Abstract
For more than a century, ionizing radiation has been indispensable mainly in medicine and industry. Radiation research is a multidisciplinary field that investigates radiation effects. Radiation research was very active in the mid- to late 20th century, but has then faced challenges, during which time funding has fluctuated widely. Here we review historical changes in funding situations in the field of radiation research, particularly in Canada, European Union countries, Japan, South Korea, and the US. We also provide a brief overview of the current situations in education and training in this field. A better understanding of the biological consequences of radiation exposure is becoming more important with increasing public concerns on radiation risks and other radiation literacy. Continued funding for radiation research is needed, and education and training in this field are also important.
Introduction
Since the discovery of X-rays in 1895, ionizing radiation (hereinafter referred to as radiation) has been indispensable in medicine and industry. Radiation represents a multi-edged sword: e.g. as a modality to diagnose and treat diseases, as a cause of cancer and non-cancer diseases, and as a tool to induce DNA damage in life science experiments (Hamada Citation2014). Radiation research is a multidisciplinary field of research encompassing physics, chemistry, biology, epidemiology, and medicine to study the effects of ionizing radiation in humans and non-human biota (in its broadest sense, radiation research further includes studies on non-ionizing radiation and heat). Radiation research has a history of about 120 years but has been the subject of intensive study in various countries since the early 1950s. Such a surge of interest in radiation research was also manifested as establishment of various relevant scientific journals, e.g. Radiation Research in 1954, International Journal of Radiation Biology and Related Studies in Physics, Chemistry and Medicine in 1959 [renamed International Journal of Radiation Biology (IJRB) in 1988, also known as a ‘blue’ journal in the radiation research community], Journal of Radiation Research in 1960, and Radiation and Environmental Biophysics in 1963 (c.f. Strahlentherapie und Onkologie in 1912). Later, other journals were also established, e.g. International Journal of Radiation Oncology•Biology•Physics (‘red’ journal) and Journal of Radiation Protection and Research both in 1976, Radiotherapy and Oncology (‘green’ journal) in 1984, International Journal of Low Radiation and International Journal of Radiation Research both in 2003, and Journal of Radiation Research and Applied Sciences in 2008.
No research can be carried out without funding, and research funding is critically important in advancing any research field. Research funding situations reflect not only the activity and quality of research but also social needs. Research funding availability has fluctuated widely over time, and a variety of events may stimulate a resurgence of interest in radiation research (and perhaps trigger concomitant increases in its funding as well), such as, but not limited to, development of new therapeutic modalities (e.g. with neutrons, protons, heavy ions, and intensity modulation) and new diagnostic modalities (e.g. computed tomography, and tomosynthesis), occurrence of nuclear accidents (e.g. Three Mile Island in March 1979, Chernobyl in April 1986, and Fukushima in March 2011), and the new or updated recommendations in radiation protection [e.g. an occupational dose limit significantly reduced for the lens of the eye and circulatory effects first listed as a radiation health hazard, both recommended in 2011 by the International Commission on Radiological Protection (ICRP) (ICRP Citation2012)]. Over the past decade or two, the radiation research community has nevertheless faced challenges in the available workforce, education, training, and funding in many countries (Coleman et al. Citation2003; NCRP Citation2015; Kirsch et al. Citation2018).
Taking the opportunity of celebrating 60 years of IJRB, this paper reviews historical changes in funding situations in the field of radiation research, and also provides a brief overview of education and training opportunities, particularly in Canada, European Union (EU) countries, Japan, the Republic of Korea (South Korea), and the US. lists the information on timing of calendar year and fiscal year for funding, currency, and currency rate compared with US dollar (USD) in these countries.
Table 1. Timing of calendar year and fiscal year for funding, currency, and currency rate compared with US dollar (USD).
Funding in Canada
Past (1890s–2007)
Early years (1898–1930s)
The history of Canadian radiation research and its funding began in 1898 when Ernest Rutherford became a professor at McGill University in Montréal, Canada. His salary, studies on radioactivity, and the construction of the Macdonald Physics Building (where his studies were conducted) were supported by Sir William Christopher Macdonald (a prominent Canadian entrepreneur and philanthropist). From the very beginning, funding of Canadian radiation research had, therefore, a strong private not-for-profit component.
Until the 1920s, the vast majority of Canadian radiation research was driven by medical applications of radioactivity (Richards Citation1915), and therefore the funding streams of radiation research were tightly connected to the funding of hospitals and medical centers. A large portion of that funding then came from private donors, fundraising campaigns, and municipalities, as was the case for Rutherford at McGill. This is exemplified by the history of the Toronto Hospital and associated medical centers and clinics (Godfrey Citation1979). A private donation of Canadian dollars (CAD) 0.1 million (M) allowed the construction of a new building for the headquarters of the National Sanitarium Association equipped with X-ray diagnostic machines in 1910s (Write et al. Citation1915). Driven by the enthusiasm of many medical practitioners (e.g. Gilbert P. Girdwood, George S. Ryerson, and Gordon Richards), medical radiology became a branch of medicine in hospitals and academic educational programs in Toronto, Montréal, Ottawa and Vancouver.
A period of 1890s–1920s in Canada can broadly be called the era of ‘discovery and integration into medicine’, closely following a similar period in the world history of radiation research (Slater Citation2012). By providing generous funding, Canadian provinces contributed substantially to the transition to the next era of ‘exploration and maturation’ during 1920s–1945 (Hayter Citation1998).
One of the key events during the era of exploration and maturation was the acknowledgment of the usefulness of radiotherapy for cancer by the Canadian Royal Commission on the Use of X-Rays and Radium in the Treatment of the Sick sponsored by Ontario (Cody Citation1932). Recognizing the outstanding progress made in the use of radioactivity in medicine and the need for further research into better understanding of its biological effects, the Federal Government of Canada subsequently joined the efforts and initiated its own funding streams for radiation research. Thus, the Ministry of Health subsidized establishment of several new radiation therapy clinics and purchases of radium, leading to the establishment of Medical Radiology in Canada in 1933 (Medical Radiology in Canada Citation1933). Around that time, two major branches of radiation biology were formed that still exist today: radiation therapy and radiation protection. Whereas the former deals with high dose radiation in search of most efficient ways of killing cancer cells, the latter aims at understanding the biological effects of low dose radiation in normal cells and associated human health risks (, top panel in 1895–1930s). Acknowledging these two branches of radiation research is important here, because qualitative and quantitative differences in the structure of their funding become more evident with time, as discussed below.
Figure 1. Longitudinal trends in funding landscape for radiation research in Canada. Key events/developments affecting funding streams or shift in scientific interest are shown at the bottom of the diagram. *, the landscape reflects qualitative, not quantitative, assessment of funding sources relative to each other; no trends for absolute amounts were assessed. **, the spike reflects a CAD 50 M investment from the CANDU Owners Group toward the construction of the Biological Research Facility for low-dose radiation biological studies at Chalk River Laboratories.
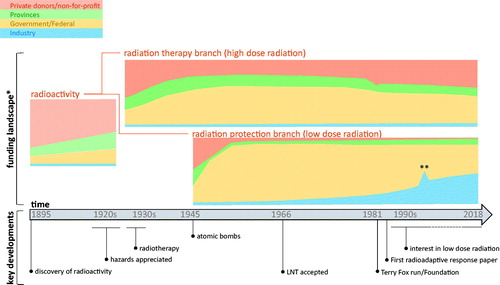
Origins and development of radiation protection research in Canada (1940s–2007)
Circumstances related to the Second World War led Canada to develop a radiation protection research program. In 1942, the British and Canadian governments reached a historic agreement to relocate the Cambridge-based nuclear laboratory to Montréal (relocated to Chalk River, Ontario, in 1945) to continue developing nuclear weapons in close collaboration with the US and away from Europe engulfed in war (Eggleston and Irwin Citation1965). The laboratory later became associated with the US Manhattan Project and the nuclear weapons program of the Allies. This required substantial federal funding, not only for nuclear engineering and weapon developments but also for related radiation protection issues. In fact, the very first set of internationally agreed radiation protection standards was developed in Chalk River during the 1949 conference (the first of the series of Tripartite Conferences on Radiation Protection Standards) organized by the Manhattan Project partners (Taylor Citation1983). The first federally funded studies of the mutagenic effects of radiation at Chalk River Laboratories started in 1948 (Newcombe Citation1948). Soon, radiation protection research in Canada centered around Chalk River Laboratories under various federal funding streams, albeit with very limited contribution from other sources (). Strikingly, the radiation biology program at Chalk River Laboratories is still in operation today, substantially leveraged by funding from industry, making it the world’s longest radiation protection research program (Wang et al. Citation2019).
Historically, research in Chalk River at the Atomic Energy of Canada Limited (AECL) has been connected with nuclear industry, via the CANDU reactor technologies. Subsequently, the radiation protection research program at AECL was supported by the CANDU Owners Group Inc (COG), and such support has undergone a steady growth since 1980s (). Importantly, in the late 1990s, COG co-funded the construction of a new animal facility specifically designed for large-scale low-dose radiation studies (Wang et al. Citation2019). This globally unique facility called the biological research facility (BRF) further facilitated low-dose radiation biology studies in Canada, attracting national and international partners and collaborators.
Radiation therapy research in Canada (1940s–2007)
In contrast to Canadian radiation protection research that was centered around the building of nuclear reactors, initially under some war-related secrecy and based in essentially one laboratory in Chalk River, radiation therapy research became closely tied with medical and academic institutions. Research funding in this area in Canada started to shape into what is currently known as grant-based science in the 1940s when the Canadian Society for the Control of Cancer [renamed into Canadian Cancer Society (CCS) in 1946] was formed. The government-sponsored CCS research program begun in 1945 and primarily focused on basic research. A substantial contribution to this early program came from an annual grant from the Canadian Medical Association based on the interest of the King George V Silver Jubilee Fund. However, federal funding streams have grown quickly to match those from charities. In 1936, the National Research Council Associated Committee on Medical Research (NRC-ACMR) began its operation as a health science promoting and science funding institution in Canada. After the Second World War was over, health sciences flourished with an active support from the NRC-ACMR. Radiation oncology research followed this general trend of active growth. Radiation therapy has always been recognized as an important part of cancer research, and its funding can, therefore, be considered within the context of funding cancer research in general. The NRC-ACMR, renamed Medical Research Council of Canada (MRC) in 1962, eventually became the principal federal government agency that funds health research. Although several major not-for-profit cancer organizations (e.g. CCS, Cancer Research Society, Canadian Breast Cancer Foundation, and Canadian Prostate Cancer Foundation) have funded radiation oncology studies from those early years, their contribution was eventually outweighed by the MRC. The landscape of funding in this area maintained its shape until 1980 when Terry Fox, a cancer patient with an amputated leg, embarked on a Marathon of Hope to raise awareness among Canadians of the importance of finding a cure for cancer (Steensma et al. Citation2014). He could not complete his run due to his worsening conditions, but Terry’s Marathon of Hope gave an indelible impression not only to Canadians but also to people around the world. By 1981 when he died, his dream of raising one dollar for every Canadian was accomplished – the Terry Fox Marathon of Hope fund collected CAD 24.17 M. Since then, the Terry Fox Foundation has become one of the largest not-for-profit cancer research funding organization in Canada. Since 1981, such organizations collectively contribute substantial proportion to overall radiation research funding in Canada ().
Table 2. Major radiation research grant-based funding institutions in Canada and respective recent past (1999–2007) and present (2008–2018) funding allocations.
In 2000, the MRC of Canada was replaced by a new organization, the Canadian Institutes of Health Research (CIHR). CIHR further increased overall grant funding, improving the international competitiveness of Canada. shows the level of funding for radiation research from CIHR and three other major institutions in Canada.
Present (2008–2018)
As in the past, the separation between the areas of radiation protection and radiation therapy has been maintained. After a relative decline in the 2000s and in the early 2010s, the interest of researchers toward biological effects of low dose radiation started to grow again. This was partially driven not only by advances in various ‘omics areas allowing systemic analyses of cellular and organismal responses but also by technical advances permitting measurement of subtle changes after low-dose exposures. A new generation of researchers started to join AECL in Chalk River from 2008, expanding AECL’s radiation protection research program. After AECL was reorganized into the Canadian Nuclear Laboratories (CNL) in 2014 (as a government-owned company-operated organization), the radiation biology program was diversified further to include therapeutic applications. It is estimated that the federal base funding level of the radiation biology program doubled between 2008 and 2018 to reach CAD ∼8 M/year. The COG also supports relevant research carried out at CNL at an estimated CAD of ∼1 M/year. Bruce Power (the Ontario utility company, part of the COG) maintains its own funding of radiation biology research through supporting Industrial Research Chairs for university researchers, operating grants on low-dose research at Northern Ontario School of Medicine and co-funding the Bruce Power Centre for Health, Environment and Radiation (Thome et al. Citation2017), and its overall contribution is estimated at a total of CAD >1 M/year since 2016.
An example of a successful province-funded research initiative is the Sylvia Fedoruk Canadian Centre for Nuclear Innovation in Saskatchewan that started its operation in 2011. Some of its research focus areas (e.g. nuclear medical technologies, radiopharmaceutical applications and health risks of radiation exposure) overlap with the radiation life science discussed here. Therefore, their funding contributes to the overall funding landscape presented in .
With respect to grant-based radiation research, funding comes from various granting institutions with open financial information. Quantitative assessment of funding levels is presented in for the four major Canadian granting agencies. A total annual budget for radiation research increased between the past (defined herein as 1999–2007) and the present (2008–2018) by about 50% from CAD 9.8 M to 14.7 M. Interestingly, federal, but not private charity funds, contributed to this increase. Data obtained for the Terry Fox Foundation and the CCS also indicate that grants related to radiation research constitute 10–15% of overall cancer research grants: c.f. 30–38% of cancer patients receiving radiotherapy in Canada (Lievens et al. Citation2017). Overall, these data suggest a positive trend towards the increasing funding for radiation research in Canada.
Future (2019–)
The Canadian government has proven its commitment to support science. The 2018 budget saw an unprecedented increase of CAD ∼4 billion in new funding for science over the next 5 years (Owens Citation2018). A substantial portion of this increase will go to the major funding Canadian Institutions, including Natural Sciences and Engineering Research Council of Canada (NSERC) and CIHR discussed above. It is feasible to expect that this new budget will further increase funding of radiation life sciences through university-based research in Canada. Arguably, the use of radiation in treating cancer may be entering a new era after the demonstration of the enhancement of the immune checkpoint therapy by radiation (Gong et al. Citation2018); however, the promising combination of the two modalities that may one day become a new standard of treatment of a large number of cancer patients requires substantial research efforts. It will not be surprising if some of the new funding available to Canadian researchers will be directed toward this area. Another promising and quickly developing radiation therapy area is targeted alpha therapy. The area of radiopharmaceutical research is linked to pharmaceutical industry, as well as to national nuclear facilities (e.g. nuclear research reactors, synchrotrons and accelerators). As such, these areas of radiation research will see a substantial combined contribution from pharmaceutical companies, provinces and federal government.
A sustainable human society, climate and environment all depend on reliable and clean energy production. It has been suggested that nuclear power generation appears to be indispensable for low carbon future on Earth. The radiation protection system, therefore, needs to evolve from the current hypothesis-based system (e.g. the use of a linear-non-threshold hypothesis) to a more knowledge-based system. Generation of such knowledge should be the goal of future studies in low-dose radiation biology. Public perception of radiation is also a psychosocial factor that requires scientific understanding. Following these needs, in 2018, the COG initiated a strategic research program on low-dose research and public perception of low-dose risks. This program will be undertaken in collaboration between the CNL, the Universities of Saskatchewan and Regina Centre for the Study of Science and Innovation Policy (CSIP) Johnson Shoyama Graduate School of Public Policy, the University of Ontario Institute of Technology, and the University of Ottawa. The initial budget of the program is CAD > 1 M, which is also open to additional funding and collaborations.
Lastly, the future of Canadian radiation research should have a strong international collaboration component. Such momentum was already established in January 2018 when Canadian representatives of the CNL low-dose radiation biology research program met with their colleagues representing similar programs and platforms from Europe, Japan and US at the International Workshop on Biological Effects of Radiation ‘bridging the gap between radiobiology and medical use of ionizing radiation’ held in Osaka, Japan. In the resulting joint statement, gaps in the current knowledge and research activities were identified, and recommendations for enhancing international cooperation were made (Weiss and Yonekura Citation2019). Delegations from more countries and organizations joined another round of discussions at the Scoping Meeting on Global Coordination of Low-Dose Research, which was held on 17–18 September 2018 in Milan, Italy under the auspices of the Organisation for Economic Co-operation and Development/Nuclear Energy Agency/Committee on Radiological Protection and Public Health (OECD/NEA/CRPPH). It is hoped that the discussion will result in coordinated efforts both in research and in obtaining new funding for research.
In conclusion, Canada has a rich legacy of radiation research that has been maintained throughout the past and to the present. Canadian radiation research owes for its success not only to talented and devoted people but also to the diverse sources of funding. They range from generous individual philanthropists to not-for-profit foundations to federal government institutions. Industries and provinces have also played important roles. It is expected that radiation research and associated funding in Canada will continue to grow in the years to come and will further contribute to the accumulation of knowledge and its application in various areas of human activities around the world.
Funding in EU countries
The Euratom program is the Research and Training (R&T) Program implemented by the European Commission (EC), under the provisions of the European Atomic Energy Community (Euratom) Treaty, in which all EU Member States participate. In Europe, the Euratom R&T Programs have been the main source of funding for radiation protection related topics such as radiation biology, epidemiology, radioecology, dosimetry, nuclear and radiological emergency preparedness, and medical use of radiation. An overview of the funding of key research areas during the past framework programs is given below. The present framework program called Horizon 2020 is still ongoing, and only some tentative information can be given regarding the distribution of funding. As for the future framework program starting in 2021, it is expected that the funding for radiation protection research will continue. Overall, radiation protection is a fairly small fraction of research supported from the Euratom program on nuclear fission where majority of funding is directed to the nuclear safety and waste management. EC also supports research on radiation metrology as part of the European Metrology Programme for Innovation and Research (EMPIR).
Past (1994–2013)
Basic information on the funding of European radiation research is available from EC’s CORDIS (Community Research and Development Information Service) website (https://cordis.europa.eu/projects/home_en.html). A report on radiation protection related EU funded projects in Euratom framework programs 4–7 (referred hereafter to as FP4–FP7) was assembled in the OPERRA (Open Project for the European Research Area) project as part of Task 2.2 on determining joint funding mechanisms for national and EU Fission research and development (R&D) programs. This report is summarized here to show the overall trends in funding. There were altogether 259 projects funded from FP4–FP7 during 1994–2013 in the radiation protection area, with a total EC contribution of EUR 255.8 M.
In recent years, European research platforms have been set up in Europe for different fields of radiation protection research. These research platforms are strategic alliances of organizations such as national funding bodies, research institutes and universities that have created joint Strategic Research Agendas (SRAs) in particular research areas. In order to follow the trends in research funding, the projects were grouped by the OPERRA project according to their research areas. The projects related to the platforms MELODI (low dose risk), ALLIANCE (radioecology), NERIS (nuclear and radiological emergency preparedness and response) and EURADOS (dosimetry), as well as medical applications (more recently organized as EURAMED), were grouped accordingly. below shows the trends over time both in topics and the number of projects. shows the overall EC funding per target platform (research area), broken down by framework programs (based on CORDIS).
Table 3. Number of Euratom research and technological development (RTD) projects grouped by field of research platforms from FP4 to FP7.
Table 4. Percentage of overall EC funding per target platform, broken down by framework programs (based on CORDIS); for FP4 as far as information could be retrieved.
It can be seen from and that the number of projects declined over the different framework programs, while the overall EC contribution increased. This reflects a trend to larger projects and new funding instruments, such as Integrated Projects adopted in FP6 and Networks of Excellence in FP7. The percentage of funding, as shown in , increased for MELODI related projects on low dose effects. The decrease for ALLIANCE (radioecology) and EURADOS (dosimetry) related projects may be due to the fact that within FP4 a large proportion of efforts was dedicated to collaboration with countries of the former Soviet Union, particularly related to the Chernobyl accident and to the situation in the Southern Urals. The consequences of the Chernobyl accident may well explain the relatively high proportion of funding going into NERIS related projects in FP5 and FP6 at the turn of the century.
Present (2014–2020)
The current Euratom work program (WP) implementing the Euratom R&T program (2014–2018) (‘Euratom program’) runs from 2014 to 2018 plus 2 years Euratom extension (2019–2020), the funding regime and the rules for participation being the same as those for Horizon 2020. Projects are funded through the Euratom program, not through Horizon 2020. The objective of the Euratom program is to pursue nuclear R&T activities with an emphasis on continuously improving nuclear safety and radiation protection.
A novelty in the current WP is the newly implemented funding tool, the European Joint Program (EJP). In the area of radiation protection research, the first and only EJP is the CONCERT (European Joint Program for the Integration of Radiation Protection Research) project launched in 2015. This project was granted with a maximum EU grant amount of EUR 19.8 M attributed to the action in topic NFRP 7-2015: ‘Integrating radiation research in the European Union’ of the NFRP–2014–2015 call.
CONCERT is a co-fund action designed to support coordinated European funds from the Commission and national research funding from EU Member States. It aims at attracting and pooling a critical mass of national resources on objectives and challenges of Horizon 2020 and at achieving significant economies of scale by adding related Horizon 2020 resources to a joint effort. By increasing the available financial capacity of radiation protection research, adjusting national research programs, and fostering synergistic effects, there will likely be a benefit of radiation protection research in Europe. Furthermore a joint effort is performed with a strategic perspective on supporting excellent science, on building and maintaining high competence in radiation science and radiation protection as well as further promoting integrative and multidisciplinary research on a European level, i.e. by initiating and funding concerted joint research actions, promoting access to research infrastructures and to optimize the radiation protection related education and training activities across Europe. All activities build on the SRAs of the five platforms MELODI, ALLIANCE, NERIS and EURADOS as well as medical applications (EURAMED).
CONCERT organized two major open research and technological development (RTD) calls to strengthen the scientific research in strategic priority areas of radiation protection defined by the five European radiation protection research platforms. CONCERT committed EUR 17.1 M (10.5 M in Call 1 and 6.6 M in Call 2) funding for the open research calls. Nine projects have been selected.
In the call NFRP-2016-2017, the action NFRP 9 – Impacts of low dose radiation exposure resulted in the funding of the MEDIRAD (Implications of Medical Low Dose Radiation Exposure) project with a maximal EU grant of EUR 10 M. MEDIRAD’s main goal is to better understand the implications of medical low dose radiation exposure in order to provide the scientific bases to improve the practice of radiation protection in the medical field. The three main activities of the project will be (1) to improve organ dose estimation, (2) to evaluate the effects of low to moderate doses of radiation, with a focus on cardiovascular disease as a result of radiotherapy and cancer risk as a result of computed tomography (CT) scans, and (3) to formulate a series of evidence-based recommendations to improve radiation protection of patients, medical workers and general public. The project will also produce recommendations that are expected to influence the SRA on future radiation protection research in Europe. The project will last for four years, and is supported by the five European medical associations (EANM, EFOMP, EFRS, ESR, and ESTRO) and EURAMED, as well as the main European platforms of radiation research (MELODI and EURADOS).
The Work Program 2018 is the last Work Program for the current Euratom program, but later in 2018, the EC intends to present a proposal for the extension of the program for the years 2019–2020. Within the WP 2018, there are two topics related to radiation protection. Within CONCERT, no extra funding was foreseen for a 3rd call for scientific projects in the EURATOM WP 2018: therefore, the topic of interest to radiation protection research is action NFRP-2018-08. This action seeks close cooperation with and complements actions of CONCERT and MEDIRAD, and must take into account prioritization of research in the field reflected in the SRAs of the radiation protection research platforms MELODI, ALLIANCE, NERIS, EURADOS, and EURAMED. In addition, the scope of projects answering the call should be based on a ‘gap analysis’, as this will be considered during the evaluation. CONCERT EJP together with the well-known European radiation protection research platforms and in close collaboration with MEDIRAD organized a joint gap analysis of the radiation protection research priorities of the platforms based on their SRAs on one side and on projects funded or co-funded by EURATOM in FP7 and H2020 projects or programs on the other. The maximum contribution to this topic is EUR 7 M.
The second topic in the radiation protection section of WP 2018 is NFRP-2018-9 – Strategy for the exploitation of research results funded under Euratom R&T Programs in the field of radiation protection. The objective is to provide a systematic assessment of the use of research results from the past Euratom FP6 and FP7 radiation protection projects by various stakeholders and to propose recommendations for future research policy in this field. It is a Coordination and Support Action (CSA), with a maximum funding of EUR 0.5 M.
Future (2021–2027)
The next Euratom framework program is expected in 2021–2025. The EC’s staff working document Annex ‘Impact assessment of the Euratom Research and Training program 2021–2025’ provides some information on the expected impacts of the coming program (EC Citation2018). This impact assessment accompanies the Commission’s proposal for the Euratom research and training program for 2021–2025 (Euratom program). In turn, the program complements the ‘Horizon Europe’ Framework Program for Research and Innovation in the area of nuclear research and training. On 2 May 2018, EC adopted its proposals for a new Multiannual Financial Framework (MFF) for 2021–2027. Under these proposals, the Euratom programs will have a budget of EUR 2400 M over this period. The impact assessment report reflects the decisions of the MFF proposals and focuses on the changes and policy choices, which are specific to this instrument. The Euratom program is one of the spending programs that will implement the EC’s vision for the period beyond 2020. Bearing in mind the lessons learned and progress achieved so far, the impact assessment will look at whether the existing program should continue with its present form or undergo changes to its scope and structure. Radiation protection and ionizing radiation applications are among the proposed challenges.
Funding in Japan
In Japan, radiation research started in 1954. Its trigger was an incident that occurred in March 1954 where Japanese fishermen were exposed to radioactive fallout from a nuclear weapons test at Bikini Atoll. The National Institute of Radiological Sciences (NIRS) was established in 1957. Laboratories for radiation biology and basic radiation medicine started being established in various schools of medicine according to the recommendation by the Science Council of Japan (SCJ) in 1958. Following the atomic bombings of Hiroshima and Nagasaki in 1945, the Atomic Bomb Casualty Commission (ABCC) was established in 1947 [renamed Radiation Effects Research Foundation (RERF) in 1975], the Institute for Nuclear Radiation Research was established in Hiroshima University in 1958 (renamed Research Institute for Radiation Biology and Medicine in 1961), and the Atomic Bomb Disease Institute was established in Nagasaki University in 1962. The Japan Radiation Research Society was established in 1959, and the Japan Health Physics Society (JHPS) was established in 1961. Kyoto University Radiation Biology Center was established in 1976. The Central Research Institute of Electric Power Industry (CRIEPI) started radiation biology studies in 1987, and the Institute for Environmental Sciences (IES) was established in 1990.
Radiation biology research in Japan has been conducted by universities as well as specialized national, public and private research institutes. At universities, research strongly depends on competitive funds, whereas direct grants from the government cover administrative expenses including basic employment costs. In national or public research institutes, activities more strongly depend on direct governmental grants, with competitive funds as supplementary sources, at least until recently. The total national budget for science and technology grew very fast at over the 10% annual increase during 1970–1980, followed by a slightly slowed annual growth of 5% continuing from late 1980s to 2000 (NISTEP Citation2013). The deteriorated financial situation of the government then stopped the growth, with a total amount of slightly over Japanese yen (JPY) 3500 billion maintained (NISTEP Citation2013). Below, a brief history and perspectives on funding systems related to radiation research are presented.
Past (1950–2010)
Grant-in-aid for Scientific Research
Grant-in-Aid for Scientific Research (also called KAKEN) is a major research grant of the Ministry of Education, Culture, Sports, Science and Technology (MEXT) of Japan, covering all fields of science ranging from humanities and social sciences to natural sciences. It is a competitive research fund of a bottom-up nature, aiming at promoting academic research based on researchers’ unfettered interest. Herein, research fields were categorized with a unique system, and proposals were reviewed by peer scientists in a specific field. Classification of radiation research has moved from ‘radiation biology’ (in 1972–1992, under a category of ‘multidisciplinary fields’) through ‘environmental effects assessment (including radiation biology)’ (in 1993–2002, under ‘environmental sciences’) to ‘risk sciences of radiation/chemicals’ (in 2003–2017, under the same category) (NII Citation2018). Investment in these three fields showed an increasing trend, reaching JPY ∼800 M, until mid-2000s (NII Citation2018) (). This partly mirrors the steady growth of the budget for the total science and technology (NISTEP Citation2013), but the share of the above fields also increased from 0.26% to 0.46% during 1996–2010 (NII Citation2018).
Figure 2. A trend in the total amount of Grants-in-aid for Scientific Research in the fields related to radiation biology. Funds for research subjects under the following fields are summed: ‘radiobiology’ (1972–1992), ‘environmental effects assessment (including radiobiology)’ (1993–2002), and ‘risk sciences of radiation/chemicals’ (2003–2012). Created from data in NII (Citation2018).
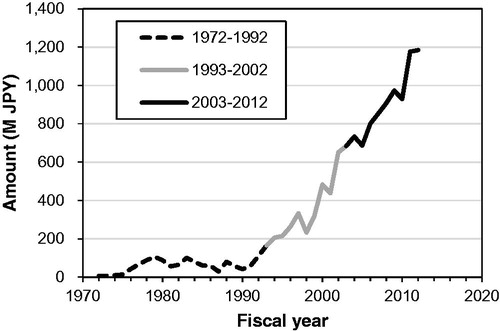
Collaborative nuclear energy research
Peaceful use of nuclear energy has been a central energy policy of Japan. Based on a long-term plan developed in 1987, the government started the ‘Nuclear Energy Fundamentals Crossover Research’ (hereafter referred to as ‘Crossover Research’), which was available in 1989–2008. ‘Crossover Research’ was a competitive funding system aiming at investing in collaborative research on fundamental and innovative R&D on relevant research areas that were indicated in a top-down manner by the Japan Atomic Energy Commission (Kuniya Citation1990). Relevant fields of research included health and environmental effects of radiation, utilization of radiation and laser technologies, materials science, artificial intelligence, and computational science (AEC Citation2003). The budget was handled first by the Science and Technology Agency (STA), and then by MEXT. The total budget in 1990 was JPY 905 M, of which 119 M (13%) was spent on radiation effects research (Kuniya Citation1990). The total amount later showed a markedly decreasing trend (e.g. total amount reduced to JPY 360 M in 2003) (AEC Citation2003).
RERF
RERF is a public corporation for scientific research on health effects of atomic bomb radiation, covering epidemiology, statistics and related basic studies. In 1947, ABCC was established with funding from the US Atomic Energy Commission. In 1975, ABCC was restructured into RERF, since when its research has been intergovernmental funded in full partnership between Japan and US (RERF Citation2018). The total amount of grants doubled during 1975–1996 (from JPY ∼2 to 4 billion), equally covered by Japan and US. Since 1997, although most parts of the activities have equally been covered by Japan and the US, some parts have been funded solely by Japan, and the total amount showed a decreasing trend during 1997–2010 (from JPY 4.0 to 3.5 billion).
NIRS
NIRS, founded in 1957, is a specialized national research institute for radiological sciences under a supervision of first STA and then MEXT. The direct governmental grant, which is the major funding source of NIRS, increased exponentially from 1957 to late 1990s (NIRS Citation1977, Citation1987, Citation2018) (). Reflecting the deterioration of the government’s fiscal health, the trend subsequently changed to a steady decrease (∼2.4% annual reduction during 2001–2010) (NIRS Citation2018) (). Radiation safety research had been a minor part (e.g. JPY ∼0.3 billion in 1995), with a half of the budget dedicated to medical application research (e.g. JPY ∼5 billion in 1995) excluding the employment cost (NIRS Citation1995) ().
Figure 3. Governmental investment on NIRS. (A) Trends in the annual budget of grants for administrative expense (orange) and facilities grant (grey). Data are from NIRS (Citation1977, Citation1987, Citation2018) and its annual project plans. Breakdown of annual budgets of grants for administrative expense in million (M) JPY in 1995 (B) and 2015 (C). (B) Nuclear energy fundamental research, safety analysis research and about two third of the radiological priority research were related to radiation safety research and radiobiology. (C) Employment expenses are included in each project in C. Data taken from NIRS (Citation1995, Citation2018).
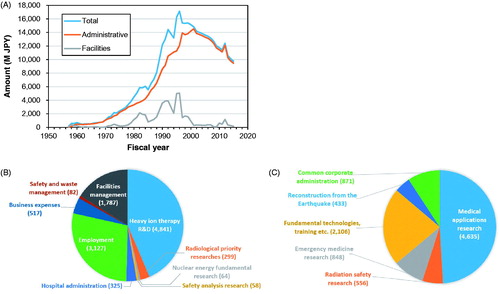
CRIEPI
CRIEPI was founded in 1951 as a cooperative research institute of Japanese electric power companies, which explores all fields of scientific research and technology related with electricity industry. CRIEPI is an incorporated foundation mainly supported by electric power companies.
CRIEPI started radiation biology studies in 1987 when the focus of the studies was placed on the hormetic effects of radiation. In 1998, a long-term low dose rate irradiation facility was constructed to study biological effects of low dose-rate γ irradiation in cultured cells and mice. In 2000, Low Dose Research Center was established. In 2007, direction of research was changed to obtain scientific evidence to improve risk assessment for radiation protection, when Low Dose Research Center was reorganized into Radiation Safety Research Center (RSC) gathering researchers in the field of radiation biology, radiation measurement, and waste management. The main aim of biological research in RSC has been to elucidate the biological mechanisms of adverse health effects following low dose rate exposures.
IES
IES, founded in 1990, is a public corporation for research on environmental effects of radioactive substances and health effects of low dose radiation. IES has two main funding sources: one is a basic grant from the Aomori prefecture for development and operation of facilities (which originates in the grant of MEXT), the other being grants for commissioned projects on specific research subjects. There were two periods of intensive investment (). Prefectural grant was invested during 1992–1997 in construction of the Low-Dose Radiation Effects Research Facility (LERF), a unique facility in which large populations of specific-pathogen-free mice are continuously exposed to low dose rate γ rays (Braga-Tanaka et al. Citation2018). Then, during 2001–2007, Advanced Molecular Bio-Science Research Center (AMBIC) was constructed at the expense of the prefectural grant, aiming at promoting cellular and molecular biological research. Excluding these periods, the total amount of investment in radiation biology showed an increasing trend, reaching JPY ∼1 billion in 2010 ().
Present (2010–2018)
Two key items of background information necessary to understand the recent (2010s) situation in Japan are the even worsened financial situation and the influence of the Fukushima Daiichi Nuclear Power Plant (FDNPP) accident in March 2011.
Grants-in-aid for Scientific Research
The field of ‘risk sciences of radiation/chemicals’ existed as of 2017, and investment in this field has been increasing during the past decade (NII Citation2018) (). This growth is of special note because the total amount of science and technology budget in Japan has been stable since 2000 (NISTEP Citation2013).
Collaborative nuclear energy research
In 2008, ‘Crossover Research’ was restructured into ‘Strategic Research Initiative in the Foundation of Nuclear Power’ (hereinafter, ‘Nuclear Initiative’). ‘Nuclear Initiative’ is a competitive funding system for proposals on several topics that directly meet the government’s nuclear energy policy (NSRA Citation2018a). Nuclear engineering represented its major part, with radiation life sciences being minor. Proposals were accepted during 2008–2013 in one of four categories: (1) strategic collaborative research (funded for 3 years), (2) hot laboratory utility (for 3 years), (3) young researchers’ study (for 2 years), and (4) foundation in restoration from the accident (for 3 years, started after the FDNPP accident). The accident also led to restructuring of ‘Nuclear Initiative’ into ‘Nuclear Energy Science and Technology and Human Resource Development Project through Concentrating Wisdom’ (hereafter, ‘Project Wisdom’) in 2014, which funds (1) strategic collaborative research and (2) nuclear-decommissioning research (NSRA Citation2018a). The total annual investment in life science was JPY ∼100 M during 2006–2014, based on the analysis of open data (NSRA Citation2018a).
Nuclear Regulation Authority (NRA)
NRA is a governmental organization established in 2012 by separating various regulatory departments from those related to operational issues. NRA invests in the Japanese Epidemiology Study on Low-Dose Radiation Effects (J-EPISODE), a large-scale follow-up of nuclear workers, conducted by Radiation Effects Association (REA) since 1990 (Kudo et al. Citation2018), which was formerly funded by MEXT. The budget for 2016 was JPY 171 M (REA Citation2016). In 2017, NRA started a project of Radiation Safety Research Promotion Fund with two arms. One arm of the project invests JPY 50 M (in 2018) in proposals of radiation safety and regulation research on several specified subjects; proposals are funded for 5 years or shorter, several proposals being accepted annually (NRA Citation2018). The other arm of the project invests JPY 30 M (in 2017) in formation of network for radiation protection research (NRA Citation2018). Proposals must be intimately related to improvement in the relevant law system rather than basic research.
Ministry of the Environment (MOE)
Before the FNDPP accident, regulation of radioactive substances was exempted from the environmental policy by the Basic Environment Act. In 2012, this provision was deleted, and assessment of radiation health effects has become under the jurisdiction of MOE. This prompted MOE to start a project called ‘the Research on the Health Effects of Radiation’ (hereafter, the MOE project) in 2012. The project invests in researches regarding (1) dose assessment techniques, (2) biological effects of radiation, (3) countermeasures against anxiety in affected areas, (4) assessment of initial radiation dose of the accident, and (5) the morbidity trend in the affected areas (MOE Citation2018a). This project accepts 5–10 new proposals every year, each funded for at most 3 years with a typical upper limit of JPY 10 M/year (including overhead expenses). The budget for the project shows an increasing trend until 2016 () (based on open information such as in MOE Citation2018b). Since 2017, some of the accepted proposals have additionally been supported by another competitive funding for employment of young researchers.
RERF
During 2010–2017, the total amount of budget from US and Japanese governments showed small fluctuation, a part of which is due to that of currency exchange rate, with a steady trend around the average of JPY 3.4 billion.
NIRS and National Institutes for Quantum and Radiological Science and Technology (QST)
The FDNPP accident greatly influenced the administration of NIRS. First, NIRS started projects to support restoration of the affected area, including through low dose radiation effect research, funded by the Special Account Budget for Reconstruction from the Great East Japan Earthquake (2012–2017) (, ‘Reconstruction from the Earthquake’). Second, NRA joined in its supervision (i.e. MEXT and NRA now jointly supervise NIRS), with the grants for administrative expenses provided only by MEXT. Third, restructuring of Japan Atomic Energy Agency (JAEA) resulted in integration of NIRS with four research institutes of JAEA, giving birth to a new national corporation QST in 2016. During 2010–2015, the direct governmental grant for administrative expenses steadily decreased from JPY 11.4 to 9.4 billion, with ∼4.1% annual reduction (NIRS Citation2018). The share for radiation safety research in 2015 was small (JPY ∼0.6 billion including employment) in the total of JPY 9.4 billion, where medical application research accounts for JPY 4.6 billion (NIRS Citation2018) ().
CRIEPI
As of 31 March 2018, among 653 researchers in CRIEPI, 15 researchers (2.3%) belong to RSC. The three major areas of research conducted in RSC are radiation biology, radiation risk assessment, and waste management, and seven researchers are directly involved in radiation biology studies. The information on the budget for individual in-house projects in CRIEPI is not open, but shows that the institutional ordinary benefit received from Japanese electric power companies decreased after the FDNPP accident, and remains nearly constant over the past few years.
Figure 6. Temporal changes in the institutional ordinary benefit received from Japanese electric power companies during fiscal years FY 2009 through 2017. The data were replotted from CRIEPI (Citation2018).
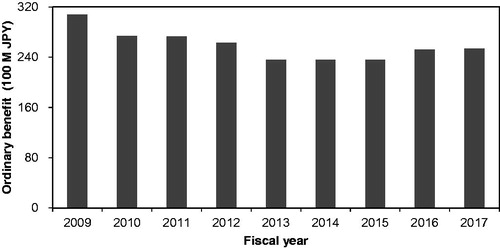
IES
The total amount of resources invested in radiation biology research has annually been JPY ∼1 billion in the last decade and shows a steady trend ().
Future
Grants-in-aid for Scientific Research
The classification of research fields was completely renewed in 2018, in which a basic section of ‘radiation influence-related’ exists under a section of ‘environmental analyses and evaluation and related fields’ (these English section names according to MEXT’s translation) (NII Citation2018). Thus, funding on radiation effects research appears likely to continue in the near future. However, as the new review system attempts to minimize excessive segmentalization, research proposals in radiation biology will be more likely subjected to competition with those from other fields in environmental sciences.
Collaborative nuclear energy research
Regarding ‘Project Wisdom’ of MEXT, several 3-year researches sequentially started during 2015–2017 (NSRA Citation2018a), implying that funding will continue at least for a few years. In 2018, the Project moved from MEXT to JAEA with minor restructuring, including reduction in the annual budget per research and recommencing of young researchers’ research (NSRA Citation2018b). There is, however, no optimistic prospect on whether life science researches will be funded as they have been over the last several years.
MOE
The MOE project has accepted nine new research projects for 2018, and there are still many ongoing projects (MOE Citation2018c). As such, the funding is expected to continue for at least 3 years in the near future.
RERF
As administration of RERF is based on intergovernmental agreement which is renewed every 5 years, the stable trend may continue at least until the current agreement finishes. The situation thereafter may be influenced by the policy of both governments.
QST (NIRS)
After the restructuring of NIRS as part of QST, it is becoming difficult to predict the future trend in the resource invested in radiation safety research. The steadily decreasing trend in the direct governmental grant will continue, likely leading to stronger dependence on competitive funding. There is a prospect that funding on life science at QST will shift towards ‘quantum life science’. ‘Quantum life science’ integrates quantum physics and related technologies, with an expectation that radiation biology therein will become ever more multidisciplinary.
CRIEPI
CRIEPI decides their own long-term roadmap for in-house research, in communication with electric power industry and other related organizations. Current projects aiming to improve the risk assessment for radiological protection will hopefully be continued for another decade, unless any drastic change in the management environment in the electric power industry occurs.
IES
Considering the recent steady trend in the total amount of funding for radiation biology researches at IES, there is an optimistic view that the trend will continue for a next decade.
Planning and Acting Network for Low Dose Radiation Research (PLANET)
Although Japan has many funding systems related to radiation research as aforementioned, these systems have different political contexts. The diversity in the purposes of investment makes it difficult to develop a strategic research plan from broader perspectives. The success in the strategic promotion of low dose radiation research in the framework of MELODI motivated Japanese radiation researchers to establish a similar platform. To this end, QST set up preparatory (2016) and the administrative (2017–) committees for PLANET. PLANET is a platform to promote low dose radiation research, which aims to identify priorities of research needs and propose support system for cooperation and collaborative researches in Japan. PLANET also intends to establish all-Japan network among regulators, academia, research institutes and stakeholders. This cross-sectoral network also aims to work together with international organizations.
Funding in South Korea
Past (1980–2011)
In South Korea, there had been a little interest or effort in radiation research until Kori Unit 1 (the first commercial nuclear power plant in South Korea) started its operation in 1978, though TRIGA Mark-II went into operation in 1962 as the first research reactor. Until 1980s, radiation research had been carried out on a relatively small scale laboratory at Korea Atomic Energy Research Institute (KAERI).
During the last several decades, the Ministry of Science and ICT (Information Communication and Technology) (MIST) of South Korea has been the source of national research grant for the advancement of science and technology in various fields including radiation biology and agricultural applications of radiation as part of pure basic science under the 21st century national R&D initiative of ‘expanding investment in R&D with global competitiveness’.
The Korean government has increased its R&D by an annual average of 12% over the last decade. However, it should be noted that successful commercialization of R&D achievements is rather low and has been found to be about 20% in terms of the amount of the investment due to many reasons such as the lack of partnership between the public and private sector and/or among academia, national research institutes and industry; isolated research by a national research institute or academia that ignores the current market situations; and an overly complex, rigid and ineffective R&D management and assessment system.
Present (2012–2016)
The total R&D budget for the area of radiation biology and agricultural applications of radiation in 2012 was Korean won (KRW) 800 M. It was then 3487 M in 2013, 3899 M in 2014, 2819 M in 2015, and 3715 M in 2016, all in KRW. It may be noted that the R&D investment in the area of radiation biology and agricultural applications of radiation increased sharply for about four times higher from 2012 to 2013, but since then, it stays about at a similar level until 2016.
The number of R&D projects for the area of radiation biology and agricultural applications of radiation in 2012 was 3, which was then 9 in 2013, 8 in 2014, 9 in 2015, and 10 in 2016. The number of papers published in peer-reviewed, Science Citation Index (SCI)-indexed international journals in the area of radiation biology and agricultural applications of radiation was 21 in 2014, 18 in 2015, and 19 in 2016.
Studies in Radiation Health Institute (RHI)
In 1996, RHI was established by Korea Hydro & Nuclear Power Co. (KHNP), with a vision of becoming world’s top radiation health professional institute by focusing its activities on radiation health-related research including the effects of low dose radiation on the human body, and started studies on the low dose radiation safety and radiation epidemiology.
RHI initially conducted a dose assessment study to measure the exposure dose to people that could be caused by radiological accident, and began the low dose radiation studies in the 2000s. In 2005, after constructing the first Korean low dose radiation irradiation facility, the research focus was shifted to the genetic and hormetic effects of low dose radiation.
The goal of the low dose radiation safety research is to promote a correct understanding of low dose radiation through the scientific results on the human health effects of low dose radiation. Low dose radiation safety research is being conducted in three main areas. The first area studies the biological defense effects of low dose radiation against various reactions in the body such as cell repair system, immune response, aging, and cancer using cells and animal model (mouse and Drosophila) systems. The second area assesses chromosomal aberrations in radiation workers at nuclear power plants (NPPs) to apply the results from the cellular and animal model to humans. The third area develops the technology for establishing the standard curves for biodosimetry of South Koreans against a potential radiological emergency accident and for assessing dose using biomaterials (e.g. lymphocytes and tooth enamel). Research objectives have so far been ensured through publications of many papers in SCI-indexed journals and patents, and have obtained biological response curves as a function of radiation dose.
The low dose radiation study involves 6 employees and 17 contract researchers, and currently spends about KRW 1300 M/year for research. In order to better understand the biological response to low dose radiation, future projects include a study on the correlation with low dose radiation and diseases, development of low dose biomarkers, and the biological effect study of NPP workers.
Radiation epidemiology research is conducted largely on three areas to assess the health effects of radiation exposure from NPPs, in both NPP workers and residents nearby NPPs. The first area is epidemiological studies in NPP workers by periodically investigating cancer incidence and mortality among those who have conducted radiation work at NPPs. Since RHI worked on a research project organized by Korean government (MEST, a predecessor of MIST) for the epidemiological survey on the NPP workers, RHI has initiated and conducted its own tasks of assessing cancer risk, by expanding the cohort of NPP workers and promoting joint research with other institutes to secure internal and external reliability and reproducibility. Currently, the cohort of Korean NPP workers has 56,598 members based on the dosimetry records for the period of 1977–2016. The second area is epidemiological studies to determine the causes of health effects in residents living near NPPs. RHI investigated the prevalence of thyroid cancer in Yeonggwang region in 2014 and the epidemiological factors related to thyroid cancer among people living around NPPs in 2015. RHI also conducted a study on clinical and genetic characteristics of thyroid cancer among the residents nearby NPP sites in 2016. The third area is development of the computational program for estimating the probability of causation of disease (RHI-PEPC) as reasonable judgment criteria for occupational diseases of radiation workers in 2004 (Jeong et al. Citation2004). The Korean Employment and Labor Ministry have employed RHI-PEPC as a reference for the review of occupational diseases.
Currently, RHI is extending their research fields to include molecular epidemiology research using human biological samples to investigate the association between low dose radiation and diseases. The radiation epidemiology research involves 6 employees and 5 contract researchers and currently spends about KRW 300 M/year for research.
Future
The policy on the use of nuclear energy of the newly incumbent South Korean government is anti-nuclear, and its policy is exerting a strong influence on the distribution of the national nuclear R&D fund in various areas related to nuclear energy. This would mean that an increase in the relative share of the national research fund in the area of radiation research is anticipated due to shrinkage of funding allocated to the areas of the new technology developments for the next generation reactor and the back-end fuel cycle, at least for next several years.
Funding in the US
Due to the less centralized nature of scientific research funding in the US compared to, for example, Canada, it is virtually impossible to assess actual sums of money that were dedicated to radiation research in any given year much less over a broader time period. While government agencies such as the US National Institutes of Health (NIH) and the Department of Energy (DOE) provide a route for government funds to reach radiation biology research, a significant addition to funding comes from other agencies such as National Aeronautics and Space Administration (NASA), Congressionally Directed Medical Research Program (CDMRP), as well as private donors who give gifts e.g. to specific clinical departments or universities. These private funds become the property of the universities or hospitals themselves and are not labeled as radiation funding in any type of general registry. It should be appreciated, however, that most of such funding goes to the laboratories that are focused on clinical radiation research and not on low dose radiation research. The same is true for most of the NIH sponsored research. NIH is the primary federal agency that supports basic, translational and clinical research in the biomedical field in the US. In the radiation field, NIH support was and continues to be focused on radiation used in biomedical procedures and, to a lesser degree, exposures from low-dose procedures (such as CT scans) and ways to mitigate the effects of radiation accidents. For example, a task force organized by the American Society for Radiation Oncology (ASTRO) Board of Directors (BOD) evaluated the state of clinical radiation oncology education in the US prior to 2014 (Wallner et al. Citation2014). Using information on NIH funding, the task force found that NIH sponsored radiation biology research falls predominantly into these ‘areas of research specialization’: tumor microenvironment, normal tissue, radiosensitizer, cell cycle/signaling and radioimmunotherapy (Wallner et al. Citation2014). Each of these areas received ≥10% of the very limited portion of the NIH budget. A slightly older study from 2013 (Steinberg et al. Citation2013) identified only 197 NIH-funded radiation oncology grants in that year, coming from only 134 individual investigators. Only 79% of them could be considered as radiation biology. Furthermore, based on the average sum per proposal, this entire budget amounted to about USD 50 M. It is very likely that none of those funds were used for low dose radiation research. General lack of enthusiasm for radiation may perhaps explain the growing tendency in the US for changing the names of departments of radiology into titles that do not include the word ‘radiation’ (Webber et al. Citation2011).
Past (1950–2015)
For most of recent US history, DOE was the primary agency managing low-dose radiation programs. This started in the 1950s when large-scale animal studies were initiated and DOE’s focus on a large-scale comprehensive radiation research program emerged. Teams of investigators begun studies of critical questions in radiation biology, including those at low doses. This DOE program was a major source of data for making policy decisions especially on the effects of low dose rate exposure to external radiation sources and internally deposited radioactive materials (Brooks Citation2015). A history of the DOE Low Dose program has recently been published (Brooks Citation2018). Policymakers rely on critical summaries issued by national agencies such as the National Council on Radiation Protection and Measurements (NCRP), and the National Academy of Sciences (NAS). These summaries are prepared using data generated primarily by researchers funded by a variety of research programs including those funded by NIH, DOE, NASA, CDMRP and others. It is important to note, however, that much of the research in the DOE Low Dose programs was conducted prior to the genomic era. Now, we have vastly improved tools to more closely and accurately interrogate the impacts of radiation at a genetic level.
Present (2015–2018)
Biological research standards have improved tremendously in the last five years let alone 50 years that have passed since DOE first organized radiation research studies. Cancer research performed today and that conducted only a few years ago are qualitatively and substantively different in many ways. Large-scale ‘omics studies make up a large part of cancer research; new animal models with targeted mutations using CRISPR-Cas9 and other gene-editing technologies are used; nanotechnology and other bioengineering combined with materials approaches are applied; approaches for new forms of super-resolution and cryomicroscopy are multiplying; and more. It is unfortunate that while the scientific capabilities to understand the biological impact of low dose radiation have advanced rapidly, funding for radiation biology research in the US has declined dramatically. At the same time, programs in other counties (e.g. EU and Japan) have gained prominence and have put those countries in leadership positions for understanding radiation risks. While the US can certainly utilize data emerging from other countries, it should be noted that complete open access to these data is not always available. For example, much of the data from animal studies in other countries is not publicly available; this means that US may have to wait for 5 years before the results of these studies are published. Considering the time to use such information to inform policy decisions, delays still longer than 5 years may be expected. In addition, approaches to science are different in all countries. This lack of the US experience is thus limiting to the entire community because the US perspective is missing and not provided.
While radiation treats ≥50% of cancer, the proportion of NIH fund that goes into radiation research is only 5% of the whole NIH budget (Steinberg et al. Citation2013). Furthermore, a recent US Government Accountability Office report (GAO Citation2017) has shown a dramatic reduction in research funding for low dose radiation research in the US. According to this report, low dose radiation research including basic radiation biology as well as epidemiological studies for the entire 2012–2016 period received about USD 210 M, with NIH obligating USD 88.6 M and DoE USD 116.3 M. The remainder of the money came through Nuclear Regulatory Commission (NRC), NASA, DOD, Environmental Protection Agency (EPA), and Center for Disease Control and Prevention (CDC). Nevertheless, for complete insight into the situation, even this report would require further verification. In other instances, manual curation of data was necessary to establish accuracy, as explained e.g. in Steinberg et al. (Citation2013). After 2016, these funds were still reduced, and the DOE’s low dose program has now been absent for several years.
EPA lists 12 superfund sites contaminated with radionuclides (Boyd Citation2016) and the National Institute of Environmental Health Sciences (NIEHS) provides ‘superfund grants’ for remedy of these sites. However, none of these grants provide funding support for radiation or radionuclides. The reasons for this gap are unclear. Exposures to man-made radiation sources that are linked to biomedical activities are also increasing, which adds to the overall uncertainty on the cumulative effects of environmental radiation exposures.
While there continues to be some funding for radiation biology research available from some US agencies, it tends to focus on much narrower applications. For example, NASA’s interest in radiation is limited to space quality radiation, i.e. high linear energy transfer (LET) radiation that one encounters in space. These high LET radiation levels do not reach the surface of the Earth due to the Van Allen belts and therefore have less impact on the general population compared with low-LET radiation. NIH has several focal areas that are oriented toward radiation; however, most of these are limited to high dose radiation research with a few exceptions. The National Institute of Allergy and Infectious Diseases (NIAID) has funded research centers on development of mitigators for high dose radiation injury, the National Cancer Institute (NCI) funds radiotherapy groups that investigate radiation types and doses suitable for cancer treatment; and a few imaging groups within NCI and National Institute of Biomedical Imaging and Bioengineering (NIBIB) conduct research aimed at limiting doses from diagnostic procedures involving radiation. Nevertheless, very few of the projects funded by all of these agencies consider the potential health impact of low doses of radiation.
With the decline in funding for low dose radiation research in the US, there is little new radiation biology (particularly at low doses) research done in the US. We must rely on data from research from EU and Japan where new findings suggest a plethora of novel low dose radiation responses. Some of these findings were identified primarily because of new technologies, improved sensitivities of old technologies, and analyses of large-scale databases. These experiments point to new responses at low doses that have not been previously identified as important, such as effects on the central nervous system (CNS), cardiovascular systems, and developmental biology effects (embryo, fetus and perinatal). These new discoveries should, but do not yet factor into regulatory guidelines. Research funded by NASA has also found CNS and cardiovascular effects at low doses of high LET radiation and has been exploring interactions with the EU in hopes to improve NASA’s understanding of low dose radiation effects (by comparing NASA’s high LET findings with the EU’s low LET findings).
Since the time when low dose radiation studies in the US began to decline, biomedical science has continued to progress. New technologies have been developed, and new discoveries have been made. Fine-tuned models could be developed to set the stage for fine-tuned decisions and evidence-based protection policies. The use of stem cells, the role of exosomes and microvesicles in intercellular communication on the level of the whole organism, the role of non-coding RNAs in intra- and intercellular regulation have all found their place in studies of cancer and cancer-related phenomena. All these areas received a significant boost by the introduction of techniques to modulate genome or gene expression (e.g. by CRISPR or silencing RNAs, respectively). Bionanotechnology is also important because of the significant inroads into daily biological (and medical) practice. Moreover, each one of these new developments could open new fields in further exploration of cell and molecular biology. At the same time, these techniques coupled with new types of precision, CT-guided animal irradiators significantly improve translational research with model organisms. Research using these tools can provide a sound scientific basis for supporting exposure regulations in the low dose region.
Researchers studying low dose radiation in the context of genomics, transcriptomics, epigenomics, proteomics, lipidomics, metallomics, metabolomics and other are relatively few. In the US, they were supported mostly through NASA-funded programs (with space-quality radiation) or focused on mixed field, simulated galactic cosmic rays. Informatics, modeling and computational techniques in recent years have become considerably more sophisticated with the development of machine learning approaches. Improved data analysis allows cross-comparisons of large-scale data from the EU and old archival datasets from DOE’s former studies to examine, e.g. the dose and dose-rate effectiveness factor (DDREF) (Haley et al. Citation2015).
Future (2019–)
The recent Congressional bill H.R.589 ‘Department of Energy Research and Innovation Act’ has passed the Senate, and it is on its way to budget negotiations and finally to the President whose action will be needed for this bill to become a law (US Congress Citation2018). This new development suggests that the DOE Low Dose radiation program may become operational again in the near future. Activities targeted by this are: (1) to accelerate breakthroughs and new knowledge to enable the cost-effective, sustainable production of biomass-based liquid transportation fuels, bioenergy, and biobased materials; (2) to improve understanding of the global carbon cycle, including processes for removing carbon dioxide from the atmosphere, through photosynthesis and other biological processes, for sequestration and storage; and (3) to understand the biological mechanisms used to transform, immobilize, or remove contaminants from subsurface environments. None of these activities is focused on low dose radiation research alone; nevertheless, the following statement concludes the bill ‘The office shall carry out a low-dose radiation research program to enhance the scientific knowledge of, and reduce uncertainties associated with, the effects of exposure to low-dose radiation to inform improved risk-management methods.’
Based on previous presentations to the Senate, some of the research directions to be considered in the development of the US low dose program are
How do cells detect and respond to very low doses of radiation?
How are the responses to low and high doses and dose rates of radiation different at the molecular and cellular level, and at tissue and whole body level?
What are the mechanisms indicating that low doses of radiation induce responses that may be protective while effects of high doses are generally damaging?
How do low-dose-rate exposures (applicable to the majority of contemporary human exposures) differ from high dose-rate exposures?
How can up-to-date techniques for data generation and evaluation be applied to new studies on dose and dose-rate effects?
To impact government regulated standards, molecular and cellular information must be linked to experimental animals and humans (advantage of having a unified DOE managed program).
Based on the research needs, funding for low dose radiation in the US should be focused on an entire program with the following components:
A broad-based, multi-faceted research program aimed at integrating ‘omics research and datasets focused on low-dose studies of in vitro and in vivo models is necessary. While some radiation oriented ‘omics exist (e.g. genomics, proteomics, transcriptomics and metabolomics), these are not well integrated. In addition, since many new ‘omics capabilities are currently in use (e.g. elemetalomics, metallomics, epigenomics, functional genomics, lipidomics), these need to be explored and the data from all of these sources integrated into existing datasets in a manner that would allow them to be easily searchable.
Low dose research that relates to each organization level of life is essential: this means research at the level of the organism, the tissue, the organ system, the cell, and cellular organelles. This would include studies on endo- and exocytosis, replenishing of cellular and organelle membranes, intra- and intercellular trafficking, and cellular communication at local and whole organism levels.
The focus must be placed on research that relates to normal healthy tissues and consequences of radiation exposures that are relevant to occupational and environmental situations; of particular relevance would be studies devoted not only to low dose mechanisms but also to low dose rate effects.
Repositories of datasets need to be established and linked to each other with unified approaches for deposition and curation. It is anticipated that new informatics-based approaches (e.g. machine learning, computational biology) can be applied to these datasets to provide new answers to relevant questions of low dose radiation biology.
Biobanking of materials should be done to enhance collaborative use and maximize data extraction.
New animal models with disruptions of specific homeostasis pathways should be developed to understand the radiation response of normal tissues.
It is essential that findings, particularly those observed at low doses be robust and reproducible. Policies about replication of data either by using multiple complementary methods to establish a single result and/or replication by teams of investigators will be established.
Websites with information for scientific investigators, for policymakers as well as for the general public should be established to educate and promote interest and understanding of low dose radiation and its effects. In addition, public forum exchanges should be supported through the website. It may be useful to have partnerships with DOE, NASA, NIH, Occupational Safety and Health Administration (OSHA), EPA and others in this effort.
Any low dose program should include the ability to develop a training program for graduate students and/or postdoctoral fellows in radiation disciplines to enhance the existing community.
Key points that can be made in support of a low dose initiative are likely to include: (1) health effects of low dose radiation exposure remain ambiguous; (2) past work has set us on a good footing for the development of new studies; (3) current policies regulating radiation exposures rely on models for high dose and high dose-rate exposures that have yet to be validated at low dose and dose-rate.; and (4) new technologies have not been adequately applied for low dose radiation research.
Perspective: our thoughts on challenges and potential improvements toward increasing the amount of investment in radiation research
In Canada, the financial climate is favorable for science overall, but whether this will play well for radiation research will mostly depend on how the current funding opportunities are used by individual investigators and heads of relevant research programs or departments. It is feasible to suggest that integration of federal research facilities with academic laboratories within collaborative research projects would be an efficient way of securing more funding, whereas enhancing educational programs in radiation biology could be a viable strategic tool to ensure the future generations of radiation researchers continue the trend.
In the EU, the EC proposed ‘Horizon Europe’ succeeding ‘Horizon 2020’. The proposed budget under Horizon Europe for the Euratom R&T Program is EUR 2.4 billion. The Euratom R&T Program is the only EU program that supports R&T and complements national funding. It supports R&T in both fission and fusion. Research in the context of non-power applications of ionizing radiation focuses on reduction of risks from low dose exposure through the use of these technologies. Research into radiation protection has already benefited the medical sector and has also a significant potential for public benefit in other sectors. The rapidly growing use of nuclear fission technologies worldwide makes Euratom research all the more important. The Euratom R&T also makes improvements in the areas of education, training and access to research infrastructure.
In Japan, the financial limitations of the government make it hard to increase the amount of investment in radiation research. In our opinion, as radiation biology itself may not necessarily be an attractive area of research from the administrative viewpoint, radiation biologists should seek new funding sources by integrating radiation biology with other fields of science.
In South Korea, the national government is in the process of implementing the policy of nuclear energy phase-out since its inception in April 2017. In the next few years, this will affect the allocation of government research fund in the areas of nuclear energy and its related topics, and result in a positive way for an increase in the share and absolute amount of the funding for radiation research.
In the US, based on a recent government bill, DOE may commit some funds for studies of radiation unrelated to radiation oncology exposures. However, these funds will be very limited and seem to be contingent on funding remnants from other DOE interest areas. NIH are inclined to integrate radiation biology with other fields of biomedical science; unfortunately, that has resulted in budgets that are disproportionate, hurting radiation biology more than helping it. Finally, benevolent donors who may be interested to fund research against specific diseases, do not expect that environmental pollution and radiation biology funding need resources other than from the government. It may be necessary to initiate grassroots movements interested in radiation protection and try to use their efforts to provide new ways to obtain funding.
Education and training
Pertinent to funding issues, the needs and problems of education and training in radiation research and radiation protection have been extensively discussed, e.g. in Europe (Ottolenghi et al. Citation2019) and in the US (Coleman et al. Citation2003; Dynlacht et al. Citation2015 ). This brief section provides an overview on the current situations in various countries.
Canada
The Canadian Association of Radiation Oncologists (CARO) has been monitoring trends, identifying gaps and creating recommendations for future developments in the Canadian radiobiological training and education space (Bristow Citation2004). ASTRO has also monitored these as part of the overall surveys and systematic analyses of radiobiological education in North America (Rosenstein et al. Citation2009). Among the general trends were the aging of the teaching faculty, the decline of the educators with graduate training specialized in radiation biology or radiation oncology and the shortening of the relative time spent on teaching activities. A number of recommendations and measures have been introduced. Unfortunately, the quantitative assessments of the progress made between the 2000s and the present day are unavailable. It is tempting to speculate that the situation has been steadily improving. The CARO continues to recognize the importance of education and to put an effort towards maintaining and enhancing related activities. Currently, in Canada there are 12 universities that have undergraduate programs with a track for radiobiology or radiology, 10 universities that have fellowship programs in various radiological medical applications, 15 universities that have radiology related residency programs, and 16 universities that have research laboratories working in the radiation biology areas.
The new CANDU Owners Group funded strategic research initiative on low-dose radiation research covers graduate students’ stipends and postdoctoral fellowships using adjunct professor appointments of the Canadian Nuclear Laboratories radiation biologists at the University of Ottawa. These investigators will also participate in the development of a new graduate course in radiation biology within the new program of Medical Biosciences. It is fair to anticipate a closer integration of radiation protection research scientists (low-dose radiation biology) with educational programs across Canada, including with the help of the increased national science budget, to enhance training and education in radiation biology over the next few years.
EU countries
The EU consists of 28 member states and the level of resources devoted to education and training in radiation protection research varies strongly between the member states. Similarly, the level of university-based research activity varies substantially, depending on available funding to support researcher positions and projects. A number of states such as Germany, France, Belgium and UK have research laboratories incorporated in radiation protection organizations. These laboratories receive statutory funding which covers the basic needs of their research and education and training activities. In other countries such as Sweden and Finland, radiation protection authorities have no research laboratories, but have resources to support research and education in external entities such as universities. In the rest of EU countries, radiation protection authorities have neither research laboratories nor research funds, and radiation researchers must obtain funding from other research supporting organizations.
One task of the EC is to coordinate and financially support research, which is of benefit to the European citizens and promotes collaboration and integration of researchers. To this end, project applications must be composed of researcher groups representing at least 3 EU countries. There are several programs responsible for research in various fields and the Euratom program is responsible for radiation protection research. Until 2010 funding provided by the Euratom program was organized via calls for projects in the general field of radiation research, without an attempt to focus attention on a particular question and without the requirement to include education and training activities. Then, it was decided to focus efforts on the problem of health risks associated with exposure to low radiation doses. Moreover, a stronger integration of efforts to better understand the health risks associated with exposure to low dose and low dose rate radiation was envisaged. The first tool to achieve this aim was the DoReMi network of excellence, which included funds to support European courses in the field radiation protection. Some 10–15, 1–2 week courses were selected from applications submitted to open calls. Moreover, it was also agreed that education and training activities should be included in every research project funded by EURATOM. DoReMi was followed by CONCERT which also includes funds for supporting EU courses. Currently, it is not yet clear how the education and training program will be organized in EU when CONCERT ends in 2019.
Japan
Following the 1958 SCJ recommendation (as described in the section for Japan), tens of medical schools had laboratories for radiation biology and basic radiation medicine, which decreased to 10 in 1999 and 6 in 2009, but increased to 11 in 2018. Three-year colleges with departments of radiological sciences (which students attend to become registered radiological technologists) started being reorganized into four-year universities (awarding a B.Sc degree) in the late 1980s, and many universities have also newly been founded. As of 5 December 2018, 35 universities have departments of radiological sciences, of which 13 have laboratories for radiation biology. The number of such universities with departments of radiological sciences continues to increase in the coming years (expectedly amounting to 40 universities by April 2021), perhaps with a concomitant increase in laboratories for radiation biology there as well. For training, QST-NIRS has offered a series of courses, of which details have recently been described (Shimizu et al. Citation2017). These situations may lead to a slightly increased opportunity for education, training and research of radiation effects, at least in the health science or medical field.
South Korea
In more than 80 colleges with departments of radiological sciences (which students attend to become registered radiological technologists), there are no radiation biology laboratories. At universities with departments of biology, there are some ‘temporary’ laboratories to conduct governmentally funded radiation biology studies, but there are no ‘permanent’ laboratories for radiation biology. Currently, there is no ongoing training course dedicated to radiation biology.
US
There is no information kept in the US on how many laboratories for radiation biology are available. However, there has recently been a drastic decline in the number of training programs in radiation research. A few that focus on radiation physics (with little if any radiation biology) have been developed (most notably at the University of Wisconsin), but new training programs with a solid radiation biology component are unavailable in the US. While there are naturally training components to all radiation programs that include graduate students and post-doctoral fellows, specialized programs devoted to training several post-doctoral fellows (e.g. those in the typical T32 training programs funded by NIH) do not exist in radiation biology research today.
The benefit of these focused training programs for multiple fellows at one time is significant because it allows for the development of centers of excellence in a discipline that fosters interactions among fellows and faculty eventually leading to greater joint scientific achievements. The absence of these programs is largely attributed to the low level of funding for radiation sciences. As a consequence, few institutions have more than one or two funded investigators working in the discipline. At the same time, most training grants require a nucleus of 5–10 funded investigators working in the discipline in order to be eligible for funding. It is therefore difficult to collect sufficient number of investigators and institutions to enable a training grant development. In the absence of these opportunities, training for new radiation scientists consists mostly of broad cancer training programs or training programs coupled with various physical sciences.
The absence of radiation biology training programs ultimately means that there are few trained Ph.D- and post-doctoral level fellows in radiation disciplines, leading in turn to a lack of qualified radiation investigators to oversee programs of radiation biology and radiation protection in departments of radiation oncology, radiology, and others. As needs for trained professionals in these disciplines rise due to the many reasons noted above (need for radiation regulatory policies, concerns about nuclear waste, etc.), the number of functioning radiation scientists continues to drop (e.g. due to retirement) with little hope for replacement with younger trained scientists. NCRP issued a WARP (Where are the Radiation Professionals) initiative to examine this problem in more detail, and Council Committee 2 (CC 2) of NCRP will be releasing a report detailing reasons for this loss (NCRP Citation2015).
Conclusions
This paper reviewed historical changes in funding for radiation research and provided a brief overview of current situations in education and training in this field, in Canada, EU countries, Japan, South Korea, and US. In addition to potential occupational or medical exposures to radiation, there have been rising concerns about nuclear exposure from terrorist attacks and other accidents that could have consequences for the population. As such, a better understanding of the biological consequences of radiation exposure (especially at low dose and low dose rate) is becoming more important. To this end, continued funding for radiation research is needed, and education and training in this field are also important.
Acknowledgments
The authors wish to thank Drs. Nobuhiko Ban (NRA, Japan), Douglas Boreham (Bruce Power and Laurentian Univ., Canada), Richard Hill (Univ. of Toronto, Canada), Meeseon Jeong (RHI, South Korea), Reiko Kanda (QST-NIRS, Japan), Takashi Kondo (Toyama Univ., Japan), Ron Mitchel (retired from AECL, Canada), Nori Nakamura (RERF, Japan), Seon Young Nam (RHI, South Korea), Peggy Olive (retired from BC Cancer Research Centre, Canada), Nicholas Priest (CANDU Owners Group, Canada), Yasuhito Sasaki (REA, Japan), and Teruhisa Tsuzuki (Kyushu Univ., Japan) for providing information. CONCERT project has received funding from the Euratom research and training program 2014–2018 under grant agreement No 662287. The European Commission is not responsible for any use that may be made of the information it contains.
Disclosure statement
The authors report no conflict of interest. The authors alone are responsible for the content and writing of the paper. The views expressed in this paper represent collective opinions of the authors and are not necessarily those of their professional affiliations.
Additional information
Notes on contributors
Kunwoo Cho
Kunwoo Cho, Ph.D., is a member of ICRP Main Commission from 2017 to 2021. He was Vice Chair of ICRP Committee 4 from 2013 to 2017 during which time he was Chair of Task Group 94 that produced ICRP Publication 138 ‘Ethical foundation of the system of radiological protection’. He was a national representative to UNSCEAR from 2013 to 2015. He has been a Vice President of Korean Association for Radiation Protection since 2012.
Tatsuhiko Imaoka
Tatsuhiko Imaoka, Ph.D., is a Team Leader at the National Institute of Radiological Sciences, National Institutes for Quantum and Radiological Science and Technology. His research interest spans basic radiation biology, including that of tissue stem cells, to understand the quantitative nature of radiation carcinogenesis and application of quantum mechanics and related technologies to radiation biology.
Dmitry Klokov
Dmitry Klokov, Ph.D., obtained a Ph.D. in Radiobiology from Moscow State University in 2000. He is currently head of Radiobiology section at Canadian Nuclear Laboratories and an Adjunct Professor at the Department of Biochemistry, Microbiology and Immunology in the University of Ottawa. He has published over 45 papers in peer-reviewed literature. His research interests lie broadly in various fields of radiobiology.
Tatjana Paunesku
Tatjana Paunesku, Ph.D., is Research Associate Professor at Radiation Oncology Department at Northwestern University’s Feinberg School of Medicine. She is currently involved in research in radiation biology, nanotechnology and synchrotron X-ray radiation. She has published over 70 peer-reviewed articles and has trained many undergraduate and graduate students and residents over her 15 years at Northwestern University.
Sisko Salomaa
Sisko Salomaa, Ph.D., is Professor of radiobiology in University of Eastern Finland and Coordinator of National Radiation Safety Research Program in STUK. As part of setting up the European low dose program (MELODI), she coordinated DoReMi Network of Excellence 2010–2015. She is representative of Finland to UNSCEAR and a member of ICRP C1.
Mandy Birschwilks
Mandy Birschwilks, Ph.D., has more than 10 years of experience in coordinating and managing large international projects (FP6, FP7, Horizon 2020): e.g. ERA-PRO, STORE, OPERRA, Dark-Risk, and is currently actively involved in the EJP CONCERT.
Simon Bouffler
Simon Bouffler, Ph.D., leads the Radiation Effects Department at Public Health England. He is involved in several European research initiatives and is currently Chair of the MELODI Strategic Research Agenda working group. He leads the UK delegation to UNSCEAR and is a Main Commission member of ICRP.
Antone L. Brooks
Antone L. Brooks, Ph.D., Is a retired professor from Washington State University. He served on the NCRP for almost 30 years and was on the Board of Directors for that organization, was a member of the EPA science advisory board, was on the National Academy of Science team that produced the BEIR VI report and was the Chief Scientist for the Department of Energy Low Dose Radiation Research Program. He recently published a book ‘Low Dose Radiation, The history of the U.S. Department of Energy Research Program.’ He has published over 200 peer-reviewed articles and served on many national and international review groups.
Tom K. Hei
Tom K. Hei, Ph.D., D.Sc., is professor and vice-chairman of radiation oncology and associate director of the Center for Radiological Research at Columbia University. Dr. Hei has a distinguished career in radiological sciences and has been honored with many awards/recognitions. He served in many advisory panels including the National Academy of Sciences and the Institute of Medicine. He has published over 250 peer-reviewed articles and has trained many fellows, graduate students and residents over his 35-year career at Columbia University.
Toshiyasu Iwasaki
Toshiyasu Iwasaki, Ph.D., is a director of Radiation Safety Research Center in Central Research Institute of Electric Power Industry. His research interest is in the dose-rate effects of radiation-induced late effects at very low dose-rate.
Tetsuya Ono
Tetsuya Ono, Ph.D., is currently the president of the Institute for Environmental Sciences. He is interested in molecular and cellular mechanisms of radiation-induced late effects such as cancer induction and life shortening, and also the ways to alleviate these effects, especially calorie restriction.
Kazuo Sakai
Kazuo Sakai, Ph.D., is currently a Professor of Faculty of Nursing, Tokyo Healthcare University. For ICRP, he was a member of Committee 5 until 2017 and now is a member of Committee 1. He obtained a Ph. D. degree in biophysics and biochemistry from the University of Tokyo. He worked for the Department of Radiation Oncology, University of Tokyo, 1982–1999, the Central Research Institute of Electric Power Industry, 1999–2006, National Institute of Radiological Sciences, 2006–2015, before he moved to the current position.
Andrzej Wojcik
Andrzej Wojcik, D.Sc., is a Professor of radiation biology at the Stockholm University. Wojcik focuses on studying the cellular effects of combined exposure to radiations of different qualities, with special reference to cytogenetic effects of radiation.
Gayle E. Woloschak
Gayle E. Woloschak, Ph.D., is Professor of Radiation Oncology and Professor of Radiology at Northwestern University’s Feinberg School of Medicine. She is currently Chair of Program Area Committee 1 for the National Council on Radiation Protection and Measurements, a member of International Commission on Radiological Protection Committee 1, and a member of the US delegation to the U.N. Scientific Committee on the Effects of Atomic Radiation.
Yutaka Yamada
Yutaka Yamada, D.V.M., Ph.D., is a director of Fukushima Project Headquarters in QST-NIRS. He earned his Ph.D. in veterinary medicine at the Hokkaido University in 1988, then, started researches in NIRS. His main field is radiation biology and radiotoxicology, and his studies have focused on the radiation-induced pulmonary carcinogenesis. He was a visiting scientist at the Life Sciences Division, Los Alamos National Laboratory, in the US from 1993 through 1995.
Nobuyuki Hamada
Nobuyuki Hamada, RT, Ph.D., is currently a corresponding member of ICRP Task Group 102 and Task Group 111, a member of NCRP PAC 1, Chair of Scientific Advisory Board for the European CONCERT LDLensRad project, and a member of IRPA Phase 3 Task Group on the implementation of the eye lens dose limits. He has published >100 papers in peer-reviewed international journals and has received 18 awards including the 2013 Michael Fry Research Award of the US Radiation Research Society.
References
- AEC. 2003. About the New Crossover Research. Tokyo: Atomic Energy Commission (AEC). [in Japanese] [Accessed 2018 Dec 5]. http://www.aec.go.jp/jicst/NC/iinkai/teirei/siryo2003/siryo25/siryo42.pdf.
- Boyd MA. 2016. Contaminated sites from the past: experience of the US Environmental Protection Agency. Ann Icrp. 45:84–90.
- Braga-Tanaka I, Tanaka S, Kohda A, Takai D, Nakamura S, Ono T, Tanaka K, Komura J-I. 3rd. 2018. Experimental studies on the biological effects of chronic low dose-rate radiation exposure in mice: overview of the studies at the Institute for Environmental Sciences. Int J Radiat Biol. 94:423–433.
- Bristow RG. 2004. Recommendations for the future of translational radiobiology research: a Canadian perspective. Radiother Oncol. 70:159–164.
- Brooks AL. 2015. A commentary on: “a history of the United States Department of Energy (DOE) low dose radiation research program: 1998–2008”. Radiat Res. 183:375–381.
- Brooks AL. 2018. Low Dose Radiation. The History of the U.S. Department of Energy Research Program. Washington: Washington State University Press.
- Cody HR. 1932. Report of the Royal Commission on the Use of Radium and X-Rays in the Treatment of the Sick. Toronto: Toronto King’s Printer: The Royal Commission. http://www.ontla.on.ca/library/repository/mon/25006/15404.pdf [Accessed 2019 Feb 6].
- Coleman CN, Stone HB, Alexander GA, Barcellos-Hoff MH, Bedford JS, Bristow RG, Dynlacht JR, Fuks Z, Gorelic LS, Hill RP, et al. 2003. Education and training for radiation scientists: radiation research program and American Society of Therapeutic Radiology and Oncology Workshop, Bethesda, Maryland, May 12–14, 2003. Radiat Res. 160:729–737.
- CRIEPI. 2018. FY2017 Annual Report. [in Japanese] [Accessed 2018 Dec 5]. https://criepi.denken.or.jp/intro/info/2017annual_report.pdf.
- Dynlacht JR, Zeman EM, Held KD, Deye J, Vikram B, Joiner MC. 2015. Education and training needs in the radiation sciences: problems and potential solutions. Radiat Res. 184:449–455.
- EC. 2018. Commission staff working document. Annex: Impact assessment of the Euratom Research and Training Programme 2021–2025. [Accessed 2018 Dec 5]. https://ec.europa.eu/info/sites/info/files/swd_2018_307_f1_impact_assesment_en_v6_p3_977548.pdf
- Eggleston W, Irwin C. 1965. Canada’s nuclear story. Toronto: Harrap Research Publications.
- GAO 2017. Low-dose radiation: Interagency Collaboration on Planning Research Could Improve Information on Health Effects. GAO-17-546 2017. Publicly Released: 26 October 2017. [Accessed 2018 Dec 5]. https://www.gao.gov/products/GAO-17-546.
- Godfrey LG. 1979. The changing years, the story of Toronto Hospital and the fight against tuberculosis. Toronto: West Park Hospital.
- Gong J, Le TQ, Massarelli E, Hendifar AE, Tuli R. 2018. Radiation therapy and PD-1/PD-L1 blockade: the clinical development of an evolving anticancer combination. J Immunother Cancer. 6:46.
- Haley BM, Paunesku T, Grdina DJ, Woloschak GE. 2015. The increase in animal mortality risk following exposure to sparsely ionizing radiation is not linear quadratic with dose. PLoS One. 10:e0140989.
- Hamada N. 2014. What are the intracellular targets and intratissue target cells for radiation effects? Radiat Res. 181:9–20.
- Hayter CR. 1998. Historical origins of current problems in cancer control. Cmaj. 158:1735–1740.
- ICRP. 2012. ICRP Statement on Tissue Reactions/Early and late effects of radiation in normal tissues and organs – threshold doses for tissue reactions in a radiation protection context. ICRP Publication 118 Ann ICRP. 41:1–322.
- Jeong M, Jun Y, Kim C. 2004. Program for estimating the probability of causation to Korean radiation workers with cancer. J Radiat Prot Res 29:221–230.
- Kirsch DG, Diehn M, Kesarwala AH, Maity A, Morgan MA, Schwarz JK, Bristow R, Demaria S, Eke I, Griffin RJ, et al. 2018. The future of radiobiology. J Natl Cancer Inst. 110:329–340.
- Kudo S, Ishida J, Yoshimoto K, Mizuno S, Ohshima S, Furuta H, Kasagi F. 2018. Direct adjustment for confounding by smoking reduces radiation-related cancer risk estimates of mortality among male nuclear workers in Japan, 1999-2010. J Radiol Prot. 38:357–371.
- Kuniya M. 1990. Nuclear underlying technology development and cross-over research, in facing the 21st century. J Atom Ener Soc Jap. 32:252–257. https://doi.org/10.3327/jaesj.32.252 [in Japanese] [Accessed 2018 Dec 5].
- Lievens Y, De Schutter H, Stellamans K, Rosskamp M, Van Eycken L. 2017. Radiotherapy access in Belgium: how far are we from evidence-based utilisation? Eur J Cancer. 84:102–113.
- Medical Radiology in Canada. 1933. Nature. 132:347. https://www.nature.com/articles/132347a0
- MOE 2018a. Reports of the Nuclear Disaster Influences Investigation Project. Ministry of the Environment (MOE). https://www.env.go.jp/chemi/rhm/reports.html [in Japanese] [Accessed 2018 Dec 5].
- MOE. 2018b. Public Project Review 2013, Project No. 335. Ministry of the Environment (MOE) https://www.env.go.jp/guide/budget/spv_eff/review_h25/sheets_h24f/sheets/335.pdf [in Japanese] [Accessed 2018 Dec 5].
- MOE. 2018c. Research on the Health Effects of Radiation. Ministry of the Environment (MOE) http://www.env.go.jp/chemi/rhm/study.html [in Japanese] [Accessed 2018 Dec 5].
- NCRP. 2015. Where are the Radiation Professionals (WARP)? NCRP Statement No. 12. https://ncrponline.org/wp-content/themes/ncrp/PDFs/Statement_12.pdf [Accessed 2018 Dec 5].
- Newcombe HB. 1948. Delayed phenotypic expression of spontaneous mutations in Escherichia coli. Genetics. 33:447–476.
- NII. 2018. Database of Grants-in-Aid for Scientific Research (KAKEN). National Institute of Informatics (NII) https://kaken.nii.ac.jp/en/ [Accessed 2018 Dec 5].
- NIRS. 1977. National Institute of Radiological Sciences 20th Anniversary Publication. Chiba: National Institute of Radiological Sciences (NIRS) [in Japanese].
- NIRS. 1987. National Institute of Radiological Sciences 30th Anniversary Publication. Chiba: National Institute of Radiological Sciences (NIRS) [in Japanese].
- NIRS. 1995. National Institute of Radiological Sciences Fiscal Year 1995 Project Plan. Chiba: National Institute of Radiological Sciences (NIRS) [in Japanese].
- NIRS 2018. National Institute of Radiological Sciences Financial Information. National Institutes for Quantum and Radiological Science and Technology (QST). http://www.nirs.qst.go.jp/public/financial/index.html [in Japanese] [Accessed 2018 Dec 5].
- NISTEP 2013. “Database of Resource Allocation in Science and Technology and Innovation Policy” 3rd Policy-Oriented Research Group, National Institute of Science and Technology Policy (NISTEP), Ministry of Education, Culture, Sports, Science and Technology. http://www.nistep.go.jp/wp/wp-content/uploads/NISTEP-NN009-FullJ.pdf [in Japanese] [Accessed 2018 Dec 5].
- NRA 2018. Radiation Safety Research Promotion Fund. Nuclear Regulation Authority (NRA). http://www.nsr.go.jp/disclosure/committee/yuushikisya/kiseikenkyuu/index.html [in Japanese] [Accessed 2018 Dec 5].
- NSRA 2018a. Evaluation: Nuclear Energy Science and Technology and Human Resource Development Project through Concentrating Wisdom. Nuclear Safety Research Association (NSRA). https://www.kenkyu.jp/nuclear/evaluation/index.html [in Japanese] [Accessed 2018 Dec 5].
- NSRA 2018b. Call: Nuclear Energy Science and Technology and Human Resource Development Project through Concentrating Wisdom. Nuclear Safety Research Association (NSRA). https://www.kenkyu.jp/nuclear/application/index.html [in Japanese] [Accessed 2018 Dec 5].
- Ottolenghi A, Trott KR, Smyth V, 2019. Education and training to support radiation protection research in Europe: the DoReMi experience. Int J Radiat Biol. 95:90–96.
- Owens B. 2018. Canadian science wins billions in new budget. Nature. 555:153.
- REA. 2016. Report on the Concept of Radiation Epidemiology Survey. Tokyo: Radiation Effects Association (REA). http://www.rea.or.jp/ire/pdf/arikata_report.pdf [in Japanese] [Accessed 2018 Dec 5].
- RERF. 2018. History of ABCC-RERF. Radiation Effects Research Foundation (RERF). http://www.rerf.jp/intro/establish/rerf30the.pdf[in Japanese] [Accessed 2018 Dec 5].
- Richards A. 1915. Recent studies on the biological effects of radioactivity. Science. 42:287–300.
- Rosenstein BS, Held KD, Rockwell S, Williams JP, Zeman EM. 2009. American Society for Radiation Oncology (ASTRO ) survey of radiation biology educators in U.S. and Canadian radiation oncology residency programs. Int J Radiat Oncol Biol Phys. 75:896–905.
- Shimizu Y, Iida H, Nenoi M. 2017. Trends of training courses conducted in the Human Resources Development Center of the National Institute for Quantum and Radiological Science and Technology after the Fukushima Dai-Ichi nuclear power plant accident. Health Phys. 113:66–70.
- Slater JM. 2012. From X-rays to ion beams: A short history of radiation therapy. In: Linz U, editor. Ion Beam Therapy: Fundamentals, Technology, Clinical Applications. Berlin, Heidelberg: Springer; p. 3–16.
- Steensma DP, Rayson D, Shampo MA, Kyle RA. 2014. Terry Fox: Canadian cancer research activist whose “Marathon of Hope” inspired millions. Mayo Clin Proc. 89:e75–e76.
- Steinberg M, McBride WH, Vlashi E, Pajonk F. 2013. National Institutes of Health funding in radiation oncology: a snapshot. Int J Radiat Oncol Biol Phys. 86:234–240.
- Taylor LS. 1983. The tripartite conferences on radiation protection. Canada, United Kingdom, United States. US Department of Energy.
- Thome C, Tharmalingam S, Pirkkanen J, Zarnke A, Laframboise T, Boreham DR. 2017. The REPAIR Project: examining the biological impacts of sub-background radiation exposure within SNOLAB, a deep underground laboratory. Radiat Res. 188:470–474.
- US Congress. 2018. United States 115th Congress (Committees: House - Science, S., and Technology | Senate - Energy and Natural Resources), H.R.589 – Department of Energy Research and Innovation Act. https://www.congress.gov/bill/115th-congress/house-bill/589 [Accessed 2018 Dec 5].
- Wallner PE, Anscher MS, Barker CA, Bassetti M, Bristow RG, Cha YI, Dicker AP, Formenti SC, Graves EE, Hahn SM, et al. 2014. Current status and recommendations for the future of research, teaching, and testing in the biological sciences of radiation oncology: report of the American Society for Radiation Oncology Cancer Biology/Radiation Biology Task Force, executive summary. Int J Radiat Oncol Biol Phys. 88:11–17.
- Wang Y, Bannister LA, Sebastian S, Le Y, Ismail Y, Didychuk C, Richardson RB, Flegal F, Paterson LC, Causey P, et al. 2019. Low-dose radiobiology program at Canadian Nuclear Laboratories: past, present and future. Int J Radiat Biol. 95:in press. doi:10.1080/09553002.2018.1562252
- Webber GR, Mullins ME, Chen Z, Meltzer CC. 2011. What's in a name? US academic radiology departments: investigation of the frequency of and associated factors in nontraditional department titles. J Am Coll Radiol. 8:186–190.
- Weiss WA, Yonekura S. 2019. The Osaka Call-for-Action. Int J Radiat Biol. 95: in press.
- Write AH, et al. 1915. Editorial: National Sanitarium Association. In: The Canadian practitioner and review. Toronto. Vol. XL. P. 197.