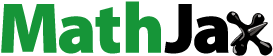
Abstract
This paper discusses margins in the context of building services, where the capacity in many buildings, such as hospitals, exceeds the requirements by huge amounts. This oversizing has a direct impact on building efficiency, capital, maintenance and operational costs, as well as environmental and societal impacts throughout its lifecycle. This paper analyses two in-depth case studies of oversized boiler and chiller systems within NHS hospitals, whereby a number of key factors leading to the oversized systems are identified. The excessive and uncoordinated use of margins that are applied during the various stages of building service projects for a variety of reasons, is one significant factor. The lack of requirements information such as energy demand profiles and the use of vague and unreliable data for initial project requirements specifications, is also a major contributing factor. The paper points to the need to develop robust processes that capture the scope and rationale for the margins applied, to communicate project assumptions and stakeholder requirements in a clear and unambiguous format and to develop systems for improved data capture and analysis.
Introduction
Oversizing building service systems due to the stacking of safety factors is stated as a well- known problem (Djunaedy et al. Citation2011; Dong et al. Citation2018), however, the factors contributing to overdesign are rarely analysed. Sun et al. (Citation2014) attribute the ‘defensive’ and blind application of a safety factor to the building engineers desire to minimise their professional risks. They suggest that existing HVAC sizing methods offer no transparent guarantees that a system design meets the required level of performance. However, they are optimistic that if the practical constraints of lack of long-term weather data and increased computational power are overcome, it would be possible to remove intentional oversizing; and propose a sizing calculation framework for this. By contrast this paper sees oversizing as a sociotechnical problem and analyses the causes of overdesign observed in two case studies in UK hospitals analysing factors that contribute to oversizing during the design and operational phases. The paper looks at overdesign through the lens of design margins and shows that oversizing is not solely caused by adding too many, or too large safety factors, but also design decisions and processes.
Most UK hospitals either form part of the NHS retained estate or are private finance initiative (PFI) buildings, where a private owner retains ownership and rents the buildings to the NHS (see Adamou, Kyriakidou, and Connolly Citation2021). Even without the NHS context, hospitals are extremely complex buildings and have to be resilient to the challenges thrown at them. Hospitals are amongst the most energy use intensive buildings and were rated 2nd highest after restaurants in a US study (Pérez-Lombard, Ortiz, and Pout Citation2008). UK hospitals account for 90% of the total ‘Health’ sector energy footprint. During 2019, this equated to over 1.5% of the total UK energy footprint (UK National Statistics Citation2020). A study of resilience of six Australian hospitals using the World Health Organization (WHO) Hospital Safety Index (HSI) showed that energy provision was the greatest vulnerability in line with previous studies of hospitals in the developing world (Luke et al. Citation2022). Hospitals therefore often have specialist building service systems as well as multiple layers of backup systems, in order to provide resilience. The UK’s Department of Health (DoH Citation2015) attributes approximately 44% of the energy used in a typical UK hospital to air and space heating including energy consumed for ventilation; and a further 5–6% is currently required for cooling (Carbon Trust Citation2010).
To understand the causes of oversizing it is not enough to look at occupancy and environment (see Dong et al. Citation2018 for a review), but it is necessary to consider the whole life-cycle of the equipment. From the way the equipment is procured, how it is maintained and how the demands on building services change over time. As the paper will illustrate, margins remain hidden as nobody takes ownership in considering them. Margins are also often hidden in engineering applications (Eckert, Isaksson, and Earl Citation2019), where different stakeholders add margins throughout the design process, but this information is then not passed on to their colleagues who add further margins. In engineering design, margins can be eroded over time as requirements increase and can cause change avalanches, that lead to significant design effort cost and disruption (Eckert, Clarkson, and Zanker Citation2004). In these cases, not only is the rationale for margins lost but also the knowledge that margins do or do not exist.
One of the reasons why margins are difficult to track is that there is no consistent language around their use. In the context of this paper, the stakeholders talked about ‘overdesign’ or ‘oversizing’ from the user perspective and ‘uplift’ from the installers’ or designers’ perspectives. The literature talks in terms of margins, reserves or excess (De Neufville et al. Citation2004; Djunaedy et al. Citation2011; Eckert, Isaksson, and Earl Citation2019), however there is a common theme that margins are added because individuals are concerned about the uncertainties within the design, they are specifically responsible for, and therefore the professional risk that they personally face.
The aim of this paper is to understand how and why oversizing occurs and to show how excess cost and carbon can arise from oversizing. By taking the lens of margins being added through the life-cycle, the systemic nature of the problem is revealed. The paper looks at hospitals in the UK. Some elements, for example how the procurement processes work, are specific to the UK health context. In terms of building services, hospitals are extremely complex and have highly safety critical systems that are particularly risk averse. Anecdotal evidence points to the fact that this can be an issue in all types of buildings, for example, Djunaedy et al. (Citation2011) look at oversizing of domestic air cooling systems. Not all factors identified by this paper apply to all building types and countries, but the factors are worth considering as potential causes of the overdesign problem.
Two case studies were carried out to investigate the following research questions:
What is the extent and cost of overdesign in building service systems?
What are the potential causes of the overdesign?
How can overdesign be avoided during the design phase?
After a brief discussion of the relevant literature and a description of the methodology, the paper looks at two hospital building service systems; a large 26 MW boiler system and a 3.7 MW centralised chilled water system. The research establishes the rationale for the overdesign across the various phases of specification, design and installation and analyses the barriers to rightsizing, the stakeholder influences and the scope and rationale for the margins added.
Overdesign in the academic literature
As design margins are reviewed systematically in this special issue in Brahma et al. (Citation2023), this literature review focusses on the concepts of design margins and design for flexibility relevant to this paper. The building service literature has not analysed the causes of overdesign but recognises the problem of overdesign as a motivation for better sizing or requirements management. The case studies in this paper revealed that the way redundancy is handled is a major cause of overdesign, therefore concepts of redundancy are included.
Redundancy
Redundancy principles aim at handling failure in the current system (Chen and Crilly Citation2014). The detailed definition varies with the context, for example, Kanno and Ben-Haim (Citation2011) describe redundancy of a structure [as] the extent of degradation which the structure can suffer without losing some specified elements of its functionality. In the case of structures, redundancy can be provided by a single structure that is more resistant to degradation, but in many domains, redundancy is provided by additional components beyond the number actually required for satisfactory operation of a system in order to improve its reliability (Elsayed Citation2012). Redundancy relates to the provision of additional capacity in a system so that system performance is maintained despite partial system failure (Chen and Crilly Citation2014), thereby an important means of achieving reliability.
Redundancy definitions fundamentally fall into two categories: duplication, where an additional system of the same specification is provided (often referred to N + 1 or ‘like for like’) and substitution, where a different solution principle is used to carry out the same function (Chen and Crilly Citation2014). The architects of a system can pursue different strategies during normal operation. Active redundancy is load that is shared across multiple potentially identical components during normal operation, but other components can step up the load if a component fails. Whereas passive redundancy leaves the components that are not required inactive (Pahl and Beitz Citation1996). Passive redundancy can further be broken down into cold redundancy, where is it not absolutely time critical for a component to come online, warm redundancy where the system can tolerate a short outage and hot redundancy, where the system has to function under all circumstances (Nguyen, Mhenni, and Choley Citation2016). Partial redundancy is provided if all components usually work, but the system can withstand individual components that do not work (Thompson Citation1999).
Design margins
The research in this paper started with the working definition by Eckert et al. (Citation2013) of a margin as the extent to which a parameter value exceeds what it needs to meet its functional requirements regardless of the motivation for which the margin was included.
Margins are fundamentally looked at from two perspectives: (1) the margins that are deliberately included against uncertainty, where the effort is placed on identifying what a suitable margin would be and (2) margins that are discovered when interacting with an existing system, where current margins are unknown.
As described in Eckert, Isaksson, and Earl (Citation2019), margins can be conceptualised as having two elements: a buffer which is used to cater for uncertainties and an excess which is the surplus versus the known requirements, see Figure . Excess is the quantity of surplus in a system once the necessities of the system are met (Tackett, Mattson, and Ferguson Citation2014). Only the excess can be used for different purposes. If more usable margins are required either the capability can be increased, e.g. something is made larger, or the uncertainty can be reduced, so that the buffer becomes smaller, and the excess is increased. A fully optimised product would have no excess margin in any of its parts (Eckert et al. Citation2020).
Figure 1. Buffer and excess in margins (Source: Eckert, Isaksson, and Earl Citation2019).
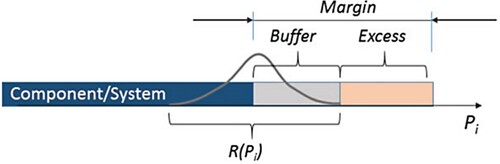
Overdesign and design for flexibility
Overdesign can be a means to increase flexibility in particular in products where requirements change rapidly between product generations (Allen et al. Citation2019). The use of standardised components or platform components can increase the flexibility of product designs (Otto et al. Citation2016), but can hide considerable overdesign as margins of standard components accumulate (Brahma and Wynn Citation2020) and components designed to the worst-case scenario of the most demanding application (Isaksson, Lindroth, and Eckert Citation2014).
Design for flexibility of long-life products can also include an element of overdesign. A design options approach applies margins into a system upfront at a comparatively low cost in anticipation of future changes or upgrades that can already be foreseen; such as an additional floor on a parking garage (De Neufville et al. Citation2004). Design options are a strategic overdesign which is likely to be the responsibility of strategic decision-makers, rather than designers, as the analysis of markets and customer usage is neither in the engineers’ job descriptions nor in their training (De Neufville et al. Citation2004). Usually, the range of expected behaviour is fixed in specification (Banerjee and de Weck Citation2004).
Overdesign in building services
Oversizing is common in building service systems across all building sectors (Abhang Citation2020; Butler Citation2020; Djunaedy et al. Citation2011; Sun et al. Citation2014). In building services, margins are added to safeguard against uncertainty and risk (CIBSE Citation1986) in particular to ‘future proof’ the system. Systems are sized based on engineer’s assumptions based upon occupancy levels, which are often standardised, leading to over-engineered systems for maximum occupancy levels (Bacon Citation2014). HVAC sizing is often based on previous experience and ‘rule of thumb’ estimates (Djunaedy et al. Citation2011) with limited consideration of overall solutions and overall performance/cost ratio (Almefelt Citation2005). Whilst these rule-of-thumb estimates accelerate the design process reducing costs in the short term, overdesigned equipment is more expensive in the long term (Abhang Citation2020). Overdesign could be counteracted by informed calculations and data analysis (Abhang Citation2020; Bacon Citation2014), however good quality energy data are often lacking, making the identification, management and traceability of requirements during the design of building service projects difficult (Chan and Liu Citation2007; Yu and Chan Citation2010). Requirements engineering is a well-recognised problem, both in terms of defining the system scope, and a lack of understanding among various project stakeholders leading to poor specifications (Christel and Kang Citation1992; Hull, Jackson, and Dick Citation2005). Ideally requirements should be unambiguous, complete, concise, traceable, feasible, consistent and necessary (Kamara and Anumba Citation2000; Young Citation2004; Zielczynski Citation2008). Standard design for building service systems looks at uncertainties during peak demand, which given the current practice of ‘defensive sizing’, often leads to oversized systems, wasted capital finance and poor system efficiency (Sun et al. Citation2014).
Traditional static-load and ‘rule of thumb’ approaches are estimated to lead to 50% oversizing at an estimated increased global capital and operational cost of £224bn, while putting the ambitious goal of decarbonising Europe’s building stock by 2050 in jeopardy. (Butler Citation2020)
Overdesign on elements of a system, such as pumps, plate heat exchangers and refrigeration compressors greatly raises the ‘in use’ energy consumption, whilst reducing general plant performance (Soliman Citation2019). Graham (Citation2016) suggests a good strategy is to plan for expansion, but don’t size for it.
Methodology
The building service literature states oversizing as a problem but does not offer a detailed explanation for why it occurs, beyond stating a lack of data and an inadequate management of requirements. This begs the question, why oversizing is occurring and why given the problem is known, it is also allowed to persist. As oversizing is a sociotechnical problem, it benefits from in-depth qualitative research, which might eventually be followed up by quantitative studies. We therefore opted for a case study approach (see Yin Citation2009). As there are only 215 NHS Trusts (Kingsfund Citation2023), getting a sufficient number of responses to a survey would be problematic, especially since – as the study revealed – the Trusts don’t have the relevant explanation readily to hand.
The research was part of a Ph.D. thesis (Jones Citation2022), which set out to look at barriers to energy management in the NHS in general and stumbled upon the issues of overdesign during a study. A further case study was then carried out in a different hospital Trust, where the responsible Director of Estates also suspected that he had taken on a highly overdesigned system, without understanding the rationale for it.
Approach of data gathering and analysis
The thesis and the research that this paper draws on a mixed methods approach combining interviews and document analysis to examine the variety of data and information held across hospital Trusts and their contracting partners. The case studies involved semi-structured interviews with open-ended questions developed to maximise the potential for participant response and rich data. It was necessary to adjust questions during the process, when for example, different participants raised similar issues that had not been accounted for in the original question set. The interviews were conducted by the first author, but the second author joined about half of the interviews in the first case study and all in the second case study. All interviews were recorded and transcribed. The analysis followed a thematic analysis approach (see Braun and Clarke Citation2012). The transcripts were initially coded from a wide range of ‘main theme’ categories, such as ‘finance’. During the second stage, these were broken down into ‘sub-themes’, such as ‘funding opportunity’ or ‘capital investment’ so that synergies could be found across different contexts. This led to the identification of the key themes, including the causes discussed in the following sections.
The Royal Stoke University Hospital (RSUH) case study
This case study took place in 2015 with the view of corroborating the findings of a previous case study into barriers to efficient energy management.
The first 10 interviews, Table addressed general barriers to energy management. During these interviews it very quickly became clear that the new energy centre played an important role, and that this system was hugely oversized. Therefore, questions concerning the sizing of the boiler house were included in subsequent interviews. Interviews that raised issues about the sizing of the boiler system are marked in Table with an asterisk (*). Gaps were addressed through follow-on interviews. It was not possible to interview the external designers of the boiler system. Instead, a Chartered building service design engineer was interviewed (CS2-P11) to better understand the processes and considerations associated with a typical boiler design. The Chartered Engineer had not worked at the RSUH but had worked on many similar design projects.
Table 1. List of interviewees, in chronological order.
In addition, a total of 567 documents concerning the boiler house project were reviewed. The documents were grouped by subject matter (see Table ). Two hundred and six of the 567 documents were provided in Microsoft Word or PDF format and could therefore be analysed with word searches in the program toolbars for terms such as ‘capacity’, ‘heating load’ or ‘margin’, which could relate to margins or overdesign. The relevant documents were then read in detail. The remaining scanned documents could not be machine processed and were instead skim read. Eight documents were selected for closer analysis, but only one document talked explicitly about the application of margins.
Table 2. Document review specific to the boiler house upgrade project.
The John Radcliffe Hospital (JRH) case study
This case study was offered by the ‘new in post’ Estate Director and Manager of the Trust, when participating in a research project on overdesign, because he did not understand why the system was so overdesigned and really wanted the authors to investigate the causes.
Similarly, to the previous study, it involved a combination of interviews with different stakeholders, see Table and a detailed documents analysis, see Table . As the case study Trust had gone through huge changes in personnel within the Estates Department during the processes of design, installation, commissioning and operation of the new boilers, chillers and power generation systems, both the current Estate Director and the Estate Director at the time the work was commissioned, were interviewed. It was also possible to interview representatives of the organisation that provided the Energy Performance Contract (EPC) framework, which provided a list of pre-selected possible suppliers, identified sources of funding, and included access to technical expertise. This organisation worked with the department of health and essentially acted as a broker. The main contractor who carried out the design and new building service installations, and now was responsible for the operation and maintenance of the system, was also interviewed.
Table 3. List of interviewees, in chronological order.
Table 4. Table of four key document categories for analysis.
Overdesigned building service systems
This section looks in detail at an example from each of the two hospital case studies: a boiler system at the RSUH and a chiller system at the JRH. However, even within the case study Trusts, there are other examples of oversizing. At the JRH the boiler system also appeared to be overdesigned providing continuous thermal heat as back up.
The RSUH boiler system
The Royal Stoke University Hospital is one of the largest in the country and a major local employer with over 6000 staff. The first hospital on the site was completed in 1842 and evolved over the years with Victorian and post Second World War buildings. New facilities funded by a PFI were completed in August 2015. The Trust is responsible for the heat and energy provision of the PFI buildings. PFI energy efficiency targets carry penalty clauses (CS2-P8) and shift the risk to the retained estate owned by the public sector. The Trust is effectively locked-in to the contract (CS2-P4). The typical duration of a PFI contract between public and private sector organisations is 25–30 years, but in the case of some hospitals can last up to 60 years (Gaffney et al. Citation1999).
The RSUH spent £4.98 m on energy consumption (gas and electricity) during 2014/2015. The cost would have risen very significantly in the meantime. Many elements of energy management had been subcontracted over recent years. The Board-level interviewee (CS2-P9) commented that:
during the PFI contract negotiations, building energy management was considered, but that it was not currently. There were a number of distractions like the merger … and our A&E issues and all the rest of it.
The Estates Operation Manager added, Due to massive clinical demands and the influencing PFI contract the Trust Board is not prioritising energy efficiency (CS2-P3).
The boiler system had been specifically upgraded to meet the requirements of the new PFI building contract specification.
To meet the thermal requirements of the new PFI development, the energy centre then had to be upgraded, something that Trust-side engineering staff didn’t agree with, as it already seemed to be rather over dimensioned, having three, 4 megawatt (MW) hot water boilers. (CS2-P3)
While the Energy Manager had been concerned about the system being oversized, he did not challenge the system specification in a way that was recorded in the documents. He stated, someone’s calculated it, passed it onto someone else who added their margins and someone else and someone else and you’ve ended up with this figure (CS2-P4).
Figure provides a detailed illustration of the boiler infrastructure developments in chronological order showing the step changes from the 1960s leading up to the time of the case study. The figure clearly shows the change in boiler-house location and the significant boiler capacity change in 2005/2006 leading up to the construction of the PFI development. The figure also illustrates the actual ‘in-use’ site maximum load requirements (yellow line) increasing from just under 4 to 6 MW, respectively, after the construction of the PFI building. The green line shows the average site load. The total installed boiler thermal capacity of the site (red line) increased significantly from 12 to 26 MW as a direct result of PFI contract requirements. This amounts to a 20 MW or 433% overcapacity on the actual ‘in-use’ requirement. Even allowing for an element of buffer in order to cover system redundancy requirements such as breakdown, this system is still significantly over-dimensioned.
Figure 2. Boiler infrastructure changes and capacity increases over time from Jones and Eckert (Citation2017).
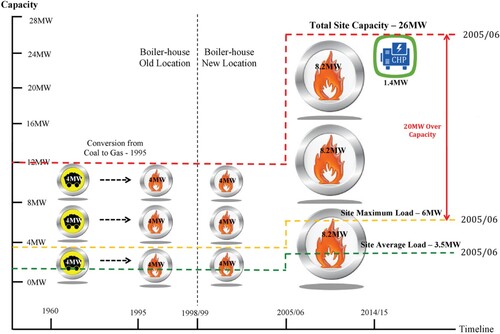
This shows that the system installed in the 1990s would have easily covered the maximum site requirements, with two of its three boilers. In fact, one of its boilers with a 4MW thermal capacity would still be sufficient to meet the site demand for the majority of the year. The current system installed during 2005/2006 covers the thermal needs of the site easily with a single boiler, as only 73% of the possible heat output of one boiler has ever been required. A second boiler is always on standby in case the main boiler fails. Boiler failure is however very rare. This system applies duplication redundancy, where one is sufficient, but two boilers are active and a third boiler is passive.
The financial impact of oversizing of building services is apparent across the whole life-cycle of the installation. The capital cost of the boiler which includes the design, supply of equipment, the installation and commissioning increases in approximate proportion to the size of the installation (Interview P11), hence a boiler that is twice the size, is twice the capital cost. Operating costs are also higher than necessary, as the plant is permanently operating at lower load than its maximum duty point. Hendrick et al. (Citation1992) estimates that a 15% increase in energy consumption is possible if a conventional boiler plant is oversized by 150%. The system operates in summer and in winter mode. In the summer two boilers are active and the third one is shut down, but in the winter the third boiler is kept warm to enable it to come on-line quickly. The overdesign leads to increase losses from turning systems on and off and venting heat through the flue as well as standing losses, i.e. ‘radiation’ from the external surfaces of the boilers. Standing losses are estimated at 3% of the boiler installed capacity (Kenna and Bannister Citation2009). Here we only use the standing losses to indicate the amount of waste as the other losses would require complex system modelling.
Based on actual gas unit costs, of 2.9p per kWh from invoices between April 2014 and March 2015 total standing boiler losses were:
However, the cost would have risen to £538,740 in 2022 for gas tariffs of approximately 10p/kWh. Based on the average of the figures above, over the 20-year life span of the boilers, standing losses will account for approximately £7M worth of expenditure and CO2e emissions of 992 tonnes (based on DEFRA CO2e emission factors for natural gas, 2017).
The chiller system at the JRH
The John Radcliffe Hospital (JRH) is Oxfordshire’s main A&E site. It provides acute medical and surgical services including trauma, intensive care and cardiothoracic services. The site is around 66 acres, and includes the JRH, the children’s Hospital, the Oxford Eye Hospital, the Oxford Heart Centre and the Women’s Centre. The study looked in detail at a £50 m building services upgrade project that was considered by the hospital organisation to be significantly oversized. It was developed across two phases:
Phase 1 (2010–2017): a 4.3 MWe combined heat and power unit (CHP) and a waste heat combination boiler, a single 1.16 MW absorption chiller, heating and chilled water pump systems
Phase 2 (2017 −2018): a further two chiller units (one electric and one absorption chiller), a number of roof mounted heat rejection units (adiabatic radiators) and additional chilled water circulation pumps were installed.
The upgrade of the heating system was scoped beyond the hospital boundaries to include the installation of district heating linkage points for future connection to neighbouring university and residential buildings with the view of enabling a staple income stream to be generated by the hospital organisation via the trading of residual heat (CS3-P5). The boilers can be modulated to adjust to the site thermal demand, but the large capacity still led to high capital expenditure. However, the system uses old steam boilers to provide redundancy, which incur standing losses as seen in the previous case study. The total cost of the entire project was just under the finance borrowing limit of £50 m over which UK Treasury approval is required (CS3-P5).
Figure provides a model overview of the original hospital systems installed in the 1980’s (in light grey), which is partially still serving as a backup system, alongside Phase 1 (in green) and Phase 2 (in blue) systems; original systems that have now been removed are also illustrated (in light grey). The blue arrows illustrate flow and return chilled water distribution from the three chillers (cold water generators) to the main hospital site via three 45 kW chilled water circulation pumps. The red arrows represent flow and return, heat rejection pipework via another set of circulation pumps. The figure illustrates the redundancy designed into the system. It offers substitution redundancy, having an electric and an absorption chiller as well as the adiabatic radiators, which themselves included duplication redundancy.
Figure 3. Modelling of the original and current hospital installations from Jones and Eckert (Citation2020).
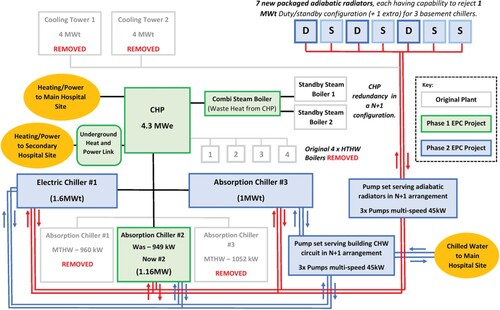
The hospital organisation requirements specification requested that the chilled water upgrade works provide for an N + 1 chilled water supply capacity of 2.5 MW; this brief was the basis of designing the chiller upgrade which informed the ‘Phase 2’ works (CS3-P6). Calculations and observations undertaken by the EPC main contractor suggested that a summer (peak) cooling demand of 900 kW was more appropriate. An uplift of 20% was applied to offset errors in the calculation methodology increasing the summer chilled water demand to 1,080 kW (circa 1 MW). Hence the new Phase 1 absorption chiller rated at 1.16 MW capacity was of sufficient duty to meet the chilling requirement of the site. The huge discrepancy between the hospital specification (brief) and the calculated cooling requirement undertaken by the main EPC contractor was brought to the attention of the hospital organisation for review. However, a decision was made by hospital managers to stay with the 2.5 MW specification as originally requested. As a result, the Phase 2 chiller project increased the installed chilled water capacity by a further 2.6 MW (1 × 1MW absorption chiller + 1 × 1.6 MW electric chiller), providing total chilled water generation capacity of 3.76 MW; a system that is 276% over and above the calculated peak capacity of the site. The various chiller systems work on different solution principles and are intended as back-up systems for each other.
In addition, 7 × 1 MW packaged heat rejection units were installed as part of the ‘Phase 2’ works, a heat rejection capability of 600% over and above the anticipated heat rejection needs of the site. Secondary chilled water systems, such as pumps, valves and pipework are required to accommodate the maximum load capability of the main chiller plant, consequently the margins of overdesign on the secondary systems, can also be considerable.
The main concern in the system design was to deliver enough energy savings; this was achieved by way of the Phase 1 CHP system and the absorption chiller installation. Whilst the main contractor questioned the design specification of the capacity at the outset, the Trust ignored the concerns of the main contractors. The Phase 1 works reduced chilled water generation costs from £70k to £14k per annum. Nobody within the hospital organisation had a full overview or understanding of the Phase 2 project economics and return on investment (ROI) period (CS3-P1, P3, P5). Due to poor base-line energy data, the hospital organisation chose to ‘err on the side of caution’ regarding the Phase 2 client specification, and hence over-estimated hospital cooling demand requirements to ensure equipment installed via the EPC, was not undersized (CS3-P6).
Uncertain operation conditions leading to overdesign
As argued in Section 2, margins are frequently put in place as a response to uncertainties. Many of these uncertainties are endogenous, i.e. arising from factors that are internal to the organisation or the project, however other uncertainties are exogenous far beyond the control of an organisation (de Weck, Eckert, and Clarkson Citation2007).
Endogenous risk from climate change and change in health care provision
HVAC has a pivotal role in preventing hospital-acquired infections (Curtis Citation2008) and infection from airborne diseases (Saran et al. Citation2020), particularly areas such as intensive care units, treatment rooms and operating theatres (Bartley, Olmsted, and Haas Citation2010; Luongo et al. Citation2016).
The need for cooling is increasing dramatically, as higher temperatures will persist for longer and more parts of the hospital will insist on air-conditioning. The climate-shift has already doubled the risk of extreme European heatwaves and overheating results in excess of 2000 deaths annually within the UK (UK Green Building Council Citation2017). Heatwaves also led to an increased number of patients as people are admitted with cardiovascular problems or injuries from collapsing. Whilst natural ventilation is a theoretical possibility, this would require wide ranging change to hospitals and in particular a reversal to a trend towards single rooms instead of open wards (Lomas et al. Citation2012; Short et al. Citation2012b). While climate change is not considered in the day-to-day management of a hospital, it will be considered in the sizing of new hospital building service systems.
I’m not sure, as clinicians we’re acutely aware of something that’s having a detrimental impact on people either through climate or energy issues. … It’s not a debate for us as an institution. I think individuals may be very concerned about it, read about it and take out what evidence they have. It certainly doesn’t play in day-to-day management. (CS2-P9)
Over the medium and long term, the future of healthcare provision is uncertain. The UK like other western countries (see Oksuzyan et al. Citation2020, for an analysis of changing demographic composition of hospital patients in Denmark) has an increasing and aging population. In the short term, this is exacerbated by hospitals not being able to discharge their patients to community care. However, in the long and medium term it is likely that more patients will be treated at home and be monitored remotely and treated through telemedicine connected through the internet of things (Qadri et al. Citation2020). This is likely to lead to fewer, but more ill patients, where the energy demand per patient will drastically increase. In the past decades, energy demand in hospitals has steadily increased through the introduction of additional diagnostic equipment and patient entertainment. However medical devices are also getting smaller and large stationary devices are replaced by small mobile devices. This means that the demand for energy might increase, but it could also go down. The estates personnel are far removed from discussions on the future of healthcare. They rely on the hospital planning documents developed by the Trusts, which are based on the estimates from the clinicians.
Expectations of resilience and reliability
Resilience in the context of hospitals can be seen as:
In the event of anticipated or surprising critical risks, or of slowly emerging threats, NHS systems must ensure the safety of individuals and provide continuity of care. Experienced personnel, along with spare or flexible reserves, redundant systems and/or shared resources, should be available to enable an appropriate response regardless of the form of the threat, or combination of threats … . Hospitals must develop the capability to adapt or reorganise systems, and temporarily or permanently transform, in response to changed or changing conditions. (Garthwaite Citation2017)
This includes short-term resilience for example, against heat waves or increased cooling requirements due to a pandemic; and long-term events, like an aging population, i.e. more admissions, or a larger population.
The management of margins in the context of resilience operates on two related levels:
The ability of the building to function within specified parameters. Where a system, such as heating or cooling, is impacted by some form of previously envisaged disturbance, the system’s resilience is measured by its speed and ability to return to its original state (Dieter and Bacon Citation1976). If unforeseen circumstances push such a system outside these specified parameters, such systems may fail catastrophically (Weick and Sutcliffe Citation2006).
The ability of the overall system to maintain its core functions, i.e. to provide adequate medical care.
In both case studies, resilience was given as a rationale for the size of the system without going into particular details or explaining how a system of that particular size, provides resilience. The hospital organisations wanted to be sure that they had a working system.
so that we were ticking some boxes about if our big boiler went down we had no answer to heating to hundreds of in-patients; so the scheme wasn’t all about efficiency, it was about what we can do to meet these big resilience issues. (CS3-P3)
In a hospital context medical risk overwrites most other considerations and the underlying assumption is that the building services need to continue to operate, no matter what happens. The interviewees did not differentiate between short-term resilience and long-term resilience, even though long-term resilience could also be provided by taking a design option approach and putting the ‘pipework and connections’ in place for later additions to the system.
Organisational issues leading to overdesign
Many of the factors discussed in this section are particular to the NHS context in the UK, where the NHS is on the one hand heavily directed by government through targets and funding, but given little practical help and advice on how to respond to these outside patient care.
Funding
Trusts are not allowed to borrow capital finance on the free market. This leaves them with two options: NHS Capital finance schemes funded by the government, which is often not sufficient to cover large building service system upgrades, such as new energy centres and large refurbishment projects or the use of PFI schemes. The RSUH example illustrates some of the problems that can arise from having an estate that is a mixture of Trust-owned and PFI buildings, as the Trust is contractually required to meet vaguely phrased PFI energy demands, so no explicit baseline was never set.
The availability of government funding for energy efficiency and decarbonisation measures is aligned to government energy targets requiring the NHS to become the world’s first ‘net zero’ national health service by 2040. In 2008 the target was set at 80% of 1990 by 2050, however Bacon (Citation2014) expressed skepticism that is achievable, as the NHS has increased its carbon footprint by 40% since 1990. When energy or health care issues rise to the top of the government agenda, funds for hospital refurbishments or energy projects are made available. This generates a feast and famine mentality within the Trusts.
The boiler system of the JRH Trust is due to a bid to such a funding initiative in 2010, when problems with the old building service system had been accumulating. The Director of Estates commented that previously the funding source just wasn’t there. The backlog maintenance was vast … so breakdowns and then the cost of repair and service of failing kit … (CS3-P3). The maximum available fund was £50 million before Treasury approval was required. The Trust therefore positioned themselves at the price point knowing that the funding opportunity for major building service improvements may not come around again for a long period of time.
Unclear requirements due to inadequate data
Most Trusts use Building Management Systems (BMS) to variable degrees of effectiveness. Theoretically, BMS allow users to quickly analyse data gained through smart metering technologies and space monitoring. In both case study Trusts the available energy consumption data were partial, and the data had not been analysed carefully to predict future energy needs. Both case studies showed a lack of clarity around the requirements. In the RSUH case study, boiler thermal capacity was thought to be based on positioning by PFI engineers and lawyers as the requirements of the new PFI building. Whilst energy data were available for the retained estate, this was not used to establish the capacity requirements. The JRH, by contrast, only had partial energy data and based the sizing of the new systems on existing ones. In both case studies it has not been possible to track down the rationale for the sizing.
The designs started with the capacity values stated in the tender specification documents and design and installation margins were added based on this. A specific study to establish the existing needs and to assess any potential undersizing or oversizing in legacy systems was not undertaken by either Trust prior to project commencement.
Lack of understanding of the system through staff turnaround and subcontracting
Energy management is usually part of the remit of estates management. The Energy Manager is responsible for monitoring the energy consumption of Trusts over many years and understanding the causes of fluctuations and demands arising from different use, and weather patterns. Whilst Estates Managers are well paid, many of their team members are not. This leads to a shortage of skilled Energy Managers staying in post for long periods. Typically, Energy Managers in hospitals are either recent graduates, have worked their way up in non-graduate positions or have entered from the side.
Both case study organisations suffered from a high turnover of staff. Two of the interviewees had recently moved to the RSUH to gain higher positions with slightly different roles, and therefore had little understanding of energy systems on site. At the JRH, the commissioning Estates Director (CS3-P5) had moved to a different Trust and the interim Estates Director (CS3-P3) was only on a temporary contract. His successor also moved on swiftly. This movement of senior staff members within NHS Estates Departments is fairly common, as a move to a different Trust is often the only way to increase salary rates. This high turnover of senior staff members impacts the understanding of a hospital Trust, about its needs, opportunities or strategic direction. Only the commissioning Estate Director (CS3-P5) of the JRH understood the vision as an integrated multi-site system, which also could generate revenue through selling heat. The interim director invited the authors for the case study, because he knew the system was oversized, but had not been able to understand the rationale from talking to his staff; and also, did not attempt to sell off surplus thermal energy.
In addition to a high staff turnover, Trusts have also increasingly relied on consultants supporting estate and energy management. The consultancies, who themselves have high staff fluctuation often operate on fixed-term contracts and are also not able to provide continuity. The number of people who understand the buildings and their performance under different circumstances well, can be extremely limited. Consultants also do not have the power to directly influence how the estate or energy management is handled. This lack of understanding of the performance of the specific hospital, in most stakeholders, was evident in both case study Trusts.
The procurement process
During the procurement process, the fundamental decisions about a system are made when tendering specification is prepared and potential contractors draft their response. This can lock overdesign into a system from the outset.
The procurement and design process
Under-priced bidding was a severe problem in the late 1980s resulting in financial collapse and poor workmanship (Witt and Liias Citation2011). In response the NHS, who as a public organisation needs to be accountable, set up transparent, open, fair and efficient processes for building services procurement. The Carbon Energy Fund (CEF) was created specifically to allow public sector and NHS organisations to access capital finance, compliant procurement support and technical expertise under one umbrella. This removed the need to publicly advertise higher-value projects and allow purchasers to select local firms from a predetermined list of approved contractors. The fee rates are predetermined but are typically more expensive than the lowest price bidding option.
Procurement through tendering
The RSUH went through a public and open tender, while the JRL was part of a framework competition, which launched a number of hospital upgrade projects simultaneously. This framework exempted the projects from the requirement to advertise through the Official Journal of the European Union (OJEU), saving a period of 6–12 months at the beginning of a construction project (Caldwell et al. Citation2005) and allowed them to draw on a limited pool of local suppliers.
The JRH tendered for a main contractor, who designed, built and operated the system. Maintenance of building services infrastructure when delivered as part of an EPC arrangement tends to be carefully monitored and rigorously applied due to the need to protect engineering assets, and to ensure optimum performance and operation of plant and equipment throughout the contract term (Lee, Lam, and Lee Citation2015; Piette et al. Citation2001). There were only a limited number of potential contractors across the UK, who then bid for all the available projects. From these the Trust only received two plausible tenders. The outstanding tender came from a company who are experts in district heating networks and proposed a district heating solution that provided heat and power to two hospitals within the Trust and had the potential to provide heating to neighbouring houses and university facilities. The success of the project is measured by energy savings compared to the performance of the old system. Excess savings over and above those anticipated and bound by contract are shared between the Trust and main contractor. The cost of operating the oversized system lies largely with the Trust.
The contractor is responsible for assuring that the system runs reliably. It is in their interest that back-up systems exist with different solution principles as in the case of the JRH chillers.
Design decisions leading to overdesign
Assumptions based on worst case scenario
The design capacity choices on building service systems tend to be based on worst case scenarios, for example, prolonged cold spells or prolonged heat waves. Within the sizing calculations, it is standard practice not to consider internal heat gains. Heat gains from humans typically equate to 100 W per person (CIBSE Citation2006). Hospital buildings may have in excess of 4000 occupants leading to significant heat gains of 400 kW. Within a hospital, there are many other types of equipment that give out heat, these include office equipment, fridges and energy intensive medical equipment such as MRI Scanners that give off so much heat they need to be permanently cooled. Importantly, none of these human or equipment heat gains appear to be taken into account when heat loss calculations are undertaken, and as a consequence, the peak load that is required by the boilers, is likely to be much less than the design heating load. Natural ventilation for cooling is also rarely taken into consideration forcing the use of mechanical refrigeration. Once a capacity requirement value is decided the designers have multiple choices.
Design choices
A stated requirement stipulates that a system has at least a certain size, but unless explicitly stated does not set an upper limit. The Design Consultant (CS2-P11) stressed that the selection of boilers to meet a certain capacity requirement was very much the choice of the Design Engineer and is rarely specified by the client. The Consultant then went on to explain an example scenario whereby a 1200 kW heating capacity is required, stating that:
two 600 kW boilers could be used, or two 800 kW boilers could be used, or instead two 1,200 kW boilers could be used; all three choices could be correct depending on the application, and how critical it is to maintain the load. As an alternative, three boilers could also be chosen. (CS2-P11)
Most building service applications apply a duplication redundancy ‘N + 1’ strategy, whereby an identical spare system component is kept to step in, in case of need. This enables them to shut one system down in case of failure or maintenance to still operate at the required capacity. At the JRH, the Estates Operational Manager commented.
we’ve always got N + 1 resilience; if you lose your electric you’ve got low temperature fed from the combined heat and power (CHP) unit and you’ve got your steam. If you lose low temperature hot water (LHHW), you’ve got your electric and your high temperature hot water (HTHW). If you lose your high temperature, you’ve got electric and low temperature. (CS3-P1)
To provide an example of possible boiler sizing, various boiler selection scenarios to meet a requirement of 12 MW, which corresponds to a like-with-like replacement of the RSUH boiler system prior to the upgrade, which opted for a design option similar to the 3 boiler C option (see Figure ) are illustrated in Figure . The capability of the installation as a percentage of the design boiler load within the selected examples, varies from 100% to 200%, a ‘worst-case’ capacity excess of 100%. The example uses a same-size boiler, which is not strictly necessary but is often a preferred option to simplify maintenance.
Once the size and selection of boilers are chosen, there is then the issue of choosing a specific product from a specific manufacturer. For example:
Assuming the choice is three 800 kW boilers when a specific product is chosen, it might be that a manufacturer only produces a boiler of 750 kW and the next size up is 900 kW; in this scenario, the installer has to choose the three 900 kW boilers. (CS2-P11)
The operating conditions also need to be considered during design.
The design information should be given to the manufacturer to allow them to produce a specific technical quotation for the particular design conditions that are required on that project. [Otherwise] … this can cause oversizing of the installed boiler capacity (CS2-P11).
Margin markup
The chartered institute of building services engineers (CIBSE) and the building services research and information association (BSRIA) have published various guidance documents that provide suggested margin values for use during building service design and installation (BSRIA Citation2008; CIBSE Citation1998, Citation2006, Citation2012). Similar guidelines exist from ASHRAE (Citation2008) but have not been looked at for this study. Within these documents, margin values are very often not stated explicitly but buried within the guidance text, and range between 5% and 25%. Margins that allow for uncertainties within the initial design and data assumptions – within client specifications, may also be necessary (e.g. assumptions relating to future demand, occupant numbers, etc.). A range of safety and precautionary margins are also added for numerous reasons (e.g. to allow for equipment power surges on start-up or excess pressure on piped systems), but also to provide an element of flexibility during commissioning which allows for variations in system and equipment performance and to provide reliability and resilience due to operational ‘wear-and-tear’. Consequential margins such as installers uplift – ‘next size up’ may also be applied due to limitations associated with the availability or production of optimum-sized equipment. A review of boiler sizes amongst a selection of manufacturers shows that choosing the next size up can account for a margin of about 15% typically (Opus Citation1996). Using the maximum margin percentage values taken from CIBSE and BSRIA guidance documents and using a nominal base capacity requirement of 100 units, Table illustrates how the accumulation of margins across various categories can result in a significant capacity increase to 313 units (Jones and Eckert Citation2017).
The case studies illustrated that these margins are applied, however it has not been possible to track the individual margins on particular systems.
The RSUH boiler system has roughly twice the capacity of that stipulated by the PFI provider. A similar value would also be reached if all the margin categories illustrated in Table had been applied to a more sensible estimate of the sites need, based on actual use. It has not been possible to establish which logic the design process followed, as the rationale for the sizing was not recorded. It would have been very interesting to study a live project to ascertain the scope and size of margins applied.
Table 5. Cumulative margins for ‘worst case’ impact on capacity from various CIBSE guidance documents.
Discussion
The case studies have shown that understanding and avoiding margins is a systemic problem. Multiple factors contributed to overdesign in each of the case studies. Some can be directly attributed to margins while others are deeply connected to the way the projects, and the design within them, was carried out. In the case studies individuals added margins to mitigate their own risks, such as the various markups during the design and installation process or the operator benefitting from a system with huge redundancy. The complexity of the systems were such that nobody in the organisations had an overview of the margins and the rationale for why they were added. One of the contributing factors in both cases was that the hospital involved legacy systems that had evolved over long periods of time, so that records were incomplete, and no individual followed the system throughout its life-cycle. To improve the management of margins it is important to recognise this systematic character. Only then can dialogue across different stakeholders lead to sensible-sized margins; and a design that maximises system reliability, whilst minimising the level of margin applied.
Margins and their adverse impact on design and efficiency are largely a hidden problem. People do not recognise margins and therefore are not aware of the problems of overdesign. Many staff members of the JRH were extremely happy with the energy savings their huge boiler system enabled them to make compared to the system it replaced without out seeing the additional costs, for example in terms of the steam boiler standing losses and the increased capital expenditure and maintenance costs associated with the Phase 2 chiller system. There was remarkably little awareness of the markup ensued by the use of standard parts. Here the organisation would benefit gratefully from greater awareness of margins and a language that enables them to describe their oversized systems. Maybe the largest source of margins is markup, whereby each actor along the process adds between 10 and 30%; here a markup register would be really beneficial. Whilst an overall joint margin might also be technically straight forward, this raises the complex issue of the legal responsibility for system failures, or loss of functionality.
In the case study Trusts, the margins were also caused by the way the design and procurement processes were managed. One of the key factors was the low level of understanding the Trust had about their own requirements. They had only partial data of their current use but made little effort to analyse the data carefully or attempt to predict their future use. Data capture in hospitals can be very difficult, because old buildings often only have limited usage sensors and sub-metering. However, the hospitals made little effort to capture the data specially for the commissioning of the systems. The sizing requirements were almost set causally, based on the capacity of the existing system with an element of markup. In the JRH the main contractor challenged the sizing specification, even though an oversized system reduced the contractors risks, but the Trust dismissed these concerns. There had generally been little communication across the different stakeholders about the issue of margins. This is in part due to the contractual arrangements with a multitude of different contractors for particular roles and little oversight by the Trusts. This might be less of an issue for simpler building types or situations where the owner or operator is involved more directly, across all stages. In particular, the different stakeholders did not discuss the implications of the fundamental design decision in detail, with each other. For example, in both case studies the systems had complex redundancy components, with multiple identical parts in each. These components, such as pumps and heat rejection units might be easier to maintain, but could also incur considerable additional cost.
There were a number of factors that arose out of the specific NHS context. The NHS has a feast and fame mentality towards building projects; grabbing finance when it becomes available is a rational response to a very uneven funding mechanism. Due to the stringent, but somewhat unobtainable energy-saving targets set by Government, the NHS hospitals were very focused on demonstrating energy savings compared to inefficient past systems, and overlooked the fact that energy savings can hide overdesign. In NHS hospitals overdesign is often justified under the heading of resilience. Overdesigned systems can provide resilience, but this also incurs costs and inefficiencies. The hospitals were unwilling to break resilience down into specific issues or at least distinguish between short-term or long-term resilience events. For long-term events, such as climate change or an ageing population an options approach (De Neufville et al. Citation2004) would be sensible where additional systems can be brought in without disrupting the operations and infrastructure that is already in place. For short-term events and in case of system failure an element of redundancy is required.
The hospitals had duplication redundancy but could take a more modular approach where smaller systems are switched on and off as required. There was little willingness to look beyond the system boundaries to back-up systems. For example, electric space heaters can be used to provide local heating in critical areas when centralised heating systems fail, patients can also be given cooling fans or local DX cooling systems can be installed in critical areas such as operating theatres to provide resilience in the event of centralised chiller failure.
Conclusions
The paper has established that the cost and extent of overdesign in building services can be very high. Even overdesign by several hundred percent can be hidden. The problem has a multitude of different causes ranging from markups stipulated by industry guidance to cultural and organisational issues. The problem is escalated by organisational staff turn around and lack of communication between different stakeholders, so that rationale is lost. Excessive margins are a systemic issue and need to be handled as such. Margins cannot and should not be fully avoided, as margins can provide protection against unexpected events, however a greater awareness and better management of margins would enable organisations to set margins deliberately.
A major limitation of the paper is that it is based on two case studies within the NHS context. In each of the case studies, significant data and rationale is missing. While this fact is illuminating in its own right, it would have been beneficial to understand the rationale behind key decisions and observe how and when margins are added deliberately. Looking at the sociotechnical aspects of margins would be very difficult in a quantitative way; however it would be interesting to conduct a survey of different hospitals and other building types to see how wide spread the problem is.
In future work, the authors are hoping to study the sizing of building projects as they unfold, so that they either observe decision-making or question the participants once decisions manifest. The research wants to raise awareness of margins to a broad range of building service stakeholders. The aim is also to develop a toolkit for establishing the right size of a building service system. It would be very interesting to apply a scenario-based simulation approach to establish suitable margins, however this would need to be prototyped in similar building types, such as the air conditioning systems studied by Djunaedy et al. (Citation2011). From a design perspective, an in-depth study of how principles of flexibility and modularity could be used to manage margins would provide some useful insights.
References
- Abhang, Sanket. 2020. “Over-Engineering: What are the Negative Effects? Nearby Engineers”. Accessed June 23, 2023. https://www.ny-engineers.com/blog/negative-effects-of-over-engineering.
- Adamou, Marios, Niki Kyriakidou, and Jon Connolly. 2021. “Evolution of Public-Private Partnership: The UK Perspective Through a Case Study Approach.” International Journal of Organizational Analysis 29 (6): 1455–1466. https://doi.org/10.1108/IJOA-08-2020-2397.
- Allen, Jeffrey D., Phillip D. Stevenson, Christopher A. Mattson, and Nile W. Hatch. 2019. “Over-design Versus Redesign as a Response to Future Requirements.” Journal of Mechanical Design 141 (3): 031101. https://doi.org/10.1115/1.4042335.
- Almefelt, Lars. 2005. “Balancing Properties While Synthesising a Product Concept – A Method Highlighting Synergies.” 15th International Conference on Engineering Design, Melbourne, Australia, 15.18.2005.
- American Society of Heating, Refrigerating and Air-Conditioning Engineers (ASHRAE). 2008. “ASHRAE HANDBOOK – Heating, Ventilating, and Air-Conditioning Systems and Equipment”, SI Edition. 1791 Tullie Circle, N.E., Atlanta, GA 30329. ISBN 978-1-933742-34-2. ISSN 1930-7705.
- Bacon, Matthew. 2014. “Occupancy Analytics: A new Basis for low-Energy–low-Carbon Hospital Design and Operation in the UK.” Architectural Engineering and Design Management 10 (1–2): 146–163. https://doi.org/10.1080/17452007.2013.837254.
- Banerjee, Prithviraj, and Olivier L. de Weck. 2004. “Flexibility Strategy-Valuing Flexible Product Options.” ICSE Conference on Synergy Between Systems Engineering and Project Management. Las Vegas, NV, INCOSE.
- Bartley, Judene M., Russell N. Olmsted, and Janet Haas. 2010. “Current Views of Health Care Design and Construction: Practical Implications for Safer, Cleaner Environments.” American Journal of Infection Control 38 (5): S1–S12. https://doi.org/10.1016/j.ajic.2010.04.195.
- Brahma, Arindam, Scott Ferguson, Claudia Eckert, and Ola Isaksson. 2023. “Margins in Design–Review of Related Concepts and Methods.” Journal of Engineering Design, 1–34. https://doi.org/10.1080/09544828.2023.2225842.
- Brahma, Arindam, and David C. Wynn. 2020. “Margin Value Method for Engineering Design Improvement.” Research in Engineering Design 31 (3): 353–381. https://doi.org/10.1007/s00163-020-00335-8.
- Brahma, A., D. C. Wynn, and O. Isaksson. 2022. “Use of Margin to Absorb Variation in Design Specifications: An Analysis Using the Margin Value Method.” Proceedings of the Design Society 2: 323–332. https://doi.org/10.1017/pds.2022.34.
- Braun, Virginia, and Victoria Clarke. 2012. “Thematic Analysis.” In APA Handbook of Research Methods in Psychology, Vol. 2. Research Designs: Quantitative, Qualitative, Neuropsychological, and Biological, edited by Harris Cooper, Paul M. Camic, Debra L. Long, A. T. Panter, Ed Rindskopf David, and Kenneth J. Sher, 57–71. Washington, DC: American Psychological Association. https://doi.org/10.1037/13620-004.
- Building Services Research and Information Association (BSRIA). 2008. The BSRIA Blue Book. Berkshire.: Old Bracknell Lane West Bracknell.
- Butler, Steven. 2020. “Sizing the Opportunity (Dynamic Modelling – Right-sizing HVAC)”. CIBSE – Chartered Institution of Building Services Engineers. Accessed June 23, 2023. http://portfolio.cpl.co.uk/CIBSE/202003/52/.
- Caldwell, Nigel, Helen Walker, Christine Harland, Louise Knight, Jurong Zheng, and Tim Wakeley. 2005. “Promoting Competitive Markets: The Role of Public Procurement.” Journal of Purchasing and Supply Management 11 (5–6): 242–251. https://doi.org/10.1016/j.pursup.2005.12.002.
- Carbon Trust. 2010. “Hospitals: Healthy Budgets Through Energy Efficiency.” June 2010. CTV024v2 Queen’s Printer and Controller of HMSO.
- Chan, Edwin HW, and Cutson Liu. 2007. “Corporate Portals as Extranet Support for the Construction Industry in Hong Kong and Nearby Regions of China.” Journal of Information Technology in Construction 12: 181–192. https://www.itcon.org/2007/12
- Chartered Institute of Building Service Engineers. 1986. Volume A – Building Environmental Engineering, Design Data. ISBN: 0900953292. London: CIBSE.
- Chartered Institute of Building Service Engineers. 1998. “Engineering Design Calculations and the Use of Margins.” Extracts from Research Report 4.
- Chartered Institution of Building Services Engineers. 2006. “Guide A – Environmental Design.” January 2006 7th ed. CIBSE London. ISBN-10: 1-903287-66-9.
- Chartered Institution of Building Services Engineers. 2012. “Inspection of Air Conditioning Systems: A Guide to EPBD Compliance.” 2nd ed. February 2012 CIBSE London. ISBN 978-1-906846-20-6.
- Chen, Chih-Chun, and Nathan Crilly. 2014. “Modularity, Redundancy and Degeneracy: Cross-Domain Perspectives on Key Design Principles.” 2014 IEEE International Systems Conference Proceedings. 546–553. IEEE.
- Christel, Michael G., and Kyo C. Kang. 1992. “Issues in Requirements Elicitation. Requirements Engineering Project.” Software Engineering Institute, Carnegie Mellon University Pittsburgh, Pennsylvania. 15213. Technical Report CMU/SEI-92-TR-012 ESC-TR-92-012.
- Curtis, L. T. 2008. “Prevention of Hospital-Acquired Infections: Review of non-Pharmacological Interventions.” Journal of Hospital Infection 69 (3): 204–219. https://doi.org/10.1016/j.jhin.2008.03.018.
- De Neufville, Richard, Olivier De Weck, Daniel Frey, Daniel Hastings, Richard Larson, David Simchi-Levi, Kenneth Oye, Annalisa Weigel, and Roy Welsch. 2004. “Uncertainty Management for Engineering Systems Planning and Design.” In Engineering Systems Symposium. Cambridge, MA: MIT.
- Department of Health. 2015. NHS Energy Efficiency Fund, Final Report Summary. February 2015.
- De Weck, Olivier, Claudia M. Eckert, and P. John Clarkson. 2007. “A Classification of Uncertainty for Early Product and System Design.” DS 42: Proceedings of ICED 2007, the 16th International Conference on Engineering Design, 159–171. Paris, France, 28.−31.07.
- Dieter, George Ellwood, and David Bacon. 1976. Mechanical Metallurgy. Vol. 3. New York: McGraw-hill.
- Djunaedy, Ery, Kevin Van den Wymelenberg, Brad Acker, and Harshana Thimmana. 2011. “Oversizing of HVAC System: Signatures and Penalties.” Energy and Buildings 43 (2–3): 468–475. https://doi.org/10.1016/j.enbuild.2010.10.011.
- Dong, Bing, Da Yan, Zhaoxuan Li, Yuan Jin, Xiaohang Feng, and Hannah Fontenot. 2018. “Modeling Occupancy and Behavior for Better Building Design and Operation – A Critical Review.” Building Simulation 11 (5): 899–921. Springer Berlin Heidelberg https://doi.org/10.1007/s12273-018-0452-x
- Eckert, Claudia, P. John Clarkson, and Winfried Zanker. 2004. “Change and Customisation in Complex Engineering Domains.” Research in Engineering Design 15 (1): 1–21. https://doi.org/10.1007/s00163-003-0031-7
- Eckert, Claudia, Chris Earl, Safaa Lebjioui, and Ola Isaksson. 2013. “Components Margins Through the Product Lifecycle.” Product Lifecycle Management for Society: 10th IFIP WG 5.1 International Conference, PLM 2013, Nantes, France, July 6–10, 2013, Proceedings 10, pp. 39–47. Berlin, Heidelberg: Springer
- Eckert, Claudia, Ola Isaksson, and Chris Earl. 2019. “Design Margins: A Hidden Issue in Industry.” Design Science 5: e9. https://doi.org/10.1017/dsj.2019.7.
- Eckert, Claudia, Ola Isaksson, Safaa Lebjioui, Christopher F. Earl, and Stefan Edlund. 2020. “Design Margins in Industrial Practice.” Design Science 6: e30. https://doi.org/10.1017/dsj.2020.19.
- Elsayed, Elsayed. A. 2012. Reliability Engineering. Hoboken, NJ: John Wiley.
- Gaffney, Declan, Allyson M. Pollock, David Price, and Jean Shaoul. 1999. “NHS Capital Expenditure and the Private Finance Initiative – Expansion or Contraction?” Bmj 319 (7201): 48–51. https://doi.org/10.1136/bmj.319.7201.48.
- Garthwaite, P. 2017. “Resilient Hospital Refurbishment.” PhD thesis. School of Engineering and Innovation, Faculty of Science, Technology, Engineering and Mathematics. The Open University.
- Graham, C. I. 2016. “High-Performance HVAC (Online). Whole Building Design Guide.” Accessed June 23, 2023, https://www.wbdg.org/resources/high-performance-hvac.
- Hendrick, R. L., M. J. Witte, N. P. Leslie, and W. W. Bassett. 1992. “Furnace Sizing Criteria for Energy-Efficient Setback Strategies.” ASHRAE Transactions 98 (part 1): 1239–1246. Lawrence Race G, BSRIA, Parand F, BRE, Engineering Design Margins, CIBSE Research Report RR04 1997.
- Hull, Elizabeth., Ken Jackson, and Jeremy Dick. 2005. Requirements Engineering. 2nd Ed. London.: Springer.
- Isaksson, Ola, Peter Lindroth, and Claudia M. Eckert. 2014. “Optimisation of Products Versus Optimisation of Product Platforms: An Engineering Change Margin Perspective.” DS 77: Proceedings of the DESIGN 2014 13th International Design Conference.
- Jones, Darren. 2022. “Strategic Energy Management Within Hospitals: Barriers to Energy Efficiency and the Impact of Design Margins.” PhD thesis. The Open University. https://doi.org/10.21954/ou.ro.000151b9.
- Jones, Darren, and Claudia Eckert. 2017. “Overdesign in Building Services: The Hidden Energy Use.” 21st International Conference on Engineering Design, 21–25 Aug 2017. Vancouver, Canada.
- Jones, Darren, and Claudia Eckert. 2020. “Managing Margins: Overdesign in Hospital Building Services.” In Proceedings of the Design Society: DESIGN Conference, Vol. 1, 215–224. Cambridge University Press. doi:10.1017/dsd.2020.151.
- Kamara, John M., and Chimay J. Anumba. 2000. “Development of a Prototype Software for CRP.” Computing in Civil and Building Engineering, 550–557. https://doi.org/10.1061/40513(279)72.
- Kanno, Yoshihiro, and Yakov Ben-Haim. 2011. “Redundancy and Robustness, or When is Redundancy Redundant?” Journal of Structural Engineering 137 (9): 935–945. https://doi.org/10.1061/(ASCE)ST.1943-541X.0000416.
- Kenna, Erica, and Paul Bannister. 2009 July. “Simple, Fully Featured Boiler Loop Modelling.” 11th Conference of International Building Performance Simulation Association, 428–433.
- Kindfund. 2023. Accessed May 24, 2023. https://www.kingsfund.org.uk/audio-video/key-facts-figures-nhs.
- Lee, P., P. T. I. Lam, and Wai Ling Lee. 2015. “Risks in Energy Performance Contracting (EPC) Projects.” Energy and Buildings 92: 116–127. https://doi.org/10.1016/j.enbuild.2015.01.054.
- Lomas, Kevin J., Renganathan Giridharan, C. Alan Short, and A. J. Fair. 2012. “Resilience of ‘Nightingale’ Hospital Wards in a Changing Climate.” Building Services Engineering Research and Technology 33 (1): 81–103. https://doi.org/10.1177/0143624411432012.
- Luke, Jenny, Richard C. Franklin, Joanne Dyson, and Peter Aitken. 2023. “Building Toward a Disaster Resilient Health System: A Study of Hospital Resilience.” Disaster Medicine and Public Health Preparedness 17: e219. https://doi.org/10.1017/dmp.2022.204.
- Luongo, Julia C., Kevin P. Fennelly, Julia A. Keen, Zhiqiang John Zhai, Byron W. Jones, and Shelly L. Miller. 2016. “Role of Mechanical Ventilation in the Airborne Transmission of Infectious Agents in Buildings.” Indoor air 26 (5): 666–678. https://doi.org/10.1111/ina.12267.
- Nguyen, Nga, Faida Mhenni, and Jean-Yves Choley. 2016. “Redundancy Handling with Model-Based Systems Engineering.” 26th European Safety and Reliability Conference (ESREL).
- Oksuzyan, Anna, Andreas Höhn, Jacob Krabbe Pedersen, Roland Rau, Rune Lindahl-Jacobsen, and Kaare Christensen. 2020. “Preparing for the Future: The Changing Demographic Composition of Hospital Patients in Denmark Between 2013 and 2050.” PLoS One 15 (9): e0238912. https://doi.org/10.1371/journal.pone.0238912.
- Otto, Kevin, Katja Hölttä-Otto, Timothy W. Simpson, Dieter Krause, Sebastian Ripperda, and Seung Ki Moon. 2016. “Global Views on Modular Design Research: Linking Alternative Methods to Support Modular Product Family Concept Development.” Journal of Mechanical Design 138 (7): 071101. https://doi.org/10.1115/1.4033654.
- Opus 96 (1996) Building Services Publications Ltd., 1996.
- Pahl, Gerhard, and Wolfgang Beitz. 1996. Engineering Design: Systematic Approach. London: Springer-Verlag.
- Pérez-Lombard, Luis, José Ortiz, and Christine Pout. 2008. “A Review on Buildings Energy Consumption Information.” Energy and Buildings 40 (3): 394–398. https://doi.org/10.1016/j.enbuild.2007.03.007.
- Piette, John D., Morris Weinberger, Frederic B. Kraemer, and Stephen J. McPhee. 2001. “Impact of Automated Calls with Nurse Follow-up on Diabetes Treatment Outcomes in a Department of Veterans Affairs Health Care System: A Randomized Controlled Trial.” Diabetes Care 24 (2): 202–208. https://doi.org/10.2337/diacare.24.2.202.
- Qadri, Yazdan Ahmad, Ali Nauman, Yousaf Bin Zikria, Athanasios V. Vasilakos, and Sung Won Kim. 2020. The Future of Healthcare Internet of Things: A Survey of Emerging Technologies. 2020.” IEEE Communications Surveys & Tutorials 22 (2): 1121–1167. https://doi.org/10.1109/COMST.2020.2973314.
- Saran, Sai, Mohan Gurjar, Arvind Baronia, Vijayalakshmi Sivapurapu, Pralay S. Ghosh, Gautham M. Raju, and Indubala Maurya. 2020. “Heating, Ventilation and Air Conditioning (HVAC) in Intensive Care Unit.” Critical Care 24 (1): 1–11. https://doi.org/10.1186/s13054-019-2683-3.
- Short, C. A., K. J. Lomas, G. Renganathan, and A. J. Fair. 2012b. “Building Resilience to Overheating Into 1960’s UK Hospital Buildings Within the Constraint of the National Carbon Reduction Target: Adaptive Strategies.” Building and Environment 55: 73–95. https://doi.org/10.1016/j.buildenv.2012.02.031
- Soliman, Mohamed Ahmed. 2019. “Adverse Effect of Oversizing Upstream Gas Oil Separation Plants on Energy Consumption.” International Petroleum Technology Conference. OnePetro.
- Sun, Yuming, Li Gu, C. F. Jeff Wu, and Godfried Augenbroe. 2014. “Exploring HVAC System Sizing Under Uncertainty.” Energy and Buildings 81: 243–252. https://doi.org/10.1016/j.enbuild.2014.06.026.
- Tackett, Morgan WP, Christopher A. Mattson, and Scott M. Ferguson. 2014. “A Model for Quantifying System Evolvability Based on Excess and Capacity.” Journal of Mechanical Design 136 (5): 051002. https://doi.org/10.1115/1.4026648.
- Thompson, Graham. 1999. Improving Maintainability and Reliability Through Design. Chichester: John Wiley & Sons Incorporated.
- UK Green Building Council. 2017. “Climate Change – UKGBC’s Vision for a Sustainable Built Environment is One that Mitigates and Adapts to Climate Change.” Accessed June 23, 2023. https://www.ukgbc.org/climate-change/ UK Green Building Council (UKGBC).
- UK National Statistics. 2020. “Department for Business, Energy & Industrial Strategy”, UK energy in brief 2020. Accessed June 23, 2021. https://assets.publishing.service.gov.uk/government/uploads/system/uploads/attachment_data/file/904503/UK_Energy_in_Brief_2020.pdf.
- Weick, Karl E., and Kathleen M. Sutcliffe. 2006. “Mindfulness and the Quality of Organizational Attention.” Organization Science 17 (4): 514–524. https://doi.org/10.1287/orsc.1060.0196.
- Witt, Emlyn, and Roode Liias. 2011. “Comparing Risk Transfers Under Different Procurement Arrangements.” International Journal of Strategic Property Management 15 (2): 173–188. https://doi.org/10.3846/1648715X.2011.582750.
- Yin, Robert K. 2009. Case Study Research: Design and Methods. Vol. 5. Thousand Oaks, CA: Sage.
- Young, Ralph Rowland. 2004. The Requirements Engineering Handbook. Boston, MA: Artech House.
- Yu, Tit Wan, and Hon Wan Edwin Chan. 2010. “Requirements Management in the Architecture, Engineering and Construction (AEC) Industry: The Way Forward.” In CIB World Congress. Salford. http://hdl.handle.net/10397/37384.
- Zielczynski, Peter. 2008. Requirements Management Using IBM Rational Requisite Pro. Upper Saddle River, NJ: IBM Press, Pearson plc.