Abstract
Purpose
This comprehensive review aims to provide a unique clinical perspective on the latest advances and ongoing boron neutron capture therapy (BNCT) trials for various cancers.
Methods
We critically analyzed clinical data from BNCT trials for head and neck cancer, glioblastoma, melanoma, meningioma, breast cancer, and liver tumors. We investigated differences in tumor responses and normal tissue toxicities among trials and discussed potential contributing factors. We also identified the limitations of early BNCT trials and proposed strategies to optimize future trial design.
Results
BNCT has shown promising results in treating head and neck cancer, with high response rates and improved survival in patients with recurrent disease. In glioblastoma, BNCT combined with surgery and chemotherapy has demonstrated survival benefits compared to standard treatments. BNCT has also been successfully used for recurrent high-grade meningiomas and shows potential for melanomas, extramammary Paget’s disease, and liver tumors. However, differences in tumor responses and toxicities were observed among trials, potentially attributable to variations in treatment protocols, patient characteristics, and evaluation methods.
Conclusions
BNCT is a promising targeted radiotherapy for various cancers. Further optimization and well-designed randomized controlled trials are needed to establish its efficacy and safety. Future studies should focus on standardizing treatment protocols and addressing limitations to guide clinical decision-making and research priorities.
Introduction
Neutron capture therapy (NCT) is a form of targeted radiotherapy involving a two-step process. First, a stable isotope is selectively delivered to the tumor cells. Subsequently, the tumor is irradiated with low-energy neutrons, inducing a nuclear reaction that generates high-linear energy transfer (LET) particles and gamma rays, which leads to localized cell death. The two main types of NCT are boron neutron capture therapy (BNCT) and gadolinium neutron capture therapy (GdNCT), each of which utilizes different isotopes with unique nuclear capture reactions and emission profiles (Lee et al. Citation2022).
In 1932, James Chadwick, a researcher at the University of Cambridge, discovered the presence of boron. Soon after, Goldhaber found that bombarding boron with slow neutron production produced alpha particles. In 1936, Locher, a researcher at Swarthmore College, recognized Goldhaber’s therapeutic potential and proposed BNCT. From 1940 to 1951, numerous scientists conducted animal experiments to translate the BNCT concept into practice. Interested readers can refer to Sweet’s review for more comprehensive information on the early history of BNCT (Sweet Citation1997).
BNCT provides cell-level targeting by using non-radioactive isotope 10B that accumulates selectively in tumor cells. When low-energy neutrons are absorbed by isotope 10B, it triggers a reaction called boron capture therapy. This reaction causes the isotope to break down into an alpha particle and a 7Li nucleus, emitting particles that generate energy over a limited distance of 7-10 μm. This range happens to match the size of a cell, which allows for targeted destruction of cancer cells while leaving healthy cells unharmed () (Locher Citation1936). Secondly, α particles and 7Li nuclei exhibit high linear energy transfer (LET) radiation, increasing the relative biological effect (RBE). Charged particles can damage DNA directly, regardless of cancer cell oxygen content. Therefore, BNCT can potentially have a lethal effect on hypoxic tumors (Ono et al. Citation2019). Furthermore, patients typically undergo BNCT once or occasionally twice, with each treatment lasting 30-60 min.
Figure 1. Mechanisms of BNCT in cancer.
Patients were injected intravenously with a boron delivery agent before and sometimes during treatment (A). Cancer cells selectively absorb the boron-delivery agent with little uptake by normal tissues (B). The boron nucleus absorbs neutrons and undergoes nuclear fission, yielding an alpha particle and a 7Li. The range of the particle is 4-7 μm, equal to the diameter of a cell (C). Tumor cells were eliminated following BNCT therapy without causing considerable damage to normal tissue cells (D).
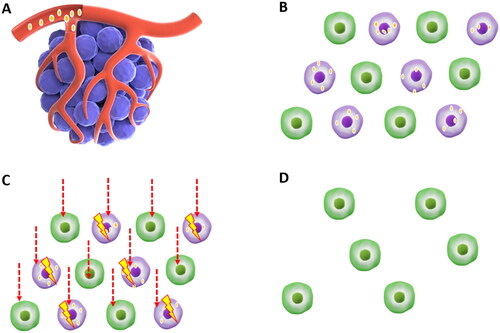
The neutron sources used in BNCT include reactor reactors and accelerator-based systems. A limited number of reactors remain operational, and many have been decommissioned. Advancements in accelerator neutron source technology have facilitated the rapid development of BNCT. Several countries, including Japan, Finland, Italy, South Korea, China, Great Britain, Russia, Israel, and Argentina, are constructing or planning BNCT centers () (Cartelli et al. Citation2020; Accelerator-based BNCT projects [date unknown]). In 2009, Sumitomo Heavy Industries, in collaboration with Kyoto University, Japan, achieved a significant milestone by successfully developing a cyclotron-based neutron source. Furthermore, in 2012 Kyoto University conducted the world’s first clinical trial of Accelerator-based- BNCT (AB-BNCT). In contrast, in 2020, AB-BNCT received approval to treat recurrent head and neck cancer in Japan, with coverage provided by health insurance. Finland had initially planned to commence the AB-BCNT clinical trials in 2023 (Porra et al. Citation2023). However, as of April 2024, we have not found evidence that they have officially initiated the clinical studies.
Figure 2. Global AB-BNCT projects: operational status of completed and under-construction facilities.
The figure illustrates the operational status of accelerator-based boron neutron capture therapy (AB-BNCT) projects worldwide that have been completed or are currently under construction. The data used to create this figure was obtained from the official website of the International Society for Neutron Capture Therapy (ISNCT) and is current as of the end of 2022.
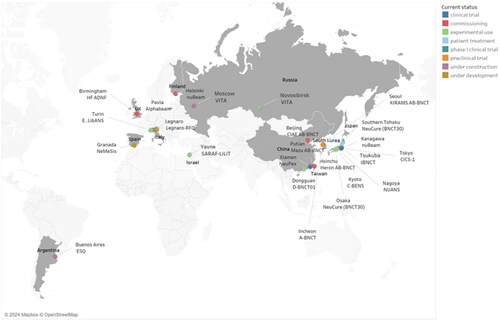
Several reviews have comprehensively summarized the evolution of boron delivery agents and neutron sources (Luderer et al. Citation2015; Barth et al. Citation2018; Farhood et al. Citation2018; Dymova et al. Citation2020). This article begins with an overview of BNCT clinical trials registered with authoritative organizations. Subsequently, we examined typical clinical studies focusing on efficacy and treatment-related adverse events, considering the anatomical site and pathological type of tumors.
Introduction to boron delivery agents
Boron compounds have extensive applications in the medical field, including antibacterial, anti-tuberculosis, anti-tumor, anti-parasitic, anti-protozoal, anti-inflammatory, anti-folate, anti-depressant, anti-allergic, anesthetic, and anti-Alzheimer’s drugs, as well as proteasome and lipogenesis inhibitors (Leśnikowski Citation2016; Das et al. Citation2022). They also play an essential role in neutron capture therapy (Xuan and Vicente Citation2018). In recent years, researchers have proposed two methods to enhance the effect of proton therapy using boron isotopes: proton–boron fusion therapy (P-BFT) using boron-11 agents and neutron capture-enhanced particle therapy (NCEPT) using boron-10 agents (Tabbakh and Hosmane Citation2020).
The boron element used in BNCT is 10B because 10B has a higher neutron capture cross-section of 3837 barns than 0.005 barns of 11B (Fithroni et al. Citation2022). Many studies consider that an ideal boron delivery agent should simultaneously meet the following four conditions: low toxicity; 10B concentration in the tumor exceeds 30 μg/g; its concentration in the cancer is much higher than that in normal tissues, generally considered tumor-to-normal tissue ratio (T/N) > 3; and it is rapidly cleared from the body after BNCT treatment (Sibrian-Vazquez and Vicente Citation2011; Takagaki et al. Citation2018; Ali et al. Citation2020). Unfortunately, no boron delivery agent met all these conditions. For convenience, we also briefly introduce and evaluate boron delivery agents by dividing them into three generations (), as in the literature.
Figure 3. Classification and structures of boron delivery agents for BNCT.
This figure illustrates the development of boron delivery agents for BNCT across three generations. The first-generation compounds, such as boric acid, borax, nanoparticles, and pentaborates, had low tumor selectivity and accumulation. The second generation introduced the clinically successful BPA and BSH, which exhibited improved tumor targeting and lower toxicity than the first generation. The third generation enhances tumor specificity and boron delivery by conjugating BPA and BSH with tumor-targeting moieties. These include amino acids, peptides, antibodies, porphyrins, liposomes, and oligonucleotides.
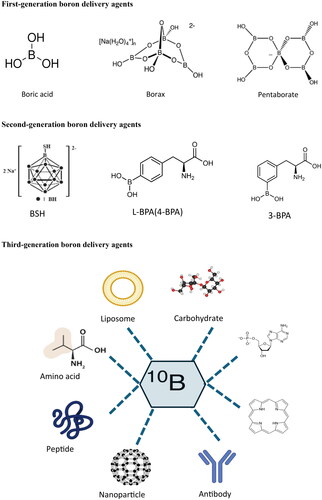
First-generation boron delivery agents
As early as the 1950s, compounds such as boric acid, borax, and pentaborate were synthesized. These small-molecule compounds lack tumor-targeting ability and are quickly metabolized out of the body, so they cannot accumulate in tumors. This is an essential reason for the unsatisfactory efficacy of early BNCT clinical studies.
Second-generation boron delivery agents
These include BPA and BSH (Lamba et al. Citation2021), two boron delivery agents approved by the FDA for BNCT clinical trials.
BSH
BSH is a cage-like polyhedral borane anion with each molecule containing 12 10B atoms. BSH can accumulate near brain tumors when the blood-brain barrier (BBB) is disrupted. It was first discovered by Soloway and Hatanaka in 1967 and was used by Dr. Mishima to treat glioblastoma in 1989 (Sauerwein et al. Citation2012). Owing to the lack of tumor cell-specific ligands, BSH has low T/N and tumor-to-blood ratio (T/B). Although the mechanism of boron concentration differences in different tissues is not entirely clear, it is generally believed that its small molecular size allows it to diffuse to various organs through blood perfusion and may be affected by physiological barriers such as the blood-brain barrier (Sauerwein et al. Citation2012).
In the European Organization for Research and Treatment of Cancer (EORTC) clinical trial 11961, the dosage of BSH was 100 mg/kg body weight. Pharmacokinetic analysis showed that the average clearance rate of boron in the blood was 19.8 mL per minute, and the terminal half-life ranged from 44.0 to 92.8 hours, indicating that the elimination of BSH in the body is relatively slow. Side effects that may occur when patients are infused with BSH, such as flushing and nausea, may be related to infusion rates exceeding 1 mg/kg/min (Hosmane Citation2012). In addition, the variability of boron concentrations in the blood of different patients is considerable, emphasizing the need for individual measurements of blood 10B concentrations during each patient’s irradiation to ensure the accuracy and safety of treatment.
BPA
BPA is a boron-containing compound designed for BNCT, including L-BPA (4-BPA) and the recently reported compound 3-BPA. L-BPA was synthesized by Snyder et al. in 1958 and was first used by Dr. Mishima to treat melanoma in 1989 (Mishima et al. Citation1989). The structure of L-BPA is highly similar to that of phenylalanine, allowing it to be selectively absorbed by tumor cells through the L-type amino acid transport system (LAT-1). BPA has good tumor cell targeting, with higher T/N and T/B values. L-BPA has been applied in BNCT treatment of various tumors, including melanoma, glioma, head and neck tumors, Paget’s disease, and breast cancer. Based on the positive results obtained from the JHN002 clinical study (Hirose et al. Citation2021), L-BPA (SPM-011) was approved for marketing in Japan in May 2020 under the name borofalan (Steboronine ®) for BNCT treatment of unresectable locally advanced or recurrent head and neck cancer (see below). Almost all ongoing clinical studies of AB-BNCT in Japan have adopted borofalan as a boron delivery agent, such as JPRN-jRCT2032230554 (JRCT Citation2024a), JPRN-jRCT2051210053 (JRCT Citation2024b), NCT04293289 (ClinicalTrials.gov Citation2000), JPRN-jRCT2031220410 (JRCT Citation2024c), JPRN-jRCT1080224974 (Mishima et al. Citation1989), JPRN-jRCT2051190044 (JRCT Citation2024d). L-BPA has a low boron content and requires high doses for treatment. It has poor water solubility and may cause hematuria due to crystallization in the urine (Kanno et al. Citation2021). L-BPA requires fructose or mannitol as the solvent, which limits its use in fructose-intolerant patients. Additionally, studies have shown that BPA is easily degraded by endogenous hydrogen peroxide, leading to loss of boronic acid groups and poor metabolic stability in vivo (Li et al. Citation2019).
3-BPA is the positional isomer of L-BPA. Comparative studies with L-BPA have shown that 3-BPA has similar biodistribution and tumor-targeting ability. The water solubility of 3-BPA is 125 g/L, more than 100 times that of L-BPA; therefore, it does not require solubilizing sugars during administration. Thus, 3-BPA is expected to replace L-BPA in the near future (Kondo et al. Citation2022).
Pharmacokinetic analysis showed that BPA clearance in vivo exhibited a biphasic process with a rapid redistribution phase and a slower elimination phase. When BPA is infused, the variability of blood boron concentrations in patients is considerable, emphasizing the need for individual measurements of blood (Dymova et al. Citation2020) B concentrations during each patient’s irradiation to ensure the accuracy and safety of treatment (Sauerwein et al. Citation2012).
18F-labeled L-BPA
When the boron concentration in a patient’s tumor reaches its maximum accumulation, it is a crucial issue for implementing BNCT, as this is the only way to maximize tumor cell killing while simultaneously optimizing the protection of normal tissues surrounding the tumor. Positron emission tomography (PET)-guided BNCT has the potential to overcome this challenge using dual-mode formulations.
In 1991, Ishiwata found that a hydrogen atom on the benzene ring of L-BPA can be replaced by 18F to obtain 18F-BPA (Ishiwata et al. Citation1991). Various examinations using 18F-BPA PET were reported in the latter part of the 1990s (Mishima et al. Citation1997; Imahori et al. Citation1998). 18F-BPA PET can help select patients with good prognoses and support dose distribution calculations. Studies have shown that the higher the 18F-BPA accumulation ratio between the tumor and normal organs, the better the anti-tumor effect and fewer adverse reactions (Nariai and Ishiwata Citation2012). Generally, the 18F-BPA accumulation ratio between the tumor and normal organs should be 2.5 or higher (Fukumitsu and Matsumoto Citation2021). However, the method used to achieve this measurement has not been standardized. In addition, other formulations, such as contrast agents for magnetic resonance imaging (boron-gadolinium compounds), are expected to be applied clinically (Shanmugam et al. Citation2023).
Third-generation boron delivery agents
Although BPA and BSH have achieved a specific efficacy in BNCT-treated patients, neither has reached the standard of an ‘ideal’ boron delivery agent, and we have described their shortcomings above. Chemists and pharmacists have been committed to synthesizing a variety of potentially highly efficient boron dosing agents. The core concept of the third-generation boron delivery agent design is to improve the tumor targeting of drugs, which has become one of the hottest directions in BNCT research. They are currently being tested in cell and animal models and have no application in clinical trials.
These delivery agents include boron-containing nucleosides (Khalil and Adam Citation2024), peptides (Nakase et al. Citation2020), polyamines (Ueda et al. Citation2021), porphyrins (Hiramatsu et al. Citation2011), liposomes (Li et al. Citation2022), monoclonal antibodies (Yamana et al. Citation2023), and various types of nanoparticles (Wu et al. Citation2006; Nakamura and Kirihata Citation2012; Ban and Nakamura Citation2018; Ali et al. Citation2020; Zhang et al. Citation2023). A recent review by Hong Xu et al. from China Pharmaceutical University introduced a new classification system for boron drugs used in BNCT based on their design strategies. This system categorizes drugs into four distinct types: Cell Membrane Targeting, which utilizes transport mechanisms such as LATs, glucose transporter (GLUT), and peptides to exploit the increased nutrient demands of tumor cells; Nuclear Targeting, where drugs interact with or integrate into DNA, targeting the nucleus for direct damage during neutron capture; Enhanced Permeability and Retention (EPR) effect, leveraging the looser vascular structures of tumors to accumulate large molecules such as liposomes, polymers, or nanoparticles; and Tumor Affinity, employing molecules such as porphyrins that naturally target and accumulate in tumor cells. This classification highlights the diverse mechanisms and design principles underlying the targeted actions of boron drugs in BNCT (Xu et al. Citation2024).
Early BNCT clinical exploration
In 1951, neurosurgeon WH Sweet conducted a BNCT trial on glioma at Massachusetts General Hospital, using borax as the boron delivery agent. Eighteen patients with gliomas were included in this study. The patients had a median overall survival [(mOS) defined as the time from randomization until death from any cause. The Median Overall Survival (mOS) refers to the survival time at which 50% of the study population has survived.)] for approximately three months (Asbury et al. Citation1972; Slatkin Citation1991). In 1968, Hatanaka from Teikyo University, part of Sweet’s research team, initiated a clinical study on BNCT brain tumors using sodium borocaptate (BSH). By 1995, 149 patients had undergone treatment in five reactors. This included 64 patients with glioblastoma (WHO grade IV), 39 with anaplastic astrocytoma (WHO grade III), 17 with low-grade astrocytoma (WHO grade I and II), and 30 with other types of cancer. The overall response rate [According to the Response Evaluation Criteria in Solid Tumors (RECIST) version 1.1, the efficacy of cancer treatment can be categorized into four levels: complete response (CR), stable disease (SD), partial response (PR), and progressive disease (PD). The overall response rate (ORR) is defined as the percentage of patients achieving either CR or PR among all treated patients.] (ORR) was 64% among glioblastoma patients, with 7 cases of glioblastoma, 22 cases of anaplastic astrocytoma, and 8 cases of low-grade astrocytoma surviving for over two years. The mOS for patients with glioblastoma, anaplastic astrocytoma, and low-grade astrocytoma was 640 days, 1811 days, and 1669 days, respectively. Six patients, two with glioblastomas and four with anaplastic astrocytomas survived for over ten years. Studies have indicated that the histological grade, patient age, neutron irradiation, neutron injection at the tumor target, and tumor target depth or size are important prognostic factors (Nakagawa and Hatanaka Citation1997).
A team led by Professor Mishima at Kobe University conducted a study that showed that Professor Mishima and his colleague at Kobe University have conducted a study that shows that L-p-boronophenylalanine (L-BPA) exhibits selective absorption by cancer cells, making it a potential boron delivery agent for clinical trials involving BNCT. The researchers treated 22 patients with melanoma and achieved a promising complete remission (CR) rate of 73% (16 22) (Fukuda et al. Citation2003).
Overview of recent BNCT clinical trials
Clinical trial registration originated in the 1990s when the United States Congress passed the Food and Drug Administration Modernization Act (FDAMA) in 1997. This legislation mandated the National Institutes of Health (NIH) to establish a database for registering NIH-funded clinical trials focused on severe or life-threatening diseases. The ClinicalTrials.gov website was officially launched in February 2000, marking the start of modern clinical trial registration. To understand the current landscape of BNCT clinical trials, we systematically searched the WHO International Clinical Trials Registry Platform using the keywords ‘BNCT’ or ‘neutron capture therapy of cancer.’ As of April 25, 2024, our search yielded 56 results. After excluding non-interventional studies, we categorized the remaining trials into two groups: reactor-based BNCT(RB-BNCT) and accelerator-based BNCT (AB-BNCT).
RB-BNCT clinical trials
Our analysis revealed that 30 clinical trials involving RB-BNCT have been registered globally, with Japan leading this way (16 trials). These trials encompass a broad spectrum of malignancies, including head and neck squamous cell carcinoma (SCC), skin angiosarcoma, brain tumors, breast cancer, glioblastoma, glioma, melanoma, pleural mesothelioma, and various skin malignancies (). Most of these trials were in phase I/II or II, underscoring the early stages of clinical development of RB-BNCT. L-BPA and BSH are the most frequently used boron carriers, with L-BPA being the most prevalent choice. The sample sizes of these trials exhibited considerable variability, ranging from one to 50 participants, with some trials not specifying the number of enrolled subjects. The recruitment status of these trials was also diverse, with some completed, some terminated, some actively recruited, and others yet to commence recruitment.
Table 1. Globally registered clinical trials of Reactor-based BNCT¶.
Notably, while 14 research reactors worldwide have previously conducted BNCT treatments (Moss Citation1993), most of these facilities have now been decommissioned because of various factors, such as funding constraints, management issues, and policy changes. At present, only a few institutions, such as the Kyoto University Research Reactor Institute (KURRI), Kyoto University Reactor (KUR) in Japan, and National Tsing Hua University (NTHU) with its Tsing Hua Open-pool Reactor (THOR) in Hsinchu, Taiwan, are actively pursuing RB-BNCT clinical research.
AB-BNCT clinical trials
Japan emerged as a frontrunner in AB-BNCT clinical trials, accounting for 10 of 12 registered studies worldwide. Renowned institutions, such as Osaka Medical and Pharmaceutical University, Southern Tohoku BNCT Research Center, National Cancer Center Hospital, Edogawa Hospital, and the University of Tsukuba, spearheaded these trials. The most common malignancies investigated in these trials included head and neck SCC, glioma, meningioma, malignant melanoma, angiosarcoma, and breast cancer. Most Japanese trials were in phase I or II, with sample sizes ranging from five to 120 participants. The neutron sources employed in these trials were BNCT30, CICS-1, CICS-2, and iBNCT001, whereas SPM-011 was consistently used as the boron carrier.
China and South Korea have also ventured into AB-BNCT clinical research, each with one registered trial. The Chinese trial, spearheaded by Xiamen Honghai Hospital, focused on recurrent head and neck cancer and primary brain tumors using a NeuPex neutron source and NBB-001 boron carrier. Meanwhile, the South Korean Gachon University Gil Medical Center trial investigated recurrent gliomas using a DM-BNCT neutron source and DMX-102 boron carrier ().
Table 2. Globally registered clinical trials of AB-BNCT.
Clinical study of BNCT for different types of tumors
Head and neck cancer
Head and neck cancer is the seventh most prevalent malignancy worldwide, with more than 660,000 new diagnoses and 325,000 deaths reported annually (Gormley et al. Citation2022). SCC accounts for approximately 90% of all head and neck cancer cases (Vigneswaran and Williams Citation2014). Between 50% and 60% of patients who undergo standard therapy may experience recurrence or metastasis within two years (Sacco and Cohen Citation2015),. The treatment of recurrent cancer poses a significant challenge (Chow Citation2020). In cases where local recurrence cannot be effectively treated with salvage surgery or radiotherapy, the prognosis is unfavorable, and untreated patients typically survive for only 6– 9 months (Chow Citation2020). shows the curative effect of BNCT on recurrent head and neck cancer.
Table 3. BNCT clinical trial of head and neck squamous cell carcinoma.
Sixty-two patients underwent BNCT at Kyoto University, Japan. The BNCT dose administered to the skin and oral mucosa was less than 10–12 Gy-Eq. Within six months of the procedure, the ORR of BNCT was 58% within six months after the procedure. The one-year and two-year survival (OS) rates were 43.1% and 24.2%, respectively. The most common acute grade 3 and 4 toxicities observed were hyperamylasemia (38.6%), fatigue (6.5%), mucositis/stomatitis (9.7%), and pain (9.7%). However, all of these toxicities are manageable and controllable (Suzuki et al. Citation2014).
At Osaka University, Japan, 26 patients with recurrent head and neck cancer, including 19 with squamous cell carcinoma, 4 with salivary adenocarcinoma, and 3 with sarcoma, underwent treatment with BNCT. Among these patients, 12 achieved CR, and 10 achieved partial response (PR) (Kato et al. Citation2009).
The University of Tsukuba, Japan University of Tsukuba, conducted a study involving 20twenty patients with head and neck cancer, including 11 patients with non-squamous non-squamous cell carcinoma treated with BNCT. After a five-month follow-up, 11 patients achieved CR, seven earned PR, and two had stable disease (SD). The mean mOS for the patients was 18.8 months. Notably, no severe acute or chronic normal tissue response was observed in these patients (Aihara et al. Citation2014).
The Helsinki University Central Hospital and Finland Helsinki University Central Hospital conducted a study involving 30 patients with recurrent head and neck cancer who had previously undergone photon RT. These patients were treated with BNCT between 2003 and 2008 and registered under the trial identifier NCT00114790. Among the patients, 26 were treated once with BNCT, whereas four received two treatments. Thirteen patients achieved CR, nine had PR, and six had SD. The median progression-free survival (mPFS) was 7.5 months, ranging: from 5.4 to 9.6 months. The 2-year progression-free survival (PFS) and OS rates were 20% and 30%, respectively.
Additionally, 27% of patients survived for two years without local recurrence. The most frequent acute adverse effects reported were mucositis (54%), mouth discomfort (54%), and weariness (Kankaanranta et al. Citation2012). The second study enrolled 79 patients with inoperable recurrent head and neck SCC from 2003 to 2012. Among these patients, 95% had previously received radiation therapy, and 71% had undergone surgery. Forty patients underwent a single treatment with BNCT, while 39 received two treatments, with a median interval gap of six weeks between sessions. The CR rate was 36%, the PR rate was 32%, and 25% of the patients had SD. Notably, the study found that BNCT fractionated treatment was more effective than a single treatment, with a CR rate of 50% compared to 19% in the single treatment group. Additionally, the PR rate was 29% in the fractionated treatment group compared with 36% among those who received a single treatment.
The Taiwan Veterans General Hospital enrolled 17 patients with recurrent head and neck cancer who had previously undergone photon RT. Of these, 15 patients underwent BNCT twice, and two underwent therapy once. The initial prescribed dose was 19.8 Gy-Eq, followed by a second dose of 14.6 Gy-Eq. Six of the total patients achieved CR, and another six earned PR. The local control rate at two years was 28%, and the OS rate at the same interval was 47%. One patient with recurrent hypopharyngeal cancer developed grade 4 laryngeal edema accompanied by carotid bleeding (Wang et al. Citation2018).
In 2020, Southern TOHOKU Hospital, Japan, presented the outcomes of the accelerator-based BNCT (AB-BNCT) clinical trial JHN002 (Hirose et al. Citation2021). The primary inclusion criteria were patients with recurrent head and neck SCC post-photon radiotherapy and those with non-squamous non-squamous cell carcinoma, who were either ineligible for surgery or had relapsed post-treatment. The participants were administered 400 mg/kg BPM-011BPA, followed by AB-BNCT. The cancer irradiation dose prescribed for the tumor was adjusted based on the maximum mucosal dose of 12 Gy-Eq. The cancer irradiation dose was adjusted based on the maximum mucosal dose of 12 Gy-Eq. Of the 21 enrolled patients who completed BNCT, 8 had squamous cell carcinoma, and 13 had non-squamous cell carcinoma. The primary endpoint ORR was 71.4% (15/21), including a CR rate of 23.8% and PR of 47.6%, which is markedly superior to the efficacy of immune checkpoint inhibitors (ORR < 20% (Borel et al. Citation2020; Kao and Lou Citation2019)). Secondary endpoints showed 1- and 2-year PFS rates of 70.6% and 60.5%, respectively, and the 1-year and 2-year OS rates were 94.7% and 85.3%, respectively. There was a 5% (one case) incidence of grade 3 oral mucositis. There were no grade 4 or 5 adverse effects (AEs) apart from temporary hyperamylasemia. Remarkably, the AE rate in this trial was lower than that in previous AB-BNCT studies, possibly because of advanced treatment planning processes, such as target delineation, dose prescription, beam adjustment, and placement optimization (Hirose et al. Citation2021).
Based on the results of this phase II clinical study, the Japan Greenlit BNCT system was developed by Sumitomo Heavy Industry Co., Ltd., Tokyo, and Steboronine®, a boron-based drug crafted by STELLA Pharmaceutical Company, was designed by Sumitomo Heavy Industry Co., March 2020. Updated safety data from BNCT in March 2022 revealed a median recovery time of 23 days for oral mucositis, 40 days for dermatitis, 58 days for one instance of alopecia areata, and 156 days for another (Hirose et al. Citation2022).
Following the success of this trial, Japan approved the BNCT system and Steboronine® for treating inoperable recurrent head and neck tumors in March 2020. A new phase II clinical trial (UMIN000044118) with a larger sample size of 120 patients is ongoing to evaluate the efficacy and safety of AB-BNCT using SPM-011 in patients with recurrent head and neck squamous cell carcinoma (UMIN Citation2021). In China, Xiamen Hongai Hospital has registered a phase I/II clinical trial (ChiCTR2200066473) investigating the safety, tolerability, and preliminary efficacy of AB-BNCT using a NeuPex neutron source and NBB-001 boron carrier in patients with recurrent head and neck cancer and primary brain tumors (ChiCTR Citation2023). This study aimed to enroll 12 patients and is expected to be completed by December 2024.
Additionally, case studies have documented the use of BNCT for newly diagnosed head and neck cancer. Teruhito Aihara and colleagues from Kawasaki Medical School treated five salivary gland cancer patients. Remarkably, all patients achieved CR within six months post-treatment, with a median CR duration of 24 months and an mOS of 32 months. Leena Kankaanranta and her team from Helsinki University Central Hospital, Finland Helsinki University Central Hospital, managed a patient with an extensive nasal cavity and paranasal sinus carcinoma. The cancer was eradicated post-treatment, and the patient’s optic nerve function remained intact (Kankaanranta et al. Citation2011). Additionally, Kimura Y and associates from Osaka Medical College, JapanOsaka Medical College, used BNCT to treat a 78-year-old patient with a newly diagnosed papillary cystadenocarcinoma of the upper lip, resulting in the complete disappearance of the tumor (Kimura et al. Citation2009).
Glioblastoma
Based on the WHO classification, glioblastoma (GBM) is the most common and aggressive brain tumor. For patients with GBM who are unable to undergo surgery, the mOS is only four months. In the standard post-treatment, the 1-year OS rate was 41.4%, with an mOS of approximately 14 months (Tan et al. Citation2020). The primary reason for resistance to photon radiotherapy is the lack of oxygen in cancerous tissues (Chédeville and Madureira Citation2021). Given that BNCT's efficacy is not contingent on the tumor’s intracellular oxygen content, it presents a promising method for GBM treatment.
Since the 1990s, RB-BNCT has been used to treat newly diagnosed recurrent GBM (). To enhance its efficacy, Japan has refined trial protocols in the following ways: (i) concomitant use of BPA and BSH, (ii) supplemental photon radiotherapy, and (iii) a combination of temozolomide and bevacizumab. Employing these strategies, the mOS for primary GBM patients has risen from 23.5 to 27.1 months, significantly improving over standard treatments (Stupp et al. Citation2005; Gilbert et al. Citation2014). Data from Tsukuba University and Osaka Medical College (Tamura et al. Citation2006; Miyatake et al. Citation2007) indicated that intraoperative BNCT offers no survival benefit compared to postoperative BNCT in patients with GBM.
Table 4. Clinical outcomes of RB-BNCT in the treatment of newly diagnosed and recurrent GBM.
JG002 represents Japan’s latest open-label multicenter phase II clinical trial that employed AB-BNCT to treat recurrent GBM (Miyatake et al. Citation2020). Preliminary results were obtained at the 2020 ASCO meeting in 2020. This study enrolled 24 patients with GBM between February 2016 and June 2018, the study enrolled 24 GBM patients, achieving a 1-year OS rate of 79.2% and an mOS of 18.7 months.
The Gachon University Gil Medical Center in South Korea has also initiated a phase I/IIa clinical trial (NCT05737212) to investigate the safety and efficacy of AB-BNCT using the DM-BNCT neutron source and DMX-101 boron carrier in patients with recurrent high-grade gliomas, including GBM (ClinicalTrials.gov Citation2023). The trial aimed to enroll 39 patients and explore the adequate radiation dose level for AB-BNCT based on confirming the maximum tolerated dose. The estimated completion date is December 2024.
Long-term survivors face a potential risk of developing secondary primary cancers; however, in Japan, the likelihood of this occurrence remains exceedingly low. Since 1968, of the 180 patients diagnosed with malignant brain tumors, only one developed multiple meningiomas within the BNCT target field (Kageji et al. Citation2015).
High-grade meningioma
Based on the 2016 World Health Organization classification, meningiomas are categorized into three grades: Grade I constitutes 80% of cases and typically has a favorable prognosis. Grade II (comprising 20%– 25%) and Grade III (encompassing 1%– 6%) meningiomas are defined as high-grade meningiomas (HGMs) and are associated with a less favorable prognosis. The prognosis for HGM cases that recurred after photon post-photon radiotherapy deteriorated further, with an mOS of 24.6 months and an mPFS of 5.2 months.
Osaka Medical College has extensively studied BNCT for recurrent HGM. Between 2005 and 2019, the institution treated 44 relapsed HGM cases using BNCT, and the results were disseminated across consecutive publications (Tamura et al. Citation2006; Miyatake et al. Citation2007; Takeuchi et al. Citation2018; Takai et al. Citation2022). For patients with WHO grade II (20 cases) and III (24 cases) HGM post-surgery, the mOS was 44.4 months and 21.55 months, respectively (p = .0009). The mPFS for WHO grade II and III HGM patients was 24.3 and 9.4 months, respectively (p = .0024). Notably, grade 2 and grade 3 radiation encephalitis occurred in 31.4% and 13.6% of the patients, respectively. Although it is often assumed that deeper tumors may not respond well to BNCT, seven patients with skull base cancers exhibited positive outcomes (Takeuchi et al. Citation2018).
Osaka Medical College, Japan, initiated a phase II clinical trial of AB-BNCT to treat HGM and patient enrollment commenced in April 2019 (JRCT2051190044) (Nakahara et al. Citation2020). This study randomized 18 patients with recurrent HGM into two groups: 12 underwent BNCT treatment, while the remaining six received the best supportive care (palliative care). The primary endpoint of this study was PFS.
Stenstam and colleagues from the Nykoping Hospital, Sweden, documented the successful treatment of two patients with recurrent HGM using BNCT (Stenstam et al. Citation2007). One patient exhibited a survival duration of 32 months after BNCT post-BNCT, whereas the other survived after a 27-month follow-up.
Brainstem tumor
Pediatric high-grade brainstem gliomas are rare tumors, accounting for 10-20% of all pediatric brain tumors. Patients with these tumors have a mean survival rate of only eight months. Multiple hospitals in Taiwan have documented the treatment of seven patients with terminal brainstem glioma who qualified for emergency and compassionate use. All these patients underwent two fractions of salvage BNCT, receiving doses of 7.22 Gy-Eq and 6.62 Gy-Eq prescribed to the skin, receiving doses of 7.22 Gy-Eq and 6.62 Gy-Eq, respectively. Among the seven patients, one achieved CR, and five achieved PR. Importantly, none of the patients experienced acute or delayed adverse events. Therefore, low-dose fractionated BNCT may be a promising, safe, and effective salvage therapy for patients with terminal brainstem glioma glioma patients (Chen et al. Citation2022).
Melanoma of the skin
Although melanoma accounts for approximately 10% of all skin cancers, it accounts for approximately 80% of skin malignancy-related deaths attributed to skin malignancies (Siegel et al. Citation2020). Radiotherapy is an essential treatment option for patients with melanoma who are unable or prefer not to undergo surgery, and radiotherapy is a vital treatment option (Fort et al. Citation2016). Melanomas are often resistant or insensitive to photon radiation (Im et al. Citation2020). In contrast, BNCT has shown notable potential for melanoma treatment.
Fukuda reviewed the treatment of 32 patients with cutaneous melanoma using L-BPA as a boron delivery agent at Ohio State University Fukuda reviewed the treatment of 32 patients with cutaneous melanoma using L-BPA as a boron delivery agent from 1987 to 2014. The overall CR rate was 78% (n = 25/32). This included a CR rate of 81% (22/27) for primary cancers and 60% (3/5) for metastatic cancer. Nodular melanoma has a CR rate of 33% (1/3), whereas non-nodular melanoma has a more impressive rate of 87.5% (21/24) (Barth et al. Citation2018).
Between 2003 and 2007, Argentina conducted a phase I/II BNCT clinical trial that enrolled seven patients with skin melanoma. The dose for the normal skin was capped between 16.5 and 24 Gy-Eq. The ORR and grade 3 skin toxicities were 69% and 69%, respectively, whereas grade 3 skin toxicities were recorded at 30% and (Menéndez et al. Citation2009).
Kawasaki Medical University administered RB-BNCT to eight patients with melanoma and monitored their progress. The age of the patients at the time of treatment ranged from 48 to 86 years old. Their tumors were located on the plantar or facial regions, were characterized by a clinical stage of cT1-2N0M0, and displayed no regional lymph node involvement. The prescribed minimum dose for the tumor was 25 Gy, whereas the maximum skin dose was 15 Gy. Remarkably, six of the eight patients achieved CR, and two attained PR, resulting in an ORR of 88%. Three patients succumbed to conditions such as pneumonia or factors related to old age that were not associated with melanoma. However, the remaining five patients continued to lead lives of good quality. No adverse events exceeding grade 2 in severity were reported as adverse events exceeding grade 2 in severity (Hiratsuka et al. Citation2020).
The Third Xiangya Hospital of Central South University, China, initiated a clinical trial for BNCT treatment targeting melanoma to enroll 30 patients (NCT02759536). However, this trial is still in progress (Yong et al. Citation2016). The latest public data indicate that at least 22 patients were enrolled (Wang et al. Citation2022).
The National Cancer Center Hospital in Japan recently completed a phase I study (NCT04293289) to evaluate BNCT drug CICS-1 (SPM-011) safety and efficacy in patients with primary melanoma or angiosarcoma (ClinicalTrials.gov Citation2000). The results of this study provide valuable insights into the potential of CICS-1 as a novel boron delivery agent for BNCT in melanoma and angiosarcoma treatment. Building on phase I results, a phase II trial (NCT05601232) was initiated to investigate further the efficacy and safety CICS-1 in patients with unresectable angiosarcomas (JRCT Citation2024c).
Breast cancer
Preliminary clinical data suggest that BNCT may be an effective treatment for locoregionally recurrent breast cancer (Kurosaki et al. Citation2024). In a case report from the University of Tokyo, Japan, a patient with recurrent breast cancer in the left axillary lymph nodes they have experienced a significant reduction in tumor size and pain relief two months after BNCT treatment with BPA without severe acute toxicities (JRCT Citation2020).
More recently, Edogawa Hospital, Japan, reported three patients with locoregionally recurrent breast cancer treated with BNCT using the CICS-2 accelerator and SPM-011 (Kurosaki et al. Citation2024). At 90 days post-treatment, one patient achieved a complete response, while the other two had partial tumor shrinkage. No grade 3 or higher adverse events were observed, and importantly, no evidence of radiation pneumonitis was detected on CT scans.
These early clinical results indicate that BNCT may be an effective and well-tolerated treatment option for recurrent breast cancer. Currently, a phase I clinical trial (jRCTs031220371) is underway at Edogawa Hospital, Japan, to evaluate the safety and efficacy of BNCT using the CICS-2 accelerator and SPM-011 for recurrent breast cancer following radiotherapy (JRCT Citation2022). The primary endpoint was the incidence of acute adverse events, while the secondary endpoints included the tumor response rate, progression-free survival, and overall survival. This trial, along with future studies, will provide further insights into the potential of BNCT as a treatment modality for recurrent breast cancer.
Extramammary Paget’s disease
Extramammary Paget’s disease (EMPD) predominantly involves the genitalia, perineum, and perianal regions. Although surgery is the primary treatment modality for EMPD, it often results in organ dysfunction, notably sexual dysfunction, which adversely affects the patient’s quality of life. BNCT is a viable alternative treatment for EMPD.
Kyoto University administered BNCT to three newly diagnosed EMPD patients, delivering a tumor dose ranging from 18 to 23 Gy-Eq and limiting the dose to the normal skin mucosa between 6.8 and 8.7 Gy-Eq. All three tumors were entirely eradicated within a six-month timeframe. However, the patient experienced mild-to-moderate radiation dermatitis and discomfort within two months post-BNCT, which resolved rapidly (Hiratsuka et al. Citation2018). BNCT offers an effective treatment for EMPD, ensuring the preservation of organ function.
Hepatocellular carcinoma and liver metastases
In 2009, Kyoto University documented a case of hepatocellular carcinoma (HCC) in a patient with hepatocellular carcinoma (HCC) who underwent BNCT and transcatheter arterial chemoembolization (TACE) (Suzuki et al. Citation2007). The patient had a history of hepatitis C cirrhosis and was categorized according to the Child-Pugh B liver function classification. While the right-lobed HCC was managed with BNCT, the left-lobed HCC was treated with TACE. Post-BNCT treatment, the lesion in the right lobe remained stable for 3.5 months.
The University of Pavia in Italy used BNCT to treat two patients who had metastatic liver cancer originating from the colon. In one patient, the tumor demonstrated size reduction and maintained stability for 20 months. Regrettably, another patient succumbed to cardiomyopathy 33 days after the treatment. An autopsy of this patient revealed considerable necrosis within the cancerous region (Zonta et al. Citation2009).
Hironobu Yanagie and colleagues from the University of Tokyo administered BNCT to a 63-year-old patient with multiple hepatocellular carcinomas in the left lobe of the liver. They used a water-in-oil emulsion containing BSH for treatment. Three months after BNCT, the patient’s cancer status remained stable, and there were no treatment-related adverse effects related to the treatment (Yanagie et al. Citation2014).
Because most patients with HCC have HCC from viral or alcoholic hepatitis, BNCT is a cellular-level radiation therapy that protects normal liver cells from irradiation and ensures treatment safety.
Other sarcomas
The National Cancer Center Hospital in Japan treated two elderly older women diagnosed with scalp hemangiosarcoma using AB-BNCT. Six months after treatment, the tumor completely disappeared. A follow-up study conducted 20 months later revealed no recurrence (Igaki et al. Citation2022). Futamura et al. of Osaka Medical College, Japan, successfully treated a photon radiotherapy-induced osteosarcoma of the left occipital bone with BNCT (Futamura et al. Citation2014). Masayoshi Inoue et al. of Osaka Medical School treated a recurrent cervical malignant nerve sheath tumor, which shrank and was maintained for 24 months after BNCT, significantly relieving brachial plexus compression symptoms (Inoue et al. Citation2010). Minoru Suzuki et al. of Kyoto University treated two patients with pleural mesothelioma and spindle cell tumors. During BNCT of the chest, two patients did not develop grade 2 or higher radiation pneumonia (Suzuki et al. Citation2008).
Differences in tumor response and normal tissue toxicity among BNCT clinical trials
The tumor response and normal tissue toxicity observed in BNCT clinical trials can vary owing to a complex interplay of factors, including tumor characteristics, treatment-related factors, differences in BNCT protocols, patient-specific genetic variations, and the study sample size.
Tumor factors, such as pathological type, grade, size, and depth can influence treatment outcomes. For instance, in head and neck cancer trials, patients with non-squamous cell carcinoma generally showed better responses than those with SCC. Similarly, in high-grade meningioma studies, patients with WHO grade II tumors had longer median overall survival and progression-free survival than those with grade III tumors.
Treatment-related factors, such as prior radiotherapy, chemotherapy, or concurrent use of antitumor drugs (e.g. bevacizumab, cetuximab, or temozolomide) and supplementary X-ray therapy during BNCT, can affect treatment efficacy and toxicity. For example, in glioblastoma trials, patients who received BNCT with concurrent temozolomide and X-ray therapy showed improved median overall survival compared to those who received BNCT alone.
Variations in BNCT protocols, including neutron sources, boron delivery agents, and prescription dose habits among clinical centers, can contribute to differences in treatment outcomes. The choice of boron delivery agent (e.g. BPA, BSH, or a combination of both) and administered dose can vary between studies.
Genetic variations can significantly affect radiotherapy’s sensitivity and side effects for cancer treatment. Studies have shown associations between specific genetic variations and the response to radiotherapy. For example, XRCC1 polymorphisms have been linked to cancer prognosis after radiotherapy (Gong et al. Citation2021). Additionally, polymorphisms in DNA repair genes, such as XPC, XPD, and XPG, have been associated with the acute side effects of radiotherapy in patients with head and neck cancer patients (Chang-Claude et al. Citation2005).
Furthermore, sample size can influence the assessment of efficacy and toxicity in BNCT clinical trials. Studies with smaller sample sizes may need help accurately assessing treatment effects and adverse event rates, while more extensive studies can provide more reliable results.
In conclusion, the observed differences in tumor response and normal tissue toxicity among BNCT clinical trials can be attributed to the complex interplay of factors related to tumor biology, treatment protocols, patient characteristics, genetic variations, and the study sample size. Future studies should aim to stratify patients based on these factors to optimize treatment efficacy and minimize toxicity. Additionally, large-scale multicenter clinical trials should be conducted to obtain more reliable evidence to support the clinical application of BNCT.
Limitations of early BNCT trial design and prospects of future trial
Early BNCT clinical trials faced several challenges and limitations that needed to be addressed to ensure the success of future studies and secure regulatory approval. One major issue is insufficient feasibility assessment, with factors such as patient recruitment difficulties and neutron source malfunctions often leading to premature trial termination. This highlights the need for thorough feasibility evaluations before initiating clinical trials to minimize the risk of study discontinuation. Another limitation lies in the design of the clinical studies, particularly in phase I/II trials. The objectives of these early phase trials often need to be clarified, with numerous observation indicators that may not be relevant to the study’s primary goals. To address this issue, future trials should have well-defined objectives and focus on the most pertinent endpoints for each developmental phase. Moreover, BNCT clinical research differs from traditional drug or radiotherapy device studies because it involves a complex combination of hardware, software, and pharmaceutical components. Consequently, effective communication and collaboration among stakeholders, including regulatory authorities responsible for medical devices and drug clinical trials, clinical physicians, physicists, pharmacists, and nuclear medicine experts, is essential for successfully designing and evaluating BNCT clinical trials.
Currently, most BNCT clinical studies aim to verify safety and determine the appropriate dosing (drug and radiation doses) using small, open-label phase I/II trials involving multiple tumor types. Although these studies have provided valuable insights, they have yet to comprehensively assess the efficacy of BNCT. Randomized controlled trials (RCTs) in phase III are the gold standard for evaluating the effectiveness and safety of new treatment modalities, building upon the foundation of phase I/II studies. RCTs require rigorous trial design, strict inclusion and exclusion criteria, adequate sample sizes, and long-term follow-up to generate the most persuasive evidence.
OS is the ideal endpoint for assessing the efficacy of cancer treatments, as temporary tumor shrinkage may not necessarily translate into prolonged OS, a phenomenon frequently observed in anticancer drug research. RCTs are essential to establish BNCT as a viable cancer treatment option.
For BNCT RCTs, it is crucial to use accelerator-based neutron sources to ensure consistency and reproducibility across study sites. The initial study population consisted of patients who failed or could not tolerate conventional treatments, with a focus on specific tumor locations and types. As the evidence base grows, the study population can gradually expand to include patients with newly diagnosed cancers. The control group received the current standard of care recommended for the investigated cancer types.
In addition to RCTs, long-term follow-up studies are necessary to assess the durability of treatment responses and monitor the potential late toxicities associated with BNCT. These studies provide valuable information about treatment’s long-term benefits and risks, which is crucial for guiding clinical decision-making and securing regulatory approval.
To optimize the efficiency and informativeness of BNCT clinical trials, innovative trial designs and statistical methods such as adaptive designs and Bayesian approaches should be incorporated. These approaches can help accelerate the development and approval of BNCT by allowing seamless integration of new data and rapid identification of promising treatment strategies.
In conclusion, while early BNCT clinical trials have provided important insights, they have also revealed several limitations that must be addressed to ensure the success of future studies and to secure regulatory approval. By conducting thorough feasibility assessments, designing trials with well-defined objectives, fostering collaboration among stakeholders, and employing rigorous methodologies, such as RCTs and long-term follow-up studies, future BNCT clinical trials can generate high-quality evidence to support the treatment’s efficacy and safety, ultimately leading to improved outcomes for cancer patients.
Authors’ contributions
Nianfei Wang was the one who came up with the idea and designed the study. Shumin Shen, Shanghu Wang, Xiuwei Wu and Dachen Zhou searched the literature and analyzed the data. Mingzhu Gao, Jinjin Wu and Yucai Yang were in charge of visualizing the data. Shanghu Wang, Xiaoxi Pan, and Shumin Shen wrote the paper. Nianfei Wang critically revised it for important intellectual content. All the authors read and approved the final manuscript.
Declaration of generative AI and AI-assisted technologies in the writing process
The authors confirm that no generative AI- or AI-assisted technologies were used in the writing of this manuscript or analysis of the data presented.
IctrpResults.csv
Download Comma-Separated Values File (155 KB)Graphical Abstract(clean).docx
Download MS Word (420 B)Highlights(clean).docx
Download MS Word (412 B)Acknowledgments
We would like to express our gratitude to Dr. Xian Ji from the International Academy of Neutron Science, Qingdao, China, for his expert guidance on BNCT and English language editing. His thoughtful feedback significantly improved this work.
Disclosure of interest
The authors declare no conflicts of interest.
Data availability statement
These data are made available upon request.
Additional information
Funding
Notes on contributors
Shumin Shen
Shumin Shen, MM, is conducting basic and clinical research in oncology radiotherapy at Anhui Medical University.
Shanghu Wang
Shanghu Wang, PhD, is a Deputy Director of Radiotherapy Department, specializing in clinical research on radiotherapy for lung and esophageal cancer and a Sub-PI for multiple Phase II and III clinical trials.
Dachen Zhou
Dachen Zhou, MD, is specializing in liver cancer and liver transplantation. Previously conducted tumor radiophysics research at Heidelberg University, Germany.
Xiuwei Wu
Xiuwei Wu, MD, is focusing on chemotherapy, immunotherapy, and endocrine therapy in oncology.
Mingzhu Gao
Mingzhu Gao, MD, is specializing in chemotherapy and immunotherapy for digestive system tumors.
Jinjin Wu
Jinjin Wu, MD, is conducting basic research on colon cancer pathogenesis and clinical translational studies.
Yucai Yang
Yucai Yang, MD, is engaged in basic and clinical translational research on nanomaterials in oncology.
Xiaoxi Pan
Xiaoxi Pan, PhD, is focusing on basic and clinical research in radionuclides.
Nianfei Wang
Nianfei Wang, PhD, is specializing in chemoradiotherapy, with a focus on basic and clinical research in digestive system tumors, particularly colorectal cancer and hepatocellular carcinoma.
References
- Accelerator-based BNCT projects. [date unknown]. [accessed 2024 Apr 20]. https://isnct.net/bnct-boron-neutron-capture-therapy/accelerator-based-bnct-projects-2021/.
- Aihara T, Morita N, Kamitani N, Kumada H, Ono K, Hiratsuka J, Harada T. 2014. BNCT for advanced or recurrent head and neck cancer. Appl Radiat Isot. 88:12–15. doi:10.1016/j.apradiso.2014.04.007
- Ali F, S Hosmane N, Zhu Y. 2020. Boron chemistry for medical applications. Molecules. 25(4):828. doi:10.3390/molecules25040828
- Asbury AK, Ojemann RG, Nielsen SL, Sweet WH. 1972. Neuropathologic study of fourteen cases of malignant brain tumor treated by boron-10 slow neutron capture radiation. J Neuropathol Exp Neurol. 31(2):278–303. doi:10.1097/00005072-197204000-00005
- Ban HS, Nakamura H. 2018. Recent development of nanoparticle-based boron delivery systems for neutron capture therapy. In: Hosmane NS, editor. Handbook of boron science: with applications in organometallics, catalysis, materials and medicine. Singapore: World Scientific Publishing; p. 49–68.
- Barth RF, Mi P, Yang W. 2018. Boron delivery agents for neutron capture therapy of cancer. Cancer Commun (Lond). 38(1):35. doi:10.1186/s40880-018-0299-7
- Barth RF, Zhang Z, Liu T. 2018. A realistic appraisal of boron neutron capture therapy as a cancer treatment modality. Cancer Commun (Lond). 38(1):36. doi:10.1186/s40880-018-0280-5
- Borel C, Jung AC, Burgy M. 2020. Immunotherapy breakthroughs in the treatment of recurrent or metastatic head and neck squamous cell carcinoma. Cancers (Basel). 12(9):2691. doi:10.3390/cancers12092691
- Cartelli DE, Capoulat ME, Baldo M, Sandín JCS, Igarzabal M, Grosso MFD, Valda AA, Canepa N, Gun M, Minsky DM, et al. 2020. Status of low-energy accelerator-based BNCT worldwide and in Argentina. Appl Radiat Isot. 166:109315. doi:10.1016/j.apradiso.2020.109315
- Chang-Claude J, Popanda O, Tan X-L, Kropp S, Helmbold I, von Fournier D, Haase W, Sautter-Bihl ML, Wenz F, Schmezer P, et al. 2005. Association between polymorphisms in the DNA repair genes, XRCC1, APE1, and XPD and acute side effects of radiotherapy in breast cancer patients. Clin Cancer Res. 11(13):4802–4809. doi:10.1158/1078-0432.CCR-04-2657
- Chédeville AL, Madureira PA. 2021. The role of hypoxia in glioblastoma radiotherapy resistance. Cancers (Basel). 13(3):542. doi:10.3390/cancers13030542
- Chen Y-W, Lee Y-Y, Lin C-F, Huang T-Y, Ke S-H, Mu P-F, Pan P-S, Chen J-K, Lan T-L, Hsu P-C, et al. 2022. Compassionate treatment of brainstem tumors with boron neutron capture therapy: a case series. Life (Basel). 12(4):566. doi:10.3390/life12040566
- ChiCTR. 2023. A Single-center, Single-arm Clinical Trial of the Safety and Efficacy of Boron Neutron Capture Therapy (BNCT) for Advanced Refractory Malignant Tumors. https://www.chictr.org.cn/showproj.html?proj=181032.
- Chow LQM. 2020. Head and neck cancer. N Engl J Med. 382(1):60–72. doi:10.1056/NEJMra1715715
- ClinicalTrials.gov. 2000. Phase 1 Clinical Trial of Boron Neutron Capture Therapy (BNCT) Using CICS-1 and SPM-011 for Malignant Melanoma and Angiosarcoma. https://clinicaltrials.gov/show/NCT04293289.
- ClinicalTrials.gov. 2023. A multi-centered, radiation dose escalation, open, exploratory, phase 1/2a clinical trial on the safety, efficacy and pharmacokinetic characteristics of BNCT(boron neutron capture therapy) in patients with recurrent high-grade gliomas. https://clinicaltrials.gov/ct2/show/NCT05737212.
- Das BC, Nandwana NK, Das S, Nandwana V, Shareef MA, Das Y, Saito M, Weiss LM, Almaguel F, Hosmane NS, et al. 2022. Boron chemicals in drug discovery and development: synthesis and medicinal perspective. Molecules. 27(9):2615. doi:10.3390/molecules27092615
- Dymova MA, Taskaev SY, Richter VA, Kuligina EV. 2020. Boron neutron capture therapy: Current status and future perspectives. Cancer Commun (Lond). 40(9):406–421. doi:10.1002/cac2.12089
- Farhood B, Samadian H, Ghorbani M, Zakariaee SS, Knaup C. 2018. Physical, dosimetric and clinical aspects and delivery systems in neutron capture therapy. Rep Pract Oncol Radiother. 23(5):462–473. doi:10.1016/j.rpor.2018.07.002
- Fithroni AB, Kobayashi K, Uji H, Ishimoto M, Akehi M, Ohtsuki T, Matsuura E. 2022. Novel self-forming nanosized DDS particles for BNCT: utilizing a hydrophobic boron cluster and its molecular glue effect. Cells. 11(20):3307. doi:10.3390/cells11203307
- Fort M, Guet S, Husheng S, Calitchi E, Belkacemi Y. 2016. Role of radiation therapy in melanomas: Systematic review and best practice in 2016. Crit Rev Oncol Hematol. 99:362–375. doi:10.1016/j.critrevonc.2016.01.016
- Fukuda H, Hiratsuka J, Kobayashi T, Sakurai Y, Yoshino K, Karashima H, Turu K, Araki K, Mishima Y, Ichihashi M, et al. 2003. Boron neutron capture therapy (BNCT) for malignant melanoma with special reference to absorbed doses to the normal skin and tumor. Australas Phys Eng Sci Med. 26(3):97–103. doi:10.1007/BF03178777
- Fukumitsu N, Matsumoto Y. 2021. Development of an imaging technique for boron neutron capture therapy. Cells. 10(8):2135. doi:10.3390/cells10082135
- Futamura G, Kawabata S, Siba H, Kuroiwa T, Suzuki M, Kondo N, Ono K, Sakurai Y, Tanaka M, Todo T, et al. 2014. A case of radiation-induced osteosarcoma treated effectively by boron neutron capture therapy. Radiat Oncol. 9(1):237. doi:10.1186/s13014-014-0237-z
- Gilbert MR, Dignam JJ, Armstrong TS, Wefel JS, Blumenthal DT, Vogelbaum MA, Colman H, Chakravarti A, Pugh S, Won M, et al. 2014. A randomized trial of bevacizumab for newly diagnosed glioblastoma. N Engl J Med. 370(8):699–708. doi:10.1056/NEJMoa1308573
- Gong L, Luo M, Sun R, Qiu L, Chen C, Luo Z. 2021. Significant association between XRCC1 expression and its rs25487 polymorphism and radiotherapy-related cancer prognosis. Front Oncol. 11:654784. doi:10.3389/fonc.2021.654784
- Gormley M, Creaney G, Schache A, Ingarfield K, Conway DI. 2022. Reviewing the epidemiology of head and neck cancer: definitions, trends and risk factors. Br Dent J. 233(9):780–786. doi:10.1038/s41415-022-5166-x
- Hiramatsu R, Kawabata S, Miyatake S, Kuroiwa T, Easson MW, Vicente MG. 2011. Application of a novel boronated porphyrin (H(2)OCP) as a dual sensitizer for both PDT and BNCT. Lasers Surg Med. 43(1):52–58. doi:10.1002/lsm.21026
- Hiratsuka J, Kamitani N, Tanaka R, Tokiya R, Yoden E, Sakurai Y, Suzuki M. 2020. Long-term outcome of cutaneous melanoma patients treated with boron neutron capture therapy (BNCT). J Radiat Res. 61(6):945–951. doi:10.1093/jrr/rraa068
- Hiratsuka J, Kamitani N, Tanaka R, Yoden E, Tokiya R, Suzuki M, Barth RF, Ono K. 2018. Boron neutron capture therapy for vulvar melanoma and genital extramammary Paget’s disease with curative responses. Cancer Commun (Lond). 38(1):38. doi:10.1186/s40880-018-0297-9
- Hirose K, Konno A, Hiratsuka J, Yoshimoto S, Kato T, Ono K, Otsuki N, Hatazawa J, Tanaka H, Takayama K, et al. 2021. Boron neutron capture therapy using cyclotron-based epithermal neutron source and borofalan ((10)B) for recurrent or locally advanced head and neck cancer (JHN002): An open-label phase II trial. Radiother Oncol. 155:182–187. doi:10.1016/j.radonc.2020.11.001
- Hirose K, Sato M, Kato T, Takayama K, Suzuki M, Yamaguchi H, Seto I, Kikuchi Y, Murakami M, Takai Y, et al. 2022. Profile analysis of adverse events after boron neutron capture therapy for head and neck cancer: a sub-analysis of the JHN002 study. J Radiat Res. 63(3):393–401. doi:10.1093/jrr/rrac012
- Hosmane NS, Maguire JA, Zhu Y, Takagaki M. 2012. Major neutron capture therapy (NCT) drug prototypes. In: Hosmane NS, Maguire JA, Zhu Y, Takagaki M, editors. Boron and gadolinium neutron capture therapy for cancer treatment. Singapore: World Scientific Publishing; p. 41–98.
- Igaki H, Murakami N, Nakamura S, Yamazaki N, Kashihara T, Takahashi A, Namikawa K, Takemori M, Okamoto H, Iijima K, et al. 2022. Scalp angiosarcoma treated with linear accelerator-based boron neutron capture therapy: A report of two patients. Clin Transl Radiat Oncol. 33:128–133. doi:10.1016/j.ctro.2022.02.006
- Im H, Lee J, Ryu KY, Yi JY. 2020. Integrin alphavbeta3-Akt signalling plays a role in radioresistance of melanoma. Exp Dermatol. 29(6):562–569. doi:10.1111/exd.14102
- Imahori Y, Ueda S, Ohmori Y, Sakae K, Kusuki T, Kobayashi T, Takagaki M, Ono K, Ido T, Fujii R, et al. 1998. Positron emission tomography-based boron neutron capture therapy using boronophenylalanine for high-grade gliomas: part I. Clin Cancer Res. 4(8):1825–1832.
- Inoue M, Lee CM, Ono K, Suzuki M, Tokunaga T, Sawa Y, Okumura M. 2010. Clinical effectiveness of boron neutron capture therapy for a recurrent malignant peripheral nerve sheath tumor in the mediastinum. J Thorac Oncol. 5(12):2037–2038. doi:10.1097/JTO.0b013e3181f1cd86
- Ishiwata K, Ido T, Mejia AA, Ichihashi M, Mishima Y. 1991. Synthesis and radiation dosimetry of 4-borono-2-[18F]fluoro-D,L-phenylalanine: a target compound for PET and boron neutron capture therapy. Int J Rad Appl Instrum A. 42(4):325–328. doi:10.1016/0883-2889(91)90133-l
- JRCT. 2020. Pilot clinical study of boron neutron capture therapy on post-radiotherapy, recurrent breast cancer (BNCTonrBC). https://jrct.niph.go.jp/en-latest-detail/jRCTs051180219?utm_medium=email&utm_source=transaction.
- JRCT. 2022. Pilot study of boron neutron capture therapy for recurrent breast cancer after radiotherapy. https://jrct.niph.go.jp/en-latest-detail/jRCTs031220371.
- JRCT. 2024a. Phase I clinical BNCT Trial to evaluate the safty of iBNCT001 and SPM-011, Accelerator-Based novel High-Power Neutron Source in patient with newly diagnosed glioblastoma. https://jrct.niph.go.jp/latest-detail/jRCT2032230554.
- JRCT. 2024b. An open label dose escalation study to investigate the safety and tolerability of boron neutron capture therapy (BNCT) with SPM-011 and BNCT treatment system (BNCT30) in patients with recurrent malignant glioma (WHO grade III and grade IV). https://jrct.niph.go.jp/latest-detail/jRCT2051210053.
- JRCT. 2024c. A phase II study of boron neutron capture therapy (BNCT) for patients with unresectable angiosarcoma, by using CICS-1 and SPM-011. https://jrct.niph.go.jp/latest-detail/jRCT2031220410.
- JRCT. 2024d. Phase 2 clinical trial of boron neutron capture therapy for recurrent high-grade meningioma using SPM-011 and BNCT30 - BNCT for high-grade meningioma. https://jrct.niph.go.jp/latest-detail/jRCT2051190044.
- Kageji T, Sogabe S, Mizobichi Y, Nakajima K, Shinji N, Nakagawa Y. 2015. Radiation-induced meningiomas after BNCT in patients with malignant glioma. Appl Radiat Isot. 106:256–259. doi:10.1016/j.apradiso.2015.06.004
- Kankaanranta L, Saarilahti K, Mäkitie A, Välimäki P, Tenhunen M, Joensuu H. 2011. Boron neutron capture therapy (BNCT) followed by intensity modulated chemoradiotherapy as primary treatment of large head and neck cancer with intracranial involvement. Radiother Oncol. 99(1):98–99. doi:10.1016/j.radonc.2011.02.008
- Kankaanranta L, Seppälä T, Koivunoro H, Saarilahti K, Atula T, Collan J, Salli E, Kortesniemi M, Uusi-Simola J, Välimäki P, et al. 2012. Boron neutron capture therapy in the treatment of locally recurred head-and-neck cancer: final analysis of a phase I/II trial. Int J Radiat Oncol Biol Phys. 82(1):e67-75–e75. doi:10.1016/j.ijrobp.2010.09.057
- Kanno H, Nagata H, Ishiguro A, Tsuzuranuki S, Nakano S, Nonaka T, Kiyohara K, Kimura T, Sugawara A, Okazaki Y, et al. 2021. Designation products: boron neutron capture therapy for head and neck carcinoma. Oncologist. 26(7):e1250–e1255. doi:10.1002/onco.13805
- Kao HF, Lou PJ. 2019. Immune checkpoint inhibitors for head and neck squamous cell carcinoma: current landscape and future directions. Head Neck. 41 Suppl 1(S1):4–18. doi:10.1002/hed.25930
- Kato I, Fujita Y, Maruhashi A, Kumada H, Ohmae M, Kirihata M, Imahori Y, Suzuki M, Sakrai Y, Sumi T, et al. 2009. Effectiveness of boron neutron capture therapy for recurrent head and neck malignancies. Appl Radiat Isot. 67(7-8 Suppl):S37–S42. doi:10.1016/j.apradiso.2009.03.103
- Khalil A, Adam MSS. 2024. Nucleoside scaffolds and carborane clusters for boron neutron capture therapy: developments and future perspective. Curr Med Chem. Epub ahead of print. doi:10.2174/0109298673245020230929152030
- Kimura Y, Ariyoshi Y, Miyatake S, Shimahara M, Kawabata S, Ono K. 2009. Boron neutron capture therapy for papillary cystadenocarcinoma in the upper lip: a case report. Int J Oral Maxillofac Surg. 38(3):293–295. doi:10.1016/j.ijom.2008.12.010
- Kondo N, Hirano F, Temma T. 2022. Evaluation of 3-Borono-l-phenylalanine as a water-soluble boron neutron capture therapy agent. Pharmaceutics. 14(5):1106. doi:10.3390/pharmaceutics14051106
- Kurosaki H, Okazaki K, Takemori M, Tate E, Nakamura T. 2024. The effects of boron neutron capture therapy on the lungs in recurrent breast cancer treatment. Cureus. 16(4):e57417. doi:10.7759/cureus.57417
- Lamba M, Goswami A, Bandyopadhyay A. 2021. A periodic development of BPA and BSH based derivatives in boron neutron capture therapy (BNCT). Chem Commun (Camb). 57(7):827–839. doi:10.1039/d0cc06557a
- Lee W, Kim KW, Lim JE, Sarkar S, Kim JY, Chang Y, Yoo J. 2022. In vivo evaluation of the effects of combined boron and gadolinium neutron capture therapy in mouse models. Sci Rep. 12(1):13360. doi:10.1038/s41598-022-17610-4
- Leśnikowski ZJ. 2016. New opportunities in boron chemistry for medical applications. In: Hosmane NS, editor. Boron science: new technologies and applications. Boca Raton (FL): CRC Press; p. 3–19.
- Li J, Shi Y, Zhang Z, Liu H, Lang L, Liu T, Chen X, Liu Z. 2019. A metabolically stable boron-derived tyrosine serves as a theranostic agent for positron emission tomography guided boron neutron capture therapy. Bioconjug Chem. 30(11):2870–2878. doi:10.1021/acs.bioconjchem.9b00578
- Li J, Sun Q, Lu C, Xiao H, Guo Z, Duan D, Zhang Z, Liu T, Liu Z. 2022. Boron encapsulated in a liposome can be used for combinational neutron capture therapy. Nat Commun. 13(1):2143. doi:10.1038/s41467-022-29780-w
- Locher GL. 1936. Biological effects and therapeutic possibilities of neutrons. Am J Roentgenol Radium Ther. 36(1):1–18.
- Luderer MJ, de la Puente P, Azab AK. 2015. Advancements in tumor targeting strategies for boron neutron capture therapy. Pharm Res. 32(9):2824–2836. doi:10.1007/s11095-015-1718-y
- Menéndez PR, Roth BMC, Pereira MD, Casal MR, González SJ, Feld DB, Santa Cruz GA, Kessler J, Longhino J, Blaumann H, et al. 2009. BNCT for skin melanoma in extremities: updated Argentine clinical results. Appl Radiat Isot. 67(7-8 Suppl):S50–S3. doi:10.1016/j.apradiso.2009.03.020
- Mishima Y, Ichihashi M, Tsuji M, Hatta S, Ueda M, Honda C, Suzuki T. 1989. Treatment of malignant melanoma by selective thermal neutron capture therapy using melanoma-seeking compound. J Invest Dermatol. 92(5 Suppl):321S–325S. doi:10.1111/1523-1747.ep13076750
- Mishima Y, Imahori Y, Honda C, Hiratsuka J, Ueda S, Ido T. 1997. In vivo diagnosis of human malignant melanoma with positron emission tomography using specific melanoma-seeking 18F-DOPA analogue. J Neurooncol. 33(1-2):163–169. doi:10.1023/a:1005746020350
- Miyatake S, Tamura Y, Kawabata S, Iida K, Kuroiwa T, Ono K. 2007. Boron neutron capture therapy for malignant tumors related to meningiomas. Neurosurgery. 61(1):82–91. doi:10.1227/01.neu.0000279727.90650.24
- Miyatake S-I, Kawabata S, Goto H, Narita Y, Suzuki M, Hirose K, Takai Y, Ono K, Ohnishi T, Tanaka H, et al. 2020. Accelerator-based BNCT in rescue treatment of patients with recurrent GBM: A multicenter phase II study. JCO. 38(15_suppl):2536–2536. doi:10.1200/JCO.2020.38.15_suppl.2536
- Moss RL. 1993. Review of Reactor-Based Neutron Beam Development for BNCT Applications. In: Soloway AH, Barth RF, Carpenter DE, editors. Advances in neutron capture therapy. Boston (MA): Springer; p. 1–7. doi:10.1007/978-1-4615-2978-1_1
- Nakagawa Y, Hatanaka H. 1997. Boron neutron capture therapy. Clinical brain tumor studies. J Neurooncol. 33(1-2):105–115. doi:10.1023/a:1005781517624
- Nakahara Y, Ito H, Masuoka J, Abe T. 2020. Boron neutron capture therapy and photodynamic therapy for high-grade meningiomas. Cancers (Basel). 12(5):1334. doi:10.3390/cancers12051334
- Nakamura H, Kirihata M. 2012. Boron compounds: new candidates for boron carriers in BNCT. In: Sauerwein W, Wittig A, Moss R, Nakagawa Y, editors. Neutron capture therapy. Berlin: Springer; p. 99–116. doi:10.1007/978-3-642-31334-9_7
- Nakase I, Aoki A, Sakai Y, Hirase S, Ishimura M, Takatani-Nakase T, Hattori Y, Kirihata M. 2020. Antibody-based receptor targeting using an fc-binding peptide-dodecaborate conjugate and macropinocytosis induction for boron neutron capture therapy. ACS Omega. 5(36):22731–22738. doi:10.1021/acsomega.0c01377
- Nariai T, Ishiwata K. 2012. Analysis and Imaging: PET. In: Sauerwein W, Wittig A, Moss R, Nakagawa Y, editors. Neutron capture therapy. Berlin: Springer; p. 229–240. doi:10.1007/978-3-642-31334-9_11
- Ono K, Tanaka H, Tamari Y, Watanabe T, Suzuki M, Masunaga S-i 2019. Proposal for determining absolute biological effectiveness of boron neutron capture therapy—the effect of 10B (n, α) 7Li dose can be predicted from the nucleocytoplasmic ratio or the cell size. J Radiat Res. 60(1):29–36. https://www.ncbi.nlm.nih.gov/pmc/articles/PMC6373679/pdf/rry080.pdf. doi:10.1093/jrr/rry080
- Porra L, Wendland L, Seppälä T, Koivunoro H, Revitzer H, Tervonen J, Kankaanranta L, Anttonen A, Tenhunen M, Joensuu H, et al. 2023. From nuclear reactor-based to proton accelerator-based therapy: the finnish boron neutron capture therapy experience. Cancer Biother Radiopharm. 38(3):184–191. doi:10.1089/cbr.2022.0059
- Sacco AG, Cohen EE. 2015. Current treatment options for recurrent or metastatic head and neck squamous cell carcinoma. J Clin Oncol. 33(29):3305–3313. doi:10.1200/JCO.2015.62.0963
- Sauerwein WAG, Bet PM, Wittig A. 2012. Drugs for BNCT: BSH and BPA. In: Sauerwein WAG, Wittig A, Moss R, Nakagawa Y, editors. Neutron capture therapy: principles and applications. Berlin: Springer; p. 117–160. doi:10.1007/978-3-642-31334-9_8
- Shanmugam M, Kuthala N, Kong X, Chiang CS, Hwang KC. 2023. Combined gadolinium and boron neutron capture therapies for eradication of head-and-neck tumor using Gd(10)B(6) nanoparticles under MRI/CT image guidance. JACS Au. 3(8):2192–2205. doi:10.1021/jacsau.3c00250
- Sibrian-Vazquez M, Vicente MGH. 2011. Boron tumor-delivery for BNCT: recent developments and perspectives. In: Hosmane NS, editor. Boron science: new technologies and applications. Boca Raton (FL): CRC Press; p. 203–232.
- Siegel RL, Miller KD, Jemal A. 2020. Cancer statistics, 2020. CA Cancer J Clin. 70(1):7–30. doi:10.3322/caac.21590
- Slatkin DN. 1991. A history of boron neutron capture therapy of brain tumours. Postulation of a brain radiation dose tolerance limit. Brain. 114 (Pt 4):1609–1629. doi:10.1093/brain/114.4.1609
- Stenstam BH, Pellettieri L, Sorteberg W, Rezaei A, Sköld K. 2007. BNCT for recurrent intracranial meningeal tumours - case reports. Acta Neurol Scand. 115(4):243–247. doi:10.1111/j.1600-0404.2006.00776.x
- Stupp R, Mason WP, van den Bent MJ, Weller M, Fisher B, Taphoorn MJB, Belanger K, Brandes AA, Marosi C, Bogdahn U, et al. 2005. Radiotherapy plus concomitant and adjuvant temozolomide for glioblastoma. N Engl J Med. 352(10):987–996. doi:10.1056/NEJMoa043330
- Suzuki M, Endo K, Satoh H, Sakurai Y, Kumada H, Kimura H, Masunaga S, Kinashi Y, Nagata K, Maruhashi A, et al. 2008. A novel concept of treatment of diffuse or multiple pleural tumors by boron neutron capture therapy (BNCT). Radiother Oncol. 88(2):192–195. doi:10.1016/j.radonc.2008.06.009
- Suzuki M, Kato I, Aihara T, Hiratsuka J, Yoshimura K, Niimi M, Kimura Y, Ariyoshi Y, Haginomori S-I, Sakurai Y, et al. 2014. Boron neutron capture therapy outcomes for advanced or recurrent head and neck cancer. J Radiat Res. 55(1):146–153. doi:10.1093/jrr/rrt098
- Suzuki M, Sakurai Y, Hagiwara S, Masunaga S, Kinashi Y, Nagata K, Maruhashi A, Kudo M, Ono K. 2007. First attempt of boron neutron capture therapy (BNCT) for hepatocellular carcinoma. Jpn J Clin Oncol. 37(5):376–381. doi:10.1093/jjco/hym039
- Sweet WH. 1997. Early history of development of boron neutron capture therapy of tumors. J Neurooncol. 33(1-2):19–26. doi:10.1023/a:1005752827194
- Tabbakh F, Hosmane NS. 2020. Enhancement of radiation effectiveness in proton therapy: comparison between fusion and fission methods and further approaches. Sci Rep. 10(1):5466. doi:10.1038/s41598-020-62268-5
- Takagaki M, Kazuko U, Hosmane NS. 2018. An overview of clinical and biological aspects of current boron neutron capture therapy (BNCT) for cancer treatment. In: Hosmane NS, Eagling R, editors. Handbook of boron science: with applications in organometallics, catalysis, materials and medicine, Volume 4: Boron in medicine. Singapore: World Scientific; p. 101–143. doi:10.1142/9781786344670_0005
- Takai S, Wanibuchi M, Kawabata S, Takeuchi K, Sakurai Y, Suzuki M, Ono K, Miyatake S-I. 2022. Reactor-based boron neutron capture therapy for 44 cases of recurrent and refractory high-grade meningiomas with long-term follow-up. Neuro Oncol. 24(1):90–98. doi:10.1093/neuonc/noab108
- Takeuchi K, Kawabata S, Hiramatsu R, Matsushita Y, Tanaka H, Sakurai Y, Suzuki M, Ono K, Miyatake S-I, Kuroiwa T, et al. 2018. Boron neutron capture therapy for high-grade skull-base meningioma. J Neurol Surg B Skull Base. 79(Suppl 4):S322–S327. doi:10.1055/s-0038-1666837
- Tamura Y, Miyatake S-I, Nonoguchi N, Miyata S, Yokoyama K, Doi A, Kuroiwa T, Asada M, Tanabe H, Ono K, et al. 2006. Boron neutron capture therapy for recurrent malignant meningioma. Case report. J Neurosurg. 105(6):898–903. doi:10.3171/jns.2006.105.6.898
- Tan AC, Ashley DM, López GY, Malinzak M, Friedman HS, Khasraw M. 2020. Management of glioblastoma: State of the art and future directions. CA Cancer J Clin. 70(4):299–312. doi:10.3322/caac.21613
- Ueda H, Suzuki M, Kuroda R, Tanaka T, Aoki S. 2021. Design, synthesis, and biological evaluation of boron-containing macrocyclic polyamines and Their Zinc(II) complexes for boron neutron capture therapy. J Med Chem. 64(12):8523–8544. doi:10.1021/acs.jmedchem.1c00445
- UMIN. 2021. Boron neutron capture therapy using cyclotron-based epithermal neutron source for squamous cell carcinoma of the head and neck refractory to standard treatments: An open-label phase II trial - REBIVAL study. [accessed 2024 Apr 25]. https://center6.umin.ac.jp/cgi-open-bin/ctr_e/ctr_view.cgi?recptno=R000050375.
- Vigneswaran N, Williams MD. 2014. Epidemiologic trends in head and neck cancer and aids in diagnosis. Oral Maxillofac Surg Clin North Am. 26(2):123–141. doi:10.1016/j.coms.2014.01.001
- Wang LW, Liu YH, Chou FI, Jiang SH. 2018. Clinical trials for treating recurrent head and neck cancer with boron neutron capture therapy using the Tsing-Hua Open Pool Reactor. Cancer Commun (Lond). 38(1):37. doi:10.1186/s40880-018-0295-y
- Wang S, Zhang Z, Miao L, Li Y. 2022. Boron neutron capture therapy: current status and challenges. Front Oncol. 12:788770. doi:10.3389/fonc.2022.788770
- Wu G, Barth RF, Yang W, Lee RJ, Tjarks W, Backer MV, Backer JM. 2006. Boron containing macromolecules and nanovehicles as delivery agents for neutron capture therapy. Anticancer Agents Med Chem. 6(2):167–184. doi:10.2174/187152006776119153
- Xu H, Liu J, Li R, Lin J, Gui L, Wang Y, Jin Z, Xia W, Liu Y, Cheng S, et al. 2024. Novel promising boron agents for boron neutron capture therapy: Current status and outlook on the future. Coord Chem Rev. 511:215795. doi:10.1016/j.ccr.2024.215795
- Xuan S, Vicente MGH. 2018. Recent advances in boron delivery agents for Boron Neutron Capture Therapy (BNCT). In: Hey-Hawkins E, Teixidor F, editors. Boron-based compounds: potential and emerging applications in medicine. Chichester (UK): John Wiley & Sons; p. 298–326.
- Yamana K, Kawasaki R, Kondo K, Hirano H, Kawamura S, Sanada Y, Bando K, Tabata A, Azuma H, Takata T, et al. 2023. HER-2-targeted boron neutron capture therapy using an antibody-conjugated boron nitride nanotube/beta-1,3-glucan complex. Nanoscale Adv. 5(15):3857–3861. doi:10.1039/d3na00028a
- Yanagie H, Higashi S, Seguchi K, Ikushima I, Fujihara M, Nonaka Y, Oyama K, Maruyama S, Hatae R, Suzuki M, et al. 2014. Pilot clinical study of boron neutron capture therapy for recurrent hepatic cancer involving the intra-arterial injection of a (10)BSH-containing WOW emulsion. Appl Radiat Isot. 88:32–37. doi:10.1016/j.apradiso.2014.01.014
- Yong Z, Song Z, Zhou Y, Liu T, Zhang Z, Zhao Y, Chen Y, Jin C, Chen X, Lu J, et al. 2016. Boron neutron capture therapy for malignant melanoma: first clinical case report in China. Chin J Cancer Res. 28(6):634–640. doi:10.21147/j.issn.1000-9604.2016.06.10
- Zhang X, Lin Y, Hosmane NS, Zhu Y. 2023. Nanostructured boron agents for boron neutron capture therapy: a review of recent patents. Med Rev (2021). 3(5):425–443. doi:10.1515/mr-2023-0013
- Zonta A, Pinelli T, Prati U, Roveda L, Ferrari C, Clerici AM, Zonta C, Mazzini G, Dionigi P, Altieri S, et al. 2009. Extra-corporeal liver BNCT for the treatment of diffuse metastases: what was learned and what is still to be learned. Appl Radiat Isot. 67(7-8 Suppl):S67–S75. doi:10.1016/j.apradiso.2009.03.087