ABSTRACT
Dactylopius opuntiae ‘stricta’ lineage was released more than 20 years ago in South Africa where it has successfully controlled Opuntia stricta. However, its host preference had not been tested against all invasive cacti in South Africa. Therefore, the possibility exists that it might successfully control other invasive cacti. We compared the performance of the ‘stricta’ lineage on Opuntia stricta, Opuntia humifusa and two lineages of Opuntia engelmannii. Additionally, we assessed if morphology of these Opuntia taxa could be used as an indicator of host selection by the ‘stricta’ lineage of D. opuntiae. Acceptability and suitability of the host taxa were measured by D. opuntiae life history parameters such as crawler development time and female weight. Principal component analysis of six morphometric characters of the Opuntia taxa was used to assess similarities between the taxa. Opuntia stricta is morphologically more similar to the O. engelmannii lineages than to O. humifusa, which showed little similarity to any of the other taxa. Additionally, the two O. engelmannii lineages showed some morphological similarity to each other. The ‘stricta’ lineage completed its development on O. humifusa such that the cochineal could be considered as a potential biological control agent for O. humifusa. Conversely, D. opuntiae ‘stricta’ was not able to complete development on O. engelmannii. Consequently, it would not be a suitable biological control agent for either lineage of O. engelmannii. The results show that O. humifusa is a suitable host for D. opuntiae ‘stricta’ and that Opuntia morphology is not an obvious indicator of host suitability.
Introduction
The native range of the genus Opuntia extends from southern Canada to southern South America. Along with Cylindropuntia, the genus Opuntia has the most widely introduced, cultivated and invasive species in the Cactaceae family (Novoa et al., Citation2014). Many Opuntia species have been deliberately introduced to other parts of the world, including South Africa. In addition, the taxonomy of the Opuntia is not totally clear and has undergone repeated revisions, resulting in renowned taxonomic difficulty caused by interspecific hybridisation, polyploidy and variability in the plant morphology (Majure et al., Citation2012). For example, Opuntia engelmannii Salm-Dyck (small round-leaved prickly pear) is diverse in its native range, in the Americas, where most of the accepted intraspecific taxa such as O. engelmannii var. engelmannii, O. engelmannii var. linguiformis and O. engelmannii var. lindheimeri (=subsp. lindheimeri) are found.
Some of these varieties have also been introduced to different parts of the world, including South Africa, Kenya, Spain and Australia. The morphological variability of O. engelmannii in South Africa has led the authors to call them lineages in this paper, based on where they are currently found in Africa. There are three recorded South African lineages of O. engelmannii that differ morphologically and are found in the provinces of Eastern Cape, Northern Cape and Limpopo (Klein, Citation2015). A fourth distinct lineage of O. engelmannii is found in Kenya (Githae, Citation2019). Dense infestations of O. engelmannii lineages and O. humifusa (Raf) Raf (creeping prickly pear) decrease the grazing potential of land and reduce access to livestock (O’Connor & van Wilgen, Citation2020). The long spines on the cladodes can injure livestock and the tiny glochids irritate their mouths in such a way that they are eventually unable to feed (O’Connor & van Wilgen, Citation2020). Dense infestations of Opuntia species displace native flora which results in serious ecological impacts (Novoa et al., Citation2016) and socioeconomic impacts (Reynolds et al., Citation2020; Shackleton et al., Citation2017). Therefore, there is need to control these plants. Fortunately, when successful, biological control of Opuntia species is a cost-effective method that has been used for many years and has been effective against numerous species in the cactus family (Paterson et al., Citation2019).
Despite these successes, the selection of biological control agents for Opuntia species can be difficult, even though the lack of native Cactaceae in the Old World reduces concerns around their host specificity. The narrow host alignment of many strains of the agents, which are known as lineages, coupled with the confusing array of target plant lineages, make it difficult to predict whether or not the agent will actually damage the target. Cochineal insects have been widely used in the biological control of the Opuntia species. They belong to the Dactylopiidae family which has a single genus, Dactylopius containing nine species, all of which are natives of North or South America. Dactylopius species such as D. austrinus De Lotto (Hosking, Citation1984) and D. opuntiae Cockerell, show similar life history characteristics and general appearance (Mathenge et al., Citation2009a).
Noteworthy successes in biological control include the biological control of O. monacantha (Wild.) Haw (Paterson et al., Citation2011), O. aurantiaca Lindl (Moran & Zimmermann, Citation1991), O. stricta (Haw) Haw (Hoffmann et al., Citation1998) and O. ficus-indica (L.) Mill (Zimmermann and Moran, Citation1991). However, many of the agents and targets appear to have been brought together by good fortune as ‘new associations’, which are combinations that do not exist in their native range (Goeden & Kok, Citation1986), making them particularly damaging when the plant is susceptible to the agent (Hokkanen & Pimentel, Citation1984). Dactylopius opuntiae has at least two host-adapted populations of D. opuntiae which are called lineages. The D. opuntiae lineage, called the ‘ficus’ lineage, found within South Africa since 1937 originated from Mexico and provides satisfactory control of O. ficus-indica, while the ‘stricta’ lineage that has been successful in controlling O. stricta, originated from the United States of America and was brought to South Africa from Australia in 1996 (Githure et al., Citation1999; Klein, Citation2011; Mathenge et al., Citation2009b; Volchansky et al., Citation1999). Unlike the plant lineages, these lineages cannot be morphologically distinguished, but are instead characterised by their feeding and development preferences on their potential host plants (Mathenge et al., Citation2009b).
Rule and Hoffmann (Citation2018) reported that O. humifusa is also a suitable host of the ‘stricta’ lineage of D. opuntiae. This would not be predicted from what is known of the phylogeny of the taxa which places O. humifusa in the Humifusa clade, distinctly separate from O. stricta, in the Scheerianae clade (Majure et al., Citation2012). The ability of a biological control agent to feed and or lay eggs on a host plant is known as the acceptability of the host to the agent (Barton-Browne & Withers, Citation2002). It is also necessary to establish if the host can support complete development of the biological control agent from neonate to a reproducing adult, which is known as host suitability (Barton-Browne & Withers, Citation2002).
Such differences in the responses of the ‘stricta’ lineage to relatively distantly-related taxa may be due to morphological differences, or other traits, which include plant secondary metabolites, within the Opuntia taxa. Identification and characterisation of a given species’ morphology has largely been based on differences in a plant’s physical features for example the colour, size and the shape of leaves and flowers (Gunnell & Gubler, Citation1992; Wäldchen et al., Citation2018). In biological control, differences in leaf morphology and complexity have been reported to cause a significant effect on natural enemy efficacy (van Lenteren & de Ponti, Citation1990).
Plant morphological complexity not only provides a physical shelter to a natural enemy but could also inhibit the foraging activities of such an enemy (Schat & Blossey, Citation2005). Comparisons of species’ morphological features can provide a basis for analysis of sister relationships (Khoury et al., Citation2010). Cluster analysis has been used to identify and differentiate plant species from each other and group accessions of plants based on their morphological similarities (Dikshit & Sivaraj, Citation2015). Such analysis could provide insights into host specificity of insect herbivores, if features of the host morphology are related to the acceptability and suitability of that host.
Alternatively, secondary metabolites similarity among potential host plants may explain host suitability of a specialised insect herbivore, sometimes more so than the phylogenetic relatedness of these particular plant species (Kergoat et al., Citation2005; Murphy & Feeny, Citation2006; Rapo et al., Citation2019). Weber et al. (Citation2019) have shown a population of wild strawberries to differ in their acceptability (‘antixenosis’ = beetle preference = acceptability) and suitability (‘antibiosis’ = beetle survival and performance = suitability) of the strawberry leaf beetle (Garelucella tennela L.). The beetles avoid laying eggs on certain host genotypes and this preference is linked to the insect’s fitness. Closer to home, the metabolomic profiles of invasive populations of alien weeds in the Kruger National Park, South Africa, were shown to differ significantly from their native populations in the country of origin (Weber et al., Citation2019). These findings reveal that the organisms on both sides of the biological control relationship are constantly evolving, even in the notoriously stable associations that have been incorporated into biological control programmes (Müller-Schärer et al., Citation2020).
The lineages of D. opuntiae and their various potential host taxa within the Opuntia in this evolutionary relationship, are only just beginning to be resolved by systematic examination of these associations (van Steenderen, Citation2019). Therefore, the aims of this study were to: (i) compare the acceptability of O. stricta, O. humifusa and two O. engelmannii lineages to the ‘stricta’ lineage of D. opuntiae; (ii) test the suitability of these taxa as hosts by comparing the survival and developmental period of the ‘stricta’ lineage on these taxa; and (iii) assess if the morphology of these taxa corresponds to these measures of host selection by the D. opuntiae ‘stricta’ lineage.
Materials and methods
Plant culture
Ten potted plants of the same age, each of O. stricta, O. humifusa, the O. engelmannii – Limpopo lineage and the O. engelmannii – Kenyan lineage, were obtained from the Agricultural Research Council-Plant Protection Research Institute (ARC-PPRI), Pretoria. Four cladodes were harvested from each plant and planted out into pots at the Wits University insectary, yielding a total of 40 plants per species.
Insect culture
A population of the ‘stricta’ lineage of D. opuntiae was obtained from the ARC-PPRI, Pretoria and maintained on cladodes of O. stricta, the preferred host of this biotype (Rule & Hoffmann, Citation2018). Conditions conducive to the development, survival and reproduction of Dactylopius species (Sullivan, Citation1990) were maintained: room temperature 27 ± 2°C, 70 ± 10% relative humidity and a 14-h daylight cycle. The D. opuntiae culture was kept in a sealed plastic container (27 cm × 18 cm × 11 cm) with a perforated lid for ventilation. The container was lined with polystyrene at the bottom. A fresh O. stricta cladode was added every 2 weeks. The old cladodes were removed after 4 weeks.
No choice comparison of ‘stricta’ lineage acceptance and suit ability on various Opuntia taxa
Ten mature cladodes of comparable size of each of the three Opuntia taxa were harvested from the potted plants of O. stricta, O. humifusa, O. engelmannii-Kenyan and O. engelmannii – Limpopo lineages grown at Wits. Clones from the same plant were not used in the same trial. This increased variability between plant replicates, since plants of the same clone with similar genetic make-up are expected to have the same degree of cochineal resistance when grown under the same conditions. Isolated cladodes which are detached from the plant remain alive for many months and even produce new growth (Hosking, Citation1984; Sullivan, Citation1990). All cladodes used in the experiments were washed thoroughly to remove unwanted organisms before the D. opuntiae ‘stricta’ lineage crawlers were placed onto them. Mature females had their wax covering removed by rolling it onto a pin to allow harvesting of crawlers (Mathenge et al., Citation2009b).
Females were then placed individually in 90-mm-diameter plastic Petri dishes. The following day, the crawlers produced by these females, were mixed together in a sealed plastic container and then used in the trials. The tests were ‘no-choice’ as crawlers were placed directly on to the cladodes of the Opuntia taxa under test in their individual containers. Crawler placement was randomised across all taxa. Cladodes were placed in plastic containers with perforated lids that allowed ventilation, excluded other insects and prevented crawlers from escaping. The containers were lined with polystyrene blocks at the bottom. Cladodes were supported by four pins embedded into a polystyrene block to ensure that the crawlers were able to settle anywhere on the horizontal cladode.
A fine paint brush was used to individually transfer 30 crawlers on-to experimental cladodes. The sex-ratio in D. opuntiae is approximately 50% females (Hoffmann et al., Citation2002), therefore, the random selection of 30 crawlers was expected to represent the prevailing sex ratio. The cladodes were monitored every three days for 86 days, using a stereo microscope to record the survival and development of each stage. Crawlers of D. opuntiae are covered with stiff bristles. Female crawlers have elongated bristles whilst the male crawlers have short bristles. The adult females resemble small puffy sacs about the size of a match-head when alive and the adult males are pink with two semitransparent wings. To record the survival of the crawlers, the following was checked: the quantity of bristles covering the crawlers, puffiness of the adult females and the colour of the adult males. Moulting was confirmed by the presence of a white exuviate near the insect (Karny, Citation1972). The number of crawlers that settled and began to feed was recorded as a measure of host acceptance; the date of first and second moult was also noted along with the numbers that completed first and second moult. The number of females and males that reached maturity was recorded as a measure of host suitability.
The time (in days) taken by the nymphs to undergo their first and second moult was recorded to determine the pre-oviposition time for the resulting females. The reproductive output of the insects that reached maturity was measured by counting the crawlers produced by the adult females. As soon as new crawlers were seen around any individual female, that female was gently dislodged from the cladode. The wax coating of the female was carefully removed as above and the mass of the female was measured using a KERN ABT-NM balance (to four decimal places). Mass measurements represent the female’s condition at maturity. Female fecundity was measured by placing the females with a piece of their host cladode into separate vials and counting the number of progeny that they produced in the vials until their death.
In this study, the ‘stricta’ lineage of D. opuntiae was considered to have high host acceptance when more than 50% of the crawlers settled on the host cladode. The Opuntia taxa were considered as being a suitable host when more than 50% of the nymphs developed to adulthood and produced progeny (Jones et al., Citation2015). Normality of the reproductive performance data was assessed using the Shapiro–Wilk W test. One-way analysis of variance (ANOVA) was used for the reproduction and survival trials to compare the development of the ‘stricta’ lineage between host plants with regard to the duration of stages and mortality rates.
Plant morphological variability
Spine and cladode measurements are important informative characters for Opuntia species taxonomy (Mosco, Citation2009). Therefore, methods similar to previous Opuntia morphology work by Mosco (Citation2009) and Peharec et al. (Citation2010) were used. Spine and cladode measurements were taken for O. humifusa, O. stricta, O. engelmannii-Kenya and O. engelmannii-Limpopo lineages ().
Table 1. Spine and cladode traits measured per species of Opuntia. Twenty-five individuals were examined and averaged per taxon for each trait.
Results
Acceptability of Opuntia taxa to the ‘stricta’ lineage
A significantly larger number of crawlers settled on O. stricta and O. humifusa () compared to those that settled on either of the O. engelmannii lineages (F3,18 = 76.11; P < 0.05).
Suitability of Opuntia taxa to the ‘stricta’ lineage
There was a significant difference in the number of days it took the ‘stricta’ lineage to reach the first moult on each host (F3,18 = 36.11; P < 0.05 – ). There was no significant difference in the mean number of progeny produced by the females that developed on O. stricta and O. humifusa (F3,18 = 80.1; P = 0.3129 – ). The number of days taken to start production of offspring was significantly different between the D. opuntiae ‘stricta’ lineage females that developed on both O. stricta and O. humifusa (F3,18 = 32.09; P = 0.001 – ).
Figure 2. Number of days to first moult of the ‘stricta’ lineage of D. opuntiae on different Opuntia species. Bars (mean ± SE) annotated with the same letter do not differ significantly (P > 0.05). L – Limpopo lineage, K – Kenyan lineage.
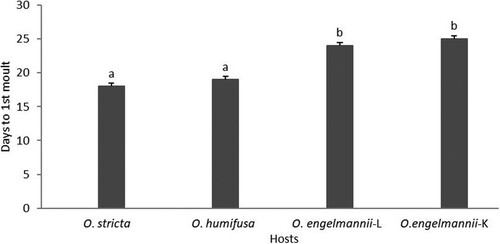
Figure 3. The number of days to first production of crawlers by the ‘stricta’ lineage of D. opuntiae on different Opuntia species. Bars (mean ± SE) with the same letters do not differ significantly (P > 0.05).
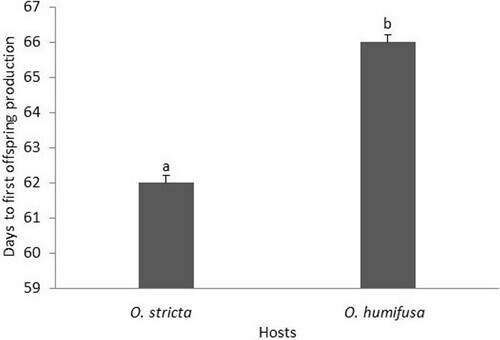
Table 2. Measures of suitability of different Opuntia taxa as hosts for the ‘stricta’ lineage of D. opuntiae. Mean (±S.E.) time to develop of the ‘stricta’ lineage of D. opuntiae (from settling to 2nd moult); female mass (n = 7) at first offspring production and number of progeny per female on different Opuntia species. Means with same letters in the same column do not differ significantly (P > 0.05). MNM: mass not measured as they did not reach maturity; NPP: no progeny produced; L: Limpopo lineage; K: Kenyan lineage.
Morphological comparisons of Opuntia taxa
A principal component analysis of spine and cladode morphological traits showed clear groupings between the taxa that match their phylogenetic relationships. The O. engelmannii – Kenya and Limpopo lineages overlapped in spine morphology, while the O. engelmannii – Kenya lineage shared cladode thickness and areole number characateristics with O. stricta. Opuntia humifisa appeared as a separate grouping separated by all six morphometric characters tested, indicating that it differs substantially in morphology to both O. stricta and the two O. engelmannii lineages ().
Figure 4. A principal component analysis (PCA) using six morphometric characters (i.e. spine length, cladode thickness) for the three Opuntia species (n = 25/species). Opuntia humifusa has a different morphology to O. stricta and the O. engelmannii lineages. The Kenyan and Limpopo lineages of O. engelmannii have a similar spine morphology while O. stricta and the Kenyan lineage of O. engelmannii share similar numbers of areoles and cladode surface area.
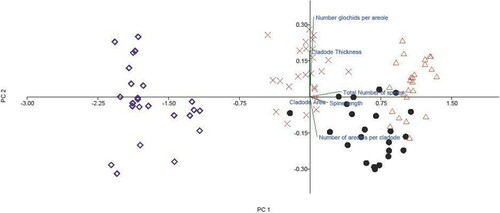
Discussion
The ‘stricta’ lineage of D. opuntiae exhibited varying degrees of acceptance of different Opuntia species, with the least acceptable being the O. engelmannii lineages from both Limpopo and Kenya. Opuntia stricta and O. humifusa are both acceptable and suitable hosts (in supporting the development of D. opuntiae from neonate to a reproductive adult). The D. opuntiae ‘stricta’ lineage showed small differences in its ability to develop and survive on these two hosts. The prolonged development time of the D. opuntiae ‘stricta’ lineage on O. engelmannii lineages and the failure of the females to produce progeny shows that they are not suitable hosts of this cochineal lineage.
Other studies have previously reported that the ‘stricta’ lineage is specific to O. stricta, but with the potential to inflict damage on other Opuntia species (Hoffmann et al., Citation2002; Volchansky et al., Citation1999). Given that O. humifusa was shown here to be morphologically different to O. stricta, it is surprising that the D. opuntiae ‘stricta’ lineage was able to utilise this taxon as a host. This affinity has been reported elsewhere (Rule & Hoffmann, Citation2018). It is more surprising still that neither lineage of O. engelmannii could support development of the cochineal given their morphological similarity to both O. stricta and each other. This shared morphological similarity is to be expected, as all three of the latter taxa are members of the Scheerianae clade, while O. humifusa is phylogenetically separate and in the Humifusa clade (Majure et al., Citation2012). An alternative hypothesis to the selective behaviour of this cochineal could be that secondary compounds produced by the host plants are what cochineal insects use to accept their host, and will dictate whether or not a host is suitable (Rapo et al., Citation2019). Secondary metabolites may offer a useful area of investigation to determine what makes a host suitable for this valuable cochineal.
A morphometric analysis of Opuntia species highlights the variability of Opuntia species. The clustering between O. engelmannii – Kenya and Limpopo is expected as these belong to the same species. Opuntia humifisa is morphologically distinct to both O. engelmannii lineages but shared a morphological quadrat to O. stricta. This may explain why there was a difference in development of crawlers between O. engelmannii and O. humifusa. For example, the first-instar nymphs have to pierce the cactus cladodes before they can start feeding, therefore the mechanical structures of the plants, such as thickness of the wax coating on the cladodes may influence the settling and development of the nymphs. Kareiva and Sahakian (Citation1990) showed the impact of the plant’s architecture and morphology on coccinellid species.
Opuntia plant morphology can be dependent on environmental conditions (Franco & Nobel, Citation1989). However, the use of multiple morphological diagnostic characters, as used in the present study, limits this problem because all the traits are unlikely to be affected similarly by the environmental conditions (Duminil & Di Michele, Citation2009). Valdez-Cepeda et al. (Citation2003) reported that spine and cladode diagnostic traits of Opuntia species are important for the morphological classification of cactus pear varieties, and that spines are a stable and reliable character for identification of the cactus varieties. Similarly, these diagnostic traits were able to differentiate between the species of Opuntia in this study. Nevertheless, they cannot be used at this point to define the limits of host specificity of D. opuntiae lineages. Investigations of other factors that might determine whether the ‘stricta’ lineage of D. opuntiae accepts or rejects other Opuntia species as its hosts must be conducted. More than 20 years after its introduction into South Africa, the ‘stricta’ lineage of D. opuntiae is still effective in controlling its target weed, O. stricta and can also be considered for control of O. humifusa.
Acknowledgements
This research was supported by funds from the National Research Foundation. Many people facilitated this research. We want to thank Hildegard Klein for her guidance with the experiments. We are also grateful to Phindile Montana, Phuluso Mudau, Maurice Mkasi, Lyriche Drude and Nic Venter for helping with the laboratory work.
Disclosure statement
No potential conflict of interest was reported by the author(s).
Additional information
Funding
References
- Barton-Browne, L., & Withers, T. M. (2002). Time-dependent changes in the host-acceptance threshold of insects: Implications for host specificity testing of candidate biological control agents. Biocontrol Science and Technology, 12(6), 677–693. https://doi.org/https://doi.org/10.1080/0958315021000039860
- Dikshit, N., & Sivaraj, N. (2015). Analysis of agro-morphological diversity and oil content in Indian linseed germplasm. Grasas y Aceites, 66(1), e060. https://doi.org/https://doi.org/10.3989/gya.0689141
- Duminil, J., & Di Michele, M. (2009). Plant species delimitation: A comparison of morphological and molecular markers. Plant Biosystems, 143(3), 528–542. https://doi.org/https://doi.org/10.1080/11263500902722964
- Franco, A., & Nobel, P. S. (1989). Effect of nurse plants on the microhabitat and growth of cacti. The Journal of Ecology, 77(3), 870–886. https://doi.org/https://doi.org/10.2307/2260991
- Githae, E. (2019). Status of Opuntia invasions in the arid and semi-arid lands of Kenya. CAB Reviews, 13(003), 1–3. https://doi.org/https://doi.org/10.1079/PAVSNNR201813003
- Githure, C. W., Zimmermann, H. G., & Hoffmann, J. H. (1999). Host specificity of biotypes of Dactylopius opuntiae (Cockerell) (Hemiptera: Dactylopiidae): prospects for biological control of Opuntia stricta (Haworth) Haworth (Cactaceae) in Africa. African Entomology, 7(1), 43–48. https://doi.org/https://hdl.handle.net/10520/AJA10213589_387
- Goeden, R., & Kok, L. (1986). Comments on a proposed “new” approach for selecting agents for the biological control of weeds. The Canadian Entomologist, 118(1), 51–58. https://doi.org/https://doi.org/10.4039/Ent11851-1
- Gunnell, P. S., & Gubler, W. D. (1992). Taxonomy and morphology of Colletotrichum species pathogenic to strawberry. Mycologia, 84(2), 157–165. https://doi.org/https://doi.org/10.1080/00275514.1992.12026122
- Hoffmann, J. H., Moran, V. C., & Zeller, D. A. (1998). Evaluation of Cactoblastis cactorum (Lepidoptera: Phycitidae) as a biological control agent of Opuntia stricta (Cactaceae) in the Kruger National Park, South Africa. Journal of Applied Ecology, 23(1), 133–139. https://doi.org/https://doi.org/10.1006/bcon.1998.0608
- Hoffmann, J. H., Impson, F. A. C., & Volchansky, C. R. (2002). Biological control of cactus weeds: Implications of hybridization between control agent biotypes. Journal of Applied Ecology, 39(6), 900–908. https://doi.org/https://doi.org/10.1046/j.1365-2664.2002.00766.x
- Hokkanen, H., & Pimentel, D. (1984). New approach for selecting agents biological control agents. The Canadian Entomologist, 116(8), 1109–1121. https://doi.org/https://doi.org/10.4039/Ent1161109-8
- Hosking, J. R. (1984). The effect of temperature on the population growth potential of Dactylopius austrinus De Lotto (Homoptera: Dactylopiidae), on Opuntia aurantiaca Lindley. Australian Journal of Entomology, 23(2), 133–139. https://doi.org/https://doi.org/10.1111/j.1440-6055.1984.tb01927.x
- Jones, P. K., Holtkamp, R. H., William, A. P., & Day, M. D. (2015). The host range of three biotypes of Dactylopius tomentosus (Lamarck) (Hemiptera: Dactylopiidae) and their potential as biological control agents of Cylindropuntia spp. Cactaceae) in Australia. Biocontrol Science and Technology, 25(6), 613–628. https://doi.org/https://doi.org/10.1080/09583157.2014.999747
- Kareiva, P., & Sahakian, R. (1990). Tritrophic effects of a simple architectural mutation in pea plants. Nature, 345(6274), 433–434. https://doi.org/https://doi.org/10.1038/345433a0
- Karny, M. (1972). Comparative studies on the three Dactylopius species (Homoptera: Dactylopiidae) attacking introduced opuntias in South Africa. Entomology Memoir, Department of Agricultural Technical Services, Republic of South Africa, 26, 1–19.
- Kergoat G. J., Delobel, A., Fediere, G., Le Rü, B., & Silvain, J. (2005). Both host-plant phylogeny and chemistry have shaped the African seed-beetle radiation. Molecular Phylogenetics and Evolution 35(3), 602–611. https://doi.org/https://doi.org/10.1016/j.ympev.2004.12.024
- Khoury, C., Laliberté, B., & Guarino, L. (2010). Trends in ex situ conservation of plant genetic resources: A review of global crop and regional conservation strategies. Genetic Resources and Crop Evolution, 57(4), 625–639. https://doi.org/https://doi.org/10.1007/s10722-010-9534-z
- Klein, H. (2011). A catalogue of the insects, mites and pathogens that have been used or rejected, or are under consideration, for the biological control of invasive alien plants in South Africa. African Entomology, 19(2), 515–549. https://doi.org/https://doi.org/10.4001/003.019.0214
- Klein, H. (2015). Research in progress to control small round-leaved prickly pear. Newsletter of the Plant Protection Research Institute. https://www.invasives.org.za/files/96/PPRI-News/752/PPRI-Newsletter—No-105-July-Sept-2015.pdf
- Majure, L. C., Puente, R., Griffith, M. P., Judd, W. S., Soltis, P. S., & Soltis, D. E. (2012). Phylogeny of Opuntia s.s. (Cactaceae): Clade delineation, geographic origins, and reticulate evolution. American Journal of Botany, 99(5), 847–864. https://doi.org/https://doi.org/10.3732/ajb.1100375
- Mathenge, C. W., Holford, P., Hoffmann, J. H., Spooner-Hart, R., Beattie, G. A. C., & Zimmermann, H. G. (2009a). The biology of Dactylopius tomentosus (Hemiptera: Dactylopiidae). Bulletin of Entomological Research, 99(6), 551–559. https://doi.org/https://doi.org/10.1017/S0007485308006597
- Mathenge, C. W., Holford, P., Hoffmann, J. H., Zimmermann, H. G., Spooner-Hart, R., & Beattie, G. A. C. (2009b). Determination of biotypes of Dactylopius tomentosus (Hemiptera:Dactylopiidae) and insights into taxonomic relationships of their hosts, Cylindropuntia spp. Bulletin of Entomological Research, 100(3), 347–358. https://doi.org/https://doi.org/10.1017/S0007485309990496
- Moran, V. C., & Zimmermann, H. G. (1991). Biological control of jointed cactus, Opuntia aurantiaca (cactaceae), in South Africa. Agriculture, Ecosystems & Environment, 37(1–3), 5–27. https://doi.org/https://doi.org/10.1016/0167-8809(91)90136-L
- Mosco, A. (2009). Micro-morphology and anatomy of Turbinicarpus (Cactaceae) spines. Revista Mexicana de Biodiversidad, 80((001|1)), 119–128. https://doi.org/https://doi.org/10.22201/ib.20078706e.2009.001.589
- Müller-Schärer, H., Bouchemousse, S., Litto, M., Mcevoy, P. B., Roderick, G. K., & Sun, Y. (2020). How to better predict long-term benefits and risks in weed biocontrol: An evolutionary perspective. Current Opinion in Insect Science, 38, 84–91. https://doi.org/https://doi.org/10.1016/j.cois.2020.02.006
- Murphy, S. M., & Feeny, P. (2006). Chemical facilitation of a naturally occurring host shift by Papilio machaon butterflies (Papilionidae). Ecological Monographs 76(3), 399–414. https://doi.org/https://doi.org/10.1890/0012-9615(2006)076[0399:CFOANO]2.0.CO;2
- Novoa, A., Le Roux, J. J., Robertson, M. P., Wilson, J. R. U & Richardson, D. M. (2014). Introduced and invasive cactus species: A global review. AoB PLANTS 7, plu078. https://doi.org/https://doi.org/10.1093/aobpla/plu078
- Novoa, A., Kaplan, H., Wilson, J. R. U., & Richardson, D. M. (2016). Resolving a prickly situation: Involving stakeholders in invasive cactus management in South Africa. Environmental Management, 57(5), 998–1008. https://doi.org/https://doi.org/10.1007/s00267-015-0645-3
- O’Connor, T. G., & van Wilgen, B. W. (2020). The impact of invasive alien plants on rangelands in South Africa. In B. W. van Wilgen, J. Measey, D. Richardson, J. Wilson, & T. Zengeya (Eds.), Biological Invasions in South Africa (pp. 459–487). Springer.
- Paterson, I. D., Hoffmann, J., Klein, H., Mathenge, C. W., & Neser, S. (2011). Biological control of Cactaceae in South Africa. African Entomology, 19(2), 230–246. https://doi.org/https://doi.org/10.4001/003.019.0221
- Paterson, I. D., Manheimmer, C. A., & Zimmermann, H. G. (2019). Prospects for biological control of cactus weeds in Namibia. Biocontrol Science and Technology, 29(4), 393–399. https://doi.org/https://doi.org/10.1080/09583157.2018.1562040
- Peharec, P., Posilović, H., Balen, B., & Krsnik-Rasol, M. (2010). Spine micromorphology of normal and hyperhydric Mammillaria gracilis Pfeiff. (Cactaceae) shoots. Journal of Microscopy, 239(1), 78–86. https://doi.org/https://doi.org/10.1111/j.1365-2818.2009.03358.x
- Rapo, C. B., Schaffner, U., Eigenbrode, S. D., Hinz, H. L., Price, W. J., Morra, M., Gaskin, J., & Schwarzländer, M. (2019). Feeding intensity of insect herbivores is associated more closely with key metabolite profiles than phylogenetic relatedness of their potential hosts. PeerJ, 7, e8203. https://doi.org/https://doi.org/10.7717/peerj.8203
- Reynolds, C., Venter, N., Cowie, B. W., Marlin, D., Mayonde, S., Tocco, C., & Byrne, M. J. (2020). Mapping the socio-ecological impacts of invasive plants in South Africa: Are poorer households with high ecosystem service use most at risk. Ecosystem Services, 42, 101075. https://doi.org/https://doi.org/10.1016/j.ecoser.2020.101075
- Rule, N. F., & Hoffmann, J. H. (2018). The performance of Dactylopius opuntiae as a biological control agent on two invasive Opuntia cactus species in South Africa. Biological Control, 119, 7–11. https://doi.org/https://doi.org/10.1016/j.biocontrol.2018.01.001
- Schat, M., & Blossey, B. (2005). Influence of natural and simulated leaf beetle herbivory on biomass allocation and plant architecture of purple loosestrife (Lythrum salicaria L.). Environmental Entomology, 34(4), 906–914. https://doi.org/https://doi.org/10.1603/0046-225X-34.4.906
- Shackleton, R. T., Witt, A. B. R., Piroris, F. M., & van Wilgen, B. W. (2017). Distribution and socio-ecological impacts of the invasive alien cactus Opuntia stricta in Eastern Africa. Biological Invasions, 19(8), 2427–2441. https://doi.org/https://doi.org/10.1007/s10530-017-1453-x
- Sullivan, P. R. (1990). Population growth potential of Dactylopius ceylonicus Green (Hemiptera: Dactylopiidae) on Opuntia Vulgaris Miller. Journal of Austral Entomology, 29(2), 123–129. https://doi.org/https://doi.org/10.1111/j.1440-6055.1990.tb00333.x
- Valdez-Cepeda, R. D., Blanco-Macías, F., & Gallegos-Vázquez, C. (2003). Ordering and numerical classification in prickly pear cactus using fruit attributes. Revista Chapingo Serie Horticultura, 9(1), 81–95. https://doi.org/https://doi.org/10.5154/r.rchsh.2001.04.024
- van Lenteren, J. C., & de Ponti, O. M. B. (1990). Plant-leaf morphology, host-plant resistance and biological control. Symposium Biologica Hungarica, 39, 365–386.
- van Steenderen, C. (2019). A genetic analysis of the species and intraspecific lineages of Dactylopius Costa (Hemiptera: Dactylopiidae) [Unpublished dissertation]. Rhodes University.
- Volchansky, C. R., Hoffmann, J. H., & Zimmermann, H. G. (1999). Host plant affinities of two biotypes of Dactylopius opuntiae: Enhanced biological control of Opuntia stricta in South Africa. Journal of Applied Ecology, 36(1), 85–91. https://doi.org/https://doi.org/10.1046/j.1365-2664.1999.00381.x
- Wäldchen, J., Rzanny, M., Seeland, M., & Mäder, P. (2018). Automated plant species identification—trends and future directions. PLoS Computational Biology, 14(4), e1005993. https://doi.org/https://doi.org/10.1371/journal.pcbi.1005993
- Weber, D., Egan, P. A., Muola, A., & Stenberg, J. A. (2019). Genetic variation in herbivore resistance within a strawberry crop wild relative (Fragaria vesca L.). Arthropod-Plant Interactions, 14(1), 31–40. https://doi.org/https://doi.org/10.1007/s11829-019-09724-w
- Zimmermann, H. G., & Moran, V. C. (1991). Biological control of prickly pear, Opuntia ficus-indica (Cactaceae), in South Africa. Agriculture, Ecosystems and Environment, 37(1-3), 29–35. doi:https://doi.org/10.1016/0167-8809(91)90137-M