ABSTRACT
The fungus, Fusarium lateritium Nees ex Fr. (FL), has shown potential as a bioherbicide for velvetleaf (Abutilon theophrasti Medik.) control. However, to achieve optimal infection and weed mortality, bioherbicide applications must be applied to weeds that are in the cotyledonary growth stage, followed by a dew treatment of at least 16 h. Greenhouse and field experiments were established to discover possible synergistic disease interactions with a phenoxy herbicide to improve the bioherbicidal potential of this fungus, and to mitigate some of the physical and environmental restrictions that limit the utility of this fungus as a bioherbicide. In greenhouse experiments, mortalities of 94% and 94% were recorded from sequential applications of 2,4-DB [4-(2,4-dichlorophenoxy)butyric acid] (0.02 kg ae ha−1) followed by FL (1.5 × 106 spores ml−1) at 5 min or 7 days after herbicide treatment. Velvetleaf plants in the fifth-to-seventh leaf growth stage were infected and killed with < 8 hrs. of dew. Similar results occurred under field conditions. Infection and weed control were inhibited by tank mixtures of 2,4-DB and FL, and by sequential applications of FL followed by 2,4-DB. These results suggest that timely applications of 2,4-DB followed by F. lateritium may provide effective control of velvetleaf.
Introduction
Velvetleaf (Abutilon theophrasti Medik.), an invasive weed native to China (Sattin et al., Citation1992), was introduced to North America in the seventeenth century primarily for fibre production (Spencer, Citation1984). Velvetleaf is self-pollinating, and a single plant can produce up to 17,000 seeds that may persist up to 50 yr in the soil and emerge throughout the crop growing season when conditions are favourable (Lueschen & Andersen, Citation1980; Warwick & Black, Citation1988). Up to 48% viability of velvetleaf seeds after 39 yr of seed burial has been reported (Toole & Brown, Citation1946). It is one of the most problematic weeds in corn (Zea mays L.) and soybean [Glycine max (L.) Merr.] in the eastern U.S. (Colton & Einhellig, Citation1980; Hagood et al., Citation1980).
The success of velvetleaf as a weed is due to inadequate control and ‘weedy’ characteristics such as seed dormancy, the ability to germinate from deep in the soil, and tolerance for many herbicides used in corn and soybeans. These factors have led to large velvetleaf seed reservoirs in many areas of the midwestern U.S. where corn and soybeans are grown (Spencer, Citation1984). Velvetleaf interference can significantly reduce the yield of most agronomic crops, including corn, soybean, and cotton. Several studies reported that corn yield loss from velvetleaf interference approached a maximum asymptote around the weed density of 10 plants m−2, with further density increases resulting in minor increases in crop damage (Lindquist et al., Citation1996).
2,4-DB [4-(2,4-dichlorophenoxy)butyric acid] is a selective systemic phenoxy herbicide used to control several annual and perennial broad-leaf weeds in crops such as alfalfa (Medicago sativa L.), peanuts (Arachis hypogaea L.), and velvetleaf in soybeans and other crops. 2,4 DB is presumably nonphytotoxic and is metabolised to 2,4-D (2,4-dichlorophenoxyacetic acid), that inhibits growth at the tips of stems and roots (Aston & Crafts, Citation1981). Research has shown that adding low rates of 2,4 DB to postemergence herbicides such as acifluorfen 5-[2-chloro-4-(trifluoromethyl)phenoxy]−2-nitrobenzoic acid and bentazon [3-isopropyl-1H-2,1,3-benzothiadiazin-4(3H)-one 2,2-dioxide] effectively controls velvetleaf in some soybean production systems (York et al., Citation1991).
The fungus, Fusarium lateritium Nees ex Fr. (FL) (), has shown potential as a bioherbicide for velvetleaf control, as well as for controlling spurred anoda (Anoda cristata L.) Schlecht. (Walker, Citation1981a), prickly sida (Sida spinosa L.) (Walker, Citation1981b). However, the utility of this fungus for velvetleaf control was limited due to the inability to control weeds larger than cotyledonary to first leaf stages of growth, and the requirement of at least 12 h of dew (Boyette & Walker, Citation1985). We hypothesised that it may be possible to apply this approach (i.e. applying a low rate of 2,4-DB and substituting a bioherbicidal agent for the herbicide) for controlling velvetleaf.
Figure 1. Photomicrograph of Fusarium lateritium macroconidia (average size ranged from 26 – 50 µm by 3 - 4 µm) as grown on modified vegetable juice agar (Miller, Citation1955).
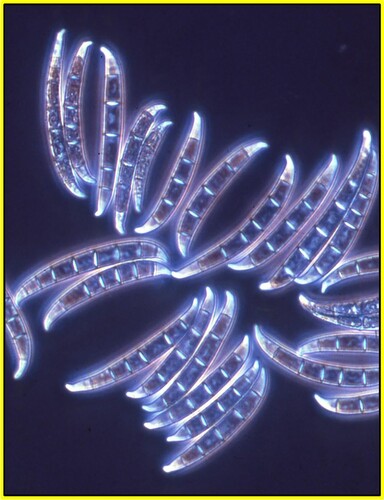
Materials and methods
Pathogen culture and storage
Cultures of Fusarium lateritium were grown on modified vegetable juice agar (Miller, Citation1955) in petri dishes inverted on open-mesh wire shelves in an incubator (Precision Scientific Inc., Chicago, IL, USA) at 25°C. Photoperiods (12 h) were provided by 20-W cool-white, fluorescent lamps, positioned 12 cm above each shelf to provide 200 μEm−2 s−1 photosynthetic active radiation (PAR). Conidia from 10 – to 12-day-old cultures were harvested by rinsing spore lawns on the petri dishes with distilled water and filtering debris through 4 layers of cheesecloth. Freshly harvested spores were used as the inoculum for all experiments. Spore concentrations were estimated and adjusted using haemacytometers. Long-term storage of the fungus was achieved in screw-capped tubes containing sterilised soil, maintained at 4°C (Bakerspigel, Citation1953).
Greenhouse experiments
In all experiments, velvetleaf plants were grown from seed obtained from Azlin Seed Co. (Leland , MS, USA) in a commercial potting mix contained in peat strips. Each strip contained 12 plants. The potting mix was supplemented with a slow-release fertiliser (14:14:14, NPK). The plants were placed in sub-irrigated trays on benches in the greenhouse. Greenhouse temperatures ranged from 25° C to 30° C with 40% to 60% relative humidity (RH). The photoperiod was 12 h with 1650 µEm2 s−1 PAR measured at midday.
Dew duration experiments
Treatments consisted of: 1) control plants treated with distilled water; 2) FL (at a concentration of 1.5 × 106 conidia ml−1) in distilled water; 3) 2,4-DB [Butoxone® (Aceto Agricultural Chemicals Corporation, Port Washington, NY, USA) at 0.02 kg ae ha−1]; 4) FL + 2,4-DB; 5) FL followed by (fb) 2-4-DB (after 5 min); 6) FL fb 2-4-DB (after 7 d); 7) 2,4-DB fb FL (after 5 min); and 8) 2,4-DB fb FL (after 7 d). Following spray applications, the plants were placed in dew chambers in the dark (Model I-36 DL; Percival Sci. Ind., Perry, IA, USA) at 25° C, 100% RH, for 2, 4, 6, 8, 10 or 12 h, and then placed on greenhouse benches. Surviving plants were excised at the soil line, oven-dried for 48 h at 85˚C, weighed, and the percent biomass reduction was determined.
Disease kinetics experiments – greenhouse
Treatments consisted of: 1) FL (at a concentration of 1.5 × 106 ml−1); 2) FL + 2,4-DB; 3) FL followed by (fb) 2-4-DB (after 5 min); and 4) 2,4-DB fb FL (after 5 min). The inoculated plants were placed in dew chambers with temperature and RH conditions as described above for 12 h, placed on greenhouse benches, and monitored over 12 d at 3-d intervals for disease kinetic studies. A subjective visual disease severity rating scale (per plant basis) (Sandrin et al., Citation2003) was used to estimate disease progression where: 0 = no disease, 1 = 1% – 25% disease, 2 = 26% – 50% disease, 3 = 51% – 75% disease, 4 = 76% – 99% disease, and 5 = plant death. Disease ratings ≤ 2.0 were considered ‘slight’, 2.1 - 3.9 were considered ‘moderate’, and ≥ 4.0 were considered ‘severe’.
Disease kinetics experiments – field
Field experiments were conducted on a Dundee very fine sandy loam (Aeric Ochraqualf) soil at the USDA-ARS, Crop Production Systems Research Unit at Stoneville, MS. Plots consisted of four rows of soybeans (‘Centennial’ cv.), 12.2 m long and 1 m apart, with the two centre rows receiving treatment. All rows were planted with scarified coffee senna seed at a density of about 100 seeds·m – 1 of row. Treatments consisted of: 1) FL (at a concentration of 1.5 × 106 ml−1) in distilled water; 2) FL + 2,4-DB; 3) FL fb 2,4 – DB at 0.02 kg ae ha−1 (after 5 min); 4) 2,4-DB fb FL (after 5 min). Velvetleaf plants were in the 5–7 leaf growth stage (40 cm, avg.) and were sprayed until fully wetted (approximately 200 L·ha – 1). Applications were made at midday with a hand-held pressurised sprayer. For the first year, environmental conditions at the time of inoculation and for 24 h following treatment were: temperature at inoculation, 33˚ C with a RH of 74%. The high temperature for the 24 h period was 33˚ C and the low temperature was 25˚ C. Maximum RH was 94%, with a dew period of 8 h. For the second year, environmental conditions at the time of inoculation and for 24 h following treatment were: temperature at inoculation, 36˚C with a RH of 58%. The high temperature for the 24 h period was 34˚C, and the low temperature was 24˚C. Maximum RH was 92%, with a dew period of 7 h. The plants were monitored for disease development at 3 d intervals for 12 d as described previously. Weed control was determined in two randomly selected 1 – m2 subplots from each treated plot, 12 d after treatment. A randomised complete block design was utilised, and the treatments were replicated four times. Data over the 2 years from the experiments were pooled following subjection to Bartlett’s test for homogeneity and analyzed using analysis of variance. Significant differences were determined using Fisher’s Protected Least Significant Difference (FLSD) at P = 0.05.
Quantification of interactions
Interactions between components in mixtures were analyzed according to Colby (Citation1967), using the formula E = XA + YB – (XA) (YB) / 100, where XA and YB represent plant survival herbicide XA (2,4-DB) and bioherbicide YB (FL), respectively. The observed response is obtained by comparing the activity of the single components (FL and 2,4-DB) with mixtures containing the same rate of the components applied singly. A deviation from the expected response, as calculated from the level of interaction (R) between the expected and the observed response of the two components, could indicate synergism or antagonism. Interactions are classified as additive when R = 1.0, synergistic when R > 1.0 and antagonistic when R < 1.0. Due to the inherent biological variability of the test systems, synergistic and antagonistic interactions are considered statistically significant when R ≥ 1.5 and R ≤ 0.5, respectively and additive interactions are significant at R values between 0.5 and 1.5 (Gisi et al., Citation1985).
Statistical analysis
In all experiments, the treatments were replicated four times. The experiments were repeated over time, and data were averaged following Bartlett’s test for homogeneity of variance (Steel et al., Citation1997). A randomised complete block experimental design with four replications was utilised. The mean percentage of plant mortalities and biomass reductions were calculated for each treatment and were subjected to arcsine transformation. The transformed data were statistically compared using analysis of variance (ANOVA) at the 5% probability level. Results were back-transformed to the original measurements (percentages) for presentation. Data were analyzed via standard mean errors and best-fit regression analysis. All data were analyzed using the PROC MIXED function of SAS v 9.3 (SAS Institute, Cary, NC, USA).
Results and discussion
Greenhouse experiments
Fl and 2,4-DB effects on mortality and biomass
Macroconida of FL () were used in these experiments to test the efficacy on velvetleaf plants when applied alone or in combination with 2,4-DB. Following an 8-h dew treatment, high levels of velvetleaf mortality (94%) and biomass reduction (98%) were achieved when 2,4-DB was applied prior to FL under greenhouse conditions (). The level of weed mortality achieved by FL alone was only 6%, and that of 2, 4 – DB alone was 22% and a mixture of FL and 2,4-DB also resulted in 22% mortality (). When FL was applied prior to 2,4-DB, mortality of only 22 - 24% occurred ().
Table 1. Biological control of Abutilon theophrasti under greenhouse conditions by an interaction with the herbicide 2,4-DB.
Effects of dew duration
Dew periods greater than 8 h provided no significant increase in mortality or biomass reduction of velvetleaf seedlings caused by FL ( and ). Plant mortality resulting from treatment of 2,4-DB followed by FL after 5 min was represented by a second-degree polynomial regression curve which provided the best fit to the data, that is best described by the equation: Y = 7.43 + 15.75 - 0.70X2, R2 = 0.96 (). Plant biomass reductions followed a similar trend and are represented by a second-degree polynomial regression curve which provided the best fit to the data, with the relationship best described by the equation: Y = 7.97 + 16.75X – 0.79X2, R2 = 0.96 (). Tan to dark-brown lesions were visible 3 days (not shown) following inoculation with an 8 h dew period, and severity increased 5 days after treatment with 2,4-DB followed 5 min later by an inoculative spray of FL conidia (). More severe lesion development (lesion coalescence) occurred after an 8-day treatment ().
Figure 2. Effect of dew period duration at 25 °C on mortality of velvetleaf inoculated with F. lateritium at 1.5 × 106 conidia ml−1 and 2,4-DB under greenhouse conditions. The relationship for these components is best described by the following equations: FL (open circle, short dash), Y = – 0.33 + 0.18X + 0.03X2, R2 = 0.96; 2,4-DB (open triangle, long dash), Y = – 0.93 + 0.24X + 0.16X2, R2 = 0.96; FL + 2,4-DB (open inverted triangle, small dots), Y = – 1.29 + 0.43X + 0.14X2, R2 = 0.96; FL fb 2,4-DB (solid square, long dash + small dots), Y = −1.60 + 0.73X – 0.12X2, R2 = 0.97; 2,4-DB fb FL (solid sphere, solid line), Y = 7.43 + 15.75 - 0.70X2, R2 = 0.96. Control values (solid triangle). Error bars represent ± 1 SEM.
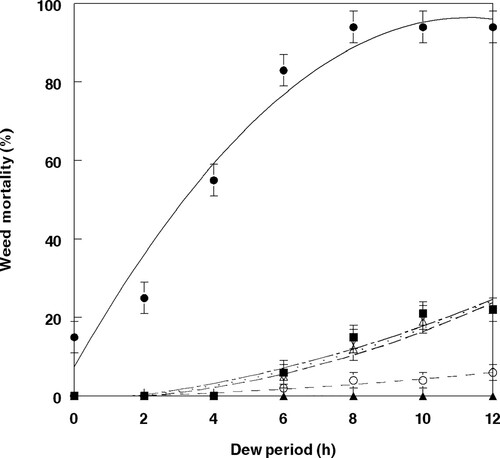
Figure 3. Effect of dew period duration at 25 °C on biomass reduction of velvetleaf inoculated with F. lateritium at 1.5 × 106 conidia ml−1 and 2,4-DB under greenhouse conditions. Error bars represent standard mean errors. The relationship for these components is best described by the following equations: FL (open circle, short dash), Y = – 0.81 + 0.43X + 0.06X2, R2 = 0.94; 2,4-DB (open triangle, long dash), Y = – 1.40 + 0.43X + 0.06X2, R2 = 0.97; FL + 2,4-DB (open inverted triangle, small dots), Y = – 1.67 + 0.82 X + 0.15X2, R2 = 0.96; FL fb 2,4-DB (solid square, long dash + small dots), Y = – 1.52 + 0.66X + 0.17X2, R2 = 0.97; 2,4-DB fb FL (solid sphere, solid line), Y = 7.97 + 16.75X – 0.79X2, R2 = 0.96. Control values (solid triangle). Error bars represent ± 1 SEM.
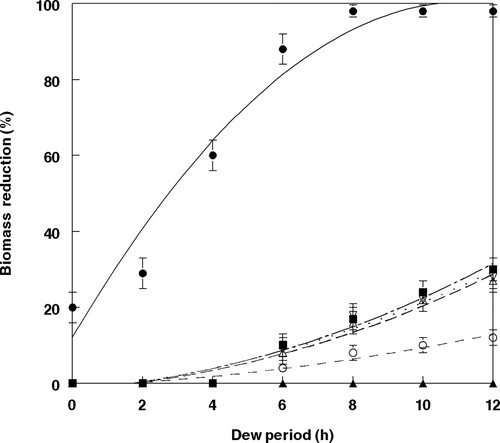
Field experiments
Results from field experiments corroborated the findings from greenhouse experiments. The only significant velvetleaf mortality and biomass reduction was achieved in test plots treated with 2, 4 – DB followed 5 min later by an FL treatment in field experiments (). The bioherbicide treatment alone caused only minimal effects, whereas FL applied to plants prior to herbicide application resulted in levels of mortality and biomass reduction equal to that of the herbicide alone suggesting herbicide toxicity to the fungus.
Table 2. Biological control of Abutilon theophrasti under field conditions by an interaction with the herbicide 2, 4 – DB.
An analysis of the possible interactions of the herbicide and bioherbicide under greenhouse and field conditions revealed that a strong synergistic interaction occurred when 2,4-DB was applied prior to FL, and that only slight additive interactions occurred with the other herbicide:pathogen treatments ().
Table 3. Interaction of Fusarium lateritium and 2,4 – DB relative to plant survival of velvetleaf under greenhouse and field conditions as analyzed using Colby’s method (Colby, Citation1967).
Disease kinetics – greenhouse
Analysis of plant injury over a 12 – day period indicated that the injury severity progressed rapidly when plants were sprayed with 2,4-DB followed by FL either 5 min or 7 d after the herbicide treatment. Because there was no significant difference in plant injury at either pathogen inoculation time interval, only data from the 2,4-DB followed by FL after 5 min are presented. Extensive leaf wilting occurred as leaf spotting lesions coalesced by 8–9 days after application. The leaves were extensively blighted, and large stem lesions were evident (). The effects on biomass reduction from the treatment of 2,4-DB followed by FL after 5 min was represented by a second-degree polynomial regression curve which provided the best fit to the data, and the relationship was best described by the equation: Y = 0.31 + 1.44X – 0.012X2, R2 = 0.98 (). Plant injury was significantly reduced when FL was applied alone, or prior to the herbicide treatment, either after 5 min or 7 d. No injury occurred to plants treated with water only, thus a regression equation is not shown.
Figure 6. Disease progression of F. lateritium (at 1.5 × 106 conidia ml−1) and 2,4-DB (at 0.02 kg a.e ha−1) infecting hemp sesbania under greenhouse conditions based upon disease rating of 0 – to 5, where 0 = no disease and 5 = plant mortality. Spray application rates were approximately 200 L ha−1. The relationship for these components is best described by the following equations: FL (open circle, short dash), Y = – 0.02 + .007 X, R2 = 0.96; FL + 2,4-DB (open inverted triangle, small dots), Y = 0.007 + 0.44 X + 0.04 X2, R2 = 0.98; FL fb 2,4-DB after 5 min (solid square, long dash + small dots), Y = 0.17 + 0.04 X – 0.02 X2, R2 = 0.98; 2,4-DB fb FL after 5 min (solid sphere, solid line), Y = 0.31 + 1.44 X – 0.01 X2, R2 = 0.98. Error bars represent ± 1 SEM.
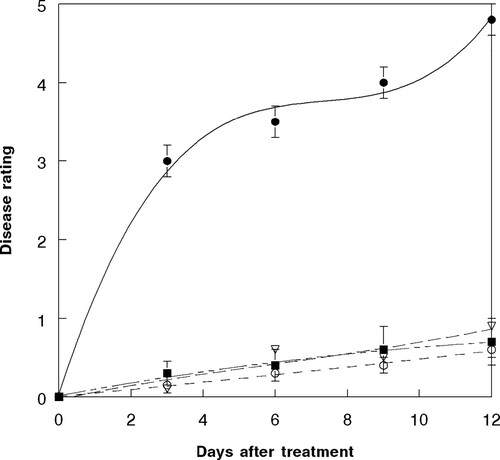
Disease kinetics – field
Because greenhouse results demonstrated that no significant differences in plant injury occurred at either pathogen inoculation time interval, only data from the 2,4-DB followed by FL after 5 min are presented. The relationship was best described by the equation: Y = 0.01 - 0.44 X – 0.01 X3, R2 = 0.98 (). No visible soybean damage was observed (data not shown). As in greenhouse experiments, a strong synergistic interaction occurred when 2,4-DB was applied prior to FL, and only slight additive interactions occurred with the other herbicide:pathogen treatments under field conditions ().
Figure 7. Disease progression of F. lateritium (at 1.5 × 106 conidia ml−1) and 2,4-DB (at 0.02 kg a.e ha−1) infecting hemp sesbania under field conditions based upon disease rating of 0 – to 5, where 0 = no disease and 5 = plant mortality. Spray application rates were approximately 200 L ha−1. The relationship for these components is best described by the following equations: FL (open circle, short dash), Y = – 0.02 + .007 X, R2 = 0.96; FL + 2,4-DB (open inverted triangle, small dots), Y = 0.007 + 0.44 X + 0.04 X2, R2 = 0.98; FL fb 2,4-DB after 5 min (solid square, long dash + small dots), Y = 0.17 + 0.04 X – 0.02 X2, R2 = 0.98; 2,4-DB fb FL after 5 min (solid sphere, solid line), Y = 0.01 - 0.44 X – 0.01 X3, R2 = 0.98. Error bars represent ± 1 SEM.
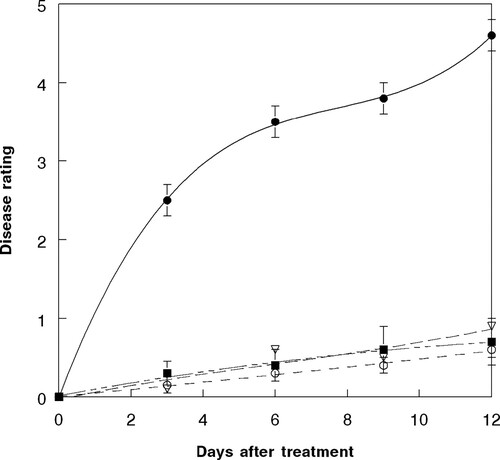
The biocontrol efficacies of some bioherbicidal pathogens can result in additive or synergistic effects via weakening weed defenses when combined with certain agrochemicals (Caulder & Stowell, Citation1988; Christy et al., Citation1993; Hoagland, Citation1996; Mitchell et al., Citation2008; Peng & Wolf, Citation2011). Sub-lethal doses of glyphosate were reported to suppress phytoalexin biosynthesis in sicklepod (Senna obtusifolia L.), reducing resistance of the weed to fungal infection by Alternaria cassiae and facilitating disease development (Sharon et al., Citation1992). The bioherbicidal activity of Pyricularia setariae Sacc. on green foxtail (Setaria viridis L.) was shown to be synergized by the herbicides quinclorac (3,7-dichloro-8-quinolinecarboxylic acid), glufosinate [(2-amino-4-[hydroxy(methylphosphonoyl)]butanoic acid and glyphosate (N-(phosphonomethyl) glycine) (Peng & Byer, Citation2005). Additive and/or synergistic effects on growth, chlorophyll accumulation, and mortality when sub-lethal concentrations of Albifimbria verrucaria [formerly Myrothecium verrucaria (Alb. & Schwein.) Ditmar (MV)] (Weaver et al., Citation2021) and quinclorac were applied to plant tissues of hemp sesbania (Sesbania exaltata (Raf.) Cory.), sicklepod, and kudzu Pueraria montana var. lobata Willd. (Ohwii)] seedlings (Hoagland et al., Citation2011). Additive or synergistic interactions also resulted in improved control of hemp sesbania following combinations of glyphosate and the bioherbicidal fungus, Colletotrichum truncatum (Schw.) Andrus & Moore) (Boyette et al., Citation2008a). Synergistic or additive interactions of MV and glyphosate on kudzu, redvine [Brunnichia ovata (Walt.) Shinners], and trumpetcreeper (Campsis radicans L.) have been demonstrated (Boyette et al., Citation2006; Boyette et al., Citation2008b). Weaver et al. (Citation2012) reported antagonistic interactions of several commercial glyphosate products and MV. The glyphosate product (Touchdown®, Syngenta Crop Protection, Greensboro, NC, USA) combined with MV spores exhibited additive and synergistic interactions for controlling kudzu in the greenhouse and field (Boyette et al., Citation2014). Phoma herbarum West. was found to be compatible with non-formulated 2,4-D (2, 4 – dichlorophenoxyacetic acid) and their combination exhibited synergistic interaction for the control of dandelion (Taraxacum officinal) under field conditions (Schnick & Boland, Citation2004). In other studies with phenoxy herbicides, combinations of Phoma proboscis conidia with a mixture of 2,4 – D and MCPP [2-(2-methyl-4-chlorophenoxy)propionic acid] caused high mortality of mature field bindweed (Convolvulus arvensis) when dew periods ranged from 8 to 10 h (Heiny, Citation1994).
The results of the studies presented in this report demonstrate that there was a synergistic effect upon weed control of velvetleaf when a conidial suspension of FL was applied subsequent to a sub-lethal dose of commercially formulated 2,4-DB. These data also suggest that a fungicidal response may have occurred when FL and 2,4-DB are tank-mixed, and that a fungistatic response may have occurred when FL was applied prior to 2,4-DB application. Herbicides may affect many secondary and tertiary pathways and/or enzymes in plants some of which are closely related to plant disease defenses. Furthermore, herbicides may also directly influence the biochemistry and infectivity mechanisms of the bioherbicide (Hoagland, Citation1996). Additional comprehensive investigations on plant and pathogen biochemistry and molecular biology with respect to a specific bioherbicide, herbicide and weed will be necessary to define the intricacies of such herbicide:bioherbicide interactions.
Acknowledgements
We thank Robin H. Jordan for valuable technical assistance.
Disclosure statement
No potential conflict of interest was reported by the author(s).
References
- Aston, F. M., & Crafts, A. S. (1981). Phenoxys. In F. M. Ashton, & A. S. Crafts (Eds.), Mode of action of herbicides (pp. 22–23). John Wiley and Sons.
- Bakerspigel, A. (1953). Soil as a storage medium for fungi. Mycologia, 45(4), 596–604. https://doi.org/10.1080/00275514.1953.12024301
- Boyette, C. D., Hoagland, R. E., & Weaver, M. A. (2008a). Interaction of a bioherbicide and glyphosate for controlling hemp sesbania in glyphosate-resistant soybean. Weed Biology and Management, 8(1), 18–24. https://doi.org/10.1111/j.1445-6664.2007.00269.x
- Boyette, C. D., Hoagland, R. E., Weaver, M. A., & Reddy, K. N. (2008b). Redvine (Brunnichia ovata) and trumpetcreeper (Campsis radicans) controlled under field conditions by a synergistic interaction of the bioherbicide Myrothecium verrucaria and glyphosate. Weed Biology and Management, 39–45.
- Boyette, C. D., Hoagland, R. E., Weaver, M. A., & Stetina, K. C. (2014). Interaction of the bioherbicide Myrothecium verrucaria and glyphosate for kudzu control. American Journal of Plant Sciences, 5(26), 3943–3956. https://doi.org/10.4236/ajps.2014.526413
- Boyette, C. D., Reddy, K. N., & Hoagland, R. E. (2006). Glyphosate and bioherbicide interaction for controlling kudzu (Pueraria lobata), redvine (Brunnichia ovata) and trumpetcreeper (Campsis radicans). Biocontrol Science and Technology, 16(10), 1067–1077. https://doi.org/10.1080/09583150600828742
- Boyette, C. D., & Walker, H. L. (1985). Factors influencing biocontrol of velvetleaf (Abutilion theophrasti) and prickly sida (Sida spinosa) with Fusarium lateritium. Weed Science, 33(2), 209–211. https://doi.org/10.1017/S0043174500082114
- Caulder, J. D., & Stowell, L. J. (1988). Synergistic herbicidal compositions comprising Colletotrichum truncatum and chemical herbicides. U.S. Patent, 4775405.
- Christy, A. L., Herbst, K. A., Kostka, S. J., Mullen, J. P., & Carlson, P. S. (1993). Synergizing weed biocontrol agents with chemical herbicides. In S. O. Duke, J. J. Menn, & J. R. Plimmer (Eds.), Pest control with enhanced environmental safety (pp. 87–100). American Chemical Society.
- Colby, S. R. (1967). Calculating synergistic and antagonistic responses of herbicide combinations. Weeds, 15(1), 20–22. https://doi.org/10.2307/4041058
- Colton, C. E., & Einhellig, F. A. (1980). Allelopathic mechanisms of velvetleaf (Abutilon theophrasti medic., Malvaceae) on soybean. American Journal of Botany, 67(10), 1407–1413. https://doi.org/10.1002/j.1537-2197.1980.tb07775.x
- Gisi, U., Binder, H., & Rimbach, E. (1985). Synergistic interactions of fungicides with different modes of action. Transactions of the British Mycological Society, 85(2), 299–306. https://doi.org/10.1016/S0007-1536(85)80192-3
- Hagood, E. S., Jr., Bauman, T. T., Williams, T. L., Jr., & Schreiber, M. M. (1980). Growth analysis of soybean (Glycine max) in competition with velvetleaf (Abutilon theophrasti). Weed Science, 28(6), 729–734. https://doi.org/10.1017/S0043174500061622
- Heiny, D. K. (1994). Field survival of Phoma proboscis and synergism with herbicides for control of field bindweed. Plant Disease, 78(12), 1156–1164. https://doi.org/10.1094/PD-78-1156
- Hoagland, R. E. (1996). Chemical interactions with bioherbicides to improve efficacy. Weed Technology, 10(3), 651–674. https://doi.org/10.1017/S0890037X00040586
- Hoagland, R. E., Boyette, C. D., & Vaughn, K. C. (2011). Interactions of quinclorac with a bioherbicidal strain of Myrothecium verrucaria. Pest Technology, 5, 88–96.
- Lindquist, J. L., Mortensen, D. A., Clay, S. A., Schmenk, R., Kells, J. J., Howatt, K., & Westra, P. (1996). Stability of corn (Zea mays) – velvetleaf (Abutilon theophrasti) interference relationships. Weed Science, 44(2), 309–313. https://doi.org/10.1017/S0043174500093930
- Lueschen, W. E., & Andersen, R. N. (1980). Longevity of velvetleaf (Abutilon theophrasti) seeds in soil under agricultural practices. Weed Science, 28(3), 341–346. https://doi.org/10.1017/S0043174500055429
- Miller, P. M. (1955). V-8 juice agar as a general-purpose medium for fungi and bacteria. Phytopathology, 45, 461–462.
- Mitchell, J. K., Yerkes, C. N., Racine, S. R., & Lewis, E. H. (2008). The interaction of two potential fungal bioherbicides and a sub-lethal rate of glyphosate for the control of shattercane. Biological Control, 46(3), 391–399. https://doi.org/10.1016/j.biocontrol.2008.02.009
- Peng, G., & Byer, K. N. (2005). Interactions of Pyricularia setariae with herbicides for control of green foxtail (Setaria viridis). Weed Technology, 19(3), 589–598. https://doi.org/10.1614/WT-04-130R.1
- Peng, G., & Wolf, T. M. (2011). Synergy between synthetic and microbial herbicides for weed control. Pest Technology, 5, 18–27.
- Sandrin, T. R., TeBeest, D. O., & Weidemann, G. J. (2003). Soybean and sunflower oils increase the infectivity of Colletotrichum gloeosporioides f. sp. aeschynomene to northern jointvetch. Biological Control, 26(3), 244–252. https://doi.org/10.1016/S1049-9644(02)00156-1
- Sattin, M., Zanin, G., & Berti, A. (1992). Case history for weed competition/population ecology: velvetleaf (Abutilon theophrasti) interference in corn (Zea mays). Weed Technology, 6(1), 213–219. https://doi.org/10.1017/S0890037X00034588
- Schnick, P. J., & Boland, G. J. (2004). 2,4-D and Phoma herbarum to control dandelion (Taraxacum officinale). Weed Science, 52(5), 808–814. https://doi.org/10.1614/WS-03-085R
- Sharon, A., Amsellem, Z., & Gressel, J. (1992). Glyphosate suppression of an elicited defense response: Increased susceptibility of Cassia obtusifolia to a mycoherbicide. Plant Physiology, 98(2), 654–659. https://doi.org/10.1104/pp.98.2.654
- Spencer, N. R. (1984). Velvetleaf, Abutilon theophrasti (Malvaceae), history and economic impact in the United States. Economic Botany, 38(4), 407–416. https://doi.org/10.1007/BF02859079
- Steel, R. G. D., Torrey, J. H., & Dickeys, D. A. (1997). Multiple comparisons. Principles and procedures of statistics – a biometrical approach. McGraw-Hill, p. 365
- Toole, E. H., & Brown, E. (1946). Final results of the buried seed experiment. Journal of Agricultural Research, 72, 201–210.
- Walker, H. L. (1981a). Factors affecting biological control of spurred anoda (Anoda cristata) with Alternaria macrospora. Weed Science, 29(4), 505–507. https://doi.org/10.1017/S0043174500040078
- Walker, H. L. (1981b). Fusarium lateritium: A pathogen of spurred anoda (Anoda cristata), prickly sida (Sida spinosa), and velvetleaf (Abutilon theophrasti). Weed Science, 29(6), 629–631. https://doi.org/10.1017/S0043174500040170
- Warwick, S. I., & Black, L. D. (1988). The biology of Canadian weeds. 90. Abutilon theophrasti. Canadian Journal of Plant Science, 68(4), 1069–1085. doi:10.4141/cjps88-127
- Weaver, M. A., Boyette, C. D., & Hoagland, R. E. (2012). Bioherbicidal activity from washed spores of Myrothecium verrucaria. World Journal of Microbiology and Biotechnology, 28(5), 1941–1946. https://doi.org/10.1007/s11274-011-0996-8
- Weaver, M. A., Hoagland, R. E., Boyette, C. D., & Brown, S. P. (2021). Taxonomic evaluation of a bioherbicidal isolate of Albifimbria verrucaria, formerly Myrothecium verrucaria. Journal of Fungi, 7(9), 694. https://doi.org/10.3390/jof7090694
- York, A. C., Wilcut, J. W., Keene, M. M., & Walls, F. R., Jr. (1991). Soybean (Glycine max) response to postemergence herbicide mixtures containing 2,4-DB. Weed Technology, 5(1), 43–47. https://doi.org/10.1017/S0890037X00033236