Abstract
Purpose
Toxicities from head and neck (H&N) radiotherapy (RT) may affect patient quality of life and can be dose-limiting. Proteins from the transforming growth factor beta (TGF-β) family are key players in the fibrotic response. While TGF-β1 is known to be pro-fibrotic, TGF-β3 has mainly been considered anti-fibrotic. Moreover, TGF-β3 has been shown to act protective against acute toxicities after radio- and chemotherapy. In the present study, we investigated the effect of TGF-β3 treatment during fractionated H&N RT in a mouse model.
Materials and methods
30 C57BL/6J mice were assigned to three treatment groups. The RT + TGF-β3 group received local fractionated H&N RT with 66 Gy over five days, combined with TGF-β3-injections at 24-hour intervals. Animals in the RT reference group received identical RT without TGF-β3 treatment. The non-irradiated control group was sham-irradiated according to the same RT schedule. In the follow-up period, body weight and symptoms of oral mucositis and lip dermatitis were monitored. Saliva was sampled at five time points. The experiment was terminated 105 d after the first RT fraction. Submandibular and sublingual glands were preserved, sectioned, and stained with Masson’s trichrome to visualize collagen.
Results
A subset of mice in the RT + TGF-β3 group displayed increased severity of oral mucositis and increased weight loss, resulting in a significant increase in mortality. Collagen content was significantly increased in the submandibular and sublingual glands for the surviving RT + TGF-β3 mice, compared with non-irradiated controls. In the RT reference group, collagen content was significantly increased in the submandibular gland only. Both RT groups displayed lower saliva production after treatment compared to controls. TGF-β3 treatment did not impact saliva production.
Conclusions
When repeatedly administered during fractionated RT at the current dose, TGF-β3 treatment increased acute H&N radiation toxicities and increased mortality. Furthermore, TGF-β3 treatment may increase the severity of radiation-induced salivary gland fibrosis.
Introduction
Radiotherapy (RT) is an important modality for the treatment of head and neck (H&N) cancer, but radiation-induced damage to normal tissue may affect the quality of life of patients and can be dose-limiting. Toxicities from H&N RT may be acute or chronic, and common side effects include dermatitis, oral mucositis, and replacement of salivary gland tissue by connective tissue followed by salivary gland dysfunction and hyposalivation (Sroussi et al. Citation2017; Siddiqui and Movsas Citation2017; Wang and Tepper Citation2021).
The transforming growth factor beta (TGF-β) family of proteins is a group of ubiquitously expressed, pleiotropic proteins with functions within diverse areas including cell cycle regulation, tissue homeostasis, and immunity (Laverty et al. Citation2009; Fujio et al. Citation2016; Du et al. Citation2023). The three isoforms found in humans, TGF-β1-3, possess high structural conformity but exert different functions in several biological processes, including radiation response (Hanson et al. Citation2023).
TGF-β1 is activated by ionizing radiation and indirectly opposes the effect of cancer radiotherapy through improved DNA repair, suppression of anti-tumor immunity, and remodeling of the tumor microenvironment which can support tumor progression (Barcellos-Hoff Citation2022). Treatment with recombinant TGF-β3 has been linked to reduced severity of acute radiation damage in vitro (Robson et al. Citation1997) and in vivo (Booth et al. Citation2000; Potten et al. Citation1997). Furthermore, topical TGF-β3 treatment was developed as a mitigator of acute chemotherapy-induced oral mucositis and showed promise in preclinical trials, but failed phase II clinical trials due to a lack of effectiveness compared to placebo (Foncuberta et al. Citation2001; Sonis et al. Citation1994, Citation1997; Wymenga et al. Citation1999).
Radiation-induced fibrosis develops when reactive oxygen species produced by ionizing radiation causes prolonged inflammation, triggering the transformation of local fibroblasts into myofibroblasts and the subsequent excessive deposition of extracellular matrix components (Straub et al. Citation2015). TGF-β1 is considered a key molecular player in fibrogenesis through fibroblast activation and TGF-β2 is believed to have a similar function (Frangogiannis Citation2020). Although the role of TGF-β3 in the development of fibrosis is more debated (Wilson Citation2021), several studies report an anti-fibrotic function (Ask et al. Citation2008; Bush et al. Citation2010; Karamichos et al. Citation2014; Wu et al. Citation2015). Additionally, treatment with TGF-β3 has been observed to delay the onset and decrease the severity of radiation-induced pulmonary fibrosis in mice (Xu et al. Citation2014).
Here, we examined if daily TGF-β3 injections could alleviate radiation-induced healthy tissue toxicity during a fractionated RT regime in a mouse model. Surprisingly, we observed increased acute oral toxicity, increased weight loss, and decreased survival in the TGF-β3 treated group. In addition, we found indications of fibrogenesis through an increased amount of collagen in the submandibular and sublingual salivary glands in the surviving RT + TGF-β3 treated animals. In the RT reference group, the increase in collagen was significant in the submandibular gland only.
Materials and methods
Animals and treatment
All experiments involving animals were approved by the Norwegian Food Safety Authority according to Directive 2010/63/EU on the protection of animals used for scientific purposes (FOTS ID 27931). C57BL/6J mice were purchased from Janvier (France) at nine weeks of age. The aim of the current study did not include an investigation of sex-based differences in radiation response, thus only female mice were included. The animals were randomly assigned into three treatment groups: Treatment with TGF-β3 and fractionated RT (RT + TGF-β3) (n = 10), fractionated RT only (RT reference) (n = 10), and non-irradiated controls (n = 10). Data for the non-irradiated controls and the RT reference group have previously been published in Juvkam et al. (Citation2022) and (Juvkam et al. Citation2023). A detailed description of the treatment setup is available in Juvkam et al. (Citation2022). Briefly, 12-week-old animals were anesthetized and placed in a holder with a custom-built lead collimator defining a radiation field covering the oral cavity, pharynx, and major salivary glands. They were then irradiated with 100 kV x-rays at a dose rate of 0.66 Gy/min in 10 fractions of 6.6 Gy over 5 d (total dose 66 Gy). The fractionation regime and radiation dose were determined based on a previously established mouse model of H&N normal tissue toxicity from our laboratory, which was designed to imitate the acute and late effects observed in human cancer patients (Juvkam et al. Citation2022). Non-irradiated controls were anesthetized and sham irradiated according to the same schedule. In the follow-up period of 105 d, the body weight and general welfare of the animals were monitored frequently. Every third day during the first 35 d, blinded oral examinations were executed under anesthesia to assess symptoms of acute skin toxicity and oral mucositis, according to criteria developed by the Radiation Therapy Oncology Group (Trotti et al. Citation2000; Sonis et al. Citation2004; Mallick et al. Citation2016). Saliva was sampled on day −8 (baseline), 35, 80, and 105 after the first fraction. Upon indication of moderate to severe oral pain for a subset of animals, all animals were fed DietGel® (ClearH2O, Westbrook, ME, USA), and received analgesic treatment in the form of subcutaneous buprenorphine injections (Temgesic, Indivior, Richmond, VA, USA) three times per day for four consecutive days (day 12 to day 15). Weight loss of more than 20% of baseline weight was defined as a humane endpoint and animals with such weight loss were euthanized by cervical dislocation. On day 105, the remaining animals were euthanized by intraperitoneal (i.p.) injection of anesthetic (Pentobarbital, Exagon® Vet, Richter Pharma AG, Austria) under terminal anesthesia to avoid H&N tissue damage.
TGF-β3 treatment
Animals in the RT + TGF-β3 group received five i.p. injections of 0.1 ml recombinant TGF-β3 (R&D Systems, MN, USA) at a concentration of 2.5 µg/mL, resulting in a dose of approximately 12.5 µg/kg body weight. Injections were given at 24-hour intervals, with the first administration 24 h before the first radiation fraction. The TGF-β3 dose and the timing of injections were determined based on an unpublished pilot study investigating the mitigation of acute radiotoxicity after 10.5 Gy total body irradiation of DBA/2 mice.
Histological evaluations
Submandibular and sublingual salivary glands were collected immediately after euthanasia. The tissues were then formalin fixed, dehydrated, paraffin-embedded, and sectioned as described in Juvkam et al. (Citation2022), before staining with Masson’s Trichrome Stain Kit (Abcam, UK) according to the manufacturer’s instructions to identify collagen. Sections were examined using a Nikon E90i microscope and images were acquired with a Nikon DS-Ri1 camera and a CFI Plan Fluor ×10 (NA 0.30) objective (Nikon, Japan). Images were analyzed using the Color Threshold function in ImageJ (Schneider et al. Citation2012) to calculate the ratio of collagen to total tissue area. A detailed image analysis protocol can be found in Supplementary File 1.
Statistical analyses
The survival data in were analyzed using the log-rank test. The correlation in was analyzed using Kendall’s τ test. The collagen content data in were analyzed using one-way ANOVA followed by Tukey’s HSD test. The saliva volume data in was analyzed using repeated measures ANOVA and the correlations in Figure 4(B,C) were analyzed using Kendall’s τ test. All comparisons used a significance level of 0.05.
Figure 1. (A) Change in body weight relative to baseline for mice in the non-irradiated control, radiotherapy (RT) reference, and RT + transforming growth factor beta 3 (RT + TGF-β3) treated groups. A subset of mice in the RT + TGF-β3 group experienced severe weight loss and was euthanized, while the others recovered. (B) Kaplan Meyer survival plot of the non-irradiated control, RT reference, and RT + TGF-β3 groups. Survival in the RT + TGF-β3 group was significantly lower than the others (p = .0044, log-rank test).
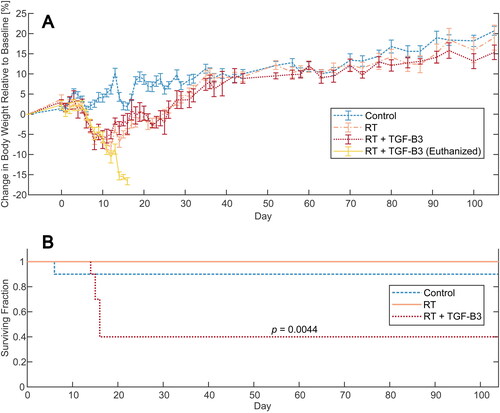
Figure 2. (A) Oral mucositis score for mice in the non-irradiated control, RT reference, and RT + TGF-β3 groups. (B) Skin toxicity scores for the same groups. (C–E) Representative images of oral mucositis and skin toxicity in non-irradiated control, RT reference, and RT + TGF-β3 groups, respectively. Outlined area marks oral mucositis. (C) Oral mucositis score 0, skin toxicity score 0. (D) oral mucositis score 2, skin toxicity score 2. (E) Oral mucositis score 3, skin toxicity score 3. (F) Correlation change in body weight relative to baseline and oral mucositis score on day 12 (p = 8.6e-06, Kendall’s τ), and relative change in body weight and skin toxicity score on day 15 (p = 2.8e-05, Kendall’s τ) in non-irradiated controls, RT reference and RT + TGF-β3 treated mice.
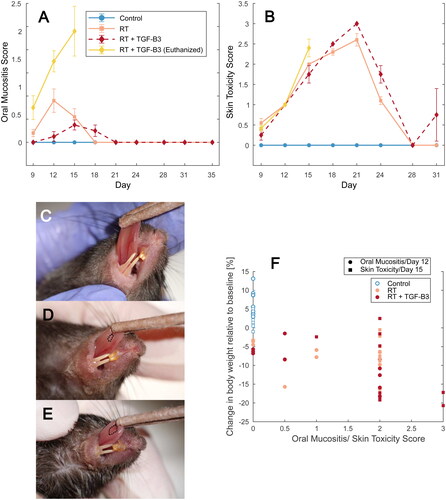
Figure 4. (A) Saliva volume sampled from mice in the non-irradiated control, RT reference, and RT + TGF-β3 groups. Time-dependent increase in non-irradiated controls was significant (p = .00015, repeated measures ANOVA). (B) Saliva volume measured on day 35 correlated with cumulative oral mucositis score up to day 35 (p = .0092, Kendall’s τ). Non-irradiated controls, RT reference group and RT + TGF-β3 treated mice. (C) Cumulative saliva volume up until day 105 correlated with collagen content in the submandibular salivary gland (p = .00058, Kendall’s τ) for the same groups as B.
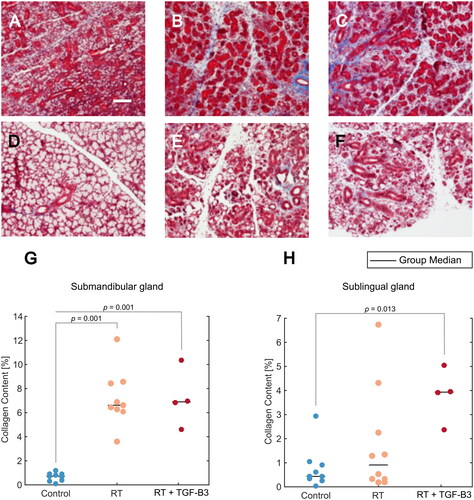
Figure 3. (A–F) Representative images of tissue sections stained with Masson’s trichrome. The blue stain indicates collagen. Scale bar = 100 µm. (A) Submandibular salivary gland from non-irradiated control. (B) Submandibular gland from RT reference group. (C) Submandibular gland from RT + TGF-β3 treated group. (D) Sublingual salivary gland from non-irradiated control. (E) Sublingual gland from RT reference group. (F) Sublingual gland from RT + TGF-β3 group. (G) Collagen content in submandibular gland for non-irradiated control, RT reference, and RT + TGF-β3 groups (p = .001, ANOVA w/Tukey’s HSD). (H) Collagen content in sublingual gland for non-irradiated control, RT reference, and RT + TGF-β3 groups (p = .013, ANOVA w/Tukey’s HSD). (G–H) black line = group median.
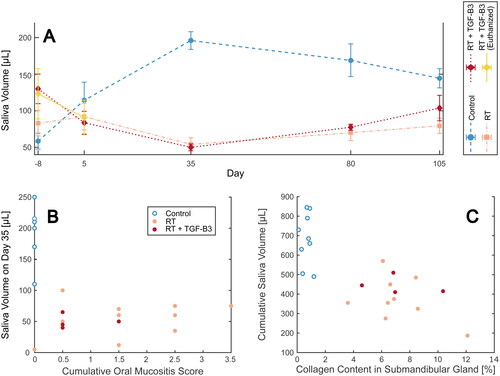
Results
TGF-β3 treatment increased the mortality after 66 Gy fractionated H&N RT
Body weight was monitored frequently throughout the experiment. Both groups of irradiated mice rapidly lost weight from around day 6, while non-irradiated controls were stable or gaining weight (). Animals in the RT reference group reached a body weight minimum around day 12 before they started gaining weight. A subset of six mice in the RT + TGF-β3 group lost more than 20% body weight compared to baseline and were euthanized on day 14–16, resulting in a significantly lower survival in this group compared to non-irradiated and RT reference groups () (p = .0044, log-rank test). The remaining mice in the RT + TGF-β3 group followed a similar pattern of weight loss as the RT reference group and started gaining weight around day 11. After the acute period, all irradiated animals including the surviving TGF-β3 treated mice followed the same pattern of stable weight gain as the non-irradiated controls.
TGF-β3 treatment increased the severity of acute oral mucositis
Blinded oral examinations were conducted every three days in the acute phase to assess the severity of radiation-induced oral mucositis and skin toxicity. Animals in the RT reference group developed mild to moderate oral mucositis from day 12 (). The RT + TGF-β3 grouped into two subsets: (1) mice with severe oral mucositis and weight loss prior to euthanasia and (2) mild to moderate oral mucositis in the survivors, although their symptoms persevered slightly longer than in the RT reference group (day 21 versus 18). For skin toxicity, animals in the RT reference group and all mice in the RT + TGF-β3 group experienced similar progression (). Skin toxicity was present on day 9 and progressed until day 21 before the symptoms declined and were resolved by day 28 for most individuals. One surviving mouse in the RT + TGF-β3 group experienced severe skin toxicity on the last day of grading (day 31), after exhibiting no signs of skin toxicity on day 28. All non-irradiated controls showed no oral mucositis or skin toxicity. Representative images of mice in the non-irradiated, RT reference, and RT + TGF-β3 groups are displayed in .
Relative change in body weight was confirmed to correlate with oral mucositis score on day 12 (p < .001, Kendall’s τ) and skin toxicity score on day 15(p < .001, Kendall’s τ) ().
TGF-β3 treatment impacted salivary gland collagen content
Submandibular and sublingual salivary glands were harvested, preserved, and stained with Masson’s trichrome staining after the termination of the experiment on day 105 (). Quantitative analysis of submandibular glands revealed increased collagen content in the RT reference and RT + TGF-β3 treated groups, compared to non-irradiated controls (p = .001, ANOVA w/Tukey’s HSD) (). In the sublingual glands, one non-irradiated control mouse was found to have a higher collagen content than the others in the group (). In the RT reference group, three mice displayed increased collagen content, but the increase relative to controls was not significant for the group as a whole. All four RT + TGF-β3 treated survivors displayed increased collagen content compared to non-irradiated controls, and the change relative to controls was significant (p = .013, ANOVA w/Tukey’s HSD). The difference between animals in the RT reference group and the RT + TGF-β3 treated group was not significant.
TGF-β3 treatment did not impact saliva production
Saliva was collected from anesthetized mice and measured at five time points during the experimental period (). In non-irradiated control mice, saliva production significantly increased during the experiment period (p < .001, repeated measures ANOVA). In the RT reference group and surviving RT + TGF-β3 treated mice, there was no increase over time, and saliva volume remained lower than that of control mice until termination. No difference was observed by treatment with TGF-β3 compared to animals in the RT reference group.
Saliva volume measured at the end of the acute period after irradiation (day 35) was significantly negatively correlated to the cumulative oral mucositis score each mouse obtained during the same period (p < .001, Kendall’s τ) ().
When the saliva volume measured throughout the experiment was pooled for each animal, this cumulative volume was significantly negatively correlated to the collagen content in the submandibular gland (p < .001, Kendall’s τ) (). A similar trend was observed for cumulative saliva volume and collagen content in the sublingual gland, but the correlation was not significant (p = .48, Kendall’s τ) (Supplementary File 2).
Discussion
The purpose of the current study was to evaluate the protective effects of TGF-β3 against acute and late radiation toxicities following fractionated radiotherapy in a mouse model. Surprisingly, TGF-β3 treated animals displayed increased oral toxicity, increased weight loss, and decreased survival. Furthermore, TGF-β3 treated animals showed indications of increased fibrogenesis in the sublingual salivary gland.
Repeated injections of TGF-β3 concurrently with fractionated RT increased oral mucositis scores and increased weight loss in a subset of animals, resulting in increased mortality compared to RT alone. Weight loss above 20% of baseline was used as a humane endpoint, thereby influencing the mortality measured in each group. Due to the relatively large total radiation dose to the H&N area in the current experiment, the food intake of the animals may have been affected by pain from the development of oral mucositis and xerostomia resulting from salivary gland damage. This dysphagia may contribute to the reduction in saliva secretion independent of the effects of irradiation on the salivary glands. To minimize animal suffering and avoid unnecessary weight loss and mortality, the administration of analgesic injections and gel fodder were attempted as alleviating measures. When data from all animals were compared, the relative change in body weight was significantly correlated with oral mucositis score on day 12 and skin toxicity score on day 15. From this, we conclude that the severe weight loss observed was a result of pain experienced due to acute radiation toxicity leading to a reduction in food consumption and that severe weight loss was a reasonable humane endpoint for the current study.
In contrast to the results presented here, i.p. injections with TGF-β3 have previously been found to decrease acute radiation toxicity in the intestines of male BDF1 mice (Potten et al. Citation1997; Booth et al. Citation2000). Furthermore, topical application of TGF-β3 in a Syrian golden hamster model (sex undisclosed) decreased the severity of chemotherapy-induced oral mucositis through inhibition of epithelial cell proliferation, thereby increasing survival (Sonis et al. Citation1994; Sonis et al. Citation1997). Mouthwash with TGF-β3 as the active ingredient was tested in cancer patients receiving chemotherapy, but failed to document an effect compared to placebo and did not progress beyond phase II clinical trials (Wymenga et al. Citation1999; Foncuberta et al. Citation2001). However, no clinically relevant adverse effects of TGF-β3 treatments were observed in any of these studies.
Alleviation of acute side effects by TGF-β3 in previous studies was attributed to the induction of a transient cell cycle arrest by inhibition of G1/S progression, allowing stem cells in regenerating tissues time for DNA damage repair and thereby reduce radiation damage (Khalil et al. Citation1994; McCormack et al. Citation1997; Robson et al. Citation1997; Nakamura et al. Citation2010). In the studies referred to above, however, a protective effect was seen when TGF-β3 treatment was administered before challenge with ionizing radiation or chemotherapy. Potten et al. observed that compared to a treatment regime with five i.p. TGF-β3 injections before irradiation, the addition of one post-irradiation injection decreased the radio-protective effect on the intestinal crypts from a factor of 4.9 to 1.6 (Potten et al. Citation1997). Furthermore, when TGF-β3 injections were administered solely post-irradiation, this induced a radio-sensitization and surviving crypts decreased to 1/3 of control. From this, the authors concluded that when the TGF-β3-induced cell cycle arrest was prolonged after irradiation, tissue regeneration was impeded. The increase in acute radiotoxicity from TGF-β3 treatment observed in the current study may result from the administration of TGF-β3 between RT fractions, which may have inhibited the healing process of radiation-damaged oral mucosa through a sustained G1/S cell cycle arrest of stem cells or transit amplifying cells (Cancedda and Mastrogiacomo Citation2023).
In addition to the timing of TGF-β3 injections, several other parameters may have influenced its impact on acute radiotoxicity. Potten et al. discovered an increasing degree of protection of intestinal mucosa with increasing TGF-β3 dose (Potten et al. Citation1997). Nevertheless, a protection factor of 3.32 was observed at the lowest dose tested at ∼ 20 µg/kg per injection, and it appears unlikely that the adverse effects of the TGF-β3 treatment in the current experiment were the result of a slightly lower dose of ∼ 12.5 µg/kg per injection. In the studies by Sonis et al. regarding the protection of oral mucosa after chemotherapy, the TGF-β3 treatment was applied topically, and therefore the dose is not directly comparable to the i.p. injections in the current study.
Besides differences in TGF-β3 dose, previous studies utilized shorter intervals between TGF-β3 injections, which may have influenced the radioprotective ability of the cytokine. Finally, the choice of animal model, age, and sex differed between the current and previous studies and may have influenced the outcomes (Boria et al. Citation2020; Li et al. Citation2023; Taliaferro et al. Citation2023).
Treatment with RT and TGF-β3 significantly increased collagen content in the submandibular and sublingual salivary glands, whereas RT alone significantly increased collagen content in the submandibular gland only. In the RT group, collagen content in the sublingual gland varied markedly between animals, and the difference between the RT + TGF-β3 group and the RT reference group was not significant for either gland. This suggests that treatment with TGF-β3 may have enhanced a trend that appeared after RT. Although TGF-β3 has been observed to exert antifibrotic functions in vitro and in vivo (Shah et al. Citation1995; Chang et al. Citation2014; Karamichos et al. Citation2014; Wu et al. Citation2015; Xue et al. Citation2019; Escasany et al. Citation2021), our results agree with evidence suggesting the role of TGF-β3 as a purely anti-fibrotic cytokine is too simplistic (Wilson Citation2021; Hanson et al. Citation2023).
Xu et al. successfully reduced and delayed the development of radiation-induced pulmonary fibrosis in 6-8-week-old female C57BL/6 mice through i.p. TGF-β3 injections (Xu et al. Citation2014). However, the RT and TGF-β3 treatment regimens employed differed from the ones in the present study. Notably, TGF-β3 injections were administered weekly after irradiation until study termination, whereas TGF-β3 treatment in the current study was terminated after the conclusion of the RT. Moreover, the dose of TGF-β3 was lower at 1 µg/kg, compared to ∼12.5 µg/kg in the current study. In previous preclinical and clinical studies where TGF-β3 has been demonstrated to reduce scar tissue formation, the treatment has typically been injected locally, and the dose is not directly comparable to the current study (Shah et al. Citation1995; Lanning et al. Citation2000; Loewen et al. Citation2001; Hosokawa et al. Citation2003; Ferguson et al. Citation2009; Bush et al. Citation2010; So et al. Citation2011; Chang et al. Citation2014).
Several studies have speculated that an observed shift in the balance between endogenously expressed TGF-β1 and TGF-β3 after irradiation may influence the development of radiation fibrosis (Wang and Robbins Citation1996; Seong et al. Citation2000; Zhao et al. Citation2000). Furthermore, the difference in the wound healing abilities of oral mucosa and cutaneous tissue may be influenced by their TGF-β1/TGF-β3 ratio (Schrementi et al. Citation2008; Jiajia Zhao Citation2015). Considering this, future investigations of TGF-β1/TGF-β3 balance may illuminate the mechanisms behind radiation-induced salivary gland fibrosis. Moreover, the functional effects of both TGF-β1 and TGF-β3 may be influenced by activation status and availability of different TGF-β signaling pathways, and these parameters may conceivably influence the development of radiation-induced fibrosis (Lux et al. Citation1999; Annes et al. Citation2003; Shi and Massagué Citation2003; De Kroon et al. Citation2015; Hanson et al. Citation2023).
In non-irradiated control animals, saliva production increased significantly throughout the experiment period. This is in agreement with previous reports in healthy C57BL/6 mice, where salivary gland weight and salivary flow rate significantly increased from 10 to 30 weeks of age (Choi et al. Citation2013). Animals in the RT reference and RT + TGF-β3 groups did not display this increase. Furthermore, saliva volume sampled at the end of the acute period was significantly negatively correlated to acute radiation damage through oral mucositis score, and the total saliva volume measured throughout the experiment for each animal was significantly negatively correlated to collagen content in the submandibular gland. Although these two parameters were not significantly correlated for the sublingual gland, a similar trend was noted here. Thus, the relative decrease in saliva production in irradiated compared to non-irradiated animals was probably a symptom of salivary gland radiation damage and was not affected by treatment with TGF-β3.
To summarize, we hypothesize that the increase in acute toxicity in the form of increased severity of oral mucositis was due to a TGF-β3-induced G1/S cell cycle arrest, which was sustained when TGF-β3 injections were repeated between irradiation fractions, disallowing stem cells or transit amplifying cells to repopulate damaged oral mucosa. The increased sublingual gland collagen content in the TGF-β3 treated animals was possibly influenced by TGF-β3 dose, the ratio of TGF-β3 to endogenous TGF-β1 concentration, or endogenous availability of TGF-β receptors. At the current dose, and with the current treatment regimen, injections with recombinant TGF-β3 did not mitigate fibrosis as expected but increased both acute and late toxicities of H&N RT in C57BL/6J mice.
Supplemental Material
Download Zip (19.3 KB)Acknowledgements
We thank Delmon Arous at the Department of Physics, University of Oslo for help with the dosimetry measurements.
Disclosure statement
No potential conflict of interest was reported by the author(s).
Data availability statement
The data that support the findings of this study are openly available in Zenodo at http://doi.org/10.5281/zenodo.8337804
Additional information
Funding
Notes on contributors
Ingunn Hanson
Ingunn Hanson is a PhD Research Fellow at the Biophysics and Medical Physics group at the University of Oslo, Norway. Her work is focused on low-dose radiation and the effects of TGF-β3 in radiation response.
Inga Solgård Juvkam
Inga Solgård Juvkam received a Master’s degree in Molecular Biosciences and a Bachelor’s degree in Biochemistry at the Institute of Biosciences at the University of Oslo. She is a PhD Research Fellow at the Institute of Oral Biology at the University of Oslo. She has experience in histology, cell biology, and preclinical experiments.
Olga Zlygosteva
Olga Zlygosteva is a PhD Research Fellow at the Biophysics and Medical Physics group at the University of Oslo, Norway. Her research interests include normal tissue effects and cytokine response after proton versus conventional radiotherapy of head and neck cancers.
Tine Merete Søland
Tine Merete Søland, PhD, is an associate professor in oral pathology at the Institute of Oral Biology, University of Oslo, Norway. In addition, she works as an oral pathologist in the diagnostic service at the Department of Pathology at Oslo University Hospital.
Hilde Kanli Galtung
Hilde Kanli Galtung, PhD, is a professor in physiology at the Institute of Oral Biology, Faculty of Dentistry, University of Oslo, Norway. Her scientific interest is salivary gland biology with special focus on cellular changes induced by conditions such as autoimmune disease and radiation damage.
Eirik Malinen
Eirik Malinen holds a PhD in physics (biophysics) and is Head of Department of Radiation Biology, Oslo University Hospital, and Professor at the Department of Physics, University of Oslo, Norway. He is engaged in radiation physics research as well as preclinical and clinical investigations utilizing ionizing radiation.
Nina Frederike Jeppesen Edin
Nina Jeppesen Edin, PhD, is an associate professor and head of Section for Biophysics and Medical Physics at the University of Oslo. Edin’s main interest is preclinical studies of biological effects and mechanisms induced by radiation of different qualities and deliveries.
References
- Annes JP, Munger JS, Rifkin DB. 2003. Making sense of latent TGFbeta activation. J Cell Sci. 116(Pt 2):217–224. doi:10.1242/JCS.00229
- Ask K, Bonniaud P, Maass K, Eickelberg O, Margetts PJ, Warburton D, Groffen J, Gauldie J, Kolb M. 2008. Progressive pulmonary fibrosis is mediated by TGF-β isoform 1 but not TGF-β3. Int J Biochem Cell Biol. 40(3):484–495. doi:10.1016/j.biocel.2007.08.016
- Barcellos-Hoff MH. 2022. The radiobiology of TGFβ. Semin Cancer Biol. 86(Pt 3):857–867. doi:10.1016/j.semcancer.2022.02.001
- Booth D, Haley JD, Bruskin AM, Potten CS. 2000. Transforming growth factor-B3 protects murine small intestinal stem cells and animal survival after irradiation, possibly by reducing stem-cell cycling. Int J Cancer. 86(1):53–59. doi:10.1002/(sici)1097-0215(20000401)86:1<53::aid-ijc8>3.0.co;2-z
- Boria AJ, Perez-Torres CJ, Perez-Torres CJ. 2020. Impact of mouse strain and sex when modeling radiation necrosis. Radiat Oncol. 15(1):141. doi:10.1186/s13014-020-01585-5
- Bush J, Duncan JAL, Bond JS, Durani P, So K, Mason T, O'Kane S, Ferguson MWJ. 2010. Scar-improving efficacy of avotermin administered into the wound margins of skin incisions as evaluated by a randomized, double-blind, placebo-controlled, phase II clinical trial. Plast Reconstr Surg. 126(5):1604–1615. doi:10.1097/PRS.0b013e3181ef8e66
- Cancedda R, Mastrogiacomo M. 2023. Transit amplifying cells (TACs): a still not fully understood cell population. Front Bioeng Biotechnol. 11:1189225. doi:10.3389/fbioe.2023.1189225
- Chang Z, Kishimoto Y, Hasan A, Welham NV. 2014. TGF-β3 modulates the inflammatory environment and reduces scar formation following vocal fold mucosal injury in rats. Dis Model Mech. 7(1):83–91. doi:10.1242/dmm.013326
- Choi JS, Park IS, Kim SK, Lim JY, Kim YM. 2013. Analysis of age-related changes in the functional morphologies of salivary glands in mice. Arch Oral Biol. 58(11):1635–1642. doi:10.1016/J.ARCHORALBIO.2013.07.008
- Du X, Cai L, Xie J, Zhou X. 2023. The role of TGF-beta3 in cartilage development and osteoarthritis. Bone Res. 11(1):2. doi:10.1038/s41413-022-00239-4
- Escasany E, Lanzón B, García-Carrasco A, Izquierdo-Lahuerta A, Torres L, Corrales P, Rodríguez Rodríguez AE, Luis-Lima S, Martínez Álvarez C, Javier Ruperez F, et al. 2021. Transforming growth factor β 3 deficiency promotes defective lipid metabolism and fibrosis in murine kidney. Dis Model Mech. 14(9):dmm048249. doi:10.1242/dmm.048249
- Ferguson MW, Duncan J, Bond J, Bush J, Durani P, So K, Taylor L, Chantrey J, Mason T, James G, et al. 2009. Prophylactic administration of avotermin for improvement of skin scarring: three double-blind, placebo-controlled, phase I/II studies. Lancet. 373(9671):1264–1274. doi:10.1016/S0140-6736(09)60322-6
- Foncuberta MC, Cagnoni PJ, Brandts CH, Mandanas R, Fields K, Derigs HG, Reed E, Sonis ST, Fay J, LeVeque F, et al. 2001. Topical transforming growth factor-β3 in the prevention or alleviation of chemotherapy-induced oral mucositis in patients with lymphomas or solid tumors. J Immunother. 24(4):384–388. doi:10.1097/00002371-200107000-00014
- Frangogiannis NG. 2020. Transforming growth factor–ß in tissue fibrosis. J Exp Med. 217(3):e20190103. doi:10.1084/jem.20190103
- Fujio K, Komai T, Inoue M, Morita K, Okamura T, Yamamoto K. 2016. Revisiting the regulatory roles of the TGF-β family of cytokines. Autoimmun Rev. 15(9):917–922. doi:10.1016/J.AUTREV.2016.07.007
- Hanson I, Pitman KE, Edin NFJ. 2023. The role of TGF-β3 in radiation response. Int J Mol Sci. 24(8):7614. doi:10.3390/ijms24087614
- Hosokawa R, Nonaka K, Morifuji M, Shum L, Ohishi M. 2003. TGF-β3 decreases type I collagen and scarring after labioplasty. J Dent Res. 82(7):558–564. doi:10.1177/154405910308200714
- Jiajia Zhao JL. 2015. The expression level of TGF-β1, TGF-β3 and VEGF in transplanted oral mucosal and cutaneous wounds. Clin Microbiol. 04(02):198. doi:10.4172/2327-5073.1000198
- Juvkam IS, Zlygosteva O, Arous D, Galtung HK, Malinen E, Søland TM, Jeppesen Edin N. 2022. A preclinical model to investigate normal tissue damage following fractionated radiotherapy to the head and neck. J Radiat Res. 64(1):44–52. doi:10.1093/jrr/rrac066
- Juvkam IS, Zlygosteva O, Malinen E, Edin NJ, Galtung HK, Søland TM. 2023. Fractionated irradiation of murine salivary glands resulted in focal acinar cell atrophy, immune cell infiltration, fibrosis, and hyposalivation. BioRxiv 20230905556313 [Preprint] [Internet doi:10.1101/2023.09.05.556313
- Karamichos D, Hutcheon AEK, Zieske JD, Dimitrios K. 2014. Reversal of fibrosis by TGF-β3 in a 3D in vitro model. Exp Eye Res. 124:31–36. doi:10.1016/j.exer.2014.04.020
- Khalil N, O'Connor RN, Flanders KC, Shing W, Whitman CI. 1994. Regulation of type II alveolar epithelial cell proliferation by TGF-β during bleomycin-induced lung injury in rats. Am J Physiol. 267(5 Pt 1):L498–507. doi:10.1152/ajplung.1994.267.5.l498
- De Kroon LMG, Narcisi R, Davidson ENB, Cleary MA, Van Beuningen HM, Koevoet WJLM, Van Osch GJVM, Van Der Kraan PM. 2015. Activin receptor-like kinase receptors ALK5 and ALK1 are both required for TGFβ-induced chondrogenic differentiation of human bone marrow-derived mesenchymal stem cells. PLOS One. 10(12):e0146124. doi:10.1371/JOURNAL.PONE.0146124
- Lanning DA, Diegelmann RF, Yager DR, Wallace ML, Bagwell CE, Haynes JH. 2000. Myofibroblast induction with transforming growth factor-β1 and -β3 in cutaneous fetal excisional wounds. J Pediatr Surg. 35(2):183–188. doi:10.1016/S0022-3468(00)90007-1
- Laverty HG, Wakefield LM, Occleston NL, O'Kane S, Ferguson MWJ. 2009. TGF-β3 and cancer: A review. Cytokine Growth Factor Rev. 20(4):305–317. doi:10.1016/j.cytogfr.2009.07.002
- Li H, Kucharavy HC, Hajj C, Zhao L, Hua G, Glass R, Paty PB, Fuks Z, Kolesnick R, Hubbard K, et al. 2023. Radiation-induced gastrointestinal (GI) syndrome as a function of age. Cell Death Discov. 9(1):31. doi:10.1038/s41420-023-01298-0
- Loewen MS, Walner DL, Caldarelli DD. 2001. Improved airway healing using transforming growth factor beta-3 in a rabbit model. Wound Repair Regen. 9(1):44–49. doi:10.1046/j.1524-475x.2001.00044.x
- Lux A, Attisano L, Marchuk DA. 1999. Assignment of transforming growth factor 1 and 3 and a third new ligand to the type I receptor ALK-1*. J Biol Chem. 274(15):9984–9992. doi:10.1074/jbc.274.15.9984
- Mallick S, Benson R, Rath GK. 2016. Radiation induced oral mucositis: a review of current literature on prevention and management. Eur Arch Otorhinolaryngol. 273(9):2285–2293. doi:10.1007/s00405-015-3694-6
- McCormack ES, Borzillo GV, Ambrosino C, Mak G, Hamablet L, Qu GY, Haley JD. 1997. Transforming growth factor-β3 protection of epithelial cells from cycle-selective chemotherapy in vitro. Biochem Pharmacol. 53(8):1149–1159. doi:10.1016/S0006-2952(97)00094-4
- Nakamura SI, Kawai T, Kamakura T, Ookura T. 2010. TGF-β3 is expressed in taste buds and inhibits proliferation of primary cultured taste epithelial cells. In Vitro Cell Dev Biol Anim. 46(1):36–44. doi:10.1007/s11626-009-9239-9
- Potten CS, Booth D, Haley JD. 1997. Pretreatment with transforming growth factor beta-3 protects small intestinal stem cells against radiation damage in vivo. Br J Cancer. 75(10):1454–1459. doi:10.1038/bjc.1997.249
- Robson H, Spence K, Anderson E, Potten CS, Hendry JH. 1997. Differential influence of TGFβ1 and TGFβ3 isoforms on cell cycle kinetics and postirradiation recovery of normal and malignant colorectal epithelial cells. Int J Radiat Oncol Biol Phys. 38(1):183–190. doi:10.1016/S0360-3016(97)00248-4
- Schneider CA, Rasband WS, Eliceiri KW. 2012. NIH Image to ImageJ: 25 years of image analysis. Nat Methods. 9(7):671–675. doi:10.1038/nmeth.2089
- Schrementi ME, Ferreira AM, Zender C, DiPietro LA. 2008. Site-specific production of TGF-β in oral mucosal and cutaneous wounds. Wound Repair Regen. 16(1):80–86. doi:10.1111/j.1524-475X.2007.00320.x
- Seong J, Kim SH, Chung EJ, Lee WJ, Suh CO. 2000. Early alteration in TGF-β mRNA expression in irradiated rat liver. Int J Radiat Oncol Biol Phys. 46(3):639–643. doi:10.1016/S0360-3016(99)00401-0
- Shah M, Foreman D, Ferguson MWJ. 1995. Neutralisation of TGF-β1 and TGF-β2 or exogenous addition of TGF-β3 to cutaneous rat wounds reduces scarring. J Cell Sci. 108(3):985–1002. doi:10.1242/jcs.108.3.985
- Shi Y, Massagué J. 2003. Mechanisms of TGF-B signaling from cell membrane to the nucleus. Cell. 113(6):685–700. doi:10.1016/s0092-8674(03)00432-x
- Siddiqui F, Movsas B. 2017. Management of radiation toxicity in head and neck cancers. Semin Radiat Oncol. 27(4):340–349. doi:10.1016/J.SEMRADONC.2017.04.008
- So K, McGrouther DA, Bush JA, Durani P, Taylor L, Skotny G, Mason T, Metcalfe A, O'Kane S, Ferguson MWJ. 2011. Avotermin for scar improvement following scar revision surgery: A randomized, double-blind, within-patient, placebo-controlled, phase II clinical trial. Plast Reconstr Surg. 128(1):163–172. doi:10.1097/PRS.0b013e318217429b
- Sonis ST, Elting LS, Keefe D, Peterson DE, Schubert M, Hauer-Jensen M, Bekele BN, Raber-Durlacher J, Donnelly JP, Rubenstein EB. 2004. Perspectives on cancer therapy-induced mucosal injury: pathogenesis, measurement, epidemiology, and consequences for patients. Cancer. 100(9 Suppl):1995–2025. doi:10.1002/CNCR.20162
- Sonis ST, Lindquist L, Van Vugt A, Stewart AA, Stam K, Qu G-Y, Iwata KK, Haley JD. 1994. Prevention of chemotherapy-induced ulcerative mucositis by transforming growth factor β3. Cancer Res. 54:1135–1138.
- Sonis ST, Van Vugt AG, Brien JP, Muska AD, Bruskin AM, Rose A, Haley JD. 1997. Transforming growth factor-β3 mediated modulation of cell cycling and attenuation of 5-fluorouracil induced oral mucositis. Oral Oncol. 33(1):47–54. doi:10.1016/s0964-1955(96)00043-7
- Sroussi HY, Epstein JB, Bensadoun R-J, Saunders DP, Lalla RV, Migliorati CA, Heaivilin N, Zumsteg ZS. 2017. Common oral complications of head and neck cancer radiation therapy: mucositis, infections, saliva change, fibrosis, sensory dysfunctions, dental caries, periodontal disease, and osteoradionecrosis. Cancer Med. 6(12):2918–2931. doi:10.1002/cam4.1221
- Straub JM, New J, Hamilton CD, Lominska C, Shnayder Y, Thomas SM. 2015. Radiation-induced fibrosis: mechanisms and implications for therapy. J Cancer Res Clin Oncol. 141(11):1985–1994. doi:10.1007/s00432-015-1974-6
- Taliaferro LP, Agarwal RK, Coleman CN, Andrea L, Hofmeyer KA, Loelius SG, Molinar-Inglis O, Tedesco DC, Satyamitra MM, Taliaferro LP. 2023. Sex differences in radiation research. Int J Radiat Biol. 100(3):466–485. doi:10.1080/09553002.2023.2283089
- Trotti A, Byhardt R, Stetz J, Gwede C, Corn B, Fu K, Gunderson L, McCormick B, Morrisintegral M, Rich T, et al. 2000. Common toxicity criteria: version 2.0. An improved reference for grading the acute effects of cancer treatment: impact on radiotherapy. Int J Radiat Oncol Biol Phys. 47(1):13–47. doi:10.1016/S0360-3016(99)00559-3
- Wang J, Robbins MEC. 1996. Radiation-induced alteration of rat mesangial cell transforming growth factor-β and expression of the genes associated with the extracellular matrix. Radiat Res. 146(5):561–568. doi:10.2307/3579557
- Wang K, Tepper JE. 2021. Radiation therapy‐associated toxicity: etiology, management, and prevention. CA Cancer J Clin. 71(5):437–454. doi:10.3322/caac.21689
- Wilson SE. 2021. TGF beta −1, −2 and −3 in the modulation of fibrosis in the cornea and other organs. Exp Eye Res. 207:108594. doi:10.1016/j.exer.2021.108594
- Wu Y, Peng Y, Gao D, Feng C, Yuan X, Li H, Wang Y, Yang L, Huang S, Fu X. 2015. Mesenchymal stem cells suppress fibroblast proliferation and reduce skin fibrosis through a TGF-β3-dependent activation. Int J Low Extrem Wounds. 14(1):50–62. doi:10.1177/1534734614568373
- Wymenga ANM, van der Graaf WTA, Hofstra LS, Spijkervet FKL, Timens W, Timmer-Bosscha H, Sluiter WJ, van Buuren A, Mulder NH, de VE. 1999. Phase I study of transforming growth factor-3 mouthwashes for prevention of chemotherapy-induced mucositis. Clin Cancer Res. 5:1363–1368.
- Xu L, Xiong S, Guo R, Yang Z, Wang Q, Xiao F, Wang H, Pan X, Zhu M. 2014. Transforming growth factor β3 attenuates the development of radiation-induced pulmonary fibrosis in mice by decreasing fibrocyte recruitment and regulating IFN-γ/IL-4 balance. Immunol Lett. 162(1 Pt A):27–33. doi:10.1016/J.IMLET.2014.06.010
- Xue K, Zhang J, Li C, Li J, Wang C, Zhang Q, Chen X, Yu X, Sun L, Yu X. 2019. The role and mechanism of transforming growth factor beta 3 in human myocardial infarction-induced myocardial fibrosis. J Cell Mol Med. 23(6):4229–4243. doi:10.1111/jcmm.14313
- Zhao W, O'Malley Y, Wei S, Robbins ME. 2000. Irradiation of rat tubule epithelial cells alters the expression of gene products associated with the synthesis and degradation of extracellular matrix. Int J Radiat Biol. 76(3):391–402. doi:10.1080/095530000138736