ABSTRACT
Background: Lower limb amputation (LLA) alters the sensorimotor control systems. Despite the self-reports of increased attention during mobility, the interaction between mobility and cognitive control mechanisms is not fully understood.
Objective: Concurrently evaluate walking performance and prefrontal cortical (PFC) activity in persons with and without LLA during different walking conditions.
Methods: Thirty-nine persons with LLA and thirty-three able-bodied controls participated. Walking performance was evaluated using the Figure-of 8-walk-test during three conditions: 1) UW (Usual walking with self-selected walking speed); 2) WCT (walking and carrying a tray with two cups filled with water); and 3) WUT (walking on uneven terrain). PFC activity was assessed using functional near-infrared spectroscopy (fNIRS). Linear mixed models were used to detect changes between groups and between walking conditions within each group.
Results: Between-group comparisons showed increased PFC activity in persons with LLA during UW and WUT, and a significant decrease in walking performance during WCT and WUT compared to controls. Within-group comparisons showed increased PFC activity during WUT compared with UW and WCT and an overall difference in walking performance between the conditions (WU > WUT > WCT) in both groups. However, the effect of walking condition on PFC activity and walking performance was not modified by group (P > .1).
Conclusion: The results suggest that persons with LLA have increased attentional demands during walking but choose the same cognitive-mobility strategy during challenging walking conditions as able-bodied persons. However, the attentional demands seem to depend on the complexity of the task.
Introduction
Persons with a lower limb amputation (LLA) have altered sensorimotor inputs and outputs from and to the prosthetic limb (Knaepen et al., Citation2015). This may challenge prosthetic mobility and have an impact on gait quality and attentional demands, particularly in challenging walking environments (Hafner, Morgan, Abrahamson, and Amtmann, Citation2016b). Many people with LLA have reported increased attention and concentration needs when walking, which might reflect an increased use of cognitive resources during ambulation (Miller, Speechley, and Deathe, Citation2001; Morgan, Hafner, Kartin, and Kelly, Citation2018).
Prior research on LLA recognizes the impact cognitive function has on walking performance (Coffey et al., Citation2012; Frengopoulos et al., Citation2017; Morgan, Hafner, Kartin, and Kelly, Citation2018) and the interaction between cognition and mobility has commonly been assessed using dual-task methods which challenge the attentional capacities (Morgan, Hafner, Kartin, and Kelly, Citation2018; Yogev-Seligmann, Hausdorff, and Giladi, Citation2008). To our knowledge, only a limited number of dual-task studies in persons with LLA exists (Frengopoulos et al., Citation2018a; Hunter et al., Citation2018; Lamoth, Ainsworth, Polomski, and Houdijk, Citation2010; Morgan, Hafner, and Kelly, Citation2016, Citation2017; Pruziner et al., Citation2019) and cognitive tasks like serial subtractions or the Stroop test have been used together with walking tasks. The results indicate that both walking and cognitive performance decreases when adding a concurrent cognitive task in combination with walking (Hunter et al., Citation2018; Morgan, Hafner, and Kelly, Citation2017; Pruziner et al., Citation2019), and a decrease in performance is observed regardless of time since amputation and etiology (Frengopoulos et al., Citation2018a). Studies have also indicated that adding a concurrent cognitive task does not affect walking performance in a different way in persons with lower limb amputation compared with able-bodied controls (Lamoth, Ainsworth, Polomski, and Houdijk, Citation2010; Morgan, Hafner, and Kelly, Citation2016; Pruziner et al., Citation2019). However, a previous study has reported that the gait quality (i.e. increased asymmetry) while dual-task walking on an unstable surface was different between persons with and without transfemoral amputation (Morgan, Hafner, and Kelly, Citation2017). This might indicate that the use of cognitive resources depends on the environmental conditions.
Except for a recent study (Pruziner et al., Citation2019), dual-task studies in persons with LLA have mainly looked at cognitive and mobility performance and not on the underlying cortical activation during the performance. Pruziner et al. (Citation2019) evaluated both gait mechanics (i.e. dual-task walking with a visual task) and cortical activity by electroencephalography (EEG) in a population with and without transtibial amputation and both groups showed overall similar cortical dynamics. Cortical structures play an important role in the control of mobility during daily activities (Hamacher et al., Citation2015). Neuroimaging studies have revealed that several different cortical and subcortical regions are involved during walking (Hamacher et al., Citation2015) and the literature describes two pathways (Bayot et al., Citation2018; Herold et al., Citation2017): 1) a direct locomotor pathway (primary motor cortex, cerebellum and spinal cord); and 2) indirect locomotor pathway (prefrontal cortex (PFC), premotor areas, and basal ganglia). During attentional demanding and challenging walking conditions, the activation of the indirect locomotor pathway increases and the PFC, in particular, plays a key role (Clark, Citation2015; Hamacher et al., Citation2015; Yogev-Seligmann, Hausdorff, and Giladi, Citation2008). According to the capacity sharing theory (Kahneman, Citation1973), the attentional capacity is limited; hence, there might be a risk of attentional overload during challenging mobility conditions.
Several studies have examined PFC activation during dual-task walking using functional near-infrared spectroscopy (fNIRS) (Herold et al., Citation2017; Vitorio et al., Citation2017) and mixed findings have been reported. Several studies report increased PFC activity during dual-task walking when using cognitive tasks (Fraser et al., Citation2016; Holtzer et al., Citation2011; Mirelman et al., Citation2017) or motor tasks (Clark, Rose, Ring, and Porges, Citation2014; Osofundiya, Benden, Dowdy, and Mehta, Citation2016). However, a decreased PFC activation has been observed in dual-task walking with a visual task in older persons (Beurskens, Helmich, Rein, and Bock, Citation2014) and during different complex walking paths in younger persons (Lin and Lin, Citation2016). When comparing different challenging dual tasks, the magnitude of the PFC activation seems to depend on: type of task and its complexity (Clark, Rose, Ring, and Porges, Citation2014); age (Holtzer et al., Citation2011; Lin and Lin, Citation2016; Mirelman et al., Citation2017); and presence of pathology (Maidan et al., Citation2016; Mori, Takeuchi, and Izumi, Citation2018). One recent study on persons with transfemoral amputation during straight-path walking showed increased brain activity in the prefrontal and motor cortex compared to healthy controls (Möller, Rusaw, Hagberg, and Ramstrand, Citation2019). However, knowledge of neuronal processes required during challenging mobility conditions in home and community is still incomplete (Hamacher et al., Citation2015; Pruziner et al., Citation2019). New knowledge about PFC activation during mobility for persons with LLA can help us to gain a better understanding of cognitive and cortical control mechanisms during mobility and may contribute to better planning of rehabilitation strategies. The present study aimed to evaluate PFC activity by fNIRS and walking performance in persons with and without LLA during different challenging walking conditions. Our main hypothesis was that there would be increased PFC activation and reduced walking performance in persons with LLA compared with able-bodied controls during all walking conditions. Secondly, we also hypothesized that the walking conditions would differentially affect PFC activation and/or walking performance in people with LLA compared with able-bodied controls.
Method
The current observational study is part of a larger project examining mobility in persons with LLA. The Regional Committee for Medical and Health Research Ethics in Norway (2015/1245) approved the study which was performed according to the principles of the Declaration of Helsinki. All participants signed an informed consent prior to participation.
The main hypothesis was to investigate between-group comparisons. To identify the minimum sample size, an a priori power analysis was conducted based on walking speed (Wezenberg et al., Citation2013). Power was set to 90%, alpha to .05 and power analysis (by Stata/SE 14.2 for Windows, College Station, TX, USA) estimated to a total sample size of 20. To increase the robustness of the analyses, a sample size of more than 30 persons in each group was considered appropriate.
Participants
Inclusion criteria: 1) 18 years or older; 2) ability to walk without an assistive device for at least 500 m; 3) no psychiatric or neurologic comorbidities; 4) no diagnosis of dementia; and 5) no comorbidities impacting the ability to complete the protocol. For persons with LLA, additional criteria were: 1) unilateral transtibial or transfemoral/knee-disarticulation; 2) non-vascular or non-diabetic etiology of amputation; and 3) walking with a prosthesis for at least 1 year. Participants were recruited from: 1) local prosthetic workshops and rehabilitation centers through flyers; 2) Facebook groups for the two prosthetic user organizations in Norway; and 3) friends, family and peers of the participants.
Baseline cognitive and physical function
Baseline cognitive function was measured by the Montreal Cognitive Assessment (MoCA), which is a tool for screening global cognition (Frengopoulos et al., Citation2017; Frengopoulos, Payne, Viana, and Hunter, Citation2018b; Nasreddine et al., Citation2005) and the Trail Making Test (TMT-A and TMT-B) which is a tool for measuring cognitive flexibility and executive functions (Tombaugh, Citation2004). Persons with LLA also performed the Amputee Mobility Predictor (AMP) (Gailey et al., Citation2002), a performance-based measure of mobility and the Prosthetic Limb Users Survey of Mobility (PLUS-M) (Amtmann et al., Citation2014), a self-report measure of mobility for persons with LLA.
Walking performance
The Figure-of-eight Walk test (F8W) (time and number of steps) was used, which is a valid measure of walking skill and involves straight and curved-path walking and is designed to stimulate more of the complexity of walking in daily life than straight-path walking (Hess, Brach, Piva, and VanSwearingen, Citation2010; Schack, Mirtaheri, Steen, and Gjøvaag, Citation2019). Three different walking conditions were used: 1) UW = Usual walking with self-selected walking speed; 2) WCT = walking and carrying a tray with two cups filled with water; and 3) WUT = walking on uneven terrain. WCT and WUT are known to be challenging mobility conditions for persons with LLA (Hafner, Morgan, Abrahamson, and Amtmann, Citation2016b). The uneven terrain consisted of six foam mats (185 cm long, 60 cm wide and 1.5 cm thick, Airex® Coronella, Airex AG, Switzerland). An additional foam mat of the same material as the six foam mats was cut into slices and these slices were placed underneath the larger mats with eight slices around each of the cones in the F8W (Schack, Mirtaheri, Steen, and Gjøvaag, Citation2019). Before applying the fNIRS equipment, the walking conditions were explained and demonstrated to the participants and they could practice the F8W (one to two laps) in each condition before data collection.
PFC activity
A portable continuous-wave NIRSport system (NIRStar, NIRX Medical Technologies LLC, Glen Head, NY, USA) with two wavelengths (760 nm, 850 nm) was used during the experiments. The system had 16 active optodes; 8 sources and 8 detectors, resulting in 20 channels in total with a sampling frequency of 7.81 Hz. The optodes were placed in a cap (EasyCap, GmbH, Germany) based on the 10–20 international standardized EEG system (Herold, Wiegel, Scholkmann, and Müller, Citation2018) (). The source and detector separation was approximately 3 cm. To ensure the correct position of the measuring cap, the Cz position was used as a reference point and the cap was centered between the nasion and the inion as well as between the left and right preauricular points. The optodes mainly covered the left and right prefrontal cortex representing Broadmann areas (BA) 8, 9 10, 11, 45 and 46 (Morais, Balardin, and Sato, Citation2018; Rorden and Brett, Citation2000) (). A block design with a random order of the three walking conditions was used. Each condition was repeated 5 times and began with a rest period of 60 seconds. The duration of each walking trial was 20 seconds, considering the temporal delay of 2 to 5 seconds in the hemodynamic response (Herold et al., Citation2017). The participants walked in the figure-of-eight-pattern during the trials and the total time duration was used in the subsequent analysis of the signals. The participants returned to the starting position in the figure-of-eight after each trial and rested for 40 seconds. The stimulus presentation software NIRSStim (NIRX Medical Technologies LLC, Glen Head, NY, USA) triggered the onset of each walking trial. Heart rate (HR) was measured simultaneously during the walking trials using SOMNOtouch NIBP (SOMNOmedics, Randersacker, Germany). HR was used as a regressor for filtering the fNIRS signal (Herold, Wiegel, Scholkmann, and Müller, Citation2018; Tachtsidis and Scholkmann, Citation2016).
Figure 1. Illustrates the location of the optode sources and detectors on the scalp. The labels starting with S (S1, S2 … S8)(light gray color) represent the sources. The labels starting with D (D1, D2, …, D8)(dark gray color) represent the detectors. The 20 source-detector pairs called channels are illustrated with bars.
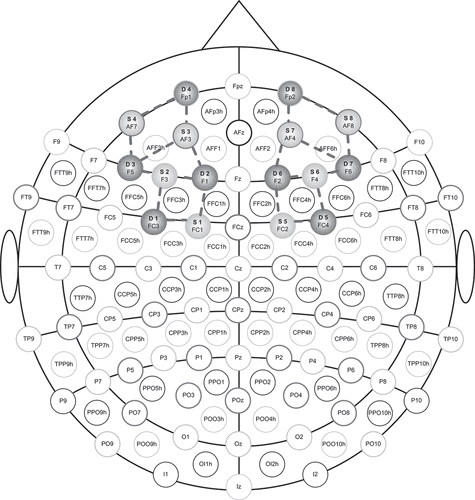
fNIRS data analysis
The nirsLAB v201706 software (https://www.nitrc.org/projects/fnirs_downstate/) was used to analyze the signals. The relative coefficient of variation (CV) was initially calculated for the raw signals for both wavelengths in order to estimate the signal-to-noise quality for each channel (Lu et al., Citation2015). The default CV (7.5%) was used as signal quality control since high standard deviation from unfiltered data might indicate the presence of motion artifact (Morais et al., Citation2017). The differential pathlength factor was calculated according to the participants’ age for both wavelengths (Herold et al., Citation2017). The last 20 seconds of the rest period preceding UW were used as baseline (Herold, Wiegel, Scholkmann, and Müller, Citation2018). The relative changes of oxygenated hemoglobin (OxyHb) and deoxygenated hemoglobin (deoxyHb) were then calculated using the modified Beer–Lambert’s law. In previous studies, it has been reported that OxyHb appears to be a more sensitive and reliable parameter for measuring mobility-dependent changes in cerebral blood flow (Harada, Miyai, Suzuki, and Kubota, Citation2009; Holtzer et al., Citation2011; Miyai et al., Citation2001). Accordingly, we used the changes in OxyHb as a primary outcome for PFC activation.
The general linear model (GLM) as incorporated in nirsLAB was used to analyze the hemodynamic signals. HR measurements were synchronized with the NIRS data and loaded as a user-defined regressor. In addition, the effect of motion artifacts and serial temporal correlation (Tachtsidis and Scholkmann, Citation2016) were reduced via an autoregressive model with the prewhitening iterative reweighted least square algorithms inside the GLM engine (Barker, Rosso, Sparto, and Huppert, Citation2016). The canonical hemodynamic response function (HRF) was used as a base function for the GLM. The GLM model-fitting coefficients for each channel were exported to SPSS for further statistical analysis.
Statistical analyses
Score distributions were evaluated for normality using histograms and Q-Q plots and found normally distributed for all variables. Descriptive statistics with means and standard deviations (SD) were calculated for participants’ demographic and health characteristics. Continuous data were compared using the Student’s t-test and categorical data were compared using the chi-square test. Linear mixed effects models using a random intercept for subject, with group (persons with LLA and able-bodied controls) as between-subject factor, walking conditions (UW, WCT, and WUT) as repeated within-subject factor and interaction terms between group and walking condition were estimated for each of the 20 channels (PFC activation), F8 W time and steps as the dependent variables. We calculated the effect sizes (Cohen’s d) and used the following definition: 0.01 = very small 0.2 = small; 0.5 = medium; 0.8 = large; 1.2 = very large and 2.0 = huge (Sawilowsky, Citation2009). We performed pairwise posthoc tests for all combinations of walking conditions and groups, and Bonferroni adjusted for three multiple tests. Significant group-by-condition interactions would indicate that the walking condition differentially affected PFC activation or walking performance in people with LLA compared with able-bodied controls. To help the interpretation of the data, Pearson’s correlation analysis was conducted to investigate potential relationships between “time since amputation” and age to PFC activation and walking performance. Furthermore, we conducted Pearson’s correlation analysis between PFC activation and mobility scores (PLUS-M and AMP) for persons with LLA.
Results
Participants
Seventy-four persons volunteered to participate in this study. Two persons with LLA were excluded because of technical problems. Thirty-nine persons with LLA and thirty-three able-bodied persons completed the study and their personal characteristics are summarized in . There were no significant differences between the groups in relation to age, sex, height, weight, MoCa score or TMT. The level of amputation was: transtibial (n = 20), transfemoral (n = 11) and knee-disarticulation (n = 8). All persons with LLA wore energy-storing prosthetic feet. Six of the persons with transfemoral amputation/knee-disarticulation used different types of mechanical knees, while the others (13) used microprocessor-controlled prosthetic knees. PLUS-M T-scores for persons with LLA indicate that average mobility for participants with LLA was higher than approximately 80% of people with lower-limb loss.
Table 1. Participant demographics and characteristics
Changes in PFC activation
Between-group comparisons for each condition (UW, WCT, WUT) are shown separately in a, b, c, respectively. A large variability in PFC activation was found across all channels in both groups. The observed results revealed a significant increase in PFC activation during UW (channel 5, p = .039, Cohen’s d = 0.62; channel 18, p = .036, Cohen’s d = 0.58) and WUT (channel 2, p = .042, Cohen’s d = 0.51; channel 4, p = .051, Cohen’s d = 0.51; channel 5, p = .039, Cohen’s d = 0.55; channel 14, p = .009, Cohen’s d = 0.63) in persons with LLA compared with able-bodied controls. No significant differences were observed between groups during WCT (p > .1).
Figure 2. a, b, c. Bar charts of the mean PFC activation (Arbitrary Unit) with 95% confidence intervals (shown as light gray lines) for each of the 20 channels in condition.
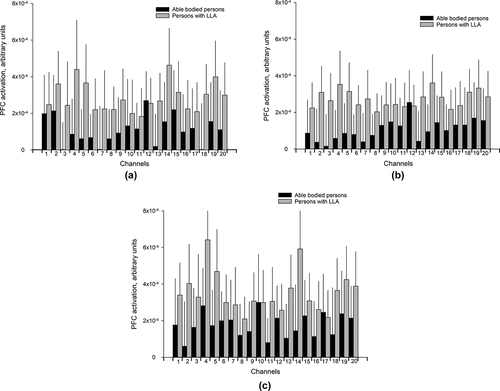
In persons with LLA, within-group comparisons showed a significantly higher PFC activation in WUT compared to UW (channel 4, p = .039, Cohen’s d = 0.44) and WCT (channel 4, p = .003, Cohen’s d = 0.50; channel 5, p = .033, Cohen’s d = 0.45; channel 14, p = .018, Cohen’s d = 0.37), respectively. In the able-bodied controls, the results were similar showing a significant increase in PFC activation during WUT compared to UW (channel 3, p = .039, Cohen’s d = 0.54; channel 6, p = .045, Cohen’s d = 0.43; channel 7, p = .039, Cohen’s d = 0.49) and WCT (channel 4, p = .039, Cohen’s d = 0.74), respectively. Both groups were not significantly different when comparing UW and WCT in all channels. The effect of walking condition on PFC activity was not modified by group, the interaction term group x condition was non-significant in all the statistical models.
Changes in walking performance
shows the results from the F8W performance in each group. Between-group comparisons of the F8W parameters revealed significant differences in both time and number of steps during WCT and WUT, but not in UW. Within-group comparisons revealed significant differences in F8W time (p < .01) and the number of steps (p < 0 .001) in both groups between the three walking conditions, except for F8W steps between UW and WUT for able-bodied controls (p = .105). However, the interaction term group x condition was non-significant (F8W time, p = .108; F8 W steps, p = .073).
Table 2. Figure-of-eight Walk test (F8W) parameters for the participants
Correlation analysis
Age was not significantly correlated with PFC activity in any channels of all three walking conditions in both groups, while age was correlated with F8W time and steps in all conditions in persons with LLA (F8W time (r = 0.48 − 0.57 and p = <0.001 − 0.002); F8W steps (r = 0.41 − 0.53 and p = .001 0.01)) and able-bodied controls (F8 W time (r = 0.47 − 0.54 and p = .001 − 0.006); F8W steps (r = 0.42 − 0.51 and p = .002 − 0.02)), respectively. “Time since amputation” was not significantly correlated with PFC activity or walking performance in any of the walking conditions. For persons with LLA, we found a significant negative correlation between PFC activity during WUT and PLUS-M (ch 10: r = −0.473; p < .011) and AMP (ch 18: r = −0.508, p < .003; ch 20: r = −0.457, p < .011)
Discussion
The aim of the current study was to evaluate the cortical activity and walking performance during different challenging walking conditions in persons with and without LLA. The results partially confirmed our hypothesis. The results from the between-group comparison showed an increased PFC activation with a medium effect size (0.58 < d < 0.62) in persons with LLA compared with able-bodied controls during UW, which was accompanied by a non-significant difference in walking performance. In the context of neural compensation, this suggests that increased levels of brain activity might be necessary to maintain walking performance and support mobility for persons with LLA (Debaere et al., Citation2004; Holtzer et al., Citation2015; Stern, Citation2009). The results suggest that persons with LLA might have less capacity for processing other concurrent cognitive tasks like talking in a mobile phone, due to the assumption that the brain capacity for information processing is limited (Kahneman, Citation1973; Woollacott and Shumway-Cook, Citation2002). This limitation might increase the risk of attentional overload during community mobility and consequently increase the risk of falling (Bayot et al., Citation2018) and reduce participation in social activities (Gallagher, O’Donovan, Doyle, and Desmond, Citation2011; Miller, Deathe, Speechley, and Koval, Citation2001).
It is interesting that the largest increase in PFC activity for persons with LLA, in contrast to able-bodied controls, was observed in channels 4 and 14 (–c) during all three walking conditions. These channels roughly represent the dorsolateral prefrontal area (dlPFC) (BA 9) in the left and right hemispheres, respectively. Although the function of the dlPFC is not fully understood, it appears to be involved in the processing of sensory information and may play a significant role during attention-demanding tasks (Debaere et al., Citation2004; MacDonald, Cohen, Stenger, and Carter, Citation2000). A possible explanation might be that increased levels of sensory information processing in dlPFC might be necessary to support mobility due to the lack of sensorimotor inputs and outputs from and to the prosthetic limb in persons with LLA (Knaepen et al., Citation2015)
The within-group results showed an increased PFC activity during WUT, in comparison with UW, which was accompanied by a significant decrease in walking performance (F8W time) in both groups. Increased activation of the PFC accompanied by a decrease in walking performance might indicate neural inefficiency rather than neural compensation (Stern, Citation2009). The results suggest that the PFC networks assessed in this study, might not be able to adequately support WUT and consequently a reduction in performance occurs. The results indicate that uneven terrain, which is an environmental condition often faced during community mobility, is a demanding activity for both able-bodied persons and persons with LLA (Gallagher, O’Donovan, Doyle, and Desmond, Citation2011; Morgan, Hafner, and Kelly, Citation2017).
The results showed in addition that the difference in PFC activation and walking performance between the groups was not differentially affected by walking conditions (i.e. the interaction term group x condition was non-significant). Several studies have reported similar findings that the addition of a secondary task during walking did not affect persons with LLA in a different way compared with able-bodied controls. (Lamoth, Ainsworth, Polomski, and Houdijk, Citation2010; Morgan, Hafner, and Kelly, Citation2016; Pruziner et al., Citation2019). Although individual differences and large variability in PFC activity are presented in both groups related to how walking conditions are handled, the results in the current study might suggest that persons with LLA in comparison with able-bodied controls use the same cognitive-mobility strategy in order to cope with challenging walking conditions (Pruziner et al., Citation2019; Stern, Citation2009).
Contrary to our hypothesis, between-group comparison of WCT and within-group comparison of UW and WCT showed a non-significant difference in PFC activation. A possible explanation for the results may be due to the “carrying a load” task. This task might be characterized as a complex vision-motor task (Bond and Morris, Citation2000) including both a motor task (“carrying the tray”) and visual tasks (“looking at the cups to avoid spilling any water” with deprivation of visual feedback by “not being able to look at the ground”) (Woollacott and Shumway-Cook, Citation2002). It has been reported that dual-task walking with a visual task might not induce increased activation of PFC in comparison with single-task walking (Beurskens, Helmich, Rein, and Bock, Citation2014). This might explain the non-significant difference in PFC activation between UW and WCT in both groups in the present study. WCT might induce activation in other deeper regions of the brain (Hamacher et al., Citation2015; Yogev-Seligmann, Hausdorff, and Giladi, Citation2008), which is not possible to examine with fNIRS due to the limited penetration depth of the fNIRS signals (Herold et al., Citation2017). The results, therefore, agree with the literature (Clark, Rose, Ring, and Porges, Citation2014; Lin and Lin, Citation2016; Mirelman et al., Citation2014), that the type and complexity of the secondary task influence PFC activation. Within-group analysis revealed that both groups reduced the walking speed and increased the number of steps during WCT compared with UW and WUT. Reducing the gait speed as a compensatory strategy when simultaneously performing a cognitive task has been reported in the literature in both healthy older adults (Yogev-Seligmann, Hausdorff, and Giladi, Citation2008) and persons with LLA (Hunter et al., Citation2018). Since the instruction to focus on “not spilling any water” was given, the participants might not have prioritized the walking performance (Yogev‐Seligmann, Hausdorff, and Giladi, Citation2012). In the current study, walking performance involved curved-path walking (F8W) and increasing the time and number of steps while turning has been reported to increase the risk of falling in persons with LLA (Dite, Connor, and Curtis, Citation2007).
Only a limited number of channels showed significant differences between the groups, although almost all channels during all the walking conditions showed an increase in PFC activation for persons with LLA in comparison with able-bodied controls. The non-significant results could be due to the advanced level of experience of prosthetic users in the current study (Pruziner et al., Citation2019), as the mobility measures (AMP and PLUS-M) showed that persons with LLA had a high mobility level (Gailey et al., Citation2002; Hafner et al., Citation2016a). Prosthetic experience and good mobility might possibly result in lower attentional demands due to motor learning and sensorimotor adjustments that occur during rehabilitation (Geurts et al., Citation1991; Pruziner et al., Citation2019; Yogev‐Seligmann, Hausdorff, and Giladi, Citation2012). The results from the correlation analysis between PFC activity during WUT and the mobility scores (PLUS-M and AMP) showed that high levels of mobility are associated with lower attentional demands during walking. However, the results did not show any significant correlations between “time since amputation” and PFC activity in any of the walking conditions. The explanation might be due to the long experience of most of the participants, as 88% had more than 5 years of experience as prosthetic walkers. An association between PFC activity and “time since amputation” might be observed in prosthetic users with less experience and lower mobility level compared to the participants in the present study.
In addition, power analysis based on PFC activation between groups may have revealed the need of a higher sample size to gain adequate statistical power. The large variability in PFC activation, which is also reported in previous fNIRS studies (de Lima-pardini et al., Citation2017; Perrey, Citation2014; Quaresima et al., Citation2009), might support this notion. Although we did increase the robustness of the analysis by increasing the power to 90% and the sample size to above 30, the study may have had insufficient statistical power to detect differences with respect to the results of PFC activation.
Limitations
The present study consisted of relatively active persons with LLA of non-diabetic or non-vascular etiology. They all walked without an assistive device, which might limit the generalizability of the results. The sample might not be representative of all prosthetic users, especially those with amputation due to vascular causes or persons with a lower mobility level (e.g. due to older age or comorbidities). In addition, there was a large age range of participants in both groups, but there was no significant difference between the groups related to age. Baseline cognitive function was only assessed using MoCA and TMT (A and B) which are screening tools to assess cognition and therefore might provide limited information about cognition. Spatio-temporal parameters of gait were not measured and might have provided additional information about walking performance and the quality of gait (Holtzer et al., Citation2015; Morgan, Hafner, and Kelly, Citation2017). During the preprocessing of fNIRS signals, we controlled for heart rate, but not for other systemic changes like blood pressure or respiratory rate, which might have changed during the walking conditions. Thus, we cannot exclude the influence of these confounding factors (Tachtsidis and Scholkmann, Citation2016). Furthermore, we used a laboratory setting for the examination, which does not replicate ecologic environmental dimensions and might have influenced the cognitive processes.
Clinical implications
The results of the present study indicate the importance of addressing walking limitations in the context of environmental challenges that persons with LLA face in their daily lives. Developing rehabilitation interventions for persons with LLA with a focus on dual-task abilities in complex environments might reduce the attentional demands over time and improve safety, increase community participation and increase quality of life.
Conclusion
Persons with LLA showed an increased PFC activation compared with able-bodied persons during UW and WUT. The activation of PFC seems to depend on the nature and complexity of the task and was not significantly different between groups in WCT. Persons with LLA had reduced walking performance during WCT and WUT compared to able-bodied controls. However, the challenging walking conditions did not affect PFC activation and walking performance in a different way in persons with LLA compared with able-bodied controls. This suggests that persons with LLA have increased attentional demands during complex walking but use the same cognitive-mobility strategy during challenging walking conditions as able-bodied persons.
Acknowledgments
This work was supported by the foundation “Stiftelsen Sophies Minde” Oslo, Norway (1203). ISPO (International Society for Prosthetics and Orthotics) Norway provided a grant for some of the data collection expenses. The authors would like to thank Lamija Pasalic, NIRX for her support with data analysis.
Disclosure Statement
The authors declare no conflicts of interest.
Additional information
Funding
References
- Amtmann D, Abrahamson DC, Morgan S, Salem R, Askew RL, Gailey R, Gaunaurd I, Kajlich A, Hafner B 2014 The PLUS-M: Item bank of mobility for prosthetic limb users. Quality of Life 23: 39–40.
- Barker JW, Rosso AL, Sparto PJ, Huppert TJ 2016 Correction of motion artifacts and serial correlations for real-time functional near-infrared spectroscopy. Neurophotonics 3: 0314100. doi:https://doi.org/10.1117/1.NPh.3.3.031410.
- Bayot M, Dujardin K, Tard C, Defebvre L, Bonnet CT, Allart E, Delval A 2018 The interaction between cognition and motor control: A theoretical framework for dual-task interference effects on posture, gait initiation, gait and turning. Neurophysiologie Clinique 48: 361–375. doi:https://doi.org/10.1016/j.neucli.2018.10.003.
- Beurskens R, Helmich I, Rein R, Bock O 2014 Age-related changes in prefrontal activity during walking in dual-task situations: A fNIRS study. International Journal of Psychophysiology 92: 122–128. doi:https://doi.org/10.1016/j.ijpsycho.2014.03.005.
- Bond JM, Morris M 2000 Goal-directed secondary motor tasks: Their effects on gait in subjects with Parkinson disease. Archives of Physical Medicine and Rehabilitation 81: 110–116. doi:https://doi.org/10.1016/S0003-9993(00)90230-2.
- Clark DJ 2015 Automaticity of walking: Functional significance, mechanisms, measurement and rehabilitation strategies. Frontiers in Human Neuroscience 9: 246. doi:https://doi.org/10.3389/fnhum.2015.00246.
- Clark DJ, Rose DK, Ring SA, Porges EC 2014 Utilization of central nervous system resources for preparation and performance of complex walking tasks in older adults. Frontiers in Aging Neuroscience 6: 217. doi:https://doi.org/10.3389/fnagi.2014.00217.
- Coffey L, O’Keeffe F, Gallagher P, Desmond D, Lombard-Vance R 2012 Cognitive functioning in persons with lower limb amputations: A review. Disability and Rehabilitation 34: 1950–1964. doi:https://doi.org/10.3109/09638288.2012.667190.
- de Lima-pardini AC, Zimeo Morais GA, Balardin JB, Coelho DB, Azzi NM, Teixeira LA, Sato JR 2017 Measuring cortical motor hemodynamics during assisted stepping - An fNIRS feasibility study of using a walker. Gait & Posture 56: 112–118. doi:https://doi.org/10.1016/j.gaitpost.2017.05.018.
- Debaere F, Wenderoth N, Sunaert S, Van Hecke P, Swinnen S 2004 Changes in brain activation during the acquisition of a new bimanual coordination task. Neuropsychologia 42: 855–867. doi:https://doi.org/10.1016/j.neuropsychologia.2003.12.010.
- Dite W, Connor HJ, Curtis HC 2007 Clinical identification of multiple fall risk early after unilateral transtibial amputation. Archives of Physical Medicine and Rehabilitation 88: 109–114. doi:https://doi.org/10.1016/j.apmr.2006.10.015.
- Fraser SA, Dupuy O, Pouliot P, Lesage F, Bherer L 2016 Comparable cerebral oxygenation patterns in younger and older adults during dual-task walking with increasing load. Frontiers in Aging Neuroscience 8: 240. doi:https://doi.org/10.3389/fnagi.2016.00240.
- Frengopoulos C, Burley J, Viana R, Payne MW, Hunter SW 2017 Association between Montreal cognitive assessment scores and measures of functional mobility in lower extremity amputees after inpatient rehabilitation. Archives of Physical Medicine and Rehabilitation 98: 450–455. doi:https://doi.org/10.1016/j.apmr.2016.06.012.
- Frengopoulos C, Payne MW, Holmes JD, Viana R, Hunter SW 2018a Comparing the effects of dual-task gait testing in new and established ambulators with lower extremity amputations. Pm & R 10: 1012–1019. doi:https://doi.org/10.1016/j.pmrj.2018.03.018.
- Frengopoulos C, Payne MW, Viana R, Hunter SW 2018b MoCA domain score analysis and relation to mobility outcomes in dysvascular lower extremity amputees. Archives of Physical Medicine and Rehabilitation 99: 314–320. doi:https://doi.org/10.1016/j.apmr.2017.09.003.
- Gailey RS, Roach KE, Applegate EB, Cho B, Cunniffe B, Licht S, Maguire M, Nash MS 2002 The amputee mobility predictor: An instrument to assess determinants of the lower-limb amputee’s ability to ambulate. Archives of Physical Medicine and Rehabilitation 83: 613–627. doi:https://doi.org/10.1053/apmr.2002.32309.
- Gallagher P, O’Donovan MA, Doyle A, Desmond D 2011 Environmental barriers, activity limitations and participation restrictions experienced by people with major limb amputation. Prosthetics and Orthotics International 35: 278–284. doi:https://doi.org/10.1177/0309364611407108.
- Geurts et al. 1991 Dual-task assessment of reorganization of postural control in persons with lower limb amputation. Arch Phys Med Rehabil 72: 1059–1064
- Hafner BJ, Gaunaurd IA, Morgan SJ, Amtmann D, Salem R, Gailey RS 2016a Construct validity of the prosthetic limb users survey of mobility (PLUS-M) in adults with lower limb amputation. Archives of Physical Medicine and Rehabilitation 98: 277–285. doi:https://doi.org/10.1016/j.apmr.2016.07.026.
- Hafner BJ, Morgan SJ, Abrahamson DC, Amtmann D 2016b Characterizing mobility from the prosthetic limb user’s perspective: Use of focus groups to guide development of the Prosthetic Limb users survey of mobility. Prosthetics and Orthotics International 40: 582–590. doi:https://doi.org/10.1177/0309364615579315.
- Hamacher D, Herold F, Wiegel P, Hamacher D, Schega L 2015 Brain activity during walking: A systematic review. Neuroscience and Biobehavioral Reviews 57: 310–327. doi:https://doi.org/10.1016/j.neubiorev.2015.08.002.
- Harada T, Miyai I, Suzuki M, Kubota K 2009 Gait capacity affects cortical activation patterns related to speed control in the elderly. Experimental Brain Research 193: 445–454. doi:https://doi.org/10.1007/s00221-008-1643-y.
- Herold F, Wiegel P, Scholkmann F, Müller N 2018 Applications of functional near-infrared spectroscopy (fNIRS) neuroimaging in exercise–cognition science: A systematic, methodology-focused review. Journal of Clinical Medicine 7: 466. doi:https://doi.org/10.3390/jcm7120466.
- Herold F, Wiegel P, Scholkmann F, Thiers A, Hamacher D, Schega L 2017 Functional near-infrared spectroscopy in movement science: A systematic review on cortical activity in postural and walking tasks. Neurophotonics 4: 041403. doi:https://doi.org/10.1117/1.NPh.4.4.041403.
- Hess RJ, Brach JS, Piva SR, VanSwearingen JM 2010 Walking skill can be assessed in older adults: Validity of the figure-of-8 walk test. Physical Therapy 90: 89–99. doi:https://doi.org/10.2522/ptj.20080121.
- Holtzer R, Mahoney JR, Izzetoglu M, Izzetoglu K, Onaral B, Verghese J 2011 fNIRS study of walking and walking while talking in young and old individuals. Journals of Gerontology. Series A, Biological Sciences and Medical Sciences 66: 879–887. doi:https://doi.org/10.1093/gerona/glr068.
- Holtzer R, Mahoney JR, Izzetoglu M, Wang C, England S, Verghese J 2015 Online fronto-cortical control of simple and attention-demanding locomotion in humans. Neuroimage 112: 152–159. doi:https://doi.org/10.1016/j.neuroimage.2015.03.002.
- Hunter SW, Frengopoulos C, Holmes J, Viana R, Payne MW 2018 Dual-task related gait changes in individuals with trans-tibial lower extremity amputation. Gait & Posture 61: 403–407. doi:https://doi.org/10.1016/j.gaitpost.2018.02.012.
- Kahneman D 1973 Attention and Effort. Englewood Cliffs, New Jersey: Prentice-Hall.
- Knaepen K, Marusic U, Crea S, Rodríguez Guerrero CD, Vitiello N, Pattyn N, Mairesse O, Lefeber D, Meeusen R 2015 Psychophysiological response to cognitive workload during symmetrical, asymmetrical and dual-task walking. Human Movement Science 40: 248–263. doi:https://doi.org/10.1016/j.humov.2015.01.001.
- Lamoth CJ, Ainsworth E, Polomski W, Houdijk H 2010 Variability and stability analysis of walking of transfemoral amputees. Medical Engineering & Physics 32: 1009–1014. doi:https://doi.org/10.1016/j.medengphy.2010.07.001.
- Lin M, Lin KH 2016 Walking while performing working memory tasks changes the prefrontal cortex hemodynamic activations and gait kinematics. Frontiers in Behavioral Neuroscience 10: 92. doi:https://doi.org/10.3389/fnbeh.2016.00092.
- Lu CF, Liu YC, Yang YR, Wu YT, Wang RY 2015 Maintaining gait performance by cortical activation during dual-task interference: A functional near-infrared spectroscopy study. PloS One 10: e0129390. doi:https://doi.org/10.1371/journal.pone.0129390.
- MacDonald AW, Cohen JD, Stenger VA, Carter CS 2000 Dissociating the role of the dorsolateral prefrontal and anterior cingulate cortex in cognitive control. Science 88: 1835–1838. doi:https://doi.org/10.1126/science.288.5472.1835.
- Maidan I, Nieuwhof F, Bernad-Elazari H, Reelick M, Bloem BR, Giladi N, Deutsch JE, Hausdorff JM, Claassen JA, Mirelman A 2016 The role of the frontal lobe in complex walking among patients with Parkinson’s disease and healthy older adults: An fNIRS study. Neurorehabilitation and Neural Repair 30: 963–971. doi:https://doi.org/10.1177/1545968316650426.
- Miller WC, Deathe AB, Speechley M, Koval J 2001 The influence of falling, fear of falling, and balance confidence on prosthetic mobility and social activity among individuals with a lower extremity amputation. Archives of Physical Medicine and Rehabilitation 82: 1238–1244. doi:https://doi.org/10.1053/apmr.2001.25079.
- Miller WC, Speechley M, Deathe B 2001 The prevalence and risk factors of falling and fear of falling among lower extremity amputees. Archives of Physical Medicine and Rehabilitation 82: 1031–1037. doi:https://doi.org/10.1053/apmr.2001.24295.
- Mirelman A, Maidan I, Bernad-Elazari H, Nieuwhof F, Reelick M, Giladi N, Hausdorff JM 2014 Increased frontal brain activation during walking while dual tasking: An fNIRS study in healthy young adults. Journal of Neuroengineering and Rehabilitation 11: 85. doi:https://doi.org/10.1186/1743-0003-11-85.
- Mirelman A, Maidan I, Bernad-Elazari H, Shustack S, Giladi N, Hausdorff JM 2017 Effects of aging on prefrontal brain activation during challenging walking conditions. Brain and Cognition 115: 41–46. doi:https://doi.org/10.1016/j.bandc.2017.04.002.
- Miyai I, Tanabe HC, Sase I, Eda H, Oda I, Konishi I, Tsunazawa Y, Suzuki T, Yanagida T, Kubota K 2001 Cortical mapping of gait in humans: A near-infrared spectroscopic topography study. Neuroimage 14: 1186–1192. doi:https://doi.org/10.1006/nimg.2001.0905.
- Möller S, Rusaw D, Hagberg K, Ramstrand N 2019 Reduced cortical brain activity with the use of microprocessor-controlled prosthetic knees during walking. Prosthetics and Orthotics International 43: 257–265. doi:https://doi.org/10.1177/0309364618805260.
- Morais ZG, Balardin JB, Sato JR 2018 fNIRS Optodes’ Location Decider (fOLD): A toolbox for probe arrangement guided by brain regions-of-interest. Scientific Reports 8: 3341. doi:https://doi.org/10.1038/s41598-018-21716-z.
- Morais ZG, Scholkmann F, Balardin JB, Furucho RA, de Paula RC, Biazoli CE, Sato JR 2017 Non-neuronal evoked and spontaneous hemodynamic changes in the anterior temporal region of the human head may lead to misinterpretations of functional near-infrared spectroscopy signals. Neurophotonics 5: 011002.
- Morgan SJ, Hafner BJ, Kartin D, Kelly VE 2018 Dual-task standing and walking in people with lower limb amputation: A structured review. Prosthetics and Orthotics International 42: 652–666. doi:https://doi.org/10.1177/0309364618785728.
- Morgan SJ, Hafner BJ, Kelly VE 2016 The effects of a concurrent task on walking in persons with transfemoral amputation compared to persons without limb loss. Prosthetics and Orthotics International 40: 490–496. doi:https://doi.org/10.1177/0309364615596066.
- Morgan SJ, Hafner BJ, Kelly VE 2017 Dual-task walking over a compliant foam surface: A comparison of people with transfemoral amputation and controls. Gait & Posture 58: 41–45. doi:https://doi.org/10.1016/j.gaitpost.2017.07.033.
- Mori T, Takeuchi N, Izumi SI 2018 Prefrontal cortex activation during a dual task in patients with stroke. Gait & Posture 59: 193–198. doi:https://doi.org/10.1016/j.gaitpost.2017.09.032.
- Nasreddine ZS, Phillips NA, Bédirian V, Charbonneau S, Whitehead V, Collin I, Cummings JL, Chertkow H 2005 The Montreal Cognitive Assessment, MoCA: A brief screening tool for mild cognitive impairment. Journal of the American Geriatrics Society 53: 695–699. doi:https://doi.org/10.1111/j.1532-5415.2005.53221.x.
- Osofundiya O, Benden ME, Dowdy D, Mehta RK 2016 Obesity-specific neural cost of maintaining gait performance under complex conditions in community-dwelling older adults. Clinical Biomechanics 35: 42–48. doi:https://doi.org/10.1016/j.clinbiomech.2016.03.011.
- Perrey S 2014 Possibilities for examining the neural control of gait in humans with fNIRS. Frontiers in Physiology 5: 204. doi:https://doi.org/10.3389/fphys.2014.00204.
- Pruziner AL, Shaw EP, Rietschel JC, Hendershot BD, Miller MW, Wolf EJ, Hatfield BD, Dearth CL, Gentili RJ 2019 Biomechanical and neurocognitive performance outcomes of walking with transtibial limb loss while challenged by a concurrent task. Experimental Brain Research 237: 477–491. doi:https://doi.org/10.1007/s00221-018-5419-8.
- Quaresima V, Giosuè P, Roncone R, Casacchia M, Ferrari M 2009 Prefrontal cortex dysfunction during cognitive tests evidenced by functional near-infrared spectroscopy. Psychiatry Research Neuroimaging 171: 252–257. doi:https://doi.org/10.1016/j.pscychresns.2008.02.002.
- Rorden C, Brett M 2000 Stereotaxic display of brain lesions. Behavioural Neurology 12: 191–200. doi:https://doi.org/10.1155/2000/421719.
- Sawilowsky SS 2009 New effect size rules of thumb. Journal of Modern Applied Statistical Methods 8: 26. doi:https://doi.org/10.22237/jmasm/1257035100.
- Schack J, Mirtaheri P, Steen H, Gjøvaag T 2019 Assessing mobility for persons with lower limb amputation: The Figure-of-Eight Walk Test with the inclusion of two novel conditions. Disability and Rehabilitation [Epub ahead of print]. doi:https://doi.org/10.1080/09638288.2019.1662495.
- Stern Y 2009 Cognitive reserve. Neuropsychologia 47: 2015–2028. doi:https://doi.org/10.1016/j.neuropsychologia.2009.03.004.
- Tachtsidis I, Scholkmann F 2016 False positives and false negatives in functional near-infrared spectroscopy: Issues, challenges, and the way forward. Neurophotonics 3: 030401. doi:https://doi.org/10.1117/1.NPh.3.3.030401.
- Tombaugh TN 2004 Trail Making Test A and B: Normative data stratified by age and education. Archives of Clinical Neuropsychology 19: 203–214. doi:https://doi.org/10.1016/S0887-6177(03)00039-8.
- Vitorio R, Stuart S, Rochester L, Alcock L, Pantall A 2017 fNIRS response during walking - Artifact or cortical activity? A systematic review. Neuroscience and Biobehavioral Reviews 83: 160–172. doi:https://doi.org/10.1016/j.neubiorev.2017.10.002.
- Wezenberg D, van der Woude LH, Faber WX, de Haan A, Houdijk H 2013 Relation between aerobic capacity and walking ability in older adults with a lower-limb amputation. Archives Of Physical Medicine And Rehabilitation 94: 1714–1720. doi:https://doi.org/10.1016/j.apmr.2013.02.016.
- Woollacott M, Shumway-Cook A 2002 Attention and the control of posture and gait: A review of an emerging area of research. Gait & Posture 16: 1–14. doi:https://doi.org/10.1016/S0966-6362(01)00156-4.
- Yogev-Seligmann G, Hausdorff JM, Giladi N 2008 The role of executive function and attention in gait. Movement Disorders 23: 329–342. doi:https://doi.org/10.1002/mds.21720.
- Yogev‐Seligmann G, Hausdorff JM, Giladi N 2012 Do we always prioritize balance when walking? Towards an integrated model of task prioritization. Movement Disorders 27: 765–770. doi:https://doi.org/10.1002/mds.24963.