Abstract
Purpose
Lymphopenia is now generally recognized as a negative prognostic factor in radiotherapy. Already at the beginning of the century we demonstrated that high-energy carbon ions induce less damage to the lymphocytes of radiotherapy patients than X-rays, even if heavy ions are more effective per unit dose in the induction of chromosomal aberrations in blood cells irradiated ex-vivo. The explanation was based on the volume effect, i.e. the sparing of larger volumes of normal tissue in Bragg peak therapy. Here we will review the current knowledge about the difference in lymphopenia between particle and photon therapy and the consequences.
Conclusions
There is nowadays an overwhelming evidence that particle therapy reduces significantly the radiotherapy-induced lymphopenia in several tumor sites. Because lymphopenia turns down the immune response to checkpoint inhibitors, it can be predicted that particle therapy may be the ideal partner for combined radiation and immunotherapy treatment and should be selected for patients where severe lymphopenia is expected after X-rays.
Introduction
Among the side effect of cancer radiotherapy, the decrease of number of lymphocytes counted in circulating blood has been recognized for over half a century. It was also soon recognized that lymphopenia is a significant risk for cancer patients, as it leads to immunosuppression that promote infection (Campbell et al. Citation1973) or development of distal metastasis (Stjernswärd et al. Citation1972).
It was, however, only in recent years that lymphopenia was recognized as a major negative prognostic factor in radiotherapy. Silvia Formenti and Sandra DeMaria had for many years noted that radiation can have immunostimulatory functions, i.e. can act as a cancer vaccine (Demaria et al. Citation2004; Formenti and Demaria Citation2009) as demonstrated by the abscopal effect (Abuodeh et al. Citation2016) occasionally observed during radiotherapy of metastatic patients. The game changer was certainly the introduction of immunotherapy with checkpoint inhibitors in 2011 (Sharma and Allison Citation2015; Chamoto et al. Citation2023; Sharma et al. Citation2023) that led to the 2018 Nobel Prize in Physiology and Medicine to James P. Allison and Tasuku Honjo. While immunotherapy quickly became a leading cancer treatment (Kirkwood et al. Citation2012), it was also recognized that it would be an ideal partner of radiotherapy to boost the abscopal effect (Postow et al. Citation2012; Formenti and Demaria Citation2013; Galluzzi et al. Citation2023).
Therefore, about 10 years ago the topic of radiation-induced immune response became extremely popular in radiotherapy and basic radiobiology, even naming it the 6th R of radiotherapy (Boustani et al. Citation2019). An immune response would only be possible if the natural immune system of the patient, challenged by cytotoxic treatments such as chemo- and radio-therapy, is preserved to the point of being able to exploit the administration of checkpoint inhibitors. Immune system in humans consists of approximately 1.8·× 1012 cells, and 40% are lymphocytes (Sender et al. Citation2023).
Several clinical studies started to test the prognostic value of radiation-induced immune system damage. Most of these studies clearly show that lymphopenia is a negative prognostic factor in radiotherapy for many different tumor sites (Sherry et al. Citation2020; Damen et al. Citation2021; Iorio et al. Citation2021; Terrones-Campos et al. Citation2021; Dai et al. Citation2022; El Houat et al. Citation2023). A meta-analysis of radiation-induced lymphopenia in all solid tumors shows that nearly 50% of the patients have a risk of developing ≥ G3 lymphopenia with and hazard ratio for overall survival ranging 1.5–3.0 depending on the tumor site (de Kermenguy et al. Citation2023). The clinical data therefore support the hypothesis that circulating T-cells, bone marrow and lymph nodes should be treated as organ-at-risk in radiotherapy.
The concern on lymphocyte sparing in radiotherapy begs the question on how can we limit the dose to lymphocyte-rich structures (Venkatesulu et al. Citation2022). Mathematical models suggested that increasing dose-rate and hypofractionation spare circulating lymphocytes, but the greatest factor is the volume effect (Yovino et al. Citation2013). Obviously a reduced integral dose will reduce the dose to both circulating lymphocytes, lymph nodes, and bone marrow. The reduced integral dose is the main hallmark of particle therapy, thanks to the depth-dose distribution described by the Bragg curve (Durante and Paganetti Citation2016) (). In this paper we will show how the evidence of sparing of lymphocytes in particle therapy was accumulated, and how it can be exploited in future trials.
Figure 1. Comparison of treatment plans with X-rays and protons, showing normal tissue sparing in every tumors site. Figure from (Durante et al. Citation2019), reproduced with permission of elsevier.
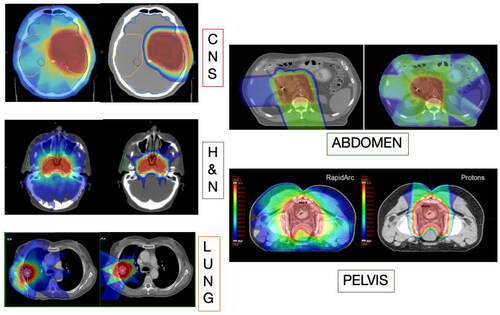
Chromosomal aberrations in lymphocytes
Induction of chromosomal aberrations in human peripheral blood lymphocytes has been studied for many decades. The measurements were used to unravel basic mechanisms of radiation action (Durante et al. Citation2013), biodosimetry (IAEA Citation2011), and for estimating quality factors in radiation protection (Harrison et al. Citation2021). During my postdoc at the NASA Johnson Space Center in Houston (TX, USA) in the 90s, I was studying chromosome aberrations by fluorescence in situ hybridization (FISH) for biodosimetry in astronauts (Yang et al. Citation1997). In fact, at that time we had excellent physical models for the prediction of the equivalent dose in space missions, but we could not know whether the space environment (particularly microgravity) and the exposure to the mixed particle field of the galactic cosmic radiation were changing the effect of a given dose. We also had a very large uncertainty on the quality factors of the heavy ions in the cosmic rays for late effects such as cancer (Durante and Cucinotta Citation2008). For these reasons, we used chromosome aberrations in lymphocytes as a biomarker of risk in space (Durante et al. Citation2001; Durante Citation2005).
When I moved from USA to the National Institute of Radiological Sciences (NIRS), now Quantum Science technology (QST), in Chiba (Japan), I decided to apply this technique to radiotherapy patients. At that time, NIRS-QST was the only clinics worldwide treating patients with carbon ions accelerated at the HIMAC synchrotron (Noda et al. Citation2017). NIRS-QST is still today the center with the most extensive experience in 12C-ion therapy and has achieved excellent results for many tumor sites (Kamada et al. Citation2015).
At that time, we knew very well that high-LET heavy ions were more effective than X-rays per unit dose in the induction of chromosome aberrations in ex-vivo human peripheral blood lymphocytes (Barendsen Citation1979; Wu et al. Citation1997; George et al. Citation2003; Di Giorgio et al. Citation2004) (). I wanted to compare high- vs. low-LET damage n vivo, exploiting the ongoing treatments at NIRS-QST with accelerated C-ions. One problem with heavy ions is the prolonged radiation-induced cell cycle delay that leads to an underestimation of the damage when mitotic cells are harvested at a given time point (Ritter et al. Citation2002). For this reason, Eisuke Gotoh and myself developed a new protocol that used the phosphatase inhibitor calyculin A to visualize chromosome in the G2-phase of the cell cycle (Durante et al. Citation1998; Gotoh and Durante Citation2006). We decided to apply this technique, combined with FISH, to the lymphocytes of the patients treated with carbon ions for esophageal or uterus cancer ().
Figure 2. In vitro dose response for the induction of structural aberrations in peripheral blood lymphocyte chromosomes 2 and 4 visualized by FISH-painting. Data for X-rays are average from 11 different patient samples, data for C-ion are average from 3 different donors. Blood was exposed ex-vivo to 200 kVp X-rays or carbon ions accelerated at HIMAC in two different positions of the Spread-out-Bragg-peak (SOBP) corresponding to the entrance channel (LET = 13 keV/µm) and the distal edge of the SOBP (LET= 83 keV/µm). Bars are standard errors of the mean values. Curves are best-fits to the data point by linear-quadratic functions. RBE values at a 2 Gy X-ray dose (15% aberrant cells) are about 1.3 for the Plateau C-ions and 4.0 for the Bragg peak ions. The inset in top-right shows a 290 MeV/n C-ion SOBP in water. Both the physical dose (left axis) and dose-averaged LET (right axis) are plotted vs. depth in the patient body. The arrows point to the position corresponding to the LET values where chromosome aberrations were measured. Figure modified from reference (Durante et al. Citation2000), reproduced with permission of Elsevier.
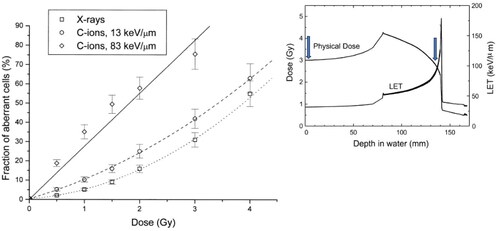
Figure 3. Cytogenetic techniques used to visualize the damage in human peripheral blood lymphocytes in patients during radiotherapy course with either X-rays or C-ion. In the original paper (Durante et al. Citation2000), we used FISH-painting of chromosomes 2 and 4. The panel a shows a dicentric and corresponding acentric fragment involving both chromosomes 2. Later studies involved multi-color FISH (Hartel et al. Citation2010) and mBAND (Pignalosa et al. Citation2013). Translocations involving chromosomes (1,7), (11, 29 and (20,4) are shown in panel B. An inversion in chromosome 2 visualized by mBAND is shown in panel C.
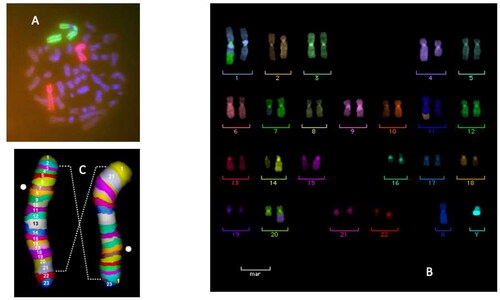
We collected blood samples at different time points before and during the treatment and measured the chromosomal aberrations and the number of lymphocytes in the circulating blood. Because we knew very well that chromosome aberrations are strongly correlated to cell death (Dewey et al. Citation1971; Bedford et al. Citation1978; Cornforth and Bedford Citation1993), we expected that the fraction of aberrant lymphocytes would be an excellent marker of lymphopenia. We compared patients treated with accelerated C-ions and conventional X-rays having tumor of similar size. The results were surprising: C-ion patients had a lower level of chromosome aberrations () and reduced lymphopenia () compared to X-ray patients (Durante et al. Citation2000). If we compare and we see that the results n vivo were opposite to those ex-vivo. The explanation is the volume effect. Because of the large sparing of normal tissue after particle therapy compared to X-rays (), less lymphocytes are exposed and therefore the damage is reduced. The level of chromosome aberrations after 10 Gy was well correlated with the nadir in absolute lymphocyte counts (ALC), showing that the chromosome aberration results correlates with lymphopenia already after 1 week treatment. Supporting these old experimental data, a recent neural network, AI-driven model trained on lung cancer patients found that ALC after 1 week treatment predicts G4 lymphopenia with high statistical significance (Kim et al. Citation2023).
Figure 4. Maximum yield of chromosome aberrations in radiotherapy patients treated in Japan for esophageal or uterus cancer. The fraction of lymphocytes with aberrations in chromosomes 2 or 4 at the end of the treatment is plotted here. Bars are standard deviations calculated on 2 patients each for esophageal cancer, 3 patients treated with X-rays for uterus cancer, and 4 patients treated with C-ions for uterus cancer. Data are available in reference Durante et al. (Citation2000).
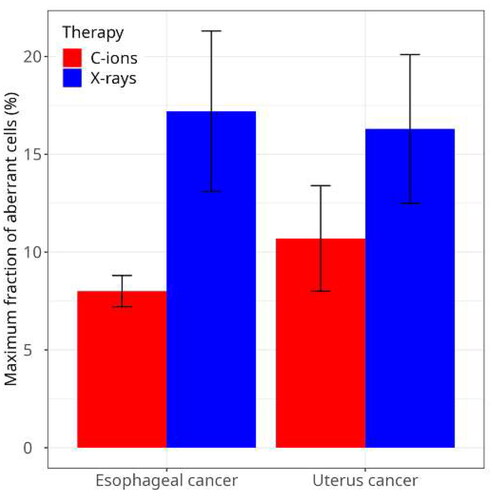
Figure 5. Absolute lymphocyte counts (ALC) in patients treated with X-rays or C-ions for esophageal cancer. Fractionation is 1.8 Gy/f for X-.ray patients, and 2.7 Gy(RBE)/f for C-ion patients. Bars are standard errors of the mean values on 4 patients with C-ions and 15 patients treated with C-ions. Data are available in reference Durante et al. (Citation2000).
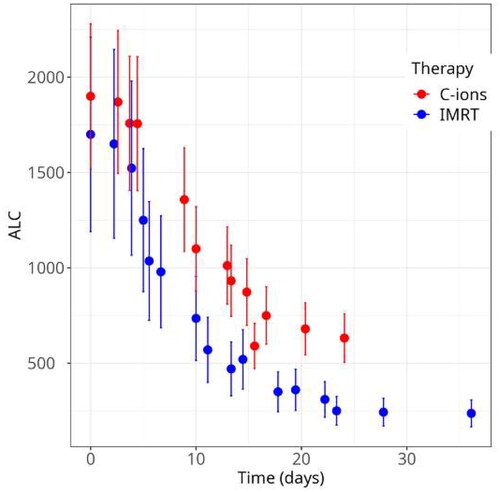
We showed the importance of the volume effect in esophageal cancer patients treated with X-rays. For those patients, we measured an increase of the aberrations and decrease of ALC proportional to the radiation field size (Durante et al. Citation1999; Yamada et al. Citation2000). Around 40% of the immune cells in our body are in the lymph nodes, while only about 2% is present at a certain time in the circulating blood (Sender et al. Citation2023). During X-ray treatment for esophageal cancer, many white blood cells are irradiated in mediastinal lymph nodes that run along the central chest, making the correlation with the large field size very strong for this type of tumor. In breast cancer, the yield of chromosome aberrations in patients receiving radiotherapy post-quadrantectomy was reduced when axillary lymph nodes were surgically removed before radiotherapy (D’Alesio et al., Citation2003), again showing that a large fraction of lymphocytes detected in circulating blood was actually exposed while in the lymph nodes. The same correlation between field size and aberrations was found in lung cancer patients treated with C-ions (Lee et al. Citation2004) ().
Figure 6. In patients treated with C-ions for NSCLC at HIMAC, we showed that the fraction of aberrant lymphocytes was correlated to both clinical target volume and minimum number of lymphocytes counted at the end of the treatment. Here we plot the ALC nadir, expressed as fraction of the pretreatment ALC, vs. the target volume. Original data in Lee et al. (Citation2004) present a large scatter. Here a general data fit is reported that shows the correlation using a log-scale.
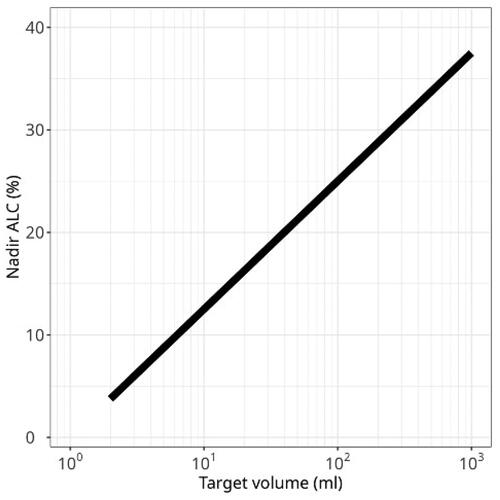
When in 2006 I moved to Germany to work at the GSI Helmholtz Center in Darmstadt, I wanted to expand those studies with the new techniques of multiplex fluorescence n situ hybridization (mFISH) and multicolor banding (mBAND) () in collaboration with the Heidelberg Ion Therapy (HIT) center, where patients were treated with C-ions and/or intensity-modulated radiotherapy (IMRT) for prostate cancer (Nikoghosyan et al. Citation2011). The more sophisticated cytogenetic techniques confirmed the Japanese results – i.e. a lower yield of aberrations with carbon ions compared to IMRT and a strong dependence on the irradiated volume (Hartel et al. Citation2010; Pignalosa et al. Citation2013).
Particle therapy and lymphopenia
The main results of the chromosome aberration studies at the beginning of this century can be summarized as follows:
Radiotherapy induces lymphopenia
The severity of lymphopenia strongly depends on the field size and on the presence of lymph nodes in the field
Particle therapy reduces lymphopenia compared to IMRT
As noted in the Introduction, these results were not very much considered until immunotherapy entered the clinics. Not only it was shown that lymphopenia was a negative prognostic factor for radiotherapy, but it also reduces the effectiveness of immunotherapy (Chen et al. Citation2020; Takanen et al. Citation2022; Yang et al. Citation2023; Zhang et al. Citation2023). Because immunotherapy is usually administered in combination with other cytotoxic therapies (Upadhaya et al. Citation2022), a great attention was finally devoted to therapeutical approaches that minimize lymphopenia. For this very reason, I proposed with Silvia Formenti and David J. Brenner already in 2016 that particle therapy could be the ideal partner for immunotherapy (Durante et al. Citation2016). The first reason is that particle therapy will spare more lymphocytes because of its physical properties (). This hypothesis has now been confirmed in several trials for cancer in esophagus, lung, liver, and brain (). All these trials show lymphocyte sparing with particles (protons or C-ions) compared to X-rays. Obviously other factors including fractionation, dose-rate, dose-volume histograms to lymphoid organs, dose to circulating blood, total irradiated volume, co-morbidities etc. play a role, but in any case charged particles offer the opportunity to spare immune cells better.
Table 1. Summary of the clinical evidence that particle therapy reduces lymphopenia compared to X-ray radiotherapy.
Sparing lymphocytes seems to be such an important prognostic factor that it could be used to select patients for particle therapy. Several biophysical models to predict radiotherapy-induced lymphopenia are now under study (Cella et al. Citation2024; Cho et al. Citation2022; Kim S et al. Citation2023; Kim Y et al. Citation2023; Takeda et al. Citation2023; van Rossum et al. Citation2023). When these model will be validated and accepted by the medical community, it would be important to use them to direct patients to particle therapy if the model predicts severe lymphopenia with IMRT.
A second argument in favor of heavy ions when combined to immunotherapy is the potential for increased antigenicity and adjuvanticity suggested in pre-clinical radiobiological studies (Durante and Formenti Citation2020; Helm et al. Citation2023, Citation2022). The differential biological effects are more pronounced with C-ions than for protons, because of the higher LET of the heavy ions that triggers different biological response pathways (Durante et al. Citation2021). Some outstanding clinical results with C-ions in tumors with high metastatic potential, such as locally advanced pancreas cancer (Kawashiro et al. Citation2018), suggest that heavy ions elicit a stronger immune response.
Conclusions
It is nowadays clear that lymphocyte-rich organs should be treated as organs-at-risk to elicit an adequate immune response in cancer patients. Already at the beginning of this century, our study on Japanese cancer patients treated with X-rays or C-ions showed that particle therapy can spare lymphocytes much more efficiently than X-rays (Durante et al. Citation2000). This early observation has been recently confirmed in several clinical trials (). It can be therefore safely stated that reducing lymphopenia is a major advantage of particle therapy compared to conventional X-rays, especially when radiotherapy is combined to immunotherapy. Nevertheless, clinical evidence that the combination of particles and immunotherapy can lead to better results than IMRT is still missing. Some clinical trials where IMRT was combined to immune checkpoint inhibitors gave excellent results (Antonia et al. Citation2018; Kelly et al. Citation2021; Spigel et al. Citation2022; Chang et al. Citation2023) but other were disappointing (Lee et al. Citation2021; Lim et al. Citation2022; Schoenfeld et al. Citation2022; Tao et al. Citation2023). There are several possible reasons that may explain why so many trials failed, but one hypothesis is indeed the inactivation of the lymphocytes induced by regional node irradiation (Rajeev-Kumar and Pitroda Citation2023). From the discussion above, the hypothesis that particle therapy will lead to better results than X-rays in combination with immunotherapy is reasonable and warrants verification in clinical settings. A few trials are currently undergoing including proton therapy following durvalumab/tremelimumab in recurrent or metastatic head and neck patients (H. Kim et al. Citation2023) and carbon ions for patients in stable disease after pembrolizumab (Cavalieri et al. Citation2023). On the Clinicaltrials.gov website, 41 trials are listed as actively recruiting patients for treatments with proton therapy and checkpoint inhibitors. It is therefore likely that soon we will have clinical evidence of the advantage of sparing lymphocytes when radiotherapy is combined to immunotherapy.
Acknowledgements
I am very grateful to IARR for their decision to select me as recipient of the prestigious Kaplan award. This is a great honour, and looking at the list of previous winners makes me feel amazed. I also thank the Italian (SIRR) and German (DeGBS) Societies of Radiation Research for their nomination and support. I would have a very long list of friends and colleagues that helped me in my career and to whom I owe this award, but the list would be definitely too long. I have to mention though Kerry George, Mariagabriella Pugliese and all my team at the GSI Helmholtz Center in Darmstadt. This paper is dedicated to the memory of my mentor and guide, Prof. Gerhard Kraft, who passed away on March 18, 2023, and is sorely missed.
Disclosure statement
No potential conflict of interest was reported by the author(s).
Additional information
Funding
Notes on contributors
Marco Durante
Marco Durante is Head of the Biophysics Department at the GSI Helmholtzzentrum für Schwerionenforschung in Darmstadt (Germany) and professor pf Physics at the Technische Universität Darmstadt (Germany) and University Federico II in Naples (Italy). He received the Kaplan award at the 17th International Congress for Radiation Research in Montreal (Canada), August 27–30, 2023.
References
- [IAEA] International Atomic Energy Agency. 2011. Cytogenetic dosimetry : applications in preparedness for and response to radiation emergencies. Manual Series. Vienna: International Atomic Energy Agency.
- Abuodeh Y, Venkat P, Kim S. 2016. Systematic review of case reports on the abscopal effect. Curr Probl Cancer. 40(1):25–37. doi:10.1016/j.currproblcancer.2015.10.001
- Antonia SJ, Villegas A, Daniel D, Vicente D, Murakami S, Hui R, Kurata T, Chiappori A, Lee KH, de Wit M, et al. 2018. Overall survival with durvalumab after chemoradiotherapy in stage III NSCLC. N Engl J Med. 379(24):2342–2350. doi:10.1056/NEJMoa1809697
- Barendsen GW. 1979. Influence of radiation quality on the effectiveness of small doses for induction of reproductive death and chromosome aberrations in mammalian cells. Int J Radiat Biol Relat Stud Phys Chem Med. 36(1):49–63. doi:10.1080/09553007914550811
- Bedford JS, Mitchell JB, Griggs HG, Bender MA. 1978. Radiation-induced cellular reproductive death and chromosome aberrations. Radiat Res. 76(3):573–586.
- Boustani J, Grapin M, Laurent P-A, Apetoh L, Mirjolet C. 2019. The 6th R of radiobiology: reactivation of anti-tumor immune response. Cancers (Basel). 11(6):860. doi:10.3390/cancers11060860
- Campbell AC, Hersey P, MacLennan ICM, Kay HEM, Pike MC. 1973. Immunosuppressive consequences of radiotherapy and chemotherapy in patients with acute lymphoblastic leukaemia. Br Med J. 2(5863):385–388. doi:10.1136/bmj.2.5863.385
- Cavalieri S, Vitolo V, Barcellini A, Ronchi S, Facoetti A, Campo C, Klersy C, Molinelli S, Agustoni F, Ferretti VV, et al. 2023. Immune checkpoint inhibitors and Carbon iON radiotherapy in solid Cancers with stable disease (ICONIC). Future Oncol. 19(3):193–203. doi:10.2217/fon-2022-0503
- Cella L, Monti S, Pacelli R, Palma G. 2024. Modeling frameworks for radiation induced lymphopenia: a critical review. Radiother Oncol. 190:110041. doi:10.1016/j.radonc.2023.110041
- Chamoto K, Yaguchi T, Tajima M, Honjo T. 2023. Insights from a 30-year journey: function, regulation and therapeutic modulation of PD1. Nat Rev Immunol. 23(10):682–695. doi:10.1038/s41577-023-00867-9
- Chang JY, Lin SH, Dong W, Liao Z, Gandhi SJ, Gay CM, Zhang J, Chun SG, Elamin YY, Fossella FV, et al. 2023. Stereotactic ablative radiotherapy with or without immunotherapy for early-stage or isolated lung parenchymal recurrent node-negative non-small-cell lung cancer: an open-label, randomised, phase 2 trial. Lancet. 402(10405):871–881. doi:10.1016/S0140-6736(23)01384-3
- Chen D, Verma V, Patel RR, Barsoumian HB, Cortez MA, Welsh JW. 2020. Absolute lymphocyte count predicts abscopal responses and outcomes in patients receiving combined immunotherapy and radiation therapy: analysis of 3 phase 1/2 trials. Int J Radiat Oncol Biol Phys. 108(1):196–203. doi:10.1016/j.ijrobp.2020.01.032
- Cho Y, Kim Y, Chamseddine I, Lee WH, Kim HR, Lee IJ, Hong MH, Cho BC, Lee CG, Cho S, et al. 2022. Lymphocyte dynamics during and after chemo-radiation correlate to dose and outcome in stage III NSCLC patients undergoing maintenance immunotherapy. Radiother Oncol. 168:1–7. doi:10.1016/j.radonc.2022.01.007
- Cornforth MN, Bedford JS. 1993. Ionizing radiation damage and its early development in chromosomes. Adv Radiat Biol. 17:423–496. doi:10.1016/B978-0-12-035417-7.50010-6
- Cortiula F, Hendriks LEL, Wijsman R, Houben R, Steens M, Debakker S, Canters R, Trovò M, Sijtsema NM, Niezink AGH, et al. 2024. Proton and photon radiotherapy in stage III NSCLC: Effects on hematological toxicity and adjuvant immune therapy. Radiother Oncol. 190:110019. doi:10.1016/j.radonc.2023.110019
- d’Alesio V, Pacelli R, Durante M, Canale Cama G, Cella L, Gialanella G, Grossi G, Pugliese M, Punzo G, Sardi I, et al. 2003. Lymph nodes in the irradiated field influence the yield of radiation-induced chromosomal aberrations in lymphocytes from breast cancer patients. Int J Radiat Oncol Biol Phys. 57(3):732–738., doi:10.1016/S0360-3016(03)00664-3
- Dai D, Tian Q, Yu G, Shui Y, Jiang H, Wei Q. 2022. Severe radiation-induced lymphopenia affects the outcomes of esophageal cancer: a comprehensive systematic review and meta-analysis. Cancers (Basel). 14(12):3024. doi:10.3390/cancers14123024
- Damen PJJ, Kroese TE, van Hillegersberg R, Schuit E, Peters M, Verhoeff JJC, Lin SH, van Rossum PSN. 2021. The influence of severe radiation-induced lymphopenia on overall survival in solid tumors: a systematic review and meta-analysis. Int J Radiat Oncol Biol Phys. 111(4):936–948. doi:10.1016/j.ijrobp.2021.07.1695
- De B, Ng SP, Liu AY, Avila S, Tao R, Holliday EB, Brownlee Z, Kaseb A, Lee S, Raghav K, et al. 2021. Radiation-associated lymphopenia and outcomes of patients with unresectable hepatocellular carcinoma treated with radiotherapy. J Hepatocell Carcinoma. 8:57–69. doi:10.2147/JHC.S282062
- de Kermenguy F, Meziani L, Mondini M, Clémenson C, Morel D, Deutsch E, Robert C. 2023. Radio-induced lymphopenia in the era of anti-cancer immunotherapy. Int Rev Cell Mol Biol. 378:1–30. doi:10.1016/bs.ircmb.2023.03.002
- Demaria S, Ng B, Devitt ML, Babb JS, Kawashima N, Liebes L, Formenti SC. 2004. Ionizing radiation inhibition of distant untreated tumors (abscopal effect) is immune mediated. Int J Radiat Oncol Biol Phys. 58(3):862–870. doi:10.1016/j.ijrobp.2003.09.012
- Dewey WC, Miller HH, Leeper DB. 1971. Chromosomal aberrations and mortality of x-irradiated mammalian cells: emphasis on repair. Proc Natl Acad Sci U S A. 68(3):667–671. doi:10.1073/pnas.68.3.667
- Di Giorgio M, Edwards AA, Moquet JE, Finnon P, Hone PA, Lloyd DC, Kreiner AJ, Schuff JA, Taja MR, Vallerga MB, et al. 2004. Chromosome aberrations induced in human lymphocytes by heavy charged particles in track segment mode. Radiat Prot Dosimetry. 108(1):47–53. doi:10.1093/rpd/nch012
- Durante M. 2005. Biomarkers of space radiation risk. Radiat Res. 164(4 Pt 2):467–473. doi:10.1667/rr3359.1
- Durante M, Bedford JS, Chen DJ, Conrad S, Cornforth MN, Natarajan AT, van Gent DC, Obe G. 2013. From DNA damage to chromosome aberrations: Joining the break. Mutat Res. 756(1-2):5–13. doi:10.1016/j.mrgentox.2013.05.014
- Durante M, Bonassi S, George K, Cucinotta FA. 2001. Risk estimation based on chromosomal aberrations induced by radiation. Radiat Res. 156(5 Pt 2):662–667. doi:10.1667/0033-7587(2001)156[0662:reboca[PMC]2.0.co;2]
- Durante M, Brenner DJ, Formenti SC. 2016. Does heavy ion therapy work through the immune system? Int J Radiat Oncol Biol Phys. 96(5):934–936. doi:10.1016/j.ijrobp.2016.08.037
- Durante M, Cucinotta FA. 2008. Heavy ion carcinogenesis and human space exploration. Nat Rev Cancer. 8(6):465–472. doi:10.1038/nrc2391
- Durante M, Debus J, Loeffler JS. 2021. Physics and biomedical challenges of cancer therapy with accelerated heavy ions. Nat Rev Phys. 3(12):777–790. doi:10.1038/s42254-021-00368-5
- Durante M, Formenti S. 2020. Harnessing radiation to improve immunotherapy: better with particles? Br J Radiol. 93(1107):20190224. doi:10.1259/bjr.20190224
- Durante M, Furusawa Y, Gotoh E. 1998. A simple method for simultaneous interphase-metaphase chromosome analysis in biodosimetry. Int J Radiat Biol. 74(4):457–462. doi:10.1080/095530098141320
- Durante M, Golubev A, Park W-Y, Trautmann C. 2019. Applied nuclear physics at the new high-energy particle accelerator facilities. Phys Rep. 800:1–37. doi:10.1016/j.physrep.2019.01.004
- Durante M, Paganetti H. 2016. Nuclear physics in particle therapy : a review. Rep Prog Phys. 79(9):096702. doi:10.1088/0034-4885/79/9/096702
- Durante M, Yamada S, Ando K, Furusawa Y, Kawata T, Majima H, Nakano T, Tsujii H. 2000. X-rays vs. carbon-ion tumor therapy: cytogenetic damage in lymphocytes. Int J Radiat Oncol Biol Phys. 47(3):793–798. doi:10.1016/S0360-3016(00)00455-7
- Durante M, Yamada S, Ando K, Furusawa Y, Kawata T, Majima H, Nakano T, Tsujii H. 1999. Measurements of the equivalent whole-body dose during radiation therapy by cytogenetic methods. Phys Med Biol. 44(5):1289–1298. doi:10.1088/0031-9155/44/5/314
- El Houat Y, Massard C, Quillien V, de Crevoisier R, Castelli J. 2023. Meta-analysis and critical review: association between radio-induced lymphopenia and overall survival in solid cancers. Adv Radiat Oncol. 8(2):101038. doi:10.1016/j.adro.2022.101038
- Formenti SC, Demaria S. 2013. Combining radiotherapy and cancer immunotherapy: a paradigm shift. J Natl Cancer Inst. 105(4):256–265. doi:10.1093/jnci/djs629
- Formenti SC, Demaria S. 2009. Systemic effects of local radiotherapy. Lancet Oncol. 10(7):718–726. doi:10.1016/S1470-2045(09)70082-8
- Friedes C, Iocolano M, Lee SH, Duan L, Li B, Doucette A, Cohen RB, Aggarwal C, Sun LL, Levin WP, et al. 2024. The effective radiation dose to immune cells predicts lymphopenia and inferior cancer control in locally advanced NSCLC. Radiother Oncol. 190:110030. doi:10.1016/j.radonc.2023.110030
- Galluzzi L, Aryankalayil MJ, Coleman CN, Formenti SC. 2023. Emerging evidence for adapting radiotherapy to immunotherapy. Nat Rev Clin Oncol. 20(8):543–557. doi:10.1038/s41571-023-00782-x
- George K, Durante M, Willingham V, Wu H, Yang TC, Cucinotta F. a 2003. Biological effectiveness of accelerated particles for the induction of chromosome damage measured in metaphase and interphase human lymphocytes. Radiat Res. 160(4):425–435. doi:10.1667/RR3064
- Gotoh E, Durante M. 2006. Chromosome condensation outside of mitosis: mechanisms and new tools. J Cell Physiol. 209(2):297–304. doi:10.1002/jcp.20720
- Harrison JD, Balonov M, Bochud F, Martin C, Menzel H-G, Ortiz-Lopez P, Smith-Bindman R, Simmonds JR, Wakeford R. 2021. ICRP publication 147: use of dose quantities in radiological protection. Ann ICRP. 50(1):9–82. doi:10.1177/0146645320911864
- Hartel C, Nikoghosyan A, Durante M, Sommer S, Nasonova E, Fournier C, Lee R, Debus J, Schulz-Ertner D, Ritter S. 2010. Chromosomal aberrations in peripheral blood lymphocytes of prostate cancer patients treated with IMRT and carbon ions. Radiother Oncol. 95(1):73–78. doi:10.1016/j.radonc.2009.08.031
- Helm A, Fournier C, Durante M. 2022. Particle radiotherapy and molecular therapies: mechanisms and strategies towards clinical applications. Expert Rev Mol Med. 24:e8. doi:10.1017/erm.2022.2
- Helm A, Totis C, Durante M, Fournier C. 2023. Are charged particles a good match for combination with immunotherapy? Current knowledge and perspectives. Int Rev Cell Mol Biol. 376:1–36. doi:10.1016/bs.ircmb.2023.01.001
- Hsieh RC-E, Lee C-H, Huang H-C, Wu S-W, Chou C-Y, Hung S-P, Lee C-W, Krishnan S, Venkatesulu BP, Lee J-C, et al. 2023. Clinical and dosimetric results of proton or photon radiation therapy for large (>5 cm) hepatocellular carcinoma: a retrospective analysis. Int J Radiat Oncol. doi:10.1016/j.ijrobp.2023.09.049
- Iorio GC, Spieler BO, Ricardi U, Dal Pra A. 2021. The impact of pelvic nodal radiotherapy on hematologic toxicity: a systematic review with focus on leukopenia, lymphopenia and future perspectives in prostate cancer treatment. Crit Rev Oncol Hematol. 168:103497. doi:10.1016/j.critrevonc.2021.103497
- Kamada T, Tsujii H, Blakely EA, Debus J, De Neve W, Durante M, Jäkel O, Mayer R, Orecchia R, Pötter R, et al. 2015. Carbon ion radiotherapy in Japan: an assessment of 20 years of clinical experience. Lancet Oncol. 16(2):e93–e100. doi:10.1016/S1470-2045(14)70412-7
- Kawashiro S, Yamada S, Okamoto M, Ohno T, Nakano T, Shinoto M, Shioyama Y, Nemoto K, Isozaki Y, Tsuji H, et al. 2018. Multi-institutional STUDY OF CARBON-ION RADIOTHERAPY FOR LOCALLY ADVANCED PANCREATIC CANCEr: Japan Carbon-ion Radiation Oncology Study Group (J-CROS) Study 1403 pancreas. Int J Radiat Oncol Biol Phys. 101(5):1212–1221. doi:10.1016/j.ijrobp.2018.04.057
- Kelly RJ, Ajani JA, Kuzdzal J, Zander T, Van Cutsem E, Piessen G, Mendez G, Feliciano J, Motoyama S, Lièvre A, et al. 2021. Adjuvant nivolumab in resected esophageal or gastroesophageal junction cancer. N Engl J Med. 384(13):1191–1203. doi:10.1056/NEJMoa2032125
- Kim H, Park S, Jung HA, Lee S-H, Park K, Ahn YC, Oh D, Ahn M-J. 2023. Phase II trial of combined durvalumab plus tremelimumab with proton therapy for recurrent or metastatic head and neck squamous cell carcinoma. Cancer Res Treat. 55(4):1104–1112. doi:10.4143/crt.2023.502
- Kim N, Myoung Noh J, Lee W, Park B, Park H, Young Park J, Pyo H. 2021. Proton beam therapy reduces the risk of severe radiation-induced lymphopenia during chemoradiotherapy for locally advanced non-small cell lung cancer: a comparative analysis of proton versus photon therapy. Radiother Oncol. 156:166–173. doi:10.1016/j.radonc.2020.12.019
- Kim S, Byun HK, Shin J, Lee IJ, Sung W. 2023. Normal tissue complication probability modeling of severe radiation-induced lymphopenia using blood dose for hepatocellular carcinoma patients. Int J Radiat Oncol. 89:100889. doi:10.1016/j.ijrobp.2023.11.060
- Kim Y, Chamseddine I, Cho Y, Kim JS, Mohan R, Shusharina N, Paganetti H, Lin S, Yoon HI, Cho S, et al. 2023. Neural network based ensemble model to predict radiation induced lymphopenia after concurrent chemo-radiotherapy for non-small cell lung cancer from two institutions. Neoplasia. 39:100889. doi:10.1016/j.neo.2023.100889
- Kirkwood JM, Butterfield LH, Tarhini A. a, Zarour H, Kalinski P, Ferrone S. 2012. Immunotherapy of cancer in 2012. CA Cancer J Clin. 62(5):309–335. doi:10.3322/caac.20132
- Lee NY, Ferris RL, Psyrri A, Haddad RI, Tahara M, Bourhis J, Harrington K, Chang PM-H, Lin J-C, Razaq MA, et al. 2021. Avelumab plus standard-of-care chemoradiotherapy versus chemoradiotherapy alone in patients with locally advanced squamous cell carcinoma of the head and neck: a randomised, double-blind, placebo-controlled, multicentre, phase 3 trial. Lancet Oncol. 22(4):450–462. doi:10.1016/S1470-2045(20)30737-3
- Lee R, Yamada S, Yamamoto N, Miyamoto T, Ando K, Durante M, Tsujii H. 2004. Chromosomal aberrations in lymphocytes of lung cancer patients treated with carbon ions. J Radiat Res. 45(2):195–199. doi:10.1269/jrr.45.195
- Li Y, Fan X, Yu Q, Zhai H, Mo M, Sun J, Mi J, Lu R, Mao J, Chen J, et al. 2023. Proton and carbon ion radiation therapy decreased severe lymphopenia by reducing thoracic vertebra and aortic doses in non-small cell lung cancer versus intensity modulated radiation therapy. Int J Radiat Oncol Biol Phys. 116(3):579–589. doi:10.1016/j.ijrobp.2022.12.030
- Lim M, Weller M, Idbaih A, Steinbach J, Finocchiaro G, Raval RR, Ansstas G, Baehring J, Taylor JW, Honnorat J, et al. 2022. Phase III trial of chemoradiotherapy with temozolomide plus nivolumab or placebo for newly diagnosed glioblastoma with methylated MGMT promoter. Neuro Oncol. 24(11):1935–1949. doi:10.1093/neuonc/noac116
- Mohan R, Liu AY, Brown PD, Mahajan A, Dinh J, Chung C, McAvoy S, McAleer MF, Lin SH, Li J, et al. 2020. Proton therapy reduces the likelihood of high-grade radiation–induced lymphopenia in glioblastoma patients: phase II randomized study of protons vs photons. Neuro Oncol. 23(2):284–294. doi:10.1093/neuonc/noaa182
- Nikoghosyan AV, Schulz-Ertner D, Herfarth K, Didinger B, Münter MW, Jensen AD, Jäkel O, Hoess A, Haberer T, Debus J. 2011. Acute toxicity of combined photon IMRT and carbon ion boost for intermediate-risk prostate cancer – acute toxicity of 12C for PC. Acta Oncol. 50(6):784–790. doi:10.3109/0284186X.2011.584558
- Noda K, Furukawa T, Fujimoto T, Hara Y, Inaniwa T, Iwata Y, Katagiri K, Kanematsu N, Mizushima K, Mori S, et al. 2017. Recent progress and future plans of heavy-ion cancer radiotherapy with HIMAC. Nucl Instrum Methods Phys Res Sect B Beam Interact Mater Atoms. 406:374–378. doi:10.1016/j.nimb.2017.04.021
- Pignalosa D, Lee R, Hartel C, Sommer S, Nikoghosyan A, Debus J, Ritter S, Durante M. 2013. Chromosome inversions in lymphocytes of prostate cancer patients treated with X-rays and carbon ions. Radiother Oncol. 109(2):256–261. doi:10.1016/j.radonc.2013.09.021
- Postow MA, Callahan MK, Barker CA, Yamada Y, Yuan J, Kitano S, Mu Z, Rasalan T, Adamow M, Ritter E, et al. 2012. Immunologic correlates of the abscopal effect in a patient with melanoma. N Engl J Med. 366(10):925–931. doi:10.1056/NEJMoa1112824
- Rajeev-Kumar G, Pitroda SP. 2023. Synergizing radiotherapy and immunotherapy: current challenges and strategies for optimization. Neoplasia. 36:100867. doi:10.1016/j.neo.2022.100867
- Ritter S, Nasonova E, Gudowska-Nowak E, Scholz M, Kraft G. 2002. Integrated chromosome aberration yields determined for V79 cells after high LET radiation. Int J Radiat Biol. 78(11):1063–1064. doi:10.1080/0955300021000020292
- Routman DM, Garant A, Lester SC, Day CN, Harmsen WS, Sanheuza CT, Yoon HH, Neben-Wittich MA, Martenson JA, Haddock MG, et al. 2019. A comparison of grade 4 lymphopenia with proton versus photon radiation therapy for esophageal cancer. Adv Radiat Oncol. 4(1):63–69. doi:10.1016/j.adro.2018.09.004
- Schoenfeld JD, Giobbie-Hurder A, Ranasinghe S, Kao KZ, Lako A, Tsuji J, Liu Y, Brennick RC, Gentzler RD, Lee C, et al. 2022. Durvalumab plus tremelimumab alone or in combination with low-dose or hypofractionated radiotherapy in metastatic non-small-cell lung cancer refractory to previous PD(L)-1 therapy: an open-label, multicentre, randomised, phase 2 trial. Lancet Oncol. 23(2):279–291. doi:10.1016/S1470-2045(21)00658-6
- Sender R, Weiss Y, Navon Y, Milo I, Azulay N, Keren L, Fuchs S, Ben-Zvi D, Noor E, Milo R. 2023. The total mass, number, and distribution of immune cells in the human body. Proc Natl Acad Sci USA. 120(44):e2308511120. doi:10.1073/pnas.2308511120
- Sharma P, Allison JP. 2015. The future of immune checkpoint therapy. Science. 348(6230):56–61. doi:10.1126/science.aaa8172
- Sharma P, Goswami S, Raychaudhuri D, Siddiqui BA, Singh P, Nagarajan A, Liu J, Subudhi SK, Poon C, Gant KL, et al. 2023. Immune checkpoint therapy—current perspectives and future directions. Cell. 186(8):1652–1669. doi:10.1016/j.cell.2023.03.006
- Sherry AD, von Eyben R, Newman NB, Gutkin P, Mayer I, Horst K, Chakravarthy AB, Rafat M. 2020. Systemic inflammation after radiation predicts locoregional recurrence, progression, and mortality in stage II–III triple-negative breast cancer. Int J Radiat Oncol Biol Phys. 108(1):268–276. doi:10.1016/j.ijrobp.2019.11.398
- Shiraishi Y, Fang P, Xu C, Song J, Krishnan S, Koay EJ, Mehran RJ, Hofstetter WL, Blum-Murphy M, Ajani JA, et al. 2018. Severe lymphopenia during neoadjuvant chemoradiation for esophageal cancer: a propensity matched analysis of the relative risk of proton versus photon-based radiation therapy. Radiother Oncol. 128(1):154–160. doi:10.1016/j.radonc.2017.11.028
- Spigel DR, Faivre-Finn C, Gray JE, Vicente D, Planchard D, Paz-Ares L, Vansteenkiste JF, Garassino MC, Hui R, Quantin X, et al. 2022. Five-year survival outcomes from the PACIFIC trial: durvalumab after chemoradiotherapy in stage III non–small-cell lung cancer. J Clin Oncol. 40(12):1301–1311. doi:10.1200/JCO.21.01308
- Stjernswärd J, Vánky F, Jondal M, Wigzell H, Sealy R. 1972. Lymphopenia and change in distribution of human B and T lymphocytes in peripheral blood induced by irradiation of mammary carcinoma. Lancet. 1(7765):1352–1356. doi:10.1016/S0140-6736(72)91091-4
- Sumiya T, Ishikawa H, Hiroshima Y, Nakamura M, Murakami M, Mizumoto M, Okumura T, Sakurai H. 2021. The impact of lymphopenia during chemoradiotherapy using photons or protons on the clinical outcomes of esophageal cancer patients. J Radiat Res. 62:1045–1055. doi:10.1093/jrr/rrab094
- Takanen S, Bottero M, Nisticò P, Sanguineti G. 2022. A systematic review on the impact of hypofractionated and stereotactic radiotherapy on immune cell subpopulations in cancer patients. Cancers (Basel). 14(21):5190. doi:10.3390/cancers14215190
- Takeda K, Umezawa R, Yamamoto T, Takahashi N, Suzuki Y, Kishida K, Omata S, Jingu K. 2023. Acute hematologic toxicity of radiation therapy – a comprehensive analysis and predictive nomogram. J Radiat Res. 64(6):954–961. doi:10.1093/jrr/rrad069
- Tao Y, Biau J, Sun XS, Sire C, Martin L, Alfonsi M, Prevost JB, Modesto A, Lafond C, Tourani JM, et al. 2023. Pembrolizumab versus cetuximab concurrent with radiotherapy in patients with locally advanced squamous cell carcinoma of head and neck unfit for cisplatin (GORTEC 2015-01 PembroRad): a multicenter, randomized, phase II trial. Ann Oncol. 34(1):101–110. doi:10.1016/j.annonc.2022.10.006
- Terrones-Campos C, Ledergerber B, Vogelius IR, Helleberg M, Specht L, Lundgren J. 2021. Hematological toxicity in patients with solid malignant tumors treated with radiation – Temporal analysis, dose response and impact on survival. Radiother Oncol. 158:175–183. doi:10.1016/j.radonc.2021.02.029
- Upadhaya S, Neftelinov ST, Hodge J, Campbell J. 2022. Challenges and opportunities in the PD1/PDL1 inhibitor clinical trial landscape. Nat Rev Drug Discov. 21(7):482–483. doi:10.1038/d41573-022-00030-4
- van Rossum PSN, Juan-Cruz C, Stam B, Rossi MMG, Lin SH, Abravan A, Belderbos JSA, Sonke J-J. 2023. Severe radiation-induced lymphopenia during concurrent chemoradiotherapy for stage III non-small cell lung cancer: external validation of two prediction models. Front Oncol. 13:1278723. doi:10.3389/fonc.2023.1278723
- Venkatesulu B, Giridhar P, Pujari L, Chou B, Lee JH, Block AM, Upadhyay R, Welsh JS, Harkenrider MM, Krishnan S, et al. 2022. Lymphocyte sparing normal tissue effects in the clinic (LymphoTEC): a systematic review of dose constraint considerations to mitigate radiation-related lymphopenia in the era of immunotherapy. Radiother Oncol. 177:81–94. doi:10.1016/j.radonc.2022.10.019
- Wang X, van Rossum PSN, Chu Y, Hobbs BP, Grassberger C, Hong TS, Liao Z, Yang J, Zhang X, Netherton T, et al. 2023. Severe lymphopenia during chemoradiation therapy for esophageal cancer: comprehensive analysis of randomized phase 2B trial of proton beam therapy versus intensity modulated radiation therapy. Int J Radiat Oncol Biol Phys. 118(2):368–377. doi:10.1016/j.ijrobp.2023.08.058
- Wu H, Durante M, George K, Yang TC. 1997. Induction of chromosome aberrations in human cells by charged particles. Radiat Res. 148(5 Suppl):S102–S7.
- Yamada S, Durante M, Ando K, Furusawa Y, Kawata T, Majima H, Tsujii H. 2000. Complex-type chromosomal exchanges in blood lymphocytes during radiation therapy correlate with acute toxicity. Cancer Lett. 150(2):215–221. doi:10.1016/S0304-3835(99)00405-X
- Yang G, Yoon HI, Lee J, Kim J, Kim H, Cho J, Lee CG, Chang JS, Cho Y, Kim JS, et al. 2023. Risk of on-treatment lymphopenia is associated with treatment outcome and efficacy of consolidation immunotherapy in patients with non-small cell lung cancer treated with concurrent chemoradiotherapy. Radiother Oncol. 189:109934. doi:10.1016/j.radonc.2023.109934
- Yang TC, George K, Johnson AS, Durante M, Fedorenko BS. 1997. Biodosimetry results from space flight Mir-18. Radiat Res. 148(5 Suppl):S17–S23.
- Yovino S, Kleinberg L, Grossman SA, Narayanan M, Ford E. 2013. The etiology of treatment-related lymphopenia in patients with malignant gliomas: modeling radiation dose to circulating lymphocytes explains clinical observations and suggests methods of modifying the impact of radiation on immune cells. Cancer Invest. 31(2):140–144. doi:10.3109/07357907.2012.762780
- Zhang Y, Huang C, Li S. 2023. Influence of treatment-related lymphopenia on the efficacy of immune checkpoint inhibitors in lung cancer: a meta-analysis. Front Oncol. 13:1287555. doi:10.3389/fonc.2023.1287555