. Micronutrients in agriculture. 2nd ed. Madison (WI): Soil Science Society of America, Inc.; 1991. p. 89.
- Bloom PK, McBride MB. Metal ion binding and exchange with hydrogen ions in acid-washed peat. Soil Sci Soc Am J. 1979;43:687–692.