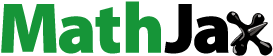
Abstract
Purpose: The combined toxicity of alpha particles and cigarette smoke to the critical cells in the lungs was investigated to assess the risk of smoking workers who handle naturally occurring radioactive materials.
Materials and methods: The toxicity of alpha particles and cigarette smoke extract (CSE) was evaluated in terms of DNA double-strand break (DSB) induction and clonogenic cell death of human lung epithelial cells in vitro. The cells were exposed to alpha particles at doses of up to 0.25 Gy for gamma-H2AX assay and from 1.25 Gy to 5 Gy for clonogenic assay. CSE exposure of the cells was facilitated in the culture medium at CSE concentrations ranging from 1% to 12%. Additional experiments were performed using mouse endothelial cells for comparison.
Results: The increases in the levels of DNA DSBs were linearly dependent on radiation dose and CSE concentration. The CSE-treated cells also responded with a linearly increasing number of DNA DSBs to the radiation dose. Both human lung epithelial cells and mouse endothelial cells showed exponential decreases in clonogenic surviving fraction as the dose from alpha particle exposure increased. Both cells responded with the clonogenic surviving fractions decreasing in a linear proportion to the CSE concentration in the culture medium.
Conclusion: In our experimental in vitro setup, CSE treatment and alpha particle exposure affected the cells in an additive manner either for DNA DSB production or for clonogenic cell death induction.
Introduction
Alpha-emitting radionuclides (RIs) released by naturally occurring radioactive materials (NORMs) are often carried by air dust particles. The RIs in the air enter the human body through the respiratory system during inhalation and accumulate on the bronchial wall. Approximately 45% of the inhaled dust particles are deposited on the inner bronchial wall (Hofmann and Asgharian Citation2003). They are absorbed into the blood over time and migrate to the target organs of each chemical component. The vascular system and target organs are exposed to radiation emitted from those RIs. The major effect of inhaled RIs is counted in the lung bronchi. When the RI-carrying particles are deposited on the bronchial wall, their distribution is inhomogeneous and especially high at the bifurcation (Hofmann et al. Citation1990; Truta-Popa et al. Citation2011). The alpha particles emitted from RIs carry sufficient energy to penetrate the bronchial epithelia. The high linear energy transfer (LET) alpha particles result in high local cellular doses, even when the average lung dose is estimated to be low (Hofmann et al. Citation1990; Truta-Popa et al. Citation2011; Madas Citation2016). The exposure of bronchial epithelia to high-LET radiation is an important issue because the bronchi are the main location of lung cancer development (National Research Council Citation1991; Sutherland and Berns Citation2010). The inhaled alpha particle-emitting RIs have received attention because of their harmful effect on the lungs, especially in comparison with that of cigarette smoke, which is widely known as the most harmful lung agent (National Research Council Citation1999).
Cigarette-smoking radiation workers who handle NORMs are exposed to cigarette smoke and radiation. The risk of alpha particle exposure to the cigarette smokers was studied from a radiation protection point of view by quantifying the extent of the increase in radiation harm due to smoking habits (Health Protection Agency Citation2013). Harmful factors that coexist in the body may affect an individual in a completely independent way or in a certain combination. Previous studies described the following three possible ways of interaction between radiation exposure (alpha particles in epidemiological studies) and cigarette smoking: additive (Narayanan and Rao Citation1988; Piao and Hei Citation1993; Zhou et al. Citation1999; Pierce et al. Citation2003; Tomasek Citation2013), multiplicative (Pershagen et al. Citation1994; Kreisheimer et al. Citation2003; Jacob et al. Citation2005; Hunter et al. Citation2015; Kreuzer et al. Citation2018), and supra-additive (or sub-multiplicative) (Moolgavkar et al. Citation1993; Zhang et al. Citation2006; Mauderly et al. Citation2010; Leuraud et al. Citation2011; Tomasek Citation2011; Meenakshi and Mohankumar Citation2013; Gilbert et al. Citation2013; Hunter et al. Citation2015; Kreuzer et al. Citation2018). In recent years, the possibility of a supra-multiplicative model has been further claimed (Kreuzer et al. Citation2018). The influence of smoking on radio-sensitivity was also debated to vary with specific conditions, including smoking rate (Monchaux et al. Citation1994; Liddell and Armstrong Citation2002; Furukawa et al. Citation2010; Egawa et al. Citation2012). No single model has been mechanistically validated for the combined effect between internal exposure and smoking.
The combined effect was supra-additive (sub-multiplicative) or multiplicative rather than additive according to multiple epidemiological studies (Moolgavkar et al. Citation1993; Leuraud et al. Citation2011; Tomasek Citation2011; Meenakshi and Mohankumar Citation2013; Gilbert et al. Citation2013; Kreuzer et al. Citation2018). However, those epidemiological studies gave no explanation regarding the mechanisms behind the supra-additivity. The combined effect of alpha particle exposure and smoking was investigated via in vitro studies as well. Piao and Hei (Citation1993) reported the additive influence of alpha particle exposure at a single dose of 50 cGy to smoking on cell proliferation and oncogenic transformation. They used their own cigarette smoke condensate for assessing the smoking risk. Zhou et al. (Citation1999) reported an additive interaction of alpha particles and a single chemical NNK (4-methylnitrosamine-1-3-pyridyl-1-butanone) in cigarettes. Both studies are not completely informative in regard to the risk of smoking radiation workers because cell lines were not specific to the lungs. Other studies have limits in delivering interpretations of the combined effect between internal alpha exposure and smoking because they conducted experiments with low-LET photons (Narayanan and Rao Citation1988; Zhang et al. Citation2006; Chen et al. Citation2012), even with human lung cells (Nishioka et al. Citation2011; Chen et al. Citation2012). Meenakshi and Mohankumar (Citation2013) observed a synergistic effect in blood samples from smokers and non-smokers by exposing those samples to alpha particles in radon chamber.
In this study, we performed in vitro experiments to investigate the influence of cigarette smoke on the response of human lung epithelial cells to the alpha particles by considering the energies of alpha particles emitted from the inhaled RIs in the NORM environment. DNA damage and clonogenic cell death were observed as an initial response and a final response of cells, respectively. We analyzed the combined effect in cells identified in ours and other previous studies in comparison with the observations in epidemiological studies.
Materials and methods
Cell lines and cell culture
Considering that bronchi are the major location of lung cancer development and that RIs carried by inhaled dust particles and ingredients of cigarette smoke are absorbed through the lung epithelia into the blood and move to target organs, we chose lung epithelial cells and vascular endothelial cells as damage targets of interest. Given that we are concerned about the harmful effects of alpha particle exposure and smoking, normal cells are proper objects. Two cell lines of normal human lung epithelia (BEAS-2B and Nuli-1) (Catalog No. CRL-9609 and CRL-4011, American Type Culture Collection (ATCC), Manassas, VA, USA) and mouse endothelial cells (SVEC4-10EHR1) (Catalog No. CRL-2161, ATCC) were used in this study.
BEAS-2B cells were cultured with LHC-9 medium (Catalog No. 12680-013, Thermo Fisher Scientific, Waltham, MA, USA). Nuli-1 cells were cultured in the airway epithelial cell basal medium (Catalog No. PCS-300-030, ATCC) with bronchial epithelial cell growth kit supplements (Catalog No. PCS-300-040, ATCC). SVEC4-10EHR1 cells were cultured with Dulbecco’s Modified Eagle Medium (Catalog No. SH30022.01, Hyclone, UT, USA) and 10% heat-inactivated fetal bovine serum (Catalog No. 30-2020, ATCC) mixture. All the cells were cultured in T-25 flasks (Nunc, Roskilde, Denmark). The BEAS-2B and Nuli-1 cells were grown at 37 °C in a humidified incubator (Catalog No. MCO-130AIC, Panasonic, Gunma, Japan) with 5% CO2, whereas the SVEC4-10EHR1 cells were incubated at 37 °C in 10% CO2. For every experiment, cells were seeded on a Mylar dish one day before alpha particle exposure or CSE treatment. The medium was changed once every three days.
Alpha particle exposure
The exposure of cells to alpha particles was conducted by using our own SNU-ALPHACELL system (Lee et al. Citation2016). The RIs of our concern contained in NORM materials are mainly alpha emitters, and those alpha particles carry energies ranging from 5 MeV to 9 MeV. In the SNU-ALPHACELL system, cells were exposed to 5.5 MeV alpha particles emitted from Am-241 disc sources. Dose rate was controlled at 0.48 Gy/min for clonogenic assay and at 0.079 Gy/min for gamma-H2AX assay. A 3.793 MBq of Am-241 disc source was installed in the system to deliver doses at 0.48 Gy/min to the cells located at a source-to-target distance (STD) of 25 mm. The dose was delivered at 0.079 Gy/min to the cells at 20 mm of STD with a 371.1 kBq of Am-241 source installed in the system.
Preparation and treatment of cigarette smoke extract (CSE)
CSE was obtained through a process based on previous studies (Carp and Janoff Citation1978; Jeon et al. Citation2016; Geng et al. Citation2017). shows a schematic of the CSE extraction and the devices used. We used cigarettes containing 4.5 mg of tar and 0.45 mg of nicotine per piece. Smoke came out of the four burning cigarettes and bubbled through 40 ml of Dulbecco’s phosphate buffered saline (DPBS) in a gas absorption bottle (Catalog No. BT1650, Daeyoung Science, Seoul, Korea) during vacuum pumping (Catalog No. BT101L + YZ15, Lead Fluid, Baoding, China). The cigarette smoke and DPBS mixture was filtered through a NalgeneTM Rapid-FlowTM sterile disposable bottle-top filter (Thermo Fisher Scientific, Waltham, MA, USA). This stock was considered as 100% CSE. The collected CSE was stored in a refrigerator at −20 °C for a maximum of two weeks before being mixed in cell culture media. The cytotoxicity of CSE was observed after 24 h of cell treatment in the culture medium. The influence of CSE on the radio-response of cells was investigated by treating the cells with CSE in a culture medium for 24 h before irradiation. No significant pH change in the culture medium was observed after adding CSE at concentrations of up to 30% both at STP and at 5% CO2 in the atmosphere ().
Figure 1. (a) A schematic diagram of CSE extraction and culture medium treatment and (b) a picture of the actual CSE extraction devices and pure CSE solution.
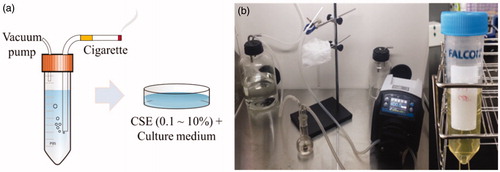
Table 1. The pHs of culture media containing varying amounts of CSE under different CO2 concentrations in atmosphere.
Gamma-H2AX analysis
Gamma-H2AX expression was quantified by counting the number of gamma-H2AX foci per cell. The gamma-H2AX foci in individual cells were identified using fluorescent cell images. For the imaging of gamma-H2AX foci, the procedure of handling the cells is as follows: Cells were seeded on a cell culture slide (Catalog No. 30108, SPL Life Science Co., Pocheon, Korea). For irradiation, the side parts of the slide were removed and the cells were covered with Mylar film. The slide was turned to have the Mylar side facing the alpha beam during irradiation. Irradiated or CSE-treated cells were washed with DPBS (Catalog No. 17-512F, Lonza, Basel, Switzerland) and fixed in 4% paraformaldehyde (Catalog No. 163-20145, Wako Pure Chemical Industries, Ltd., Japan) for 15 min. The samples were then washed twice in ice-cold DPBS and permeabilized with 1% Triton X-100 (Catalog No. T9500-010, GenDEPOT, TX, USA) solution for 15 min. These cells were washed three times with DPBS and blocked with 10% bovine serum albumin (BSA) (Catalog No. A0100-010, GenDEPOT) in 0.05% Tween 20 (Catalog No. T9100-010, GenDEPOT) and DPBS mixture (PBST). The blocked samples were stained for an hour with an anti-gamma-H2AX phosphor S139 antibody (Catalog No. Ab2893, Abcam, Cambridge, UK) and 1% BSA in PBST solution. These cells were washed three times, treated with goat anti-rabbit IgG (H&L) fluorescein isothiocyanate antibody (Catalog No. Ab6717, Abcam) in 1% BSA solution for an hour, and then stained with 4” 6-diamidino-2-phenylindole (DAPI) in fluoroshield mounting medium (Catalog No. Ab104139, Abcam) and covered with a coverslip.
Foci images were taken using a fluorescence microscope (BX53F, Olympus, Tokyo, Japan) at a 10× objective. At least nine local spots that showed approximately 100 nuclei were randomly selected for taking images. Not only hit cells but also non-hit cells were included in the images and altogether taken into account for foci analysis. The nuclei and foci were differentiated with a DAPI fluorescent filter and a green fluorescent protein filter, respectively, which were installed in the microscope. The gamma-H2AX foci were identified using the open-source software CellProfiler (version 2.1.1, Broad Institute’s Imaging Platform, USA). The number of foci per cell was recorded for different combinations of radiation dose and CSE concentration. The average number of foci in control cells (at 0 Gy in CSE-free medium) was normalized to 1. At least four independent experiments were conducted for a single data point.
Clonogenic assay
The cells on Mylar dishes were either treated with CSE in culture medium or exposed to alpha particle emissions from Am-241 disc sources in CSE-free culture medium and then exposed to alpha particles in the culture medium containing CSE. After treatment with CSE or exposure to alpha particles, the cells were prepared in single cell suspensions with TrypLE Express (Catalog No. 12605-028, Gibco, Grand Island, NY, USA) and seeded on a six-well cell culture dish (Nunc). After eight days of incubation, the cells were fixed with 70% ethanol and stained with 0.5% (w/v) crystal violet (Catalog No. C0775, Sigma Aldrich, Saint-Louis, MO, USA) in distilled water. Colonies with more than 50 cells were counted as survivors and the surviving fraction (SF) was calculated. At least four independent experiments were conducted for a single data point.
Isobologram analysis
The concepts of isobologram and envelope of additivity (EOA) suggested by Steel and Peckham (Citation1979) were adopted to evaluate the combined effects of alpha particle exposure and cigarette smoking on clonogenic cell death. presents an exemplary schematic of isobologram and the classifications regarding the combined effect of the two factors for a target consequence, such as a specific percentage of clonogenic cell death. In the isobologram, a combination of dose of A and dose of B required for a target consequence residing inside the EOA denotes that the effects of factors A and B are additive regardless of their mechanisms. The dose combination of factors A and B operating via the same mechanism is close to the iso-addition curve, whereas that via independent mechanisms is near the hetero-addition curve. The dose combination to the left of the iso-addition curve denotes that factors A and B operate in a synergistic way (supra-additive). The dose combination to the right of the hetero-addition curve implies that factors A and B operate in an antagonistic way (sub-additive), whereas that in the “protective effect” zone indicates that the administration of either factor A or B allows the other to be prescribed at a dose higher than its single dose required for the same effect. In this study, isobolograms were drawn to analyze the influence of alpha particle dose and CSE concentration on clonogenic cell death.
Statistical analysis
The significance of the difference between the experimental data and mathematically calculated data was judged by both two-way ANOVA and Student’s t-test with a 95% confidence interval (p < .05).
Results
DSB formation due to alpha particle exposure or via CSE treatment
The individual effects of alpha particle exposure and CSE treatment on the induction of DNA DSB in cells are presented in terms of the number of gamma-H2AX foci per cell in . In all cell lines of BEAS-2B, Nuli-1, and SVEC4-10EHR1, the mean number of gamma-H2AX foci per cell increased linearly with the dose of alpha particle exposure and with CSE concentration in the cell culture medium. According to the ANOVA test, both CSE treatment and alpha particle exposure were significant factors for all cell lines (p-values from ANOVA .001). SVEC4-10EHR1 cells were the most sensitive to alpha particle exposure, whereas BEAS-2B and Nuli-1 cells responded in a similar level of lower sensitivities. SVEC4-10EHR1 cells were the most sensitive to CSE treatment, followed by BEAS-2B cells, whereas Nuli-1 cells were the least sensitive.
Figure 3. The numbers of gamma-H2AX foci per cell, normalized to 1 for the control (0 Gy or CSE-free culture medium, respectively), in BEAS-2B, SVEC4-10EHR1 and Nuli-1 cells after (a) alpha particle exposures in CSE-free culture media at doses of up to 0.25 Gy and (b) CSE treatments at concentrations of up to 10% without alpha particle exposure. The error bars represent standard errors.
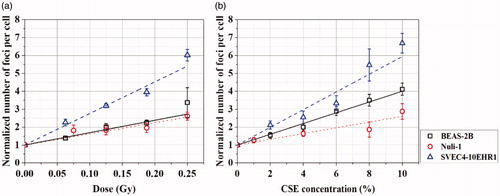
The combined effect of alpha particle exposure and CSE treatment was investigated in the cells exposed to alpha particles at different doses of up to 0.25 Gy in the culture media treated with 4%, 6%, and 8% concentrations of CSE. For each cell line, we chose two levels of CSE concentrations that would give different baselines (number of foci per cell at 0 Gy). Nuli-1 is the least sensitive to CSE treatment as shown in and hence, higher concentration was needed for a differentiable baseline from that at 4%. presents the numbers of gamma-H2AX foci per cell observed (black rectangles) in BEAS-2B, Nuli-1, and SVEC4-10EHR1 cells after alpha particle exposure in CSE-treated culture media. Each experimental data point is compared with the values calculated by combining two separate data obtained from alpha particle exposure () and CSE treatment () in an additive (blue triangles) or multiplicative (red circles) mode (Piao and Hei Citation1993; Kreisheimer et al. Citation2003; Tomasek Citation2013). The calculations in additive and multiplicative modes were made by the following equations on the basis of previous studies (Furukawa et al. Citation2010; Egawa et al. Citation2012), with the number of gamma-H2AX foci at no treatment (0 Gy in CSE-free culture medium) normalized to 1:
Figure 4. The numbers of gamma-H2AX foci per cell (black rectangles), normalized to 1 for the control (0 Gy and CSE-free culture medium), in (a) BEAS-2B, (b) Nuli-1 and (c) SVEC4-10EHR1 cells after alpha particle exposures in culture media treated with 4, 6 or 8% of CSE. The values for 0.00 Gy equal the corresponding numbers of foci at 4%, 6%, or 8% of CSE treatment from . The numbers of gamma-H2AX foci per cell expected from a multiplicative mode (red circles) or an additive mode (blue triangles) of combined effect are marked in the corresponding graph for comparison. The error bars represent standard errors.
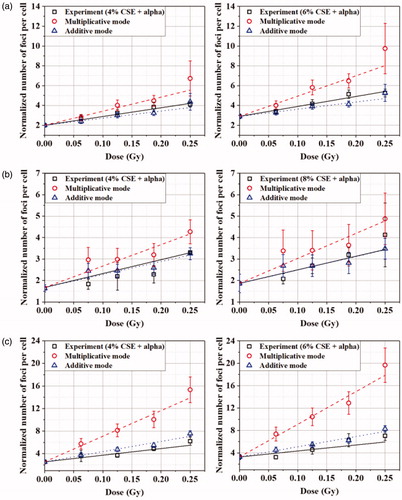
Additive mode: f(a, b)=f(a)+f(b) – 1;
Multiplicative mode: f(a, b)=
with proportional constant k = 1; and
f(x): the number of foci per cell counted under the treatment factor x.
In all three cell lines, the numbers of gamma-H2AX foci per cell from the combined treatment were closer to the additive data. Two-way ANOVA interaction test showed that the interaction between CSE treatment and alpha particle exposure is not significant (p-values from ANOVA >0.75 for all cell lines). In other words, the variation of CSE concentration does not affect the number of gamma-H2AX foci induced by alpha particle exposure, and vice versa.
Clonogenic cell death due to alpha particle exposure and CSE treatment
presents the clonogenic cell death due to alpha particle exposure and CSE treatment. BEAS-2B and SVEC1-10EHR1 cells responded with a reduced clonogenic surviving fraction as the dose of alpha particle exposure and the CSE concentration in culture medium increased. The fitting curves of cell surviving fractions from alpha particle exposure at dose D (Gy) in accorded with the tendency expected in exposure to high linear energy transfer (LET) radiation: SF = exp(-αD) with α =0.791 for BEAS-2B and 0.674 for SVEC4-10EHR1. Those from the CSE treatment in showed the clonogenic cell death that is linearly proportional to the CSE concentration C (%): 1-SF = aC (0 C
1/a) with a = 0.0751 for BEAS-2B and 0.0788 for SVEC4-10EHR1. BEAS-2B cells were slightly more radiosensitive than SVEC4-10EHR1 at high doses but responded to CSE with a similar sensitivity to SVEC4-10EHR1 cells. In Nuli-1 cells, the clones were separated and dispersed in culture medium soon after multiplication without colony formation.
Figure 5. Clonogenic surviving fractions of BEAS-2B and SVEC4-10EHR1 cells after (a) alpha particle exposures at doses of up to 5 Gy and (b, b*) CSE treatments at concentrations of up to 12%. The linearly-fitted surviving fractions (b*) from CSE treatment were depicted in a semi-log scale (b) for direct comparison with those from alpha particle exposure. The error bars represent standard errors.
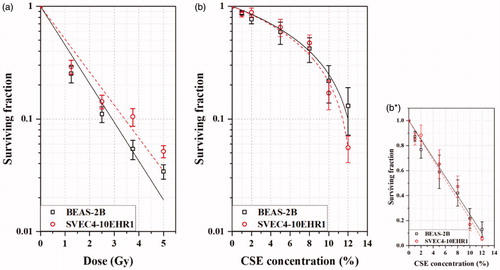
The influence of CSE on the cellular response to alpha particle exposure was investigated by irradiating the cells in the culture medium containing 1.5%, 4%, and 7% of CSE at doses of up to 5 Gy. present the fitting curves of the SF values varying with the dose of alpha particle exposure for BEAS-2B and SVEC4-10EHR1 cells, respectively. The solid lines in are the same as the fitting curves for BEAS-2B and SVEC4-10EHR1 cells in , respectively, which correspond to the CSE-free culture medium. Each broken curve corresponds to one of the CSE concentrations at 1.5%, 4%, and 7%. In , the SF value of each cell line at 0 Gy in the CSE-free culture medium was normalized to 1. For both cell lines, the SF values at 0 Gy are approximately 0.9, 0.7, and 0.5 in the culture media containing 1.5%, 4%, and 7% of CSE, respectively (see ). The SF values were well fitted (R2 ∼ 0.99 when ignoring the standard error of y-intercept) to an individual straight line, exp(−αiD) (i = 1, 2, 3, and 4 for 0, 1.5%, 4%, and 7% of CSE, respectively), even though the slopes (αi’s for i = 1 to 4) are not the same. In , the SF values at 0 Gy were normalized to 1 individually for different CSE concentrations. In both cell lines, no significant differences (p-values from student’s t-test ≫.05) were observed in the decreasing relative SF values with the radiation dose among the sample cells treated with CSE at different concentrations of up to 7%.
Figure 6. The clonogenic surviving fractions of BEAS-2B (a, c) and SVEC4-10EHR1 (b, d) cells, varying with the dose of alpha particle exposure in the CSE-free or CSE-treated culture medium: (a, b) with the SF value at 0 Gy in CSE-free culture medium normalized to 1 and (c, d) with the SF values at 0 Gy normalized to 1 individually for different CSE concentrations in the culture medium. The error bars represent standard errors.
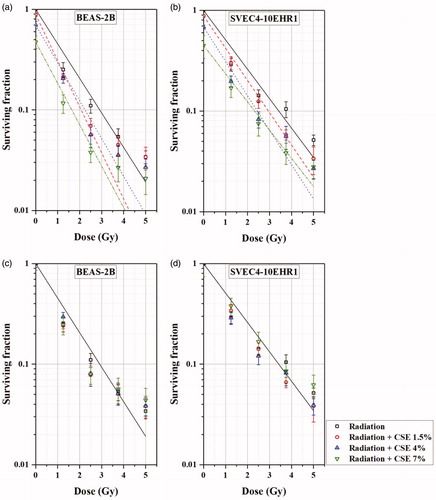
Discussion
Cytotoxicity of alpha particles and CSE
The cytotoxic aspect of CSE was investigated previously in different terms of biological endpoints. CSE inhibits cell division and reduces cell viability (Narayanan and Rao Citation1988; Piao and Hei Citation1993; Baglole et al. Citation2006; Hsu et al. Citation2009). At low concentrations in the culture media, CSE tended to increase cell viability (Baglole et al. Citation2006; Jeon et al. Citation2016). The number of apoptotic cells increased with increasing CSE concentration (Baglole et al. Citation2006; Liu et al. Citation2010). Liu et al. (Citation2005) reported the results of TUNEL assay, stating that CSE does not affect the expression level of apoptosis and the DNA damage produced by CSE is not lethal.
Our gamma-H2AX assay results showed that alpha particles and CSE induced DNA DSB, separately () and together (). The clonogenic cell death was attributed to each alpha particle exposure and CSE treatment () and to the combined effect of both (). Both CSE and alpha particles cause lethal DNA damages.
Influence of CSE treatment on the DNA damage induction via alpha particle exposure
shows that CSE and alpha particles induced DNA DSB in an additive mode rather than in a multiplicative mode in all three cell lines of BEAS-2B, Nuli-1 and SVEC4-10EHR1. The additivity of CSE treatment and alpha particle exposure in DNA DSB production can be attributed to two aspects. First, alpha particles and CSE do not interfere with each other in the process of DNA damage. Ionizing radiation induces DNA damage by directly breaking the atomic bonds in molecules via electron release or by producing reactive oxygen species, which will cause abasic sites and single-strand breaks (Santivasi and Xia Citation2014). CSE affects DNA by forming DNA adducts (Wiencke Citation2002). Second, the radiation-induced gamma-H2AX foci hardly overlapped with the CSE-induced ones because the numbers of gamma-H2AX foci were small in both cases. The additive manner of the production of gamma-H2AX foci was also reported between different radiation types (X-rays and alpha-rays) (Staaf et al. Citation2012).
Influence of CSE treatment on clonogenic cell death under alpha particle exposure
The Valeriote and Carpentier formulas (Valeriote and Lin Citation1975; Carpentier et al. Citation1993; Kuo et al. Citation2012) were applied in evaluating the combined effects of alpha particle exposure (α) and CSE treatment (CSE) as one category out of the following four choices:
synergistic: SFα+CSE<SFα ⋅ SFCSE;
additive: SFα+CSE=SFα ⋅ SFCSE;
sub-additive: SFα+CSE>SFα ⋅ SFCSE, with SFα+CSE<SFα and SFα+CSE<SFCSE; and
protective: SFα+CSE>SFα and/or SFα+CSE>SFCSE;
The isobolograms in were drawn according to the concept of additivity suggested by Steel and Peckham (Citation1979). Five isobolograms correspond to five levels of SF, namely, 10%, 20%, 30%, 40%, and 50%, for each of the BEAS-2B and SVEC4-10EHR1 cell lines. The iso-additive and hetero-additive curves in were formed by reading the parametric conditions (dose of alpha particle exposure and CSE concentration in culture medium) from the fitting curves in . The parametric values resulting in each level of SF under alpha particle exposure in CSE-treated medium are displayed in and marked in . The curves in fitted the experimental data points with R2 > 0.99. The errors attributed to the curve fitting must have propagated to those iso-additive and hetero-additive curves in .
Figure 7. Isobolograms for the target clonogenic survivals of 10 to 50%: BEAS-2B cells (left column) and SVEC4-10EHR1 cells (right column).
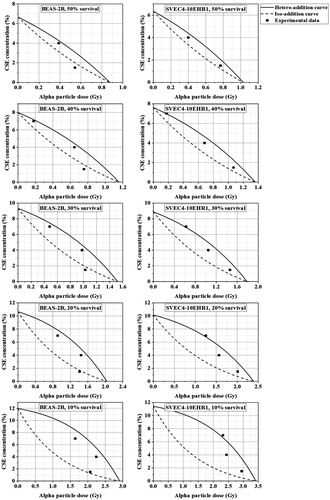
In , the majority of experimental data were confined in the EOA. The data points located outside the EOA belonged to BEAS-2B cells in 1.5% CSE-treated culture media and SVEC4-10EHR1 cells in 7% CSE-treated culture media. The BEAS-2B cells in the culture medium containing 1.5% CSE became more sensitive to alpha particle exposure than those in CSE-free media at doses lower than 2 Gy. The SVEC4-10EHR1 cells in the culture medium containing 7% CSE became slightly less sensitive to alpha particle exposure at all dose levels under observation compared with those in the CSE-free media.
In summary, CSE and alpha particles influenced the clonogenic cell death of BEAS-2B cells in an additive manner. However, CSE at the lowest investigated concentration in culture medium may work synergistically with alpha particles, causing the clonogenic death of BEAS-2B cells. With SVEC4-10EHR1 cells, CSE has an additive influence on the effect of alpha particle exposure on clonogenic cell death, except at the highest investigated concentration where an antagonistic influence was implied. In an additive combined effect, the cells that survived the immediate toxicity of CSE hardly showed any change in radiosensitivity for clonogenic cell death. Considering that CSE and alpha particles induced DNA DSB in an additive manner, the additional clonogenic cell death by the irradiated cells in the CSE-treated culture medium may be due to the additional DNA DSB induced by CSE.
Combined effect in in vitro cell studies versus in animal and epidemiology studies
Cigarette smoke and radiation affect the cells in vitro in an additive way (Narayanan and Rao Citation1988; Piao and Hei Citation1993; Zhou et al. Citation1999). The synergistic action, whether sub-, supra-multiplicative or multiplicative, of cigarette smoking and radiation exposure was observed in epidemiological studies (Moolgavkar et al. Citation1993; Leuraud et al. Citation2011; Tomasek Citation2011; Gilbert et al. Citation2013; Hunter et al. Citation2015; Kreuzer et al. Citation2018). Mauderly et al. (Citation2010) explained in their animal study the supra-additivity of cigarette smoke to alpha particle exposure in causing malignant lung cancer via delayed clearance of the alpha-emitting PuO2 from the lungs due to particulate smoke.
In animal and epidemiology studies, the harmful effect of cigarette smoking on lung cells is diversified depending on the habit of smokers, e.g. swallowing the smoke or not, the size of smoke particulates, and the solubility of smoke particulates in the lung mucosa lining fluid. In in vitro cell experiments, those disturbing factors can be controlled, which is not possible under in vivo exposure conditions. Even though our in vitro observations do not reflect the in vivo action of the body, they have not been complicated either by the randomness involved in the delivery of cigarette smoke or radioactive matter to the critical cells in the lungs.
Cell treatment conditions versus realities
Radiation workers in NORM-handling workplaces will not be allowed to inhale NORM-containing dust particles to induce more than 20 mSv of annual effective dose (ICRP Citation2007). Provided that the lungs are the only organs under radiation exposure, the maximum allowable annual dose to the lungs would be less than 0.2 Sv in equivalent dose with the tissue weighting factor of 0.12 (ICRP Citation2007) applied and less than approximately 0.01 Gy with the alpha weighting factor of 20 (ICRP Citation2007) applied. In our study, the cells were exposed to alpha particles at a minimum of 1.25 Sv in the equivalent dose for several minutes in consideration of the radiation weighting factor of 20 for alpha particles (ICRP Citation2007). Our experiments treated the cells in a much harsher condition in terms of dose level and dose rate.
In our experiments, the cells were tested at the levels of alpha particle exposure that are higher than the level to which radiation workers are commonly exposed. CSE treatment was administered at comparable concentrations (1% to 12% in culture medium) to the actual conditions of heavy smokers. According to SF = exp(−0.791D) (D in Gy) and SF= −0.0751C + 1 (0 C
13.3%) for BEAS-2B human lung epithelial cells, SF ∼0.992 was extrapolated for 0.01 Gy of alpha particle exposure, whereas SF ∼ 0.910 was interpolated for 1.2% CSE environment (corresponding to one pack of cigarettes smoked). Through an additive action, 90.3% of clonogenic survival (SF ∼0.903) will be expected with BEAS-2B from 0.01 Gy of alpha particle exposure while being exposed to 1.2% CSE environment. Exposure to 2.4% CSE (due to two packs of cigarettes smoked) will reduce the clonogenic survival to approximately 81.3%. If the additive action of alpha particles on cigarette smoke is assumed for the lung epithelial cells of radiation workers who are controlled at low dose (<0.01 Gy/yr to the lungs) from internal exposure, the health problem of heavily smoking radiation workers will be caused mainly by smoking rather than by exposure to natural radioactive matter in workplaces. Our observation of the minimal cellular effect from alpha exposure as compared with CSE agrees with the epidemiological data published by Kreuzer et al. (Citation2018) and Zarnke et al. (Citation2019). Kreuzer et al. (Citation2018) showed that smoking increased lung cancer mortality more than cumulative radon exposure over five years. They suggested that smoking induces a higher biological impact on human health in actual internal exposure and smoking conditions. Zarnke et al. (Citation2019) claimed that radon has less health effect than smoking and interpreted that radon alone is not the leading cause of lung cancer, but the interaction of radon with other factors such as cigarette smoke is a key factor.
Effect of radioactive ingredients in CSE
Cigarettes contain natural radioactive ingredients, which are mainly polonium (Po)-210 and lead-210. The radioactivity in one cigarette ranges from several to tens of mBq (Sakoda et al. Citation2012; Taroni et al. Citation2014). Provided that 10 mBq of Po-210 ingredients were dissolved in the CSE we used, the culture medium treated with 10% CSE would contain 0.2 mBq of Po-210 in 200 μl. Simple calculation results using the advanced alpha spectrometric simulation Monte Carlo code (Siiskonen and Pollanen Citation2005) suggested that the cellular dose in the culture medium was approximately 5 nGy/h, which corresponds to 0.12 μGy from 24 h exposure. The influence of radioactive ingredients in CSE on the cellular response will be negligible and was not considered in our data analysis.
Conclusion
In this study, we performed in vitro experiments to investigate the influence of cigarette-smoke exposure on the response of human lung epithelial cells to alpha particle exposure. Evidence suggests that cigarette smoke and alpha particles have an additive effect on lung epithelial cells.
Disclosure statement
The authors report no conflicts of interest. The authors alone are responsible for the content and writing of the paper.
Additional information
Funding
Notes on contributors
Ui-Seob Lee
Mr. Ui-Seob Lee is a Ph.D. candidate in Department of Nuclear Engineering at Seoul National University. He focuses on the radiation biology and dosimetry, especially for internal alpha particle exposure.
Eun-Hee Kim
Professor Eun-Hee Kim is a nuclear engineer with a speciality in radiation protection. She explores new approaches for better understanding the biological effect of radiation. Her research team has built an electron microbeam irradiation system, a hard X-ray irradiation facility and an alpha irradiator for its own research purposes.
References
- Baglole CJ, Bushinsky SM, Garcia TM, Kode A, Rahman I, Sime PJ, Phipps RP. 2006. Differential induction of apoptosis by cigarette smoke extract in primary human lung fibroblast strains: implications for emphysema. Am J Physiol Lung Cell Mol Physiol. 291:L19–L29.
- Carp H, Janoff A. 1978. Possible mechanisms of emphysema in smokers. In vitro suppression of serum elastase-inhibitory capacity by fresh cigarette smoke and its prevention by antioxidants. Am Rev Respir Dis. 118:617–621.
- Carpentier Y, Demange L, Loirette M, Hivet J, Desoize B. 1993. Chronology of combined chemotherapy (5FU) and radiotherapy. I. In vitro study. Anticancer Res. 13:2177–2180.
- Chen W, Xu X, Bai L, Padilla MT, Gott KM, Leng S, Tellez CS, Wilder JA, Belinsky SA, Scott BR, et al. 2012. Low-dose gamma-irradiation inhibits IL-6 secretion from human lung fibroblasts that promotes bronchial epithelial cell transformation by cigarette-smoke carcinogen. Carcinogenesis. 33:1368–1374.
- Egawa H, Furukawa K, Preston D, Funamoto S, Yonehara S, Matsuo T, Tokuoka S, Suyama A, Ozasa K, Kodama K, et al. 2012. Radiation and smoking effects on lung cancer incidence by histological types among atomic bomb survivors. Radiat Res. 178:191–201.
- Furukawa K, Preston D, Lonn S, Funamoto S, Yonehara S, Matsuo T, Egawa H, Tokuoka S, Ozasa K, Kasagi F, et al. 2010. Radiation and smoking effects on lung cancer incidence among atomic-bomb survivors. Radiat Res. 174:72–82.
- Geng H, Zhao L, Liang ZF, Zhang ZQ, Xie DD, Bi LK, Wang Y, Zhang T, Cheng L, Yu DX, et al. 2017. Cigarette smoke extract-induced proliferation of normal human urothelial cells via the MAPK/AP-1 pathway. Oncol Lett. 13:469–475.
- Gilbert ES, Sokolnikov ME, Preston DL, Schonfeld SJ, Schadilov AE, Vasilenko EK, Koshurnikova NA. 2013. Lung cancer risks from plutonium: an updated analysis of data from the Mayak worker cohort. Radiat Res. 179:332–342.
- Health Protection Agency. 2013. Human radiosensitivity: report of the independent advisory group on ionising radiation. RCE-21.
- Hofmann W, Asgharian B. 2003. The effect of lung structure on mucociliary clearance and particle retention in human and rat lungs. Toxicol Sci. 73:448–456.
- Hofmann W, Martonen TB, Menache MG. 1990. A dosimetric model for localised radon progeny accumulations at tracheobronchial bifurcations. Radiat Prot Dosimetry. 30:245–259.
- Hsu CL, Wu YL, Tang GJ, Lee TS, Kou YR. 2009. Ginkgo biloba extract confers protection from cigarette smoke extract-induced apoptosis in human lung endothelial cells: role of heme oxygenase-1. Pulm Pharmacol Ther. 22:286–296.
- Hunter N, Muirhead CR, Bochicchio F, Haylock RG. 2015. Calculation of lifetime lung cancer risks associated with radon exposure, based on various models and exposure scenarios. J Radiol Prot. 35:539–555.
- International Commission on Radiological Protection. 2007. The 2007 recommendations of the international commission on radiological protection: ICRP Publication 103. Ann ICRP. 37:1–332.
- Jacob V, Jacob P, Meckbach R, Romanov SA, Vasilenko EK. 2005. Lung cancer in Mayak workers: interaction of smoking and plutonium exposure. Radiat Environ Biophys. 44:119–129.
- Jeon SY, Go RE, Heo JR, Kim CW, Hwang KA, Choi KC. 2016. Effects of cigarette smoke extracts on the progression and metastasis of human ovarian cancer cells via regulating epithelial-mesenchymal transition. Reprod Toxicol. 65:1–10.
- Kreisheimer M, Sokolnikov ME, Koshurnikova NA, Khokhryakov VF, Romanow SA, Shilnikova NS, Okatenko PV, Nekolla EA, Kellerer AM. 2003. Lung cancer mortality among nuclear workers of the Mayak facilities in the former Soviet union. An updated analysis considering smoking as the main confounding factor. Radiat Environ Biophys. 42:129–135.
- Kreuzer M, Sobotzki C, Schnelzer M, Fenske N. 2018. Factors modifying the radon-related lung cancer risk at low exposures and exposure rates among German uranium miners. Radiat Res. 189:165–176.
- Kuo YC, Lin WC, Chiang IT, Chang YF, Chen CW, Su SH, Chen CL, Hwang JJ. 2012. Sorafenib sensitizes human colorectal carcinoma to radiation via suppression of NF-κB expression in vitro and in vivo. Biomed Pharmacother. 66:12–20.
- Lee KM, Lee US, Kim EH. 2016. A practical alpha particle irradiator for studying internal alpha particle exposure. Appl Radiat Isot. 115:304–311.
- Leuraud K, Schnelzer M, Tomasek L, Hunter N, Timarche M, Grosche B, Kreuzer M, Laurier D. 2011. Radon, smoking and lung cancer risk: results of a joint analysis of three European case-control studies among uranium miners. Radiat Res. 176:375–387.
- Liddell FD, Armstrong BG. 2002. The combination of effects on lung cancer of cigarette smoking and exposure in Quebec chrysotile miners and millers. Ann Occup Hyg. 46:5–13.
- Liu X, Conner H, Kobayashi T, Kim H, Wen F, Abe S, Fang Q, Wang X, Hashimoto M, Bitterman P, et al. 2005. Cigarette smoke extract induces DNA damage but not apoptosis in human bronchial epithelial cells. Am J Respir Cell Mol Biol. 33:121–129.
- Liu Y, Gao W, Zhang D. 2010. Effects of cigarette smoke extract on A549 cells and human lung fibroblasts treated with transforming growth factor-b1 in a coculture system. Clin Exp Med. 10:159–167.
- Madas BG. 2016. Radon exposure and the definition of low doses – the problem of spatial dose distribution. Health Phys. 111:47–51.
- Mauderly JL, Seilkop SK, Barr EB, Gigliotti AP, Hahn FF, Hobbs CH, Finch GL. 2010. Carcinogenic interactions between a single inhalation of 239PuO2 and chronic exposure to cigarette smoke in rats. Radiat Res. 173:665–676.
- Meenakshi C, Mohankumar MN. 2013. Synergistic effect of radon in blood cells of smokers – an in vitro study. Mutat Res. 757:79–82.
- Monchaux G, Morlier JP, Morin M, Chameaud J, Lafuma J, Masse R. 1994. Carcinogenic and cocarcinogenic effects of radon and radon daughters in rats. Environ Health Perspect. 102:64–73.
- Moolgavkar SH, Luebeck G, Krewski D, Zielinski JM. 1993. Radon, cigarette smoke, and lung cancer: a re-analysis of the Colorado plateau uranium miners’ data. Epidemiology. 4:204–217.
- Narayanan NS, Rao BS. 1988. Interaction between cigarette smoke condensate and radiation for the induction of genotoxic effects in yeast. Mutat Res. 208:45–49.
- National Research Council. 1991. Comparative dosimetry of radon in mines and homes – 8. Cells of origin for lung cancer. Washington (DC): The National Academies Press.
- National Research Council. 1999. Health effects of exposure to radon: BEIR VI. Washington (DC): The National Academies Press.
- Nishioka T, Yamamoto D, Zhu T, Guo J, Kim SH, Chen CY. 2011. Nicotine overrides DNA damage-induced G1/S restriction in lung cells. PloS One. 6:e18619.
- Pershagen G, Akerblom G, Axelson O, Clavensjo B, Damber L, Desai G, Enflo A, Lagarde F, Mellander H, Svartengren M, et al. 1994. Residential radon exposure and lung cancer in Sweden. N Engl J Med. 330:159–164.
- Piao CQ, Hei TK. 1993. The biological effectiveness of radon daughter alpha particles. I. Radon, cigarette smoke and oncogenic transformation. Carcinogenesis. 14:497–501.
- Pierce DA, Sharp GB, Mabuchi K. 2003. Joint effects of radiation and smoking on lung cancer risk among atomic bomb survivors. Radiat Res. 159:511–520.
- Sakoda A, Fukao K, Kawabe A, Kataoka T, Hanamoto K, Yamaoka K. 2012. Radioactivity of 210Pb in Japanese cigarettes and radiation dose from smoking inhalation. Radiat Prot Dosimetry. 150:109–113.
- Santivasi WL, Xia F. 2014. Ionizing radiation-induced DNA damage, response, and repair. Antioxid Redox Signal. 21:251–259.
- Siiskonen T, Pollanen R. 2005. Advanced simulation code for alpha spectrometry. Nucl Instrum Meth A. 550:425–434.
- Staaf E, Brehwens K, Haghdoost S, Czub J, Wojcik A. 2012. Gamma-H2AX foci in cells exposed to a mixed beam of x-rays and alpha particles. Genome Integr. 3:8.
- Steel GG, Peckham MJ. 1979. Exploitable mechanisms in combined radiotherapy-chemotherapy: the concept of additivity. Int J Radiat Oncol Biol Phys. 5:85–91.
- Sutherland KD, Berns A. 2010. Cell of origin of lung cancer. Mol Oncol. 4:397–403.
- Taroni M, Zaga V, Bartolomei P, Gattavecchia E, Pacifici R, Zuccaro P, Esposito M. 2014. 210Pb and 210Po concentrations in Italian cigarettes and effective dose evaluation. Health Phys. 107:195–199.
- Tomasek L. 2011. Interaction of radon and smoking among Czech uranium miners. Radiat Prot Dosimetry. 145:238–242.
- Tomasek L. 2013. Lung cancer risk from occupational and environmental radon and role of smoking in two Czech nested case-control studies. Int J Environ Res Public Health. 10:963–979.
- Truta-Popa LA, Hofmann W, Cosma C. 2011. Prediction of lung cancer risk for radon exposures based on cellular alpha particle hits. Radiat Prot Dosimetry. 145:218–223.
- Wiencke JK. 2002. DNA adduct burden and tobacco carcinogenesis. Oncogene. 21:7376–7391.
- Valeriote F, Lin HS. 1975. Synergistic interaction of anticancer agents: a cellular perspective. Cancer Chemother Rep. 59:895–900.
- Zarnke AM, Tharmalingam S, Boreham DR, Brooks AL. 2019. BEIR VI radon: the rest of the story. Chem Biol Interact. 301:81–87.
- Zhang R, Li J, Burns FJ, Huang C. 2006. Ionizing radiation synergistic induction of cyclooxygenase-2 with benzo[a]pyrene diol-epoxide through nuclear factor of activated T cells in mouse epidermal CI41 cells. Oncol Repl. 15:721–727.
- Zhou H, Zhu LX, Li K, Hei TK. 1999. Radon, tobacco-specific nitrosamine and mutagenesis in mammalian cells. Mutat Res. 430:145–153.