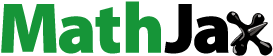
ABSTRACT
Excessive F- in drinking water due to natural and anthropogenic activities is a serious health hazard affecting humans worldwide. In this study, a comparative assessment was made of eight mineral-based materials with advantageous structural properties for F- uptake: layered-double-hydroxides (LDHs), geopolymers, softening pellets and struvite. These materials are considered low-cost, for being either a waste or by-product, or can be locally-sourced. It can be concluded that Ca-based materials showed the strongest affinity for F- (Ca-Al-CO3 LDHs, slag-based geopolymer, softening pellets). The Langmuir adsorption capacity of Ca-Al-CO3 LDHs, slag-based geopolymer and softening pellets was observed to be 20.83, 5.23 and 1.20 mg/g, respectively. The main mechanism of F- uptake on Ca-Al-CO3 LDHs, Mg-Al-Cl LDHs, slag-based geopolymers and softening pellets was found to be sorption at low initial F- concentrations (<10 mg/L) whereas precipitation as CaF2 is proposed to play a major role at higher initial F- concentrations (>20 mg/L). Although the softening pellets had the highest Ca-content (96-97%; XRF), their dense structure and consequent low BET surface area (2–3 m2/g), resulted in poorer performance than the Ca-based LDHs and slag-based geopolymers. Nevertheless, geopolymers, as well as struvite, were not considered to be of interest for application in water treatment, as they would need modification due to their poor stability and/or F- leaching.
GRAPHICAL ABSTRACT
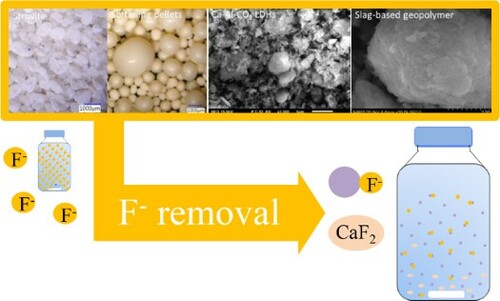
1. Introduction
Fluoride (F-) is very important to human health, as it prevent tooth decay, however, excessive F- intake via drinking water, at concentrations above 1.5 mg/L, causes dental or skeletal fluorosis [Citation1] and hormone imbalance of the thyroid [Citation2]. Fluorosis due to F- in drinking water has affected around 200 million people from 35 nations over the world [Citation3,Citation4]. In order to mitigate F- exposure through drinking water, various methods have been developed to remove F- from water. Particularly, F- sorption and precipitation are methods of interest, as F- ions are small and, as such, difficult to remove with most separation technologies, including membranes [Citation5]. In the past several decades, over 100 materials have been developed for the removal of F- [Citation6,Citation7], including, activated alumina, granular ferric hydroxides and rare earth oxide-based materials [Citation8–10]. Particularly mineral-based materials are promising for F- removal for drinking water production [Citation6], due to the fact that mineral-based materials generally possess a layered or reticulate structure with electropositive multivalent metal ions [Citation11]. This positive charge on the surface could attract the small F- anions [Citation12].
provides an overview of tested mineral-based materials, illustrating the variability in performance for F- removal capacity (qe). The materials with high qe, such as Mg-Al-CO3 LDHs, nanomagnesia and calcium-rich Attapulgite, have generally been tested at F- concentrations higher than typically found in natural groundwater, complicating extrapolation to field-relevant conditions. An additional challenge is that most well-performing materials, such as nano goethite [Citation13] and CaO nanoparticles [Citation14], are not widely available, making their supply chain for rural water supply challenging. Finally, quick lime and limestone could not obtain an equilibrium F- concentration below 7.9 mg/L, due to the solubility constraints of CaF2, making it unacceptable for drinking water production [Citation15].
Table 1. Overview of F- removal by mineral-based materials.
Various mineral-based materials have thus been tested to remove F- from water, but affordable and accessible materials for typical F- concentrations in natural groundwater remain limited. The objective of this study is, therefore, to perform a comparative assessment of low-cost mineral-based materials, from the perspective of their F- removal capacity, structure and stability. Eight different materials were selected, based on their presumed affinity for F-, as their structure/composition resembles earlier reported F- materials: Layered Double Hydroxides (LDHs) (Ca-Al-CO3 LDHs and Mg-Al-Cl LDHs), geopolymers (slag-, fly ash-, metakaolin-based), softening pellets (two types) and struvite. The first being a natural clay and the others being sourced from waste streams, namely, from industrial processes, drinking water treatment and wastewater treatment.
LDHs and their modified products are considered as effective adsorbents for removal of a variety of anionic and cationic pollutants from aqueous solutions such as F-, Cl-, BrO3-, P, NO3-, As(III) and heavy metals such as Cr(VI), Cu(II), Cd(II), Pb(II) [Citation25–32]. Slag, fly ash and metakaolin are solid precursors of geopolymers and also have promising removal capacities for F- [Citation19,Citation33,Citation34]. However, F- removal by geopolymers is rarely reported. Softening pellets are spherical crystal beads of calcite nature and a waste product of hardness removal in pellet crystallization reactors [Citation35]. Other calcium carbonate rich materials such as calcite particles, limestone and eggshell have been observed to have affinity for F- sorption [Citation36–38], making softening pellets an attractive alternative material. Struvite is magnesium ammonium phosphate hexahydrate (MgNH4PO4·6H2O, MAP) [Citation39] and occurs naturally in geochemical and biological systems [Citation40,Citation41]. Struvite recovered from wastewater has been observed to remove arsenic (As) through adsorption [Citation42] and co-precipitation [Citation43], but has so far not been investigated for F- removal.
2. Materials and methods
2.1. Materials and their preparation
Ca-Al-CO3 LDHs and Mg-Al-Cl LDHs were supplied by Nabaltec (Germany) and Shaoyang Tiantang Additives Chemical Co., Ltd (China), respectively. Three types of geopolymers, slag-, fly ash- and metakaolin-based geopolymers, were prepared in the laboratory using commercial blast furnance slag (ORCEM, the Netherlands), fly ash (VLIEGASUNIE BV, the Netherlands), and metakaolin (Sigma-Aldrich). The softening pellets and struvite were obtained from full-scale installations, Dutch groundwater treatment plants (Bunnik and Sint Jansklooster) and a crystallization reactor, respectively.
The preparation process of geopolymers was as follows: 100 g of solid precursors was mixed with 14M NaOH solution using a molar ratio of SiO2/Na2O=1.0. Geopolymer paste then started to form, which was mixed for 3–5 min to give complete homogenization. Then the mixtures were put in a polypropylene bottle (200 mL) precured for 28 days in a curing chamber, which had a temperature of 20 ℃ [Citation44]. Before use, apart from the LDHs, all materials were crushed using a ball mill, then washed using deionized water at least three times and dried in an oven at 65 ℃ for 24 h (25 ℃ for struvite).
F- solutions with different concentrations were prepared with sodium fluoride (NaF) (Sigma-Aldrich) as the source. 2.21 g NaF was dissolved in 1L deionized water to prepare the stock solution and then diluted into desired concentrations.
2.2. Characterization of materials
Digital images of the materials were taken by a digital microscope (KEYENCE VHX-5000). The specific surface area of the materials was determined by a gravimetric nitrogen Brunauer–Emmett–Teller (BET) specific surface area analysis device (Micrometrics Gemini VII 2390 V1.03). The chemical composition was determined by X-ray fluorescence (Panalytical Epsilon 3 XL). The surface morphology was carried out using the scanning electron microscope (SEM) (JEOL JSM-IT100).
2.3. Batch experiments
Batch equilibrium experiments were conducted in six glass bottles (100 mL with cap) with 100 mL F- solutions at room temperature (25 ± 1℃). Initial solutions were adjusted to pH 8.5 (after materials dosing) using HCl (0.1 M) and NaOH (0.1 M), because F--containing groundwater, e.g. in China, tend to have a pH>8 [Citation45]. The initial F- concentrations were 2, 5, 10, 20, 40 and 80 mg/L. For the softening pellets, struvite and geopolymers, the dose was 20 g/L. For the Ca-Al-CO3 LDHs and Mg-Al-Cl LDHs, the dose was 1 and 10 g/L, respectively, which was adjusted according to their performance in preliminary experiments. The results of preliminary experiments indicated that the equilibrium time for LDHs, geopolymers, softening pellets and struvite were 1, 7 days, 7 and 4 days, respectively. After constant stirring for equilibrium, the solutions were filtered through a 0.45 μm membrane and were then analyzed by Ion chromatography (IC) (Metrohm, Switzerland).
The equilibrium uptake capacity (qe: mg/g) of the materials at different initial F- concentrations were calculated using the formula:
where C0 (mg/L) and Ce (mg/L) are initial and equilibrium F- concentrations, respectively; V (L) is the initial volume of solution; and m (g) is the mass of the material.
2.4. Ion leaching from materials
Ion leaching experiments were carried out by adding the materials (the same dose as the batch equilibrium experiments) into 100 mL of deionized water, which had been adjusted to pH 8.5 by dosing HCl (0.01M) and NaOH (0.01 M). Then the mixture was to stand for the same amount of time as the batch equilibrium experiments, and was then filtered by 0.45 μm membrane. The concentrations of cations (K+, Ca2+, Na+, Mg2+, NH4+) and anions (F-, Cl-, NO3-, NO2-, PO43-, SO42-) leaching from materials were measured using IC.
2.5. Dissolution and precipitation modelling using PHREEQC
PHREEQC is a geochemical model that can be used in speciation and saturation-index calculations, batch-reaction, one-dimensional reactive-transport, and inverse modelling [Citation46]. In this study, PHREEQC was employed to calculate the dissolution of materials (softening pellets and struvite) and precipitation of calcium fluoride (CaF2) and to calculate the solubility and relative thermodynamic stability of the materials. To study dissolution, a beaker was defined in the model with demineralized water to which the minerals calcite and (or) struvite were added. Step-wise, the minerals dissolved to equilibrium, and the concentrations and saturation indexes were calculated and plotted. The thermodynamic information on struvite was added because the phreeqc.dat database did not contain this information (included in supplementary data). For precipitation, F- solution was defined by using the experimental major-ion data. Detailed descriptions of simulation and input files can be found in example 2 and 3 from Parkhurst and Appelo [Citation46].
3. Results and discussion
3.1. Digital microscope and SEM images
shows the digital microscope and SEM images of LDHs (A), geopolymers (B), softening pellets (C) and struvite (D). The SEM images of LDHs show that Ca-Al-CO3 LDHs (1–5μm) has finer particles than Mg-Al-Cl LDHs (5–40μm). The particle sizes of geopolymers were distributed from approximately 1–100 μm. A flake structure was observed for the metakaolin-based geopolymer. C depicts digital microscope and SEM images of the softening pellets, showing the dense structure of the crystal. The softening pellets ranged in size from 0.5 mm to approximately 2 mm. D depicts images by digital microscope and SEM of the struvite, and illustrates that the crystal is constructed from small subunits with porous nature. The struvite particles were approximately 1 mm. The particle sizes of the crashed softening pellets and struvite were in the range from 1–5 μm.
3.2. Specific surface area (BET) and chemical composition (XRF)
It has been hypothesized that a high specific surface area is advantageous for sorbents, since it increases the number of surface sorption sites per unit area [Citation5]. However, it has also been suggested that the fractures, pore structure, size, and shape can impact the sorption rate and capacity by hindering the solute transport process [Citation5,Citation47]. BET surface areas of the selected materials are presented in . The results indicate that all materials have a relatively small specific surface area, below 10 m2/g, except for struvite (13.2 m2/g). Softening pellets had the lowest surface area (2.4 and 3.4 m2/g), which is lower than the value of 9.15 m2/g of commercial calcite [Citation48]. The surface areas of LDHs (7.6 and 8.4 m2/g) were relatively high, but still low compared with the values reported in literature (20–120 m2/g) [Citation24].
Table 2. BET surface area and XRF analysis of chemical composition.
The chemical composition of the materials, from XRF analyses, indicates that CaO was the predominant component of the softening pellets and Ca-Al-CO3 LDHs. Geopolymers consisted mainly of SiO2 and Al2O3, although the slag-based geopolymer also contained a high CaO concentration. MgO was the predominant component of the Mg-Al-Cl LDHs and struvite.
3.3. Fluoride uptake
A shows that for the same initial F- concentration, the F- uptake by Ca-Al-CO3 LDHs was higher than by Mg-Al-Cl LDHs. This cannot be explained by ion exchange, since it was reported that LDHs have greater affinity for multivalent inorganic anions compared with monovalent inorganic anions [Citation49], although Kameda et al. [Citation50] proposed that F- uptake by Mg-Al LDHs (Mg-Al-NO3 and Mg-Al-Cl LDHs) is the result of exchange between aqueous F- and NO3- or Cl- anions positioned in the Mg-Al LDHs interlayer [Citation50]. However, adsorption is more likely, also because Ca2+ presents a stronger affinity for F- than Mg2+ [Citation51]. Alternatively, the sudden shift in F- uptake at initial F- concentrations over 40 mg/L by Ca-Al-CO3 LDHs might also be explained by the precipitation of CaF2.
In B the F- uptake by the geopolymers is presented. The results indicate that the slag-based geopolymer was effective in F- removal, whereas the metakaolin-based geopolymer did not show a defluorination effect. The fly ash-based geopolymer only showed a limited F- removal at high initial F- concentrations (>20 mg/L), which may be explained by the release of F- into the solution (). The relatively good performance of slag-based geopolymers compared to fly ash- and metakaolin-based geopolymers may be explained by the presence of Ca2+ ().
C shows the F- uptake by softening pellets from softening reactors at water treatment plants Bunnik and Sint Jansklooster. It can be found that both pellets have a moderate affinity for F- in relation to the small surface area. For the same initial F- concentration, the F- uptake by softening pellets from Bunnik was higher than that from Sint Jansklooster. This could be caused by a different pellet composition, i.e. softening pellets from Bunnik have a higher content of CaO, whereas Sint Jansklooster have a higher content of Fe2O3 (). It has been suggested that the F- sorption capacity of Ca-rich attapulgite can go up to 140 mg/g, which was comparable with the sorption capacities of some nanoengineered materials [Citation22]. Such efficiencies were not achieved by softening pellets, likely due to the relatively low specific surface area.
The F- uptake by struvite was tested at pH 7.5 and 8.5, and it was found to be highest at pH 7.5 (D). The mechanism of F- sorption onto struvite has not been studied previously, but earlier research on As adsorption indicated that As forms monodentate mononuclear surface complexes with struvite through the formation of a Mg-O-As bond as the main removal mechanism [Citation42]. In addition, Brunet et al. [Citation39] found that tetracycline was removed via sorption and precipitation with struvite (approximately 16%–22%). Therefore, it is hypothesized that the uptake of F- on struvite can also be explained by the formation of (surface) complexes.
3.4. Equilibrium isotherms
provides an overview of the Langmuir and Freundlich isotherm parameters for the studied materials. The F- removal results obtained at higher F- concentrations (>20 mg/L) for Ca-Al-CO3 LDHs were considered to be influenced by the precipitation of CaF2 and therefore excluded from this analysis. In addition, the fly ash- and metakaolin-based geopolymers did not show affinity for F- sorption and were therefore not included in the isotherm model calculations. The Freundlich model provides a consistently better fit of the results than Langmuir. When viewing the Freundlich isotherm plots depicted in , the values of the Freundlich exponent 1/n (except for Mg-Al-Cl LDHs) are smaller than 1 (n>1) which is quite normal for sorption processes, still implying favourable conditions for higher initial concentrations and reflecting a chemisorption process [Citation52–54].
Table 3. Isotherm parameters for F- uptake on mineral-based materials.
3.5. Ions leaching and PHREEQC calculation
The results of ion leaching are shown in . It can be found that the concentrations of most ions leaching from softening pellets, Ca-Al-CO3 LDHs, Mg-Al-Cl LDHs and the metakaolin-based geopolymer, remained below 10 mg/L. For Ca2+, however, the concentrations were higher, with 13.2, 30.7 and 13.7 mg/L leaching from softening pellets, Ca-Al-CO3 LDHs and the slag-based geopolymer, respectively. The PHREEQC calculation indicated that approximately 0.25% of the softening pellets dissolved. For struvite, the molar concentration of Mg2+, NH4+ and PO43- was 2.8, 1.4 and 2.3 mmol/L, respectively, which indicates the occurrence of dissolution of struvite. PHREEQC calculations indicated that this corresponds to approximate 1.5% of struvite dissolution.
Figure 4. Ion leaching from low-cost mineral-based materials; Inset: F- leaching from geopolymers. (BGP: based geopolymer).
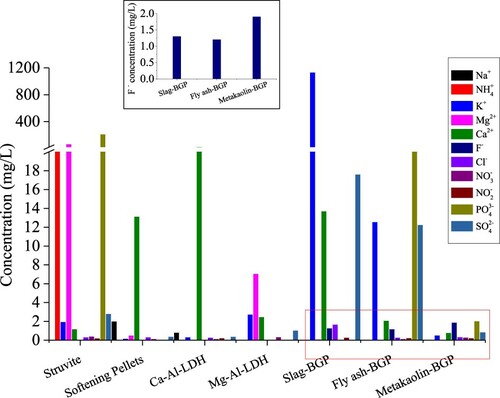
For the three geopolymers, F- leaching was detected, namely 1.3, 1.2 and 1.9 mg/L from slag-, fly ash- and metakaolin-based geopolymer respectively. This may influence the defluorination effect of geopolymers. Also, high concentrations of K+ (1134 mg/L) and SO42- (17.6 mg/L) were observed leaching from the slag-based geopolymer. From the fly ash-based geopolymer 23.5 mg/L PO43- and 12.3 mg/L SO42- were found to leach, which is undesirable from a drinking water supply perspective.
However, the consequence of the Ca2+ leaching (13.2 mg/L) could have resulted in F- removal by precipitation of CaF2. PHREEQC calculations indicated that the precipitation of CaF2 would occur for initial F- concentrations over 12.9 mg/L. It is therefore hypothesized that the mechanism of F- removal by Ca-Al-CO3 LDHs, the slag-based geopolymer and softening pellets is sorption at low initial F- concentrations, whereas precipitation plays a more prominent role at higher initial F- concentrations. This finding is of critical importance when considering the life-time of these materials, as re-use or generation, which is desirable from a sustainability and costs perspective, might be more challenging for precipitation products.
4. Conclusions
A comparative assessment of low-cost materials from the perspective of their F- removal capacity, structure and stability was performed. It can be concluded that Ca-based materials showed the strongest affinity for F- (Ca-Al-CO3 LDHs, slag-based geopolymer, softening pellets). The main mechanism of F- uptake on Ca-Al-CO3 LDHs, Mg-Al-Cl LDHs, slag-based geopolymers and softening pellets was found to be sorption at low initial F- concentrations (<10 mg/L), whereas precipitation as CaF2 is proposed to play a major role at higher initial F- concentrations (>20 mg/L). Although the softening pellets had the highest Ca-content (96–97%; XRF), their dense structure and consequent low BET surface area (2–3 m2/g), resulted in poorer performance than the Ca-based LDHs and slag-based geopolymers. Nevertheless, geopolymers, as well as struvite, should not be considered to be of interest for application in water treatment, as they would need modification due to their poor stability and/or F- leaching.
Supplementary_data.docx
Download MS Word (13.4 KB)Acknowledgements
The authors are grateful for the financial support from the China Scholarship Council (No. 201504910742). We express our sincere appreciation to Waterlab of Delft University of Technology. We thank John van den Berg from Microlab of Delft University of Technology for his assistance on the XRF and BET analysis. Yageng Li at the Department of Materials Science and Engineering of the Delft University of Technology is acknowledged for the SEM analysis.
Data availability statement
The data that support the findings of this study are available on request from the corresponding author.
Disclosure statement
No potential conflict of interest was reported by the author(s).
Correction Statement
This article was originally published with errors, which have now been corrected in the online version. Please see Correction (http://dx.doi.org/10.1080/09593330.2021.1946600)
Additional information
Funding
References
- Krupadam RJ, Khan MS, Das S. Adsorption of fluoride from water by surface-functionalized polyurethane foam. Water Sci Technol. 2010;62(4):759–765.
- Kheradpisheh Z, Mirzaei M, Mahvi AH, et al. Impact of drinking water fluoride on human thyroid hormones: a case-control study. Sci Rep. 2018;8(1):1–7.
- Ayoob S, Gupta AK. Fluoride in drinking water: a review on the status and stress effects. Crit Rev Env Sci Tec. 2006;36(6):433–487.
- Mohammadi AA, Yousefi M, Yaseri M, et al. Skeletal fluorosis in relation to drinking water in rural areas of West Azerbaijan, Iran. Sci Rep. 2017;7(1):1–7.
- Craig L, Stillings LL, Decker DL, et al. Comparing activated alumina with indigenous laterite and bauxite as potential sorbents for removing fluoride from drinking water in Ghana. Appl Geochem. 2015;56:50–66.
- Bhatnagar A, Kumar E, Sillanpää M. Fluoride removal from water by adsorption—a review. Chem Eng J. 2011;171(3):811–840.
- Shams M, Qasemi M, Dobaradaran S, et al. Evaluation of waste aluminum filing in removal of fluoride from aqueous solution. Fresenius Environ Bull. 2013;22:2604–2609.
- Bahena JLR, Cabrera AR, Valdivieso AL, et al. Fluoride adsorption onto α-Al2O3 and its effect on the zeta potential at the alumina–aqueous electrolyte interface. Sep Sci Technol. 2002;37(8):1973–1987.
- Jagtap S, Yenkie MK, Das S, et al. Synthesis and characterization of lanthanum impregnated chitosan flakes for fluoride removal in water. Desalination. 2011;273(2–3):267–275.
- Shams M, Nabizadeh Nodehi R, Hadi Dehghani M, et al. Efficiency of granular ferric hydroxide (GFH) for removal of fluoride from water. Fluoride. 2010;43(1):61.
- Miretzky P, Cirelli AF. Fluoride removal from water by chitosan derivatives and composites: a review. J Fluor Chem. 2011;132(4):231–240.
- Nie Y, Hu C, Kong C. Enhanced fluoride adsorption using Al (III) modified calcium hydroxyapatite. J Hazard Mater. 2012;233:194–199.
- Mohapatra M, Rout K, Gupta S, et al. Facile synthesis of additive-assisted nano goethite powder and its application for fluoride remediation. J Nanopart Res. 2010;12(2):681–686.
- Patel G, Pal U, Menon S. Removal of fluoride from aqueous solution by CaO nanoparticles. Sep Sci Technol. 2009;44(12):2806–2826.
- Turner BD, Binning P, Stipp S. Fluoride removal by calcite: evidence for fluorite precipitation and surface adsorption. Environ Sci Technol. 2005;39(24):9561–9568.
- Fan X, Parker DJ, Smith MD. Adsorption kinetics of fluoride on low cost materials. Water Res. 2003;37(20):4929–4937.
- Srimurali M, Pragathi A, Karthikeyan J. A study on removal of fluorides from drinking water by adsorption onto low-cost materials. Environ Pollut. 1998;99(2):285–289.
- Sarkar M, Banerjee A, Pramanick PP, et al. Use of laterite for the removal of fluoride from contaminated drinking water. J Colloid Interface Sci. 2006;302(2):432–441.
- Geethamani CK, Ramesh ST, Gandhimathi R, et al. Fluoride sorption by treated fly ash: kinetic and isotherm studies. J Mater Cycles Waste Manage. 2013;15(3):381–392.
- Islam M, Patel R. Evaluation of removal efficiency of fluoride from aqueous solution using quick lime. J Hazard Mater. 2007;143(1–2):303–310.
- Jain S, Jayaram RV. Removal of fluoride from contaminated drinking water using unmodified and aluminium hydroxide impregnated blue lime stone waste. Sep Sci Technol. 2009;44(6):1436–1451.
- Yin H, Kong M, Tang W. Removal of fluoride from contaminated water using natural calcium-rich attapulgite as a low-cost adsorbent. Water Air & Soil Pollution. 2015;226(12):1–11.
- Maliyekkal SM, Antony K, Pradeep T. High yield combustion synthesis of nanomagnesia and its application for fluoride removal. Sci Total Environ. 2010;408(10):2273–2282.
- Lv L, He J, Wei M, et al. Treatment of high fluoride concentration water by MgAl-CO 3 layered double hydroxides: kinetic and equilibrium studies. Water Res. 2007;41(7):1534–1542.
- Batistella L, Venquiaruto LD, Luccio MD, et al. Evaluation of acid activation under the adsorption capacity of double layered hydroxides of Mg–Al–CO3 type for fluoride removal from aqueous medium. Behav Brain Res. 2011;224(1):180–188.
- Caius R. Calcined Mg–Al layered double hydroxides for uptake of trace levels of bromate from aqueous solution. Ind.eng.Chem.res. 2011;50(15):9280–9285.
- Caporale AG, Pigna M, Azam SMGG, et al. Effect of competing ligands on the sorption/desorption of arsenite on/from Fe-Mg-layered double hydroxides (Fe-Mg-LDH). Chem Eng J. 2013;225(3):704–709.
- Das J, Patra BS, Baliarsingh N, et al. Adsorption of phosphate by layered double hydroxides in aqueous solutions. Appl Clay Sci. 2006;32(3–4):252–260.
- Halajnia A, Oustan S, Najafi N, et al. The adsorption characteristics of nitrate on Mg–Fe and Mg–Al layered double hydroxides in a simulated soil solution. Appl Clay Sci. 2012;70(7):28–36.
- Lazaridis NK, And TAP, Matis KA. Chromium(VI) removal from aqueous solutions by Mg−Al−CO3 hydrotalcite: sorption−desorption kinetic and equilibrium studies. Ind Eng Chem Res. 2004;43(9):2209–2215.
- Lv L, Sun P, Gu Z, et al. Removal of chloride ion from aqueous solution by ZnAl-NO 3 layered double hydroxides as anion-exchanger. J Hazard Mater. 2009;161(s 2–3):1444–1449.
- Pérez MR, Pavlovic I, Barriga C, et al. Uptake of Cu 2+, Cd 2+ and Pb 2+ on Zn–Al layered double hydroxide intercalated with edta. Appl Clay Sci. 2006;32(s 3–4):245–251.
- Horváth E, Frost RL, Makó É, et al. Thermal treatment of mechanochemically activated kaolinite. Thermochim Acta. 2003;404(1–2):227–234.
- Islam M, Patel R. Thermal activation of basic oxygen furnace slag and evaluation of its fluoride removal efficiency. Chem Eng J. 2011;169(1):68–77.
- Rankin AH, Sutcliffe PJC. Morphology, chemistry and growth mechanisms of calcite concretions from an industrial water-softening process: implications for the origin of natural ooids in sediments. P Geologist Assoc. 1999;110(1):33–40.
- Bhaumik R, Mondal NK, Das B. Eggshell powder as an Adsorbent for removal of fluoride from aqueous solution: equilibrium, kinetic and thermodynamic studies. J Chem. 2015;9(3):1457–1480.
- Simonsson D. Reduction of fluoride by reaction with limestone particles in a fixed Bed. Industrial & Engineering Chemistry Process Design & Development. 1979;18(2):288–292.
- Yang M, Hashimoto T, Hoshi N, et al. Fluoride removal in a fixed bed packed with granular calcite. Water Res. 1999;33(33):3395–3402.
- Brunet S, Martin SR, Escobar E, et al. Adsorption and precipitation of tetracycline with struvite. Water Environ Res Res Publ Water Environ Federation. 2007;79(13):2551–2556.
- Abdelrazig BEI, Sharp JH. Phase changes on heating ammonium magnesium phosphate hydrates. Thermochim Acta. 1988;129(2):197–215.
- De-Bashan LE, Bashan Y. Recent advances in removing phosphorus from wastewater and its future use as fertilizer (1997–2003). Water Res. 2004;38(19):4222–4246.
- Rouff AA, Ma N, Kustka AB. Adsorption of arsenic with struvite and hydroxylapatite in phosphate-bearing solutions. Chemosphere. 2015;146:574–581.
- Ma N, Rouff AA. Influence of pH and oxidation state on the interaction of arsenic with struvite during mineral formation. Environ Sci Technol. 2012;46(16):8791–8798.
- Al-Zboon K, Al-Harahsheh MS, Hani FB. Fly ash-based geopolymer for Pb removal from aqueous solution. J Hazard Mater. 2011;188(1–3):414–421.
- Wen D, Zhang F, Zhang E, et al. Arsenic, fluoride and iodine in groundwater of China. J Geochem Explor. 2013;135:1–21.
- Parkhurst DL, Appelo CAJ. Description of input and examples for PHREEQC Version 3--A computer program for speciation, batch-reaction, one-dimensional transport, and inverse geochemical calculations. Center for Integrated Data Analytics Wisconsin Science Center, 2013.
- Hay MB, Stoliker DL, Davis JA, et al. Characterization of the intragranular water regime within subsurface sediments: pore volume, surface area, and mass transfer limitations. Water Resour Res. 2011;47(10). doi:10.1029/2010WR010303.
- Gunawan EK, Warmadewanthi X, Liu J. Removal of phosphate and fluoride from optoelectronic wastewater by calcite. Int J Environ Technol Manage. 2010;12(2–4):308–321.
- Huang PP, Cao CY, Wei F, et al. Mgal layered double hydroxides with chloride and carbonate ions as interlayer anions for removal of arsenic and fluoride ions in water. RSC Adv. 2015;5(14):10412–10417.
- Kameda T, Oba J, Yoshioka T. Recyclable Mg–Al layered double hydroxides for fluoride removal: kinetic and equilibrium studies. J Hazard Mater. 2015;300:475–482.
- Mohapatra M, Anand S, Mishra BK, et al. Review of fluoride removal from drinking water. J Environ Manage. 2009;91(1):67–77.
- Foo KY, Hameed BH. Insights into the modeling of adsorption isotherm systems. Chem Eng J. 2010;156(1):2–10.
- Sousa DNRD, Insa S, Mozeto AA, et al. Equilibrium and kinetic studies of the adsorption of antibiotics from aqueous solutions onto powdered zeolites. Chemosphere. 2018;205:137–146.
- Yu J-G, Yu H-G, Cheng B, et al. The effect of calcination temperature on the surface microstructure and photocatalytic activity of TiO2 thin films prepared by liquid phase deposition. J Phys Chem B. 2003;107(50):13871–13879.