Abstract
Use of clay minerals for removing mercury is an effective technology for the treatment of industrial wastewaters and can become an effective tool for the remediation of coastal ecosystems polluted with this metal. Calcic montmorillonite was employed for adsorbing Hg(II) ions from aqueous solutions at different conditions of salinity (0, 20 and 35 g NaCl L−1), temperature (15, 25 and 35 °C), and initial concentrations (0.25, 0.50, 1.00, 2.50, 5.00 and 10.00 mg Hg L−1). It was observed that 0.4 g dry weight of montmorillonite removed from 0.02 mg g−1 (at 0.25 mg L−1 of Hg(II)) to 0.68 mg g−1 (at 10.0 mg L−1 of Hg(II)) at 25 °C, salinity of 0 g NaCl L−1 and pH of 6. The initial concentration of Hg(II) and salinity had an effect on the behaviour of the adsorption process, which was temperature independent. The data fit well the Freundlich adsorption isotherm, indicating that heterogeneous conditions prevail in this process.
Keywords:
Introduction
Heavy metal pollution due to the release of these elements into the aquatic environment has been a worldwide concern for the last few decades. Mercury is one of the most studied pollutants because of its high toxicity and availability to be accumulated by organisms. This metal is released to the environment from natural sources, which include the degassing from surface water and from the Earth's crust through volcanic eruptions, weathering of mercuriferous areas, lightning‐induced forest fires and biogenic emissions, and from anthropogenic activities, which include mining, metallurgical processes, fossil‐fuel burning, wood pulping, paint and chlor‐alkali industries, battery production and agriculture [Citation1,Citation2]. The input of mercury from all these sources has led to critical, localized mercury‐polluted sites around the world, and the risk to the environment and the health of organisms, including humans, has been increased. Thus, clean‐up technologies for the treatment of huge volumes of water, sediments and soils contaminated with this metal are needed.
Mechanical, chemical and biological methods have been developed for water purification and recovery of mercury from wastewater [Citation3,Citation4,Citation5]. Because of the physical and chemical characteristics of clay minerals, such as large surface area, moderate to high cation exchange capacity and high negative surface charge, these materials can act as concentrators of trace elements in aquatic ecosystems [Citation6], and can play an important role in controlling the background levels of such trace elements in sediments [Citation7]. In addition, other features of these materials include their common occurrence in soils and sediments from most ecosystems and low cost [Citation8]. Hirst [Citation7] suggested that clay minerals concentrate trace elements in the sequence: montmorillonite > vermiculite > illite = chlorite > kaolinite. Frenet‐Robin and Ottmann [Citation9] studied the fixation of inorganic Hg on four different clay minerals and found that Hg fixation on calcic montmorillonite increased when the salinity also increased. Conversely, fixation of this metal on sodic montmorillonite, illite and kaolinite decreased when salinity increased, as they expected. They did not explain the behaviour of the calcic montmorillonite, which was contrary to expectation. Saeed et al. [Citation10] studied the adsorption of Hg(II) in the presence/absence of sodium thiocyanate (NaSCN) onto polyurethane foam and observed that, when salt was present, metal adsorption decreased as pH increase. Conversely, when salt was not present, metal adsorption increased as pH increased. The first behaviour is attributed to the instability of the Hg(II)‐SCN complex at higher pH and the subsequent hydrolysis of metal ions. As a result of this fact, for low pH values (< 5) the adsorption of Hg is higher in the presence of Na, but this trend is the inverse in neutral or alkaline pH.
According to Manohar et al. [Citation5] and Echeverría et al. [Citation11], increasing the temperature from 30 to 60 °C and 5 to 45 °C, respectively, led to an increase in the amount of metals retained on clay minerals. In the present study, calcic montmorillonite, which is considered as a suitable adsorbent for mercury removal, has been used in adsorption experiments in order to learn the effects of the salinity and temperature on the ability of this mineral to trap mercury.
Materials and methods
Untreated calcic montmorillonite (2.2% of CaO and 0.24% of Na2O) was obtained from Fluka (Sigma‐Aldrich Co.). More details of the characteristics (granulometric and chemical composition) of this clay are given in a previous paper [Citation12], Its specific surface area is 117 m2 g−1. This montmorillonite was employed directly for Hg(II) batch adsorption experiments. For each run, 0.4 g of montmorillonite was put into 50 mL polyethylene centrifuge tubes each containing 40 mL of Hg(II) solution at six different initial concentrations (0.25, 0.5, 1.0, 2.5, 5.0 and 10.0 mg L−1) or a mercury‐free control solution, and three different salinities (0, 20 and 35 g NaCl L−1). Then, they were agitated on an oscillating shaker at 150 rpm for a period of 120 min at 15, 25 and 35 °C.
Mercury(II) solutions were prepared from mercuric chloride (HgCl2), Milli‐Q purified water and NaCl (for adjusting the salinity). Initial pH of all the metal solutions (pH = 6) was adjusted with sodium hydroxide. No additional pH measurements were made during the experiment. Samples of 3 ml were taken from each flask at the beginning and the end of the experiments. The samples were centrifuged at 6000 rpm for 10 min and the supernatants were analysed for Hg concentration using a cold vapour mercury analyser, Buck Scientific Model 400A, and SnCl2 as reducing agent. According to Haase et al. [Citation13], a concentration of NaCl of 3.5% (35 g NaCl L−1) in metal solution should have no effect on the determination of Hg concentration by this method. All the material was acid washed [Citation14] prior to use. Metal solutions without montmorillonite were also shaken for 120 min (controls) and no decrease in Hg concentrations in solutions was observed.
Similar adsorption experiments were carried out with mercury‐free saline solution (initial concentration of 0, 20 and 35 g NaCl L−1) and a solution with an initial concentration of 10.0 mg Hg L−1 and 2.9 mg NaCl L−1 (same molar concentration for Hg and Na). The Na concentration was measured after shaking for 120 min. Sodium was determined using a Flame Photometer (Instrumental Laboratory).
Langmuir and Freundlich adsorption isotherms were constructed from the experimental data. For Langmuir isotherms the following equation was used:
where qeq and Ceq correspond to the milligrams of Hg adsorbed per gram of montmorillonite and the equilibrium Hg concentration in the solution (in this case, after 120 min), respectively, and Kd and qm are the Langmuir constant and the maximum adsorption capacity for this model. Freundlich isotherm constants were calculated with the equation:
where KF and n are the Freundlich constants characteristic of the experimental system [Citation15].
All the experiments were conducted in triplicate and the averages of the measurements for each treatment were used. To compare the effects of salinity and temperature on the adsorption of Hg(II), significant differences among means were evaluated by the Tukey HSD test (Statistica 5.5, StatSoft, Inc., 1995–2000).
Results and discussion
Effect of salinity on Hg(II) adsorption
Three values of salinity were used: 0, 20 and 35 g anhydrous sodium chloride L−1. In general terms, the adsorption of Hg(II) decreased in a significant way (Tukey HSD test: p < 0.05) when salt is present (Figure , Table ); however, significant differences between salinities 20 and 35 g L−1 are only detected when the initial concentration of Hg is as high as 10 mg Hg L−1. In addition, for Hg concentrations lower than 5 mg L−1 and salinities of 20 and 35 g L−1, no adsorption of metal was observed. According to Frenet‐Robin and Ottmann [Citation9], mercury does not fix much on clay minerals when the sodium chloride concentration is higher than 5.6 g L−1, because of the formation of the very resistant complex, HgCl4 ‐. Conversely, Celis et al. [Citation8] did not find a significant difference between adsorption of mercury in the presence of up to 0.1 M NaNO3 (8.5 g L−1) and the adsorption measured in the absence of the electrolyte. On the other hand, Echeverría et al. [Citation11] suggested that nickel adsorption on illite, another clay mineral, at below pH 6.8 is via ion exchange with hydrogen and sodium that saturate the exchange sites. A similar process could be occurring in the Hg‐montmorillonite system. At low Hg concentrations, sodium is more competitive and binds to all or most of the adsorption sites, whereas, when the Hg concentration increases, sodium is less competitive leaving some adsorption sites free and available for mercury.
Figure 1 Adsorption of Hg(II) by montmorillonite as a function of initial metal concentration at different salinities and temperatures, and pH = 6.
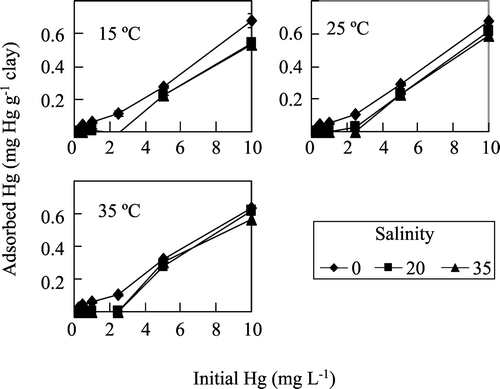
Table 1. Effect of temperature, salinity and initial concentration on Hg(II) adsorption (mean ± standard deviation in %) on Ca‐montmorillonite after 120 min (pH = 6).
Adsorption of Na on Ca‐montmorillonite in the absence of Hg was evaluated and the results are shown in Table . Although the amount of Na adsorbed at the NaCl concentrations of 20 and 30 g L−1 was higher than for a NaCl concentration of 0.0029 g L−1, this amount represents a negligible percentage of the total Na mmoles in solution (0.48 and 0.35%, respectively). Two experiments were performed at a salinity of 0.0029 g L−1 of NaCl, one of them free of Hg and the other one including the same molar concentration of Hg (0.05 mM). When Hg was not present, 90.62% of Na was adsorbed from solution, while in the presence of Hg this percentage goes down to 14.91%. Unfortunately, Ca desorption was not determined in these experiments; however, a preferential mechanism favouring Hg adsorption was in evidence, possibly due to the similar oxidation state of Hg(II) and Ca(II), and the dissimilar oxidation state of Na(I). Finally, Na desorption from the Ca‐montmorillonite was observed when the clay was immersed in a solution free of Hg and Na.
Table 2. Na adsorption means, standard deviation and percentage, with respect to the initial Na concentration, on Ca‐montmorillonite after 120 min (pH = 6).
Effect of temperature on Hg(II) adsorption
The temperature of the adsorption medium could be important for energy‐dependent mechanisms in the metal‐binding process [Citation16]. According to Tsai et al. [Citation17], in a physical exothermic adsorption process the amount of adsorbate adsorbed increases with a decrease in adsorption temperature. Conversely, Manohar et al. [Citation5] observed that increasing the temperature favoured the retention of Hg on 2‐mercaptobenzimidazole‐clay, and mentioned that this process must be endothermic. The adsorption of Hg(II) by the calcic montmorillonite used in these experiments appears to be independent of temperature in the range of 15 to 35 °C (Figure , Table ). Similar results have been reported by other researchers [Citation15,Citation18].
Effect of initial concentration of Hg(II) on its adsorption
The effect of six different initial mercury concentrations on the adsorption of Hg(II) on montmorillonite was evaluated experimentally. The amount of mercury adsorbed per gram of montmorillonite was calculated by the difference between the initial metal concentration (0 min) and the metal concentration after 120 min. This amount ranged from 0.02 mg g−1 for an initial concentration of 0.25 mg L−1 (temperature = 25 °C and salinity = 0) to 0.68 mg g−1 when the concentration of Hg was 10.00 mg L−1 (same temperature and salinity) at the beginning of the experiment (Table ). The observed increase in Hg(II) adsorption as the initial concentration increased (Figures and ) is possibly due to the higher Hg(II)‐ionic‐strength of the more concentrated solutions. Nevertheless, if calculated as the percent of the initial Hg, adsorption decreased from 74 to 56% when the initial Hg(II) concentration increased from 0.25 to 5.00 mg L−1 (Table ). These observations agree with the results obtained by Bajpai [Citation19] for Ni(II) adsorption by illite at different initial metal concentrations.
Langmuir and Freundlich isotherms
There are several isotherm models described in the literature; however, the two most used are the Langmuir and Freundlich models. The Langmuir model is valid for modelling monolayer adsorption onto a homogeneous surface with constant adsorption energy, while the Freundlich equation considers a heterogeneous surface with molecules attached to a surface site influencing neighbouring sites [Citation15]. The adsorption data from the experiments varying the initial Hg(II) concentration were analysed in terms of both Langmuir and Freundlich equations at pH = 6, 25 °C and salinity of 0 g anhydrous sodium chloride L−1 (Figure ). Data from experiments at salinities of 20 and 35 g anhydrous sodium chloride L−1 do not fit any model (r2 < 0.6), and there are no differences between experiments at 15, 25 and 35 °C. The values obtained for the respective model constants are shown in Table . According to the correlation coefficient (r2), used to describe the goodness of fit between the experimental data and the theoretical models, the Freundlich isotherm equation (r2 = 0.89) better described the adsorption process of mercury(II) by montmorillonite, as compared with the Langmuir equation (r2 = 0.15). This fact suggests that heterogeneous conditions (i.e. the heterogeneity of the montmorillonite surface and its internal structure) prevail for monolayer adsorption under the experimental conditions used here. According to Acar and Malkoc [Citation20], if 0.1 < 1/n < 1.0, the adsorption of an adsorbate on the anion exchanger is favourable. In this study, 1/n was 0.778, indicating that montmorillonite can be used effectively for the removal of Hg(II) from aqueous solutions.
Table 3. Langmuir and Freundlich constants for Hg(II) sorption by a Ca‐montmorillonite after 120 min at 25 °C, salinity of 0 and pH = 6.
Comparison with other mineral sorbents
Comparison of the results of this study with those from other studies reported in the literature is difficult because of the different parameters that play a role in the adsorption mechanism, such as inherent characteristics of the adsorbent (surface area, chemical composition, internal and external structure, surface charge capacity), metal affinity, and experimental conditions (pH, temperature, salinity, sampling periods). For instance, Savenko [Citation21] registered 0.006 mg of adsorbed Hg per gram at pH 7.6, a salinity of 21 g L−1, an initial Hg concentration of 0.050 mg L−1 and 2 g L−1 of montmorillonite (Table ), while, in the present study, the amount of adsorbed mercury was 100 times higher (0.650 mg g−1) at pH 6 and a salinity of 20 g L−1, but an initial Hg concentration of 10 mg L−1 (200‐fold) and 10 g L−1 of montmorillonite (five‐fold). However, as indicative information, Table summarises the results from several studies found in the existing literature.
Table 4. Mercury sorption on different clay adsorbents.
Conclusions
In this study, the sorption of Hg(II) depended, in a significant and direct way, on the initial metal concentration in the solutions, and a significant decrease in Hg(II) adsorption was observed when other ions are present (salinity of 20 and 35 g NaCl L−1). According to the Tukey HSD test used here, temperatures between 15 and 35 °C had no significant effect on the adsorption capacity, which is important for the removal of Hg(II) in different climates or processes. The Freundlich adsorption isotherm appeared to fit the data better than the Langmuir model for describing the adsorption behaviour of Hg(II) by montmorillonite, indicating that heterogeneous conditions prevail on monolayer adsorption in this process. The present investigation shows that the calcic clay mineral montmorillonite can be employed as an effective adsorbent for the removal of Hg(II) (up to 74%) from aqueous solutions with concentrations up to 10 mg L−1.
Acknowledgements
The author thanks Denisse Flores‐Hernández, Roberto Rey‐Herrera and Leonor Tripp‐Quezada for their help in the experimental development, and H. Bojórquez‐Leyva for his help in chemical analysis. This research was supported in part by the PAPIIT Program of the DGAPA‐UNAM Grant IN111406.
References
- Morel , F.M.M. , Kraepiel , A.M.L. and Amyot , M. 1998 . The chemical cycle and bioaccumulation of mercury . Annu. Rev. Ecol. Syst. , 29 : 543 – 566 .
- Boening , D.W. 2000 . Ecological effects, transport, and fate of mercury: A general review . Chemosphere , 40 : 1335 – 1351 .
- Wagner‐Döbler , I. , von Canstein , H. , Li , Y. , Timmis , K.N. and Deckwer , W.‐D. 2000 . Removal of mercury from chemical wastewater by microorganisms in technical scale . Environ. Sci. Technol. , 34 : 4628 – 4634 .
- King , J.K. , Harmon , S.M. , Fu , T.T. and Gladden , J.B. 2002 . Mercuty removal, methylmercury formation, and sulfate‐reducing bacteria profiles in wetland mesocosms . Chemosphere , 46 : 859 – 870 .
- Manohar , D.M. , Anoop , K.K. and Anirudhan , T.S. 2002 . Removal of mercury(II) from aqueous solutions and chlor‐alkali industry wastewater using 2‐mercaptobenzimidazole‐clay . Water Res. , 36 : 1609 – 1619 .
- Horowitz , A.J. 1991 . “ A Primer on Sediment‐Trace Element Chemistry ” . Boca Raton, FL : Lewis Publishers .
- Hirst , D. 1962 . The geochemistry of modern sediments from the Gulf of Paria . Geochim. Cosmochim. Acta , 26 : 1147 – 1187 .
- Celis , R. , Hermosín , M.C. and Cornejo , J. 2000 . Heavy metal adsorption by functionalized clays . Environ. Sci. Technol. , 34 : 4593 – 4599 .
- Frenet‐Robin , M. and Ottmann , F. 1978 . Comparative study of the fixation of inorganic mercury on the principal clay minerals and the sediments of the Loire Estuary . Estuar. Coast. Mar. Sci. , 7 : 425 – 436 .
- Saeed , M.M. , Hasany , S.M. and Ahmed , M. 1999 . Adsorption and thermodynamic characteristics of Hg(II)‐SCN complex on to polyurethane foam . Talanta , 50 : 625 – 634 .
- Echeverría , J. , Indurain , J. , Churio , E. and Garrido , J. 2003 . Simultaneous effect of pH, temperature, ionic strength, and initial concentration on the retention of Ni on illite . Colloids Surf. A: Physicochem. Eng. Asp. , 218 : 175 – 187 .
- Green‐Ruiz , C. 2005 . Adsorption of mercury(II) from aqueous solutions by the clay mineral montmorillonite . Bull. Environ. Contam. Toxicol. , 75 : 1137 – 1142 .
- Haase , O. , Klare , M. , Krengel‐Rothensee , K. and Broekaert , J.A.C. 1998 . Evaluation of the determination of mercury at the trace and ultra‐trace levels in the presence of high concentrations of NaCl by flow injection‐cold vapour atomic absorption spectrometry using SnCl2 and NaBH4 as reductands . Analyst , 123 : 1219 – 1222 .
- Moody , J.R. and Lindstrom , R.M. 1977 . Selection and cleaning of plastic containers for storage of trace element samples . Anal. Chem. , 49 : 2264 – 2267 .
- Volesky , B. 1990 . “ Removal and recovery of heavy metals by biosorption ” . In Biosorption of Heavy Metals , Edited by: Volesky , B. 7 – 43 . Boca Raton, FL : CRC Press Inc. .
- Kaçar , Y. , Arpa , Ç. , Tan , S. , Denizli , A. , Genç , Ö. and Arica , M.Y. 2002 . Bioadsorption of Hg(II) and Cd(II) from aqueous solutions: Comparison of biosorptive capacity of alginate and immobilized live and heat inactived Phanerochaete chrysosporium . Process Biochem. , 37 : 601 – 610 .
- Tsai , W.T. , Hsien , K.J. , Chang , Y.M. and Lo , C.C. 2005 . Removal of herbicide paraquat from aqueous solution by adsorption on to spent and treated diatomaceous earth . Bioresour. Techonol. , 96 : 657 – 663 .
- Ting , Y.‐P. and Sun , G. 2000 . Use of polyvinyl alcohol as a cell immobilization matrix for copper biosorption by yeast cells . J. Chem. Technol. Biotechnol. , 75 : 541 – 546 .
- Bajpai , S.K. 1999 . Effect of temperature on removal of Ni(II) from aqueous solutions by adsorption on to fire clay . Asian, J. Chem. , 11 : 171 – 180 .
- Acar , F.N. and Malkoc , E. 2004 . The removal of chromium(VI) from aqueous solutions by Fagus orientalis L . Bioresour. Technol. , 94 : 13 – 15 .
- Savenko , A.V. 2000 . Experimental studying Hg sorption on mineral suspensions in the zone of sea and river water mixing . Water Resour. , 27 : 755 – 758 .
- Sajidu , S.M.I. , Persson , I. , Masamba , W.R.L. , Henry , E.M.T. and Kayambazinthu , D. 2006 . Removal of Cd2+, Cr3+, Cu2+, Hg2+, Pb2+ and Zn2+ cations and AsO4 3‐ anions from aqueous solutions by mixed clay from Tundulu in Malawi and characterisation of the clay . Water SA , 32 : 519 – 526 .