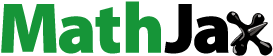
ABSTRACT
Memory for object locations in proximity is an essential aspect of everyday activities and has high clinical relevance. Immersive Virtual Reality (VR) has the potential to bridge the gap between the experimental control of laboratory conditions and reliable predictions of functional abilities. We developed the immersive Virtual Memory Task (imVMT), a neuropsychological assessment tool for spatial memory that combines gesture-based natural hand interaction and a head-mounted display. Our aim was to investigate the imVMT task characteristics, to validate the imVMT regarding the underlying cognitive processes, and to evaluate its applicability and feasibility in the clinical context in a sample of thirty-five patients with various neurological disorders and functional deficits. We further aimed to assess object-location memory in a small-scale virtual environment. Our results show that task difficulty can be efficiently manipulated by increasing the number of objects to memorize and rotation angle against the encoding perspective. We demonstrate construct validity as our primary outcome measure was significantly related to spatial memory performance in conventional neuropsychological tests. The imVMT was highly feasible and received high ratings for usability. The imVMT represents an ecologically relevant and valid VR task for assessing object-location memory in a wide range of neurological patients.
Trial registration
German Clinical Trials Register identifier: DRKS00024005.
Introduction
Recalling the location of objects in proximity is an essential aspect of everyday activities, such as finding items in the household or in a grocery store. Spatial memory refers to the ability to encode, store, and retrieve information about spatial locations, compositions, and routes relevant for object location retrieval and navigation (De Renzi et al., Citation1977; Postma et al., Citation2004; Postma & De Haan, Citation1996). Investigating object-location memory (i.e., object-to-location associations) in small-scale spaces is of high functional relevance, as impairments in this domain frequently occur in age-related cognitive decline (Cherry et al., Citation1993; Pertzov et al., Citation2012) and are associated with early symptoms in mild cognitive impairment and Alzheimer's disease (McShane et al., Citation1998; Monacelli et al., Citation2003; Rowe et al., Citation2011).
Spatial cognition is important for everyday activities and involves many spatial abilities, such as visuospatial perception to process object locations in space, mental imagery required for mental rotation, and spatial memory (Burgess, Citation2006; Klencklen, Després, & Dufour, Citation2012). Spatial memory is closely intertwined with several cognitive processes required to retrieve object locations (Ruggiero et al., Citation2008). Thus, working memory (Magen & Emmanouil, Citation2019; Zimmer et al., Citation2003), attention (Barel, Citation2019; Siegel & Castel, Citation2018), and short – and long-term memory (Lavenex et al., Citation2015; Morris & Mayes, Citation2004) are involved in the retention and retrieval of objects and their interrelationships.
Conventional neuropsychological assessment of spatial memory
Despite its high relevance for everyday life, testing and quantifying spatial memory has been challenging. Conventional, particularly paper-pencil-based, neuropsychological tests of spatial memory are static, two-dimensional, and thus do not fully capture the multi-faceted nature of spatial memory in the real world (for a review, see Diaz-Orueta et al., Citation2022; Howieson, Citation2019). Typical examples include the Rey-Osterrieth Complex Figure Test (ROCF; Osterrieth, Citation1944; Rey, Citation1941), in which different objects are integrated into a complex figure and have to be copied and memorized. The Cognitive Map Task of the Visual and Verbal Memory Test (VVM; Schellig & Schächtele, Citation2009), a German memory test, requires memorizing and recalling a route on a map from a given point A to a given point B. In both tasks, the presentation is two-dimensional and therefore unlikely to represent the three-dimensional spatial processing required for object localization or spatial navigation. The Route Learning Task (Wilson et al., Citation2008) applies a route in the real world and therefore succeeds in representing the three-dimensional world. However, it consists of only five positions in a small room, and is therefore relatively simple, leading to ceiling effects. Another option, the Recurrent Figures test (Kimura, Citation1963), which requires the recognition of a series of figures and their discrimination from similar ones, uses very abstract items and therefore has little relevance to everyday tasks. This overview shows that conventional neuropsychological tests cannot measure spatial memory in a way that is comparable to the demands of the natural world. Therefore, when designing spatial memory tasks that aim to represent the complexities and challenges of everyday life, it is important to use three-dimensional environments and objects with spatial features.
Potential of Virtual Reality for the assessment of spatial memory
In recent years, immersive Virtual Reality (VR), the real-time presentation of computer-generated, three-dimensional, and viewpoint-adapted content delivered through stereoscopic head-mounted displays (HMDs), has received much attention in cognitive (Hofmann et al., Citation2021) and clinical neuroscience (Krohn et al., Citation2020). While non-immersive VR (on a desktop screen) has a limited field of view, immersive VR (on an HMD) embeds the user in the virtual and separates them from the physical world. HMDs can simulate virtual environments from a first-person perspective, are intuitive, naturalistic to use, and do not require prior gaming experience. Due to the potential to enhance attention in immersive VR (Cho et al., Citation2002), the likelihood of presence (i.e., the sense of being there) increases (Chirico et al., Citation2016; Riva, Citation2022). Immersive VR provides powerful alternatives to bridge the gap between laboratory conditions and promises increased ecological relevance (Krohn et al., Citation2020), that means to reliably predict functional abilities from laboratory-based assessments (Rose et al., Citation2005). VR allows to measure human behaviour in multiple dimensions (e.g., movement patterns, gaze direction) and to draw conclusions about underlying cognitive functions or processes with high spatial and temporal resolution and provides for high experimental control (Freeman et al., Citation2017; Riva et al., Citation2019; Rizzo et al., Citation2004). In addition, spatial cognitive tests that were previously difficult to perform can now be simulated in a standardized way, accurately measured and immediately evaluated. For instance, performance can be measured after manipulating objects in space, rather than relying on mental representations of objects. Thus, immersive VR has the potential to enhance both the extent to which the cognitive demands of an assessment instrument resemble those of a real-world task and the extent to which tests are empirically related to everyday life functioning (Chaytor & Schmitter-Edgecombe, Citation2003; Spooner & Pachana, Citation2006).
Given its potential to sensitively detect cognitive impairment (Jonson et al., Citation2021; Neguț et al., Citation2016) and enable three-dimensional depth perception, VR has been proposed as a valuable tool to investigate spatial memory (Astur et al., Citation2004; Diersch & Wolbers, Citation2019; Faria et al., Citation2016). Both non-immersive (Montana et al., Citation2019) and immersive VR tasks (Cogné et al., Citation2017) have been applied to investigate spatial memory performance for wayfinding in large-scale spaces. Consequently, there is a large body of literature on virtual cities (e.g., Plancher et al., Citation2008; van der Ham et al., Citation2010; Weniger et al., Citation2011), buildings (e.g., van der Ham et al., Citation2015; White & Moussavi, Citation2016) and supermarkets (e.g., Plechatá et al., Citation2019; Rand et al., Citation2007) that serve as experimental virtual environments. In addition to such larger-scale spaces, everyday life functions involve small-scale spaces. Therefore, investigating the processing of object retrieval in proximity is needed.
Challenges in applying VR in neuropsychological rehabilitation
Despite its increasing application in neurological rehabilitation, VR technology has rarely been applied to measure spatial memory in clinical populations, so far. For instance, Fernandez Montenegro and Argyriou (Citation2017) developed a virtual Alzheimer's screening test in which patients had to indicate the location of an object previously seen on a virtual table. However, object names were also presented during retrieval, potentially leading to interference and less ecologically relevant results. Immediate memory traces of object locations may have been maintained in spatial working memory (Awh et al., Citation1998; Baddeley, Citation1995), and object names may have activated spatial associations due to the spatial interference effects (Estes et al., Citation2015). Parsons and Rizzo (Citation2008) found moderate correlations between conventional memory tests and a VR memory score within an immersive virtual city. However, their study had a small sample size, did not include a clinical population, and used outdated (i.e., relatively low-quality) hardware, which may have affected the reliability of the results. Kourtesis et al. (Citation2021) applied naturalistic, daily tasks to assess everyday cognitive functions in settings resembling real-life challenges. Their virtual test battery, however, primarily assessed cognitive functions other than object-location memory (e.g., prospective memory, visuospatial attention) and was also not applied to clinical populations.
A major challenge in assessing clinical populations with VR is to ensure that the interaction with the virtual environment is naturalistic and intuitive. Artificial forms of interaction (e.g., controllers, computer mouse) may elicit additional cognitive load or motor challenges that may interfere with task performance and disturb immersion in the task environment (Bellgardt et al., Citation2020).
Development of the immersive Virtual Memory Task (imVMT)
In line with previous research, our aim was to develop a memory task suitable for investigating spatial memory in neurological patients in a small-scale virtual environment. To ensure the quality of the task, we established the multidimensional evaluation criteria for clinical VR applications outlined by Krohn et al. (Citation2020) in parallel with the actual development process of the imVMT. Later, Kourtesis and MacPherson (Citation2021) provided methodological guidelines for enhancing neuropsychological assessment using immersive VR, which the imVMT follows. Our aims were to use a realistic task and environment, to implement a small-scale virtual environment to minimize the risk of adverse effects, and to create a task that was highly appropriate for neurological patients. The small-scale spatial memory task was chosen for its practicality and plausibility, enabling the presentation and evaluation of hypothetical scenarios that were not previously feasible in a clinical context. This task offers several practical advantages, including reduced effort and time for clinicians, as no real objects are needed. This results in less time for test administration and scoring, increased flexibility, and immediate feedback on performance. To improve usability, we incorporated infrared hand tracking with online gesture recognition, thus enabling a naturalistic, controller-free interaction. The software we developed focused on maximizing patient feasibility, minimizing side effects (e.g., no movement, standing or sitting possible), VR-induced side effects (VRISE), also known as cybersickness in VR (Caserman et al., Citation2021), and using objective quantification (object and user position sampling).
Study aims
The aim of the presented study was threefold: (1) to investigate the imVMT task characteristics, particularly the effect of difficulty parameters on task performance, (2) to validate the imVMT in terms of underlying cognitive processes, and (3) to evaluate the applicability and feasibility of the imVMT in a clinical context with neurological patients. Therefore, we evaluated the effect of the imVMT difficulty parameters, namely number of objects and rotation angle, on the outcome measures (mean distance, placement time) to provide empirical evidence and understanding of their effects on performance. Our primary objective was to examine the construct validity of the imVMT in a convenience sample of neurological patients using conventional paper-and-pencil neuropsychological tests of spatial memory. Finally, we aimed to assess the feasibility and usability of the imVMT in the clinical setting, particularly with regard to bare-hand gesture-based interaction and cybersickness, by administering the VR task in a sample of patients with various sensorimotor and cognitive disorders and little experience with traditional game controllers.
Materials and methods
Immersive Virtual Memory Task
Based on our recently proposed systematic framework for clinical VR applications (Krohn et al., Citation2020), we identified the non-immersive virtual memory task by Koenig et al. (Citation2011) as a promising basis for the development of a fully immersive clinical object-location memory task. Koenig et al. (Citation2011) applied their task in a heterogeneous sample of neurological patients and healthy adults and found a significant correlation with established neuropsychological tests for spatial memory and perspective taking. The newly developed immersive Virtual Memory Task (imVMT) requires participants (1) to memorize the location of everyday objects displayed on a table and, after the objects have been removed or reshuffled, (2) to re-arrange the object constellation on the table during recall. The virtual objects were selected based on the validation of Tromp et al. (Citation2020). This validation was crucial, as previous studies have shown that real-looking, familiar objects resulted in better memory performance compared to abstract objects (Zimmer et al., Citation2003). The presentation and interaction within the virtual environment were specially designed for maximal inclusivity (e.g., the application in older adults and neurological patients): (1) The interaction with the virtual objects was developed to be intuitive; it is performed with bare hands from a first-person perspective. (2) The task can be completed while standing or sitting with an adjustable table height, and no prior knowledge of computer use is required. (3) Instructions are displayed on virtual boards and simultaneously read aloud through built-in headphones, providing audiovisual instructions.
Introduction and tutorial
All participants completed the imVMT in a seated position. They were first introduced to the gesture-based natural hand interaction in a virtual room with two windows, a door, a plant, a clock, and a table in the middle (). While using their hands to interact with virtual objects from the first-person perspective, they saw a virtual representation of their hands performing simultaneous hand postures and motions. After an introduction with a tutorial and practice trials, the experiment started.
Figure 1. Procedure of the imVMT with (a) introduction and blocks including (b) no rotation and (c) rotation trials.
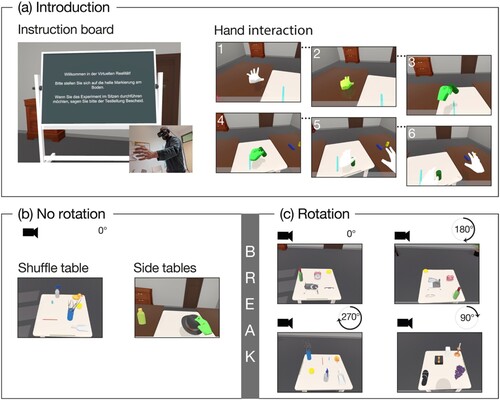
Task manipulations
The procedure of the imVMT is illustrated in . The task consisted of two blocks of 18 trials each (i.e., one trial begins by memorizing and ends by recalling the initial object arrangement on the table) and a 10-minute break in between to avoid fatigue and ensure the participants’ well-being. The following factors were parametrically manipulated:
An arrangement of three, five, or seven objects (covering a typical range of human visual working memory capacities; Luck & Vogel, Citation1997) was placed on the table and had to be memorized for 15 s (number of objects).
During recall, participants were required to place objects on the table from either the side tables or the shuffle table. The shuffle condition intended to increase the load on the visuospatial sketchpad (Baddeley, Citation2002) during the memorization phase. The idea was, to make it more difficult for participants to mentally imagine the original arrangement of objects when the objects on the table were presented randomly than when they saw an empty table in front of them (placement condition).
To further increase the mental load of the task, we manipulated the table side from which object retrieval was performed during the recall phase. The side of retrieval could either be identical to the side of memorization (0° rotation angle), or the participants were rotated around the centre of the table by 90°, 180°, or 270° (rotation angle).
The viewing angle for the memorization and recall phases in the first block remained identical (0°). The rotation condition was introduced with practice trials at the start of the second block. A green bar located close to one edge of the table in the immediate proximity of the participants indicated from which side the subjects had memorized the object locations. For practical and authenticity reasons, the side table condition was only applied at 0° and 180° as participants would otherwise have been rotated into the side tables. In the rotation block, subjects completed a total of 18, 6 trials each with 3, 5 and 7 objects (i.e., 0°: 6 trials, 180°: 6 trials, 90°: 3 trials, 270°: 3 trials). Two outcome measures of the imVMT were considered: (1) the mean distance error (MDE), defined as the Euclidean distance in centimetres between the initial object location (i.e., the object's centre of gravity) during memorization and the final object location after placement in the recall phase, and (2) the placement time, calculated as the total time between the start and end of each trial during recall, averaged for each object.
Participants
The study was conducted at the Clinic for Cognitive Neurology at the University Hospital Leipzig, Germany, from May 2019 to June 2021. A total of N = 35 participants (mean age: 47.34 ± 14.36; range 19–63 years; n = 12 females) with neurological disorders were included. Patient charts were consulted to obtain detailed information on patients’ medical history. Patients with neurological disorders of different etiologies, including vascular, inflammatory, neurodegenerative, and traumatic etiology, were included in (). Inclusion criteria were: neurological disorder, age between 18 and 80 years, and sufficient speech, communication, and visual abilities as determined by a therapist. For safety reasons, given the VR exposure, individuals with acute psychosis, a history of epilepsy in the past two years, symptoms of vertigo, or a history of alcohol or drug abuse were not eligible. We further excluded pregnant women, women who planned to become pregnant or were breastfeeding. In agreement with the Declaration of Helsinki, the study was approved by the Ethics Committee of the Medical Faculty of Leipzig University (177/18-lk) and was registered at the German Clinical Trial Registry (https://www.drks.de/drks_web/setLocale_EN.do; Identifier: DRKS00024005). Written informed consent was obtained from all participants prior to study participation and after a detailed explanation of study procedures. Participants could withdraw from the study at any time without providing any reasons and were ensured that they would be fully anonymized.
Table 1. Patient demographics.
Study design
We used a within-participant experimental design. For an overview of the two diagnostic sessions, see . A neuropsychological assessment battery of conventional paper-and-pencil tests was administered in the first session, including spatial memory tests. In the second session, the VR task was conducted using an HMD. Before and after the VR exposure, customized questionnaires were conducted to assess current well-being, usability, presence, and motivation. More detailed information about the procedure, the items used, and the neuropsychological test battery can be found in the Supplement.
Figure 2. Overview of the study design, including clinical screening, neuropsychological testing, questionnaire, and the imVMT.
Note. MoCA: Montreal Cognitive Assessment Test (Nasreddine et al., Citation2005), ROCF: Rey-Osterrieth Complex Figure Test (Osterrieth, Citation1944; Rey, Citation1941), VVM: Visual and Verbal Memory Test, figural subtest (Schellig & Schächtele, Citation2009), Corsi: Corsi Block-Tapping Test (Corsi, Citation1972), TMT: Trail Making Test (Rodewald et al., Citation2012), LPS: Proficiency Testing System, subtest 4 (Horn, Citation1983).
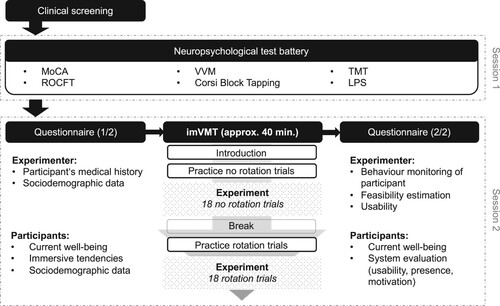
Neuropsychological assessment
A neuropsychological test battery of various conventional instruments was administered. We applied a comprehensive test-battery, in order to be able to examine the underlying cognitive processes in our newly developed VR-task. For the validation of the spatial memory component of the imVMT, we administered two conventional psychometric tests to measure different aspects of spatial memory abilities in different timeframes. The ROCF in the immediate recall condition measures object location and visuospatial memory, and the figural subtests of the VVM in the immediate recall condition is a measure for short-term visuospatial memory with navigation. As mentioned above, spatial memory acquires several additional cognitive processes, which we controlled for, by conducting the following tests: a screening for global cognitive impairment (MoCA), executive function (logical reasoning: LPS-3; cognitive flexibility: TMT-B), visual attention (TMT-A), visuospatial processing (ROCF Figure copying), and visuospatial working memory (Corsi Block Tapping) ().
Table 2. Paper-and-pencil tests in the neuropsychological test battery.
Questionnaires
Participants completed the Simulator Sickness Questionnaire (SSQ; Kennedy et al., Citation1993), a self-report inventory for physical well-being, and a short version of the Motion Sickness Susceptibility Questionnaire (MSSQ; Golding, Citation2006) to measure any symptoms associated with cybersickness. To systematically evaluate the feasibility and usability of the imVMT, the participants and experimenters completed questionnaires before and after VR exposure: Before testing, the participants were asked about their technical and computer skills and affinity to technology, immersive tendencies, and sociodemographic background. After VR exposure, items on immersive tendency, presence, motivation, object selection, hand interaction, and general impression were rated on five-point Likert scales (1 = low rating, 5 = high rating). Immersion was measured using the Immersive Tendencies Questionnaire (ITQ; Witmer & Singer, Citation1998), and presence levels were assessed with subscales of the Presence Questionnaire (PQ; Witmer & Singer, Citation1998) for concentration/focus and orientation, and the Self-Presence Questionnaire (SPQ; Ratan & Hasler, Citation2009). The strategies used to solve the task were collected in open questions, and general satisfaction, the impression of the virtual task, motivation, and usability were assessed by the Virtual Reality Usability Evaluation (VRUSE; Kalawsky, Citation1999) and self-generated items. An overview of all items used in this study is provided in the supplementary material.
Hardware and software components
After a careful and systematic analysis of the requirements and the available hardware (e.g., suitability to cover the imVMT, state-of-the-art visual resolution, minimal latency, easy-to-use hard – and software to ensure suitability for the long-term operation by clinical staff), the Oculus Rift consumer version 1 was selected. We used an Oculus Rift® (CV1) HMD (with two Pentile OLED displays and 1080 × 1200 resolution per eye, a refresh rate of 90 Hz, integrated headphones, a weight of 470 g, and a 110° field of view). A Leap Motion Controller, a depth-sensing stereo camera that enables natural and controller-free hand interaction in real-time, was attached to the HMD. Given a variety of neurological disorders and disabilities (e.g., motor impairments, neglect, hemianopsia) and the participants’ limited experience with traditional gaming controllers, the use of gesture-based natural hand interaction was deemed appropriate for VR applications in neurological patients. The Oculus Rift® (CV1) external infrared sensors were connected to the Omen 880-570 ng desktop computer (NVIDIA® GeForce RTX™ 2080Ti, Intel® Core™ i7 9700 K, 8 cores, 3.6 GHz) and placed in the room to track the HMD and by that allow the correct (6 degrees of freedom) representation of the virtual environment within the test room. The imVMT was programmed with Unity3D (version 2019.4.6f1), a 3D real-time development platform and game engine. Virtual 3D household objects were standardized and validated on recognizability, familiarity, visual complexity, contact, and usage in daily life to ensure the suitability of the virtual items used in this task (Tromp et al., Citation2020).
Statistical analysis
All data were analyzed using R (version 4.0.2) and RStudio (version 1.3.1093). Statistical significance was defined as p < .05. Missing values were imputed by mean substitution at participant level to preserve statistical power. We computed two outcome measures, the MDE and the placement time, as direct proxies of spatial memory.
Construct validity was analyzed by comparing the MDE with conventional tests of spatial memory, namely the immediate recall of the Rey Osterrieth Complex Figure Test and the visual subtest of the VVM. The primary criterion for this assessment was the Pearson correlation between MDE the two immediate recall test scores. Post-hoc comparisons were performed with Bonferroni correction for significant differences. Cronbach's alpha was used to assess the internal consistency across the correlational analyses using the psych package (Revelle, Citation2021) and interpreted according to Kline (Citation2000). We ran two linear mixed models to explain the variance in (1) MDE and (2) placement time with rotation angle and number of objects as fixed effects and participants as a random effect. A factor analysis was conducted to extract an underlying latent dimension that explains common variance and covariation between the MDE and the spatial memory tests (VVM and ROCF) in the immediate recall. Bartlett's Test of Sphericity and the Kaiser-Meyer-Olkin (KMO) for measuring sampling adequacy were conducted on the correlation matrix to check the suitability of the factor analysis. The KMO examines the strength of the partial correlation between the variables, and a minimum of .5 (Field et al., Citation2012) or .6 (Hutcheson & Sofroniou, Citation1999) has been recommended. A unit-weighted composite score for spatial memory was calculated, equally comprising scores of VVM and ROCF in the immediate recall and used in a linear mixed model to explain variance in MDE. The use of a composite score may be beneficial in providing information about overall spatial memory that may not be available from each test alone (Gibbons et al., Citation2012).
To examine feasibility, separate linear mixed models were fitted to regress the SSQ subscores for disorientation, nausea, oculomotor symptoms, and the total SSQ score on the time of measurement (immediately before and after VR exposure, fixed effect) and participants as a random effect. Usability measures are summarized as descriptive statistics.
Results
Effects of difficulty parameters on MDE
Effect of placement condition (shuffle vs. side tables)
Linear regression was carried out to investigate the relation between MDE and placement condition (shuffle vs. side tables). The model explained an insignificant and very weak proportion of variance (R2 = .00, F(1, 68) = 0.09, p = .767, = −0.013) and no significant differences were found for placement condition, t(68) = –0.30, p = .767. Therefore, data from shuffle and side table conditions were combined for further analyses.
Effect of number of objects and rotation angle on MDE
The linear mixed model to assess variance in MDE with rotation angle and number of objects as fixed effects and participants as a random effect had substantial explanatory power ( = .45). The variance explained by the fixed effects was 14.2% and 35.6% of the total variance of the random effects was attributed to the nested effect. We found significant main effects for number of objects, F(2) = 19.94, p < .001,
= 0.09, and rotation angle, F(3) = 18.98, p < .001,
= 0.13, but no significant interaction between number of objects and rotation angle, F(6) = 1.11, p = .354,
= 0.02. Increasing the number of objects and introducing rotation resulted in gradually higher MDEs (). The MDE averaged for all trials was highest for seven objects (M(SD) = 11.46(4.00)) compared to five objects (M(SD) = 9.45(3.58)), t(381) = 4.23, p < .001, and three objects (M(SD) = 7.94(2.70)), t(381) = 6.07, p < .001. We found no significant difference in MDE between three and five objects, t(380) = 1.85, p = .196. The 0° condition yielded lower MDEs (M(SD) = 8.92(3.42)) compared to 90° (M(SD) = 11.8(4.87)), t(385) = −4.43, p < .001, 180° (M(SD) = 12.8(4.42)), t(384) = −7.31, p < .001, and 270° (M(SD) = 11.6(5.59)), t(385) = −4.67, p < .001, while for the three rotation conditions only 90° and 180° differed significantly from each other, t(381) = – 2.81, p < .05. For detailed information, see Figures 1 and 2 in the supplementary material.
Figure 3. Mean distance error for three, five, and seven objects averaged for the no rotation and rotation condition.
Note. Seven objects yielded higher mean distance errors compared to five and three objects. * Indicates p < .05. ** indicates p < .01. *** indicates p < .001
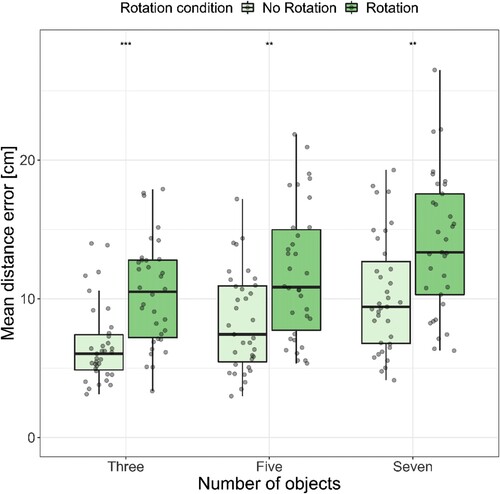
Influence of number of objects and rotation on placement time
No effects were found for number of objects and rotation on the mean placement time. More information on this is provided in the supplementary material.
Construct validity
Validation concerning spatial memory
shows the descriptive statistics of patient performance in the neuropsychological tests. Reliability analysis for MDE, ROCF, and VVM showed overall acceptable internal reliability with Cronbach’s alpha of α = .79. The KMO yielded a mediocre overall value of 0.69, with values for MDE, ROCF, VVM ranging between 0.64 and 0.78 (i.e., above the minimum level of .5). Bartlett’s test of sphericity (χ2(3) = 31.23, p < .001) showed that the correlations between the three outcome variables were sufficiently large. Thus, factor analysis was appropriate. Factor analysis suggested that the three tests load onto the same latent construct, as VVM (0.92), ROCF (0.77), and MDE (0.64) showed large positive loadings and together accounted for 62% of the variance with an eigenvalue of 1.85. The correlation of the factor and the observed data is r = 0.94, with a multiple R2 = .89. With the three items loading on the same latent construct, we referred to it as “spatial memory.”
Table 3. Neuropsychological tests with subtests, maximum scores, and descriptive statistics.
The linear model regressing the ROCF scores (immediate recall) on the MDE explained 35.1% of variance, F(1, 30) = 16.2, p < .001, = 0.32. The effect of MDE was statistically significant and negative (β = −.59, 95% CI [−0.89, –0.29]), t(30) = −4.02, p < .001. An analogous linear model to explain variance in VVM (immediate recall) with MDE explained 26.4% of variance, F(1, 29) = 10.4, p = .003,
= 0.23. Likewise, the effect of MDE was statistically significant and negative (β = −.51, 95% CI [−0.84, –0.19]), t(29) = −3.23, p = 0.003. Modelling the unit-weighted composite score with MDE again explained 34.6% of the variance, F(1, 28) = 14.78, p < .001,
= 0.32 with a negative effect of MDE (β = −.59, 95% CI [−0.90, –0.27]), t(28) = −3.84, p < .001. These results were largely independent of the rotation condition, as these results were similar in the no rotation condition and rotation condition. The regression results are shown in and shows the correlations between MDE and the two spatial memory tests.
Figure 4. Correlations between MDE and ROCF (left) and VVM (right) in the immediate recall.
Note. MDE correlated significantly with ROCF, r = -0.59, p < .001, and VVM, r = -0.51, p = .003, in the immediate recall. Higher scores in ROCF and VVM indicate better performance, whereas higher MDE indicate worse performance. ROCF: Rey-Osterrieth Complex Figure Test (Osterrieth, Citation1944; Rey, Citation1941), VVM: Visual and Verbal Memory Test, figural subtest (Schellig & Schächtele, Citation2009).
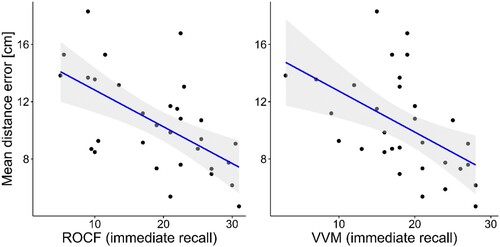
Table 4. Regression results of the ROCF and VVM in the immediate recall and their composite score with MDE as regressor.
Validation concerning control variables
The results of the neuropsychological tests are shown in . We found weak to moderately negative correlations between the MDE and VVM in the delayed recall condition, r = −0.56, p = .001, the ROCF in the copy condition, r = −0.47, p = .006, between the MDE and the LPS, r = −0.46, p = .008, the TMT-A, r = 0.40, p = .018, and TMT-B, r = 0.44, p = .010. There was a statistically significant, moderate negative correlation between MDE and MoCA total score, r = −0.66, p < .001.
We controlled for the MoCA score by including it as a covariate with VVM and ROCF immediate recall in a linear regression model with grand-average MDE as the regressor variable. A significant regression equation was found, F(3, 25) = 9.28, p < .001, with an R2 of .53. The effects of VVM (immediate recall), β = −.14, 95% CI [−0.54, 0.26], t(25) = −0.72, p = .477, = 0.28, and ROCF (immediate recall), β = −.22, 95% CI [−0.66, 0.22], t(25) = –1.03, p = .313,
= 0.15, were non-significant, whereas the MoCA score was a significant predictor of MDE, β = −.50, 95% CI [−0.83, –0.16], t(25) = −3.06, p = .005,
= 0.22. Additionally, Table 2 in the supplementary material shows the intercorrelations between the different neuropsychological tests, and with the MDE.
Applicability and feasibility
All participants successfully completed the VR task without noticeable side-effects. The HMD was not removed during the task, and no discomfort was expressed. All statistical effects for the SSQ subscores before and after imVMT were insignificant: nausea, β = −.02, 95% CI [–0.29, 0.25], t(66) = −0.14, p = .892 = 0.00, oculomotor, β = −.11, 95% CI [−0.44, 0.22], t(66) = −0.63, p = .530,
= 0.00, disorientation, β = −.03, 95% CI [−0.35, 0.28], t(66) = −0.21, p = .837,
= 0.00, and total SSQ score, β = −.07, 95% CI [−0.38, 0.24], t(66) = −0.44, p = .659,
= 0.00. The average MSSQ sum score (range: 0–12) for the assessment of the predisposition to VRISE was 4.26 points (SD = 2.96), suggesting that most participants encountered motion sickness-related symptoms in the last 10 years, for example induced by cars, buses, trains or airplanes (M(SD) = 1.6(0.97)), ships (M(SD) = 1.54(1.22)), or funfair rides (M(SD) = 1.11(1.21)).
Immersive tendencies and presence
In general, participants tended to concentrate well on enjoyable activities (M(SD) = 3.91(0.66)) and lost track of time when involved in tasks (M(SD) = 3.57(0.97)). During the imVMT, participants felt present at the table (M(SD) = 3.45(1.15)) and experienced their virtual hands as their own (M(SD) = 3.40(1.1)). Furthermore, presence within the virtual world was high, as participants indicated that their body was in the physical testing room during the simulation, but they felt like being within the virtual world (M(SD) = 3.97(0.74)).
Motivation
On average, the participants enjoyed the task regardless of the technical setup (M(SD) = 4.14(0.70)) and the technical setup regardless of the task (M(SD) = 4.37(0.49)). They could imagine using the programme for a more extended period (M(SD) = 3.77(0.69)) and their motivation to accomplish the task within the virtual world was moderately comparable to real-life (M(SD) = 3.83(0.98)).
Object selection and hand interaction
The objects in the virtual world were evaluated as realistic (M(SD) = 3.74(0.87)) and the motion control was perceived as natural (M(SD) = 3.87(0.70)). There was an ease of moving and selecting the virtual objects (M(SD) = 3.47(0.74)) and undo mistakes (M(SD) = 3.63(0.73)). Some participants felt they kept making mistakes while interacting with the system (M(SD) = 2.40(0.98)), and they were bothered that they did not feel the virtual objects in their hands (M(SD) = 2.31(0.99)). We found no significant differences between patients with and without hemiparesis in rating for object selection, F(1, 33) = 0.42, p = .523, = −0.02, and hand interaction, F(1, 33) = 0.008, p = .926,
= −0.03. Despite their hemiparesis, it was easy to select and move objects in the virtual world (M(SD) = 3.36(0.84)) and undo mistakes (M(SD) = 3.64(0.84)).
General impression
There was a strong willingness to use VR glasses regularly (M(SD) = 3.97(0.62)). No further help was needed while the programme was running (M(SD) = 4.00(0.80)) and, in the end, participants felt confident using the imVMT (M(SD) = 3.97(0.62)). Most respondents felt that they could anticipate well what would follow as a reaction to their actions (M(SD) = 3.69(0.75)). The overall general impression of the task was mixed (M(SD) = 3.30(0.63)).
Discussion
This study evaluated the Immersive Virtual Memory Task (imVMT), a novel VR paradigm for assessing spatial memory with naturalistic hand interaction, in N = 35 individuals with acquired brain injuries. We evaluated the effects of the applied difficulty parameters: number of objects (3, 5, 7), rotation angles (No rotation: 0°, Rotation: 90°, 180°, 270°), and placement condition (shuffle vs. side tables). The primary objective was to validate the imVMT in terms of spatial memory performance and other cognitive functions with conventional neuropsychological tests. Finally, we evaluated the clinical feasibility and applicability of the immersive VR task with gesture-based hand interaction in a heterogeneous neurological sample with different sensorimotor and cognitive deficits, with a special focus on cybersickness and usability.
There are three main findings: (1) We showed that key experimental parameters, such as the number of objects to be memorized and the rotation of the retrieval perspective, efficiently manipulate task difficulty and affect the outcome variable MDE, while the placement condition does not. (2) We demonstrated the construct validity of the paradigm, as the MDE was significantly correlated with spatial memory performance in conventional tests of spatial memory. However, the MDE also correlated with global cognition (MoCA), deductive reasoning (LPS), visuomotor processing speed, task switching, and visual attention (TMT), which calls into question the specificity of the MDE as an indicator of spatial memory. (3) The imVMT was highly feasible in a wide range of neurological patients with different functional deficits and was rated high in usability. All participants, including those with motor impairments and visual field defects, successfully engaged in object selection and hand interaction and rated the imVMT as easy to use. There were no adverse effects. Overall, these findings suggest that the imVMT is a clinically feasible, ecologically relevant and externally valid task for assessing object location memory in immersive VR in a wide range of neurological disorders.
Effect of difficulty parameters on MDE
As expected, both the number of objects to be recalled and the rotation angle resulted in a higher level of task difficulty. This suggests that our task manipulations were successful and allow the measurement of spatial memory in both easy and more challenging conditions, thereby avoiding floor or ceiling effects. This may be relevant for future studies that apply the task to individuals with specific impairments in spatial processing or spatial memory and compare their performance to healthy controls. We did not, however, find an interaction between the number of objects and the rotation angle for the MDE. It is possible that this lack of an additive effect is due the relatively small sample size and heterogeneous study population, or the high level of task difficulty. There was no effect of the retrieval condition (shuffle or side tables). There seemed to be no difference whether the subjects memorized the object arrangement while looking at an empty table or at a table with the objects in a newly shuffled arrangement. The suggestion that the latter might increase the load on visuospatial sketchpad, the modality-specific store of visuospatial working memory according to Baddeley's (Citation2002) model, could not be demonstrated. This confirms that the task appears to rely less on short-term and more on longer-term memory processing. Furthermore, the lack of differences between shuffle and side tables allows for one of these conditions to be omitted, reducing the number of trials and shortening the task duration.
Construct validation
The test validation results revealed an intricate pattern regarding the underlying cognitive functions that correlated with imVMT performance. We observed that the MDE, as the primary outcome measure of object-location memory, correlated significantly with the immediate recall of two routine tests of spatial memory, i.e., the ROCF and the VVM. Moderate correlations were expected as conventional tests probably address different aspects of the construct of spatial memory. As mentioned above, paper-based tests are static, abstract and two-dimensional, whereas the imVMT is dynamic, interactive and set in a fully immersive three-dimensional environment. Thus, the validity of paper-pencil tasks for measuring spatial memory has been described as limited (Fastenau et al., Citation1999; Shin et al., Citation2006). Nevertheless, these tests were used as validation criteria because of their clinical relevance as established procedures. The moderate correlation may indicate that there is some shared variance between conventional spatial memory tasks and the imVMT, but the imVMT also goes beyond this. To better understand which processes characterize these additional aspects, further neuropsychological functions were analyzed in a comprehensive test battery.
Multiple cognitive processes are involved in object retention and retrieval. For instance, visuo-perceptual processing and visuo-constructive abilities, as measured by the ROCF copy task, are correlated with both conventional spatial memory tests and the MDE. However, participants’ sensorimotor deficits may affect the performance of paper-and-pencil tests differently than the VR task. Additionally, impairments in visuo-perceptual and visuo-constructive abilities, independent of motor deficits, may contribute to the spatial memory performance on both the paper-and-pencil and VR tasks.
As a neuropsychological screening covering multiple cognitive domains, the MoCA was applied as an overall estimate of global cognitive function (Harvey, Citation2012). The observed strong negative correlation between the MDE and the MoCA total score may indicate that the imVMT is affected not only by visual memory, but also by global cognitive impairment. A variety of cognitive functions, rather than isolated ones, are required to perform everyday tasks (e.g., finding keys). Similarly, the imVMT may measure global cognition in addition to spatial memory because of its high everyday relevance and similarity to everyday life. However, given the nature of the task (remembering and recalling the location of objects in a spatial environment), it is likely that a large proportion of the cognitive functions required to perform the task correctly can be attributed to spatial memory. Correlations between the MDE and conventional tests of spatial memory (VVM, ROCF), as well as between the MOCA and VVM, ROCF, support this assumption. Another rather global measure of cognition may be logical reasoning (measured by the LPS-4), which also shows moderate correlations with the MDE as well as ROCF and VVM. This may indicate that other basic functions, such as cognitive flexibility (i.e., adapting behaviour to changing situations, such as the rotation conditions or considering alternative object locations; used for problem solving), are necessary to complete the tasks. This may lead to a general discussion as to whether a VR task, due to its high degree of relevance and similarity to real-life situations, can effectively measure distinct and specific cognitive functions, or whether it always assesses a conglomerate of numerous cognitive functions.
Furthermore, the observed correlation seems plausible given that several different cognitive functions were impaired in our study population and that several cognitive functions are required to perform the imVMT. However, when we controlled for MoCA total score, the correlations between the MDE and the spatial memory tests (i.e., VVM and ROCF in immediate recall) were no longer significant, while the correlation with the MoCA remained moderate and significant. These results suggest that the MDE is sensitive to spatial ability and spatial memory as an integral part of global cognitive function. Furthermore, Bellmund et al. (Citation2018) suggest that spatial memory may be a core cognitive function that is relevant to all cognitive processing. They argue that the hippocampal neural mechanisms identified in spatial navigation research operate across information domains to support a wide range of cognitive functions. Given the heterogeneity of our population, with motor (e.g., paresis) and perceptual (e.g., hemianopia, neglect) deficits, we were not able to further identify the specificity of our task. However, examination of more specific study populations, especially those with hippocampal deficits as a model for spatial memory, those with hemispatial neglect as a model for spatial deficits, and a comparison of younger vs. older healthy adults will show which parameters of the imVMT can further discriminate different underlying cognitive processes.
Applicability and feasibility
Our results on system feasibility confirmed that the virtual everyday objects used in the imVMT are appropriate for clinical application. In particular, the object selection ratings showed that the virtual objects were perceived as realistic, the motion control as natural, and the object selection as easy. These results are in line with Tromp et al. (Citation2020), who previously validated the same objects as highly realistic and recognizable. Furthermore, it has been reported that the use of an HMD leads to higher object localization accuracy, suggesting that immersive VR may better reflect spatial memory performance than two-dimensional tasks (Murcia-López & Steed, Citation2016). Taken together, the virtual objects and the application of immersive VR for our spatial memory task are suitable for a clinical immersive object localization memory task.
The imVMT was successfully applied in a neurological population with a wide range of functional impairments, and we demonstrated clinical acceptability, feasibility, and natural hand interaction usability. It was well-tolerated and did not induce any symptoms of cybersickness. Our findings, therefore, provide further evidence for the clinical applicability of immersive VR in neurological patients, confirming previous findings in Alzheimer's disease (Allain et al., Citation2014; Davis & Ohman, Citation2016; Morganti et al., Citation2013; Serino et al., Citation2015), traumatic brain injury (Christiansen et al., Citation1998), and stroke (Brooks et al., Citation2004; da Silva Ribeiro et al., Citation2015; Held et al., Citation2018; Mousavi Hondori et al., Citation2016). Our findings highlight that patients with additional sensorimotor deficits, such as hemiparesis and hemianopia, successfully engaged in gesture-based natural hand interaction in VR.
Clinical application and study limitations
In addition to the scientific benefits of investigating object location memory in realistic, small-scale spaces, these results have broader implications for clinical practice. We have shown that participants with motor and speech impairments quickly learned to use the imVMT, enjoyed the VR task, and were willing to use HMDs more often. Immersive VR applications with gesture-based hand interaction, such as the imVMT, could thus be integrated into the diagnostic and rehabilitation process to identify and train spatial memory deficits alongside traditional paper-and-pencil tests. A mobile (i.e., wireless and not requiring a separate PC), intuitive and easy-to-use version of the imVMT has already been developed to extend the use of the imVMT from the clinical setting to the patient's home environment.
Nevertheless, certain limitations of this study are worth mentioning and will be addressed in future research. The present study was planned as a first feasibility study in patients with various neurological disorders to examine possible side effects and the applicability of neuropsychological assessment in VR. The small sample size made it difficult to generalize the results, given the heterogeneous sample of patients, which also limited the statistical analyses. For example, the sample was too small to run a factor analysis for all neuropsychological tests and subtests, which might have provided more insight into the underlying cognitive processes. In addition, feasibility and acceptability in clinical practice may be considerably lower as the study inclusion criteria were specific to patients with adequate visual, language, cognitive and motor function. In an ongoing study, our research group targets more specific and homogeneous study populations with relevant spatial memory deficits, such as Alzheimer's disease. We compare healthy young and elderly participants to validate the imVMT and investigate the underlying cognitive mechanisms. The exploratory nature of the task further made the imVMT potentially complex and time consuming. As we found no difference between the placement conditions (shuffle and sides tables), it will be possible to reduce the total number of trials by omitting one of the placement conditions while not forfeiting valuable data. This would be of interest to increase the clinical applicability and to reduce the execution time of the task.
Furthermore, we chose the MDE as the central outcome measure for this first evaluation study, fully aware that it represents a summary estimate of spatial memory performance. However, it is important to note that intra – and individual error patterns, such as site-, object-, or group-specific variations, may be inadequately and insufficiently captured by a single metric like the MDE. In line with this, previous research criticized mean displacement scores for spatial memory assessment and proposed scores that accounted for whether an item was placed within a specific range (e.g., target score, first quadrant score) (Sharps & Gollin, Citation1986). A follow-up study or analysis could extend the current findings by including several appropriate outcome parameters to assess spatial memory and explore their relationship, especially in more selected patient groups. This may help to draw more profound conclusions about underlying cognitive processes and disorder-specific spatial memory deficits by deriving a cognitive profile rather than a single score. Thus, future research should include indices of spatial reference to the environment, such as the room or inter-object relation, viewer-dependent measures (Easton & Sholl, Citation1995), deviation angles, and examine object locations independent of their identity (Postma & De Haan, Citation1996). Furthermore, VR tasks can be complemented by evaluation algorithms to disentangle visuo-perceptual processing and memory performance, representing a promising target for future studies. For instance, the integration of visual discrimination as well as visual sequencing tasks with and without rotation, proximity, and distance estimation tasks could provide valuable information. Finally, it should be considered that the perceived novelty of VR applications in clinical settings may lead patients to perceive the task more positively (Wells et al., Citation2010).
Conclusion
As immersive VR applications are increasingly used for clinical applications, it is essential to identify appropriate parameters for cognitive processes within the vast amount of behavioural data generated during VR. We investigated spatial memory performance in a small-scale space using the imVMT, an immersive VR application with gesture-based hand interaction, highlighting the importance of object-location memory. Our results suggest that this paradigm was well-designed in terms of parameters that can be used to manipulate task difficulty, making it suitable for severely impaired subjects, such as those with Alzheimer's disease, as well as for healthy subjects without floor or ceiling effects. The VR task is well-accepted by a wide range of neurological patients, the clinical feasibility is excellent and the imVMT provides a sensitive assessment of object-location memory in small-scale spaces.
3_Supplementary_Material_Revision_NRH-ES_45.22.R1_CLEAN.docx
Download MS Word (2.4 MB)Acknowledgements
This research was supported by the German Federal Ministry of Education and Research under grant number 136W0206 and originated within the consortium of the research project “Virtual Realities for Digital Diagnostics and Cognitive Rehabilitation” (VReha). We thank our project partner HASOMED and especially Bert Vehmeier and Mark Hofman for their support in designing the web-based interface to collect participant details and questionnaires. We gratefully acknowledge Henrike Bieber, Kristina Schimpl, and Anna Schatz for their valuable assistance with data collection.
Data availability statement
The data that support the findings of this study are available from the corresponding author, JB, upon reasonable request.
Disclosure statement
No potential conflict of interest was reported by the author(s).
Additional information
Funding
References
- Allain, P., Foloppe, D. A., Besnard, J., Yamaguchi, T., Etcharry-Bouyx, F., Le Gall, D., Nolin, P., & Richard, P. (2014). Detecting everyday action deficits in Alzheimer's disease using a nonimmersive virtual reality kitchen. Journal of the International Neuropsychological Society, 20(5), 468–477. https://doi.org/10.1017/S1355617714000344
- Astur, R. S., Tropp, J., Sava, S., Constable, R. T., & Markus, E. J. (2004). Sex differences and correlations in a virtual Morris water task, a virtual radial arm maze, and mental rotation. Behavioural Brain Research, 151(1–2), 103–115. https://doi.org/10.1016/j.bbr.2003.08.024
- Awh, E., Jonides, J., & Reuter-Lorenz, P. A. (1998). Rehearsal in spatial working memory. Journal of Experimental Psychology: Human Perception and Performance, 24(3), 780–790. https://doi.org/10.1037/0096-1523.24.3.780
- Baddeley, A. (1995). Working memory (Repr.). In Oxford Psychology Series, 11. Oxford Clarendon Press.
- Baddeley, A. (2002). Is working memory still working? European Psychologist, 7(2), 85–97. https://doi.org/10.1027//1016-9040.7.2.85
- Barel, E. (2019). Effects of attention during encoding on sex differences in object location memory. International Journal of Psychology, 54(4), 539–547. https://doi.org/10.1002/ijop.12490
- Bellgardt, M., Krause, N., & Kuhlen, T. W. (2020). When spatial devices are not an option: Object manipulation in virtual reality using 2D input devices. https://doi.org/10.18420/VRAR2020_9
- Bellmund, J. L. S., Gärdenfors, P., Moser, E. I., & Doeller, C. F. (2018). Navigating cognition: Spatial codes for human thinking. Science, 362(6415). https://doi.org/10.1126/science.aat6766
- Brooks, B. M., Rose, F. D., Potter, J., Jayawardena, S., & Morling, A. (2004). Assessing stroke patients’ prospective memory using virtual reality. Brain Injury, 18(4), 391–401. https://doi.org/10.1080/02699050310001619855
- Burgess, N. (2006). Spatial memory: How egocentric and allocentric combine. Trends in Cognitive Sciences, 10(12), 551–557. https://doi.org/10.1016/j.tics.2006.10.005
- Caserman, P., Garcia-Agundez, A., Gámez Zerban, A., & Göbel, S. (2021). Cybersickness in current-generation virtual reality head-mounted displays: Systematic review and outlook. Virtual Reality, 25(4), 1153–1170. https://doi.org/10.1007/s10055-021-00513-6
- Chaytor, N., & Schmitter-Edgecombe, M. (2003). The ecological validity of neuropsychological tests: A review of the literature on everyday cognitive skills. Neuropsychology Review, 13(4), 181–197. https://doi.org/10.1023/B:NERV.0000009483.91468.fb
- Cherry, K. E., Park, D. C., & Donaldson, H. (1993). Adult age differences in spatial memory: Effects of structural context and practice. Experimental Aging Research, 19(4), 333–350. https://doi.org/10.1080/03610739308253942
- Chirico, A., Yaden, D. B., Riva, G., & Gaggioli, A. (2016). The potential of virtual reality for the investigation of awe. Frontiers in Psychology, 7, 1766. https://doi.org/10.3389/fpsyg.2016.01766
- Cho, B. H., Lee, J. M., Ku, J. H., Jang, D. P., Kim, J. S., Kim, I. Y., Lee, J. H., & Kim, S. I. (2002). Attention enhancement system using virtual reality and EEG biofeedback. Proceedings IEEE Virtual Reality 2002, 156–163.
- Christiansen, C., Abreu, B., Ottenbacher, K., Huffman, K., Masel, B., & Culpepper, R. (1998). Task performance in virtual environments used for cognitive rehabilitation after traumatic brain injury. Archives of Physical Medicine and Rehabilitation, 79(8), 888–892. https://doi.org/10.1016/S0003-9993(98)90083-1
- Cogné, M., Taillade, M., N’Kaoua, B., Tarruella, A., Klinger, E., Larrue, F., Sauzéon, H., Joseph, P.-A., & Sorita, E. (2017). The contribution of virtual reality to the diagnosis of spatial navigation disorders and to the study of the role of navigational aids: A systematic literature review. Annals of Physical and Rehabilitation Medicine, 60(3), 164–176. https://doi.org/10.1016/j.rehab.2015.12.004
- Colombo, D., Serino, S., Tuena, C., Pedroli, E., Dakanalis, A., Cipresso, P., & Riva, G. (2017). Egocentric and allocentric spatial reference frames in aging: A systematic review. Neuroscience & Biobehavioral Reviews, 80, 605–621. https://doi.org/10.1016/j.neubiorev.2017.07.012
- Corsi, P. M. (1972). Human memory and the medial temporal region of the brain. Dissertation Abstracts International, 34(2), 891B.
- da Silva Ribeiro, N. M., Ferraz, D. D., Pedreira, É, Pinheiro, Í, da Silva Pinto, A. C., Neto, M. G., dos Santos, L. R. A., Pozzato, M. G. G., Pinho, R. S., & Masruha, M. R. (2015). Virtual rehabilitation via Nintendo Wii® and conventional physical therapy effectively treat post-stroke hemiparetic patients. Topics in Stroke Rehabilitation, 22(4), 299–305. https://doi.org/10.1179/1074935714Z.0000000017
- Davis, R., & Ohman, J. (2016). Wayfinding in ageing and Alzheimer's disease within a virtual senior residence: Study protocol. Journal of Advanced Nursing, 72(7), 1677–1688. https://doi.org/10.1111/jan.12945
- De Renzi, E., Faglioni, P., & Previdi, P. (1977). Spatial memory and hemispheric locus of lesion. Cortex, 13(4), 424–433. https://doi.org/10.1016/S0010-9452(77)80022-1
- Diaz-Orueta, U., Rogers, B. M., Blanco-Campal, A., & Burke, T. (2022). The challenge of neuropsychological assessment of visual/visuo-spatial memory: A critical, historical review, and lessons for the present and future. Frontiers in Psychology, 13, 962025. https://doi.org/10.3389/fpsyg.2022.962025
- Diersch, N., & Wolbers, T. (2019). The potential of virtual reality for spatial navigation research across the adult lifespan. Journal of Experimental Biology, 222(Pt Suppl 1), jeb187252. https://doi.org/10.1242/jeb.187252
- Easton, R. D., & Sholl, M. J. (1995). Object-array structure, frames of reference, and retrieval of spatial knowledge. Journal of Experimental Psychology: Learning, Memory, and Cognition, 21(2), 483–500. https://doi.org/10.1037/0278-7393.21.2.483
- Estes, Z., Verges, M., & Adelman, J. S. (2015). Words, objects, and locations: Perceptual matching explains spatial interference and facilitation. Journal of Memory and Language, 84, 167–189. https://doi.org/10.1016/j.jml.2015.06.002
- Faria, A. L., Andrade, A., Soares, L., & i Badia, S. B. (2016). Benefits of virtual reality based cognitive rehabilitation through simulated activities of daily living: A randomized controlled trial with stroke patients. Journal of NeuroEngineering and Rehabilitation, 13(1), 96. https://doi.org/10.1186/s12984-016-0204-z
- Fastenau, P. S., Denburg, N. L., & Hufford, B. J. (1999). Adult norms for the Rey-Osterrieth complex figure test and for supplemental recognition and matching trials from the extended complex figure test. The Clinical Neuropsychologist, 13(1), 30–47. https://doi.org/10.1076/clin.13.1.30.1976
- Fernandez Montenegro, J. M., & Argyriou, V. (2017). Cognitive evaluation for the diagnosis of Alzheimer's disease based on turing test and virtual environments. Physiology & Behavior, 173, 42–51. https://doi.org/10.1016/j.physbeh.2017.01.034
- Field, A. P., Miles, J., & Field, Z. (2012). Discovering statistics using R. Sage.
- Freeman, D., Reeve, S., Robinson, A., Ehlers, A., Clark, D., Spanlang, B., & Slater, M. (2017). Virtual reality in the assessment, understanding, and treatment of mental health disorders. Psychological Medicine, 47(14), 2393–2400. https://doi.org/10.1017/S003329171700040X
- Gibbons, L. E., Carle, A. C., Mackin, R. S., Harvey, D., Mukherjee, S., Insel, P., Curtis, S. M., Mungas, D., & Crane, P. K. (2012). A composite score for executive functioning, validated in Alzheimer’s Disease Neuroimaging Initiative (ADNI) participants with baseline mild cognitive impairment. Brain Imaging and Behavior, 6(4), 517–527. https://doi.org/10.1007/s11682-012-9176-1
- Golding, J. F. (2006). Motion sickness susceptibility. Autonomic Neuroscience, 129(1–2), 67–76. https://doi.org/10.1016/j.autneu.2006.07.019
- Harvey, P. (2012). Clinical applications of neuropsychological assessment. Dialogues in Clinical Neuroscience, 14(1), 91–99. https://doi.org/10.31887/DCNS.2012.14.1/pharvey
- Held, J. P. O., Klaassen, B., Eenhoorn, A., van Beijnum, B.-J. F., Buurke, J. H., Veltink, P. H., & Luft, A. R. (2018). Inertial sensor measurements of upper-limb kinematics in stroke patients in clinic and home environment. Frontiers in Bioengineering and Biotechnology, 6, 27. https://doi.org/10.3389/fbioe.2018.00027
- Hofmann, S. M., Klotzsche, F., Mariola, A., Nikulin, V., Villringer, A., & Gaebler, M. (2021). Decoding subjective emotional arousal from EEG during an immersive virtual reality experience. ELife, 10, e64812. https://doi.org/10.7554/eLife.64812
- Horn, W. (1983). Leistungsprüfsystem [Performance testing system]. Verl. für Psychologie Hogrefe (2nd ed.). Hogrefe.
- Howieson, D. (2019). Current limitations of neuropsychological tests and assessment procedures. The Clinical Neuropsychologist, 33(2), 200–208. https://doi.org/10.1080/13854046.2018.1552762
- Hutcheson, G., & Sofroniou, N. (1999). The multivariate social scientist: Introductory statistics using generalized linear models. Sage. https://doi.org/10.4135/9780857028075
- Jonson, M., Avramescu, S., Chen, D., & Alam, F. (2021). The role of virtual reality in screening, diagnosing, and rehabilitating spatial memory deficits. Frontiers in Human Neuroscience, 15, 628818. https://doi.org/10.3389/fnhum.2021.628818
- Kalawsky, R. S. (1999). VRUSE—a computerised diagnostic tool: For usability evaluation of virtual/synthetic environment systems. Applied Ergonomics, 30(1), 11–25. https://doi.org/10.1016/S0003-6870(98)00047-7
- Kennedy, R. S., Lane, N. E., Berbaum, K. S., & Lilienthal, M. G. (1993). Simulator sickness questionnaire: An enhanced method for quantifying simulator sickness. The International Journal of Aviation Psychology, 3(3), 203–220. https://doi.org/10.1207/s15327108ijap0303_3
- Kimura, D. (1963). Right temporal-lobe damage: Perception of unfamiliar stimuli after damage. Archives of Neurology, 8(3), 264. https://doi.org/10.1001/archneur.1963.00460030048004
- Klencklen, G., Després, O., & Dufour, A. (2012). What do we know about aging and spatial cognition? Reviews and perspectives. Ageing Research Reviews, 11(1), 123–135. http://doi.org/10.1016/j.arr.2011.10.001
- Kline, P. (2000). The handbook of psychological testing (2nd ed.). Taylor & Frances/Routledge.
- Koenig, S., Crucian, G. P., Dünser, A., Bartneck, C., & Dalrymple-Alford, J. (2011). Development of a spatial memory task in realistic virtual environments. In Proceedings of virtual reality international conference (VRIC 2011), 6–8 April 2011, Laval, France. https://doi.org/10.13140/2.1.5052.9286
- Kourtesis, P., Collina, S., Doumas, L., & MacPherson, S. (2021). Validation of the Virtual Reality Everyday Assessment Lab (VR-EAL): An immersive virtual reality neuropsychological battery with enhanced ecological validity. Journal of the International Neuropsychological Society, 27(2), 181–196. https://doi.org/10.1017/S1355617720000764
- Kourtesis, P., & MacPherson, S. E. (2021). An ecologically valid examination of event-based and time-based prospective memory using immersive virtual reality: The influence of attention, memory, and executive function processes on real-world prospective memory. Neuropsychological Rehabilitation, 1–26. https://doi.org/10.1080/09602011.2021.2008983
- Krohn, S., Tromp, J., Quinque, E. M., Belger, J., Klotzsche, F., Rekers, S., Chojecki, P., de Mooij, J., Akbal, M., McCall, C., Villringer, A., Gaebler, M., Finke, C., & Thöne-Otto, A. (2020). Multidimensional evaluation of virtual reality paradigms in clinical neuropsychology: Application of the VR-check framework. Journal of Medical Internet Research, 22(4), e16724. https://doi.org/10.2196/16724
- Lavenex, P., Boujon, V., Ndarugendamwo, A., & Lavenex, P. (2015). Human short-term spatial memory: Precision predicts capacity. Cognitive Psychology, 77, 1–19. https://doi.org/10.1016/j.cogpsych.2015.02.001
- Luck, S., & Vogel, E. (1997). The capacity of visual working memory for features and conjunctions. Nature, 390(6657), 279–281. https://doi.org/10.1038/36846
- Magen, H., & Emmanouil, T. A. (2019). Estimation in self-initiated working memory for spatial locations. Psychonomic Bulletin & Review, 26(1), 315–324. https://doi.org/10.3758/s13423-018-1514-x
- McShane, R., Gedling, K., Keene, J., Fairburn, C., Jacoby, R., & Hope, T. (1998). Getting lost in dementia: A longitudinal study of a behavioral symptom. International Psychogeriatrics, 10(3), 253–260. https://doi.org/10.1017/S1041610298005365
- Monacelli, A. M., Cushman, L. A., Kavcic, V., & Duffy, C. J. (2003). Spatial disorientation in Alzheimer's disease: The remembrance of things passed. Neurology, 61(11), 1491–1497. https://doi.org/10.1212/WNL.61.11.1491
- Montana, J. I., Tuena, C., Serino, S., Cipresso, P., & Riva, G. (2019). Neurorehabilitation of spatial memory using virtual environments: A systematic review. Journal of Clinical Medicine, 8(10), 1516. https://doi.org/10.3390/jcm8101516
- Morganti, F., Stefanini, S., & Riva, G. (2013). From allo- to egocentric spatial ability in early Alzheimer's disease: A study with virtual reality spatial tasks. Cognitive Neuroscience, 4(3–4), 171–180. https://doi.org/10.1080/17588928.2013.854762
- Morris, R. G., & Mayes, A. R. (2004). Long-term spatial memory: Introduction and guide to the special section. Neuropsychology, 18(3), 403–404. https://doi.org/10.1037/0894-4105.18.3.403
- Mousavi Hondori, H., Khademi, M., Dodakian, L., McKenzie, A., Lopes, C. V., & Cramer, S. C. (2016). Choice of human–computer interaction mode in stroke rehabilitation. Neurorehabilitation and Neural Repair, 30(3), 258–265. https://doi.org/10.1177/1545968315593805
- Murcia-López, M., & Steed, A. (2016). The effect of environmental features, self-avatar, and immersion on object location memory in virtual environments. Frontiers in ICT, 3. https://doi.org/10.3389/fict.2016.00024
- Nasreddine, Z. S., Phillips, N. A., Bédirian, V., Charbonneau, S., Whitehead, V., Collin, I., Cummings, J. L., & Chertkow, H. (2005). The Montreal cognitive assessment, MoCA: A brief screening tool for mild cognitive impairment. Journal of the American Geriatrics Society, 53(4), 695–699. https://doi.org/10.1111/j.1532-5415.2005.53221.x
- Neguț, A., Matu, S.-A., Sava, F. A., & David, D. (2016). Virtual reality measures in neuropsychological assessment: A meta-analytic review. The Clinical Neuropsychologist, 30(2), 165–184. https://doi.org/10.1080/13854046.2016.1144793
- Osterrieth, P. A. (1944). Le test de copie d’une figure complexe; contribution à l’étude de la perception et de la mémoire [Test of copying a complex figure; contribution to the study of perception and memory]. Archives de Psychologie, 30, 206–356.
- Parsons, T. D., & Rizzo, A. A. (2008). Initial validation of a virtual environment for assessment of memory functioning: Virtual reality cognitive performance assessment test. CyberPsychology & Behavior, 11(1), 17–25. https://doi.org/10.1089/cpb.2007.9934
- Pertzov, Y., Dong, M. Y., Peich, M.-C., & Husain, M. (2012). Forgetting what was where: The fragility of object-location binding. PLoS One, 7(10), e48214. https://doi.org/10.1371/journal.pone.0048214
- Plancher, G., Nicolas, S., & Piolino, P. (2008). Virtual reality as a tool for assessing episodic memory. In Proceedings of the 2008 ACM symposium on virtual reality software and technology – VRST ‘08 (pp. 179–182). https://doi.org/10.1145/1450579.1450617
- Plechatá, A., Sahula, V., Fayette, D., & Fajnerová, I. (2019). Age-related differences with immersive and non-immersive virtual reality in memory assessment. Frontiers in Psychology, 10, 1330. https://doi.org/10.3389/fpsyg.2019.01330
- Postma, A., & De Haan, E. H. F. (1996). What was where? Memory for object locations. The Quarterly Journal of Experimental Psychology Section A, 49(1), 178–199. https://doi.org/10.1080/713755605
- Postma, A., Kessels, R. P. C., & Van Asselen, M. (2004). The neuropsychology of object-location memory. In G. L. Allen (Ed.), Human spatial memory: Remembering where (pp. 143–160). Lawrence Erlbaum Associates Publishers.
- Rand, D., Katz, N., & Weiss, P. L. (2007). Evaluation of virtual shopping in the VMall: Comparison of post-stroke participants to healthy control groups. Disability and Rehabilitation, 29(22), 1710–1719. https://doi.org/10.1080/09638280601107450
- Ratan, R., & Hasler, B. S. (2009). Self-presence standardized: Introducing the self-presence questionnaire (SPQ). Proceedings of the 12th annual international workshop on presence.
- Revelle, W. (2021). Psych: Procedures for personality and psychological research. R package version 2.1.9.
- Rey, A. (1941). L’examen psychologique dans les cas d’encéphalopathie traumatique. (Les problems.) [The psychological examination in cases of traumatic encepholopathy. Problems]. Archives de Psychologie, 28, 215–285.
- Riva, G. (2022). Virtual reality in clinical psychology. Reference Module in Neuroscience and Biobehavioral Psychology, 91–105. https://doi.org/10.1016/B978-0-12-818697-8.00006-6
- Riva, G., Wiederhold, B. K., & Mantovani, F. (2019). Neuroscience of virtual reality: From virtual exposure to embodied medicine. Cyberpsychology, Behavior, and Social Networking, 22(1), 82–96. https://doi.org/10.1089/cyber.2017.29099.gri
- Rizzo, A. A., Schultheis, M., Kerns, K. A., & Mateer, C. (2004). Analysis of assets for virtual reality applications in neuropsychology. Neuropsychological Rehabilitation, 14(1–2), 207–239. https://doi.org/10.1080/09602010343000183
- Rodewald, K., Bartolovic, M., Debelak, R., Aschenbrenner, S., Weisbrod, M., & Roesch-Ely, D. (2012). Eine Normierungsstudie eines modifizierten Trail Making Tests im deutschsprachigen Raum [A standardization study of a modified trail making test in German-speaking countries]. Zeitschrift für Neuropsychologie, 23(1), 37–48. https://doi.org/10.1024/1016-264X/a000060
- Rose, F. D., Brooks, B. M., & Rizzo, A. A. (2005). Virtual reality in brain damage rehabilitation: Review. CyberPsychology & Behavior, 8(3), 241–262. https://doi.org/10.1089/cpb.2005.8.241
- Rowe, M. A., Vandeveer, S. S., Greenblum, C. A., List, C. N., Fernandez, R. M., Mixson, N. E., & Ahn, H. C. (2011). Persons with dementia missing in the community: Is it wandering or something unique? BMC Geriatrics, 11(1), 28. https://doi.org/10.1186/1471-2318-11-28
- Ruggiero, G., Sergi, I., & Iachini, T. (2008). Gender differences in remembering and inferring spatial distances. Memory, 16(8), 821–835. https://doi.org/10.1080/09658210802307695
- Schellig, D., & Schächtele, B. (2009). Visueller und verbaler Merkfähigkeitstest [Visual and verbal memory test] (2nd ed.). Hogrefe.
- Serino, S., Morganti, F., Di Stefano, F., & Riva, G. (2015). Detecting early egocentric and allocentric impairments deficits in Alzheimer’s disease: An experimental study with virtual reality. Frontiers in Aging Neuroscience, 7. https://doi.org/10.3389/fnagi.2015.00088
- Sharps, M. J., & Gollin, E. S. (1986). Methods of evaluating performance on spatial memory tasks. Bulletin of the Psychonomic Society, 24(1), 18–20. https://doi.org/10.3758/BF03330491
- Shin, M.-S., Park, S.-Y., Park, S. R., Seol, S.-H., & Kwon, J. S. (2006). Clinical and empirical applications of the Rey-Osterrieth complex figure test. Nature Protocols, 1(2), 892–899. https://doi.org/10.1038/nprot.2006.115
- Siegel, A. L. M., & Castel, A. D. (2018). The role of attention in remembering important item-location associations. Memory & Cognition, 46(8), 1248–1262. https://doi.org/10.3758/s13421-018-0834-4
- Spooner, D., & Pachana, N. (2006). Ecological validity in neuropsychological assessment: A case for greater consideration in research with neurologically intact populations. Archives of Clinical Neuropsychology, 21(4), 327–337. https://doi.org/10.1016/j.acn.2006.04.004
- Tromp, J., Klotzsche, F., Krohn, S., Akbal, M., Pohl, L., Quinque, E. M., Belger, J., Villringer, A., & Gaebler, M. (2020). Openvirtualobjects: An open set of standardized and validated 3d household objects for virtual reality-based research, assessment, and therapy. Frontiers in Virtual Reality, 1. https://doi.org/10.3389/frvir.2020.611091
- van der Ham, I. J. M., Faber, A. M. E., Venselaar, M., van Kreveld, M. J., & Löffler, M. (2015). Ecological validity of virtual environments to assess human navigation ability. Frontiers in Psychology, 6. https://doi.org/10.3389/fpsyg.2015.00637
- van der Ham, I. J. M., van Zandvoort, M. J. E., Meilinger, T., Bosch, S. E., Kant, N., & Postma, A. (2010). Spatial and temporal aspects of navigation in two neurological patients. Neuroreport, 21(10), 685–689. https://doi.org/10.1097/WNR.0b013e32833aea78
- Wells, J. D., Campbell, D. E., Valacich, J. S., & Featherman, M. (2010). The effect of perceived novelty on the adoption of information technology innovations: A risk/reward perspective. Decision Sciences, 41(4), 813–843. https://doi.org/10.1111/j.1540-5915.2010.00292.x
- Weniger, G., Ruhleder, M., Lange, C., Wolf, S., & Irle, E. (2011). Egocentric and allocentric memory as assessed by virtual reality in individuals with amnestic mild cognitive impairment. Neuropsychologia, 49(3), 518–527. https://doi.org/10.1016/j.neuropsychologia.2010.12.031
- White, P. J. F., & Moussavi, Z. (2016). Neurocognitive treatment for a patient with Alzheimer's disease using a virtual reality navigational environment. Journal of Experimental Neuroscience, 10, JEN.S40827. https://doi.org/10.4137/JEN.S40827
- Wilson, B. A., Greenfield, E., Clare, L., Baddeley, A., Cockburn, J., Watson, P., Tate, R., Sopena, S., & Nannery, R. (2008). The rivermead behavioural memory test (3rd ed). Pearson Assessment.
- Witmer, B. G., & Singer, M. J. (1998). Measuring presence in virtual environments: A presence questionnaire. Presence: Teleoperators and Virtual Environments, 7(3), 225–240. https://doi.org/10.1162/105474698565686
- Zimmer, H. D., Speiser, H. R., & Seidler, B. (2003). Spatio-temporal working-memory and short-term object-location tasks use different memory mechanisms. Acta Psychologica, 114(1), 41–65. https://doi.org/10.1016/S0001-6918(03)00049-0