ABSTRACT
Patients with developmental amnesia (DA) have suffered hippocampal damage in infancy and subsequently shown poor episodic memory, but good semantic memory. It is not clear how patients with DA learn semantic information in the presence of episodic amnesia. However, patients with DA show good recognition memory and it is possible that semantic learning may be supported by recognition. Building on previous work, we compared two methods for supporting semantic learning in DA; recognition-learning and recall-learning. In each condition, a patient with DA (aged 8 years) was presented with semantic information in animated videos. After each presentation of a video, learning was supported by an immediate memory test. Two videos were paired with a cued recall test. Another two videos were paired with a multiple-choice test to enable recognition-based learning. The outcome measure was semantic recall performance after a short delay of 30 min and a long delay of one week. Results showed a benefit of recognition-learning compared to recall-learning on cued recall in the patient with DA (76% vs. 35%). This finding indicates that young people with severe hippocampal damage can utilize recognition to support semantic learning. This has implications for the support of school-aged children with episodic memory difficulties.
Introduction
Children with developmental amnesia (DA) have suffered a hippocampal injury in early life. Although there are a range of aetiologies, a common cause is a traumatic birth and asphyxia causing a hypoxic-ischemic injury in the neonatal period (Gadian et al., Citation2000; Gilboa et al., Citation2006; Picard et al., Citation2013; Vargha-Khadem et al., Citation1997; Vicari et al., Citation2007). After recovery from the initial trauma, early development appears normal. Children with DA meet the expected developmental milestones in infancy and early childhood for motor, language and cognitive development (Bindschaedler et al., Citation2011; Jonin et al., Citation2018; Vargha-Khadem et al., Citation1997) However, difficulties with memory emerge during the preschool years (Cooper et al., Citation2015). Parents and caregivers notice that the child does not remember events that they are expected to remember, including birthday parties and memorable occasions (Bindschaedler et al., Citation2011; Jonin et al., Citation2018; Vargha-Khadem et al., Citation1997). They cannot provide a reliable account of the day’s activities, remember conversations, retell stories etc. Children with DA often lose their belongings and can get lost in familiar surroundings (Rosenbaum et al., Citation2015; Vargha-Khadem et al., Citation1997). These memory difficulties interfere with daily living to the extent that children and adolescents with DA cannot gain independence commensurate with their age and aspirations. They depend heavily on support from family members and teachers.
A fascinating feature of developmental amnesia is that semantic memory (that is, memory for general knowledge retrieved independently of a specific context) is spared (for review, see Elward & Vargha-Khadem, Citation2018). Children with DA have age-appropriate world knowledge that they can express through their well-developed speech and language abilities. In fact, one report indicates that semantic memory in adults with a history of DA can be superior to that of matched controls (Jonin et al., Citation2018 see also Blumenthal et al., Citation2017). Semantic memory is a strength that can be used to compensate for some aspects of the developmental amnesic syndrome. For example, if the child cannot recall a specific event, they may use their semantic memory to guess at an appropriate response (Brandt et al., Citation2006).
Despite this strength, children with DA struggle to keep up with peers in education. In laboratory studies, patients with DA take longer to learn semantic information than controls (Baddeley et al., Citation2001; Gardiner et al., Citation2008). It’s likely that typically developing children use their episodic memory (viz. autonoetic memory of their life experiences) to recall previous lessons and learning episodes and use this to support newly-learned information before it is consolidated into the semantic system. Patients with DA do not have access to episodic memory and so are at a considerable disadvantage when learning new information. As a result, young people with DA find school a struggle and fall behind their peers. In secondary education and beyond they tend to take up vocational skills training instead of formal education, even if this is not in-line with their interests and cognitive abilities. Anecdotally, young people with DA fail to thrive in education and do not find fulfilling employment in adulthood.
This patient group has inspired scientific research; however, the academic community has not been able to develop rehabilitative techniques that can support children with DA to reach their potential in education and employment. Some techniques that originally seemed promising (i.e., the fast-mapping technique) have failed to support learning in DA (Elward et al., Citation2019). From the research that has been conducted, three conclusions can be drawn about the optimum conditions for semantic learning in DA. The first of these findings is that patients with DA will benefit from repeated presentations of the study material. Baddeley et al., (Citation2001) demonstrated that when patient Jon was shown four presentations of previously unfamiliar newsreel footage, he performed as well as controls in an immediate recall test, however, underperformed relative to controls in an overnight recall test. Second, patients seem to benefit from consolidation over time, or rather, healthy controls show more forgetting over time than patients, and therefore testing memory after a delay of several days or weeks will show smaller group differences than testing on the same day (Elward & Vargha-Khadem, Citation2018; Gardiner et al., Citation2008). Lastly, patients with DA perform well on recognition memory tests. That is, when given a multiple-choice test in which the patient only needs to recognize the familiar option from a list of alternatives, then patients with DA perform as well as their peers (Adlam et al., Citation2009; Baddeley et al., Citation2001). However, when given a free recall test, in which the patient must bring back to mind the relevant information from memory, then patients with DA markedly underperform (Baddeley et al., Citation2001; Gardiner et al., Citation2006). This last finding may be crucial for supporting learning in patients with DA. It demonstrates that patients with DA show some evidence of semantic learning but that the memory is accessible to recognition only (Elward & Vargha-Khadem, Citation2018, Vargha-Khadem et al. Citation2006). The key to promoting semantic learning in DA may be to consider how this nascent memory may be strengthened so that patients can retrieve the information that they have learned through recall.
In our review, we reported two unpublished datasets suggesting that testing through recognition can indeed support semantic retrieval in DA (Elward & Vargha-Khadem, Citation2018). In both studies, patients with DA and controls were asked to learn semantic information in the form of short narratives. The information was presented six times, and after each presentation, the patient underwent an immediate memory test intended to support the long-term retention of the new material. The key difference between the two studies was the type of memory test that was used to support learning: A recall test or a recognition test.
In the first experiment, participants completed an immediate recall test after each presentation of the semantic information. In this condition, the patients underperformed relative to controls on all the learning trials and the delayed recall test (see : Recall Learning).
Figure 1. Reproduced from Elward and Vargha-Khadem (Citation2018). Data from two studies designed to investigate semantic learning in DA. Recall Learning (top panel): Participants complete six recall tests during learning. The cued recall test (highlighted with a box) indicates that participants did not show good learning with this method. Recognition Learning (bottom panel): Participants complete recognition tests during learning. In this case, performance on the cued recall test (highlighted with a box) was similar to controls.
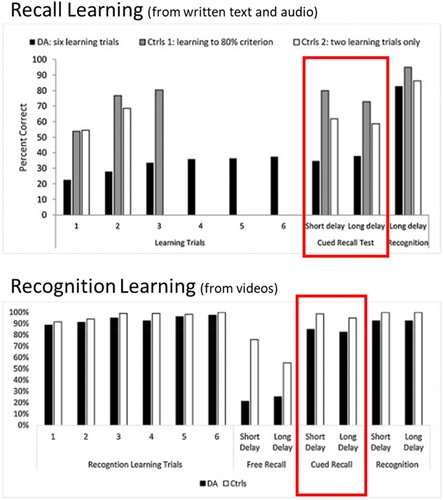
The second experiment (see , Recognition learning) was conducted several years later. This time, the participants learned semantic information from videos instead of text, but the same semantic information was used. After each presentation of the video, participants completed a recognition test (i.e., a multiple-choice test). As expected from the typical profile of DA, the patients performed well on these recognition tests. The true test of learning occurred one week later in a delayed test. In the recognition learning experiment, patients with DA were able to recall the semantic information at a level comparable to controls in the cued recall test. In fact, patients recalled twice as much information in this condition as in the previous version of the experiment. We interpret that having repeated opportunities to recognize the correct information during the learning strengthened the semantic memory and facilitated recall.
This finding is encouraging but must be interpreted with caution. Some of the same patients participated in both the recall learning experiment and the recognition learning test several years later. Therefore, it is possible that participants had consolidated the information from the recall learning experiment in the intervening years and if so, this would explain their improved performance on the later test. Furthermore, the first experiment used written materials and audio recordings, so learning the semantic information relied only on auditory/phonological processing, but the second experiment used videos which contained visual images too. It’s not possible to determine if recognition testing is driving the new semantic learning, or if the addition of visual stimuli is key. In addition, the DA patients were adults at the time of the recognition learning experiment and so it’s not clear that this method would support learning in school age children where it could have the biggest impact on education and life success.
In order to test the hypothesis that recognition-based learning will facilitate semantic learning in children with DA, here we report a direct comparison between the two learning methods: recognition learning and recall learning. A child with DA (aged 8) encountered new semantic information (presented via videos) for the first time. Across three weeks of testing, two videos were presented in the recognition learning condition (where learning is supported with multiple-choice tests), and two were presented in the recall learning condition (where learning is supported with open-ended questions). The outcome measure is cued recall performance after a delay of one week.
Materials and methods
Participants
Case presentation
Patient H, an eight-year-old boy, was referred by his General Practitioner to a Consultant Neuropsychologist (FVK) at Great Ormond Street Hospital NHS Foundation Trust due to concerns about his “excessive forgetfulness.”
During the clinical interview, Patient H’s mother reported that her son had a complicated birth involving meconium aspiration and asphyxia. Shortly after birth, he was transferred to intensive care where he was ventilated for three days, and then remained in hospital for two weeks. Following this stormy period, Patient H’s condition improved and at discharge he showed no neurological symptoms. Patient H met his developmental milestones as expected. His mother described him as an easy baby who did not raise any concerns until the age of three when she noticed his difficulties remembering daily events. These concerns became more prominent when Patient H started school and had difficulty settling in; he was distressed, could not remember where his mother was and asked to go home. In school, he could not follow the teacher’s instructions and soon started to fall behind his peers. Teachers shared his mother’s concerns about his memory. Apart from the memory difficulties, Patient H’s mother does not report any other cognitive difficulties or behavioural problems. She describes her son as a healthy, bright, and sociable boy who has good general knowledge and language skills. She remarked that some aspects of Patient H’s memory seem to be working well as he had learned the names of many Pokémon characters despite his amnesia.
Neuropsychological assessment
Patient H was assessed on test of intelligence, vocabulary, and a range of memory tests that include measures of recall, recognition, short-term, and long-term memory in visual and verbal domains (see : Neuropsychological Assessment Results).
Table 1. Neuropsychological assessment results of patient H.
Brain volume measurements
A T1-weighted MRI scan (flip angle of 8°, field of view = 25.6 cm, repetition time = 2300 msec, and 1 mm isotropic voxels) was acquired on a Siemens 3-T Prisma scanner equipped with a 32-channel receiver head coil at Great Ormond Street Hospital for Children NHS Foundation Trust, London, UK. The clinical neuroradiology report indicated that the hippocampi were small and the mamillary bodies were visible, but atrophied. No other abnormalities were identified.
Manual segmentation of the MRI acquisitions was used to estimate the volume of the hippocampus as a whole and several subregions, namely the uncus, CA-DG (including CA fields and dentate gyrus) and subicular complex (including subiculum, presubiculum and parasubiculum). These subregions are plotted alongside those obtained from the group of thirty-two controls shown in (Chareyron et al., Citation2023). The hippocampus was reduced by 34% of normal. We have previously reported that early hippocampal pathology leads to the memory impairments characteristic of Developmental Amnesia when the volume of this structure is reduced below normal by about 20% to 30% on each side (Isaacs et al. Citation2003, Cooper et al., Citation2015). Crawfords one-sample t-tests indicated that Patient H’s hippocampus was significantly smaller than the control group (t(32) = −3.18, p < 0.005). When the subregions of the hippocampus are compared, Patient H shows reduced volumes of the subicular complex (t (31) = 4.16, p < 0.001) and the CA-DG region (t(32) = −2.46, p < 0.01) but the volume of the uncus was not significantly reduced compared to controls (t(32) = 0.70, n.s.)
Figure 2. Manual segmentation of the hippocampus. Top: 3T-MRI scan of a 14-year-old male control participant (left) alongside Patient H (right) showing the reduced volume of the subicular complex (in green) and CA-DG region in (orange). Bottom. ICV-corrected hippocampal volumes for Patient H compared with 32 healthy controls (8y – 38y; 16male) indicating a 34% volume atrophy of the hippocampus.
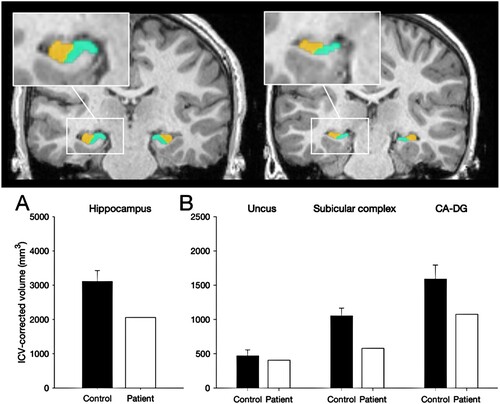
Typically developing controls
Five control children (1 male) between ages of 8 and 10 years were recruited via an opportunity sample through the local community at UCL and LSBU. The inclusion criteria were that children must be aged between 7 and 10 years of age, having no major medical event at birth, generally healthy with no medical conditions and no learning difficulties.
Control children participated in a short neuropsychological assessment consisting of the Weschler Abbreviated Scale of Intelligence (WASI-II) and the Children’s Memory Scale. The five controls performed within the normal range on all subtests of each test. Full-scale IQ ranged from 112 to 134 (median 128). Crawfords one-sample t-tests were performed on every subtest of the IQ test and the CMS to establish whether the patient significantly differed from controls. There were no significant differences in the IQ measures, but the patient significantly underperformed relative to controls on five of the CMS subscales (see ).
Table 2. Crawford’s Bayesian test for single case assessment (Crawford & Garthwaite, Citation2007) to identify significant differences between patient to the controls on IQ and CMS test scores.
The research was overseen by the Research and Development Department of Great Ormond Street Hospital for Children NHS Foundation Trust, and the UCL Great Ormond Street Institute of Child Health, London, UK. The project was approved by the Hampstead NHS Research Ethics Committee and was also approved by the Ethics committee of School of Applied Sciences, London South Bank University. Testing occurred in a laboratory space at the Wolfson Centre laboratories at the UCL Great Ormond Street Institute of Child Health or in the Child Development Laboratory at London South Bank University. Each child was accompanied to the testing sessions by a parent who provided informed consent and waited in a nearby waiting room during testing. The child was given an age-appropriate description of the experiment and asked for assent. At the end of the study, each child compensated with a £15 gift voucher and a factsheet about the brain to thank them for their time and effort.
Materials
As described in Elward and Vargha-Khadem (Citation2018), four videos were created that contain semantic information on a particular topic. The topics were Egyptians, Mistletoe, Vikings, and Ex-Presidents. Each topic concerns a semantic construct that would be familiar to children (e.g., children understand the concept of Ancient Egyptians) but crucially, the videos contain novel information that could be incorporated into that semantic construct (e.g., Many years before the Pyramids were built, the Egyptian people were nomads). Each video contained narration that was comparable in word length (189–190 words) and reading level (Flesch Reading Ease score ranged from 60.9 to 64.2). A complete transcript of each of the videos and the 20 memory test questions are provided in supplementary materials. This text was animated with cartoon drawings to produce four videos which ranged in length from 72-84seconds. Two videos were presented under the recall-based learning condition and two were presented in the recognition-based learning condition.
Design & procedure
An overview of the experimental design is provided in . The experiment was designed to compare two learning conditions while controlling for order effects. To achieve this, each child attended the laboratory three times. Each visit was spaced one week apart. Participants learned two videos per session. There was a short delay memory test in the same session and a delayed memory test the following week. In the third session, children completed a neuropsychological assessment. Each appointment lasted approx. 2.5 h.
Table 3. Overview of the test procedures over three weeks.
Session 1
The first video was presented in the recall learning condition. The video was presented on a laptop computer. After the video, the child was presented with a recall test. This test consisted of open-ended questions such as “What sort of machine was discovered in the Viking home?” Twenty such questions were presented on PowerPoint slides. The child responded verbally, and the researcher recorded the child’s answer on a response sheet then moved the presentation to the next slide. There was no time limit for responding. The researcher encouraged the child to guess if they weren’t sure of the answer. No feedback was provided. After the test was complete, the same video was played again. Six study-test cycles were completed (See : Recall-Based learning). After the six learning trials, there was a 15-minute break before the short delay test.
Figure 3. Schematic of the protocols.
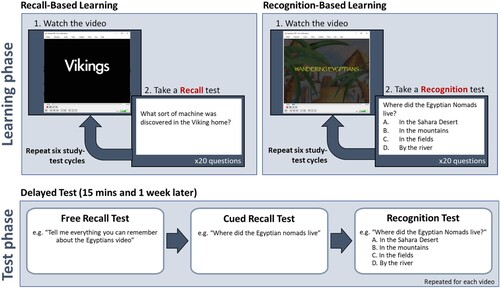
The short-delayed test had three stages. First, there was a free-recall test, in which the child was asked to recall anything they could remember about the video. Second, there was a cued recall test in which the child was asked the same 20 questions that they were asked in the recall learning phase. Finally, there was a recognition test where the same 20 questions were presented again but with multiple-choice response options (e.g., “What sort of machine was discovered in the Viking home?" (a) Stove, (b) A Loom, (c) A Plough, (d) A set of scales. See : Test Phase). This was followed by a self-paced break.
The second video was presented in the recognition learning condition (See : Recognition-Based learning). The recognition learning condition is identical to the recall learning condition except that after each presentation of the video the child is presented with a multiple-choice test instead of a recall test. The questions were presented on PowerPoint slides. The researcher read out the questions and response options. The child responded verbally at their own pace and was encouraged to guess if they were not sure. The researcher recorded the child’s responses. After six learning trials, there was a 15-minute break followed by a short-delay test which followed the same procedure as in the recall learning condition; first there was a free recall test, then a cued recall test and then a recognition test.
Figure 4. Mean memory performance across the two learning conditions. Error bars indicate 1+/- the standard error of the mean. The outcomes of the case-control statistical tests are indicated with symbols, *p < 0.05, **p < 0.01, ***p < 0.001.
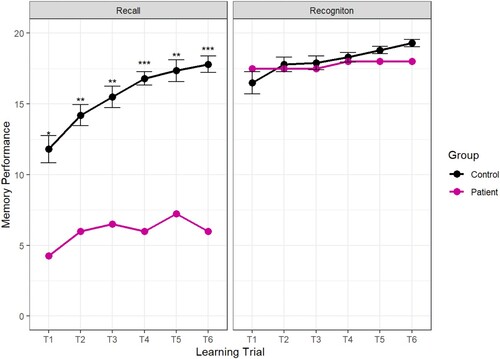
Session 2
One week later, the child returned to the lab for the second session. At the start of this session, the child was tested on the material that they learned in the previous week. This is the 1-week delayed test and follows the same protocol as the short delay test. The test started with the free recall test, then a cued recall test and then a recognition test.
Then the child was asked to learn information contained in two more videos. The order of the learning conditions was counterbalanced so that the child learned the third video via recognition learning and the fourth video via recall learning. This is the reverse of the order in week 1 where the recall learning condition came first. Apart from the change in order, the procedure for the learning conditions and tests is identical to week 1.
To ensure that the controls undergo the same protocols as Patient H the videos are taught in the same order (Video 1 = Vikings, Video 2 = Mistletoe, Video 3 = Egyptians, Video 4 = Presidents).
Session 3
In the third session, the child completed a 1-week delayed test for information that was learned in the second session. Lastly, control children completed a neuropsychological assessment which included the WAIS-II and the Children’s Memory Scale.
Data analysis
All data analysis and visualization were conducted in R (a programming language for statistical computing and graphics). The code and data are available on the open science framework (https://osf.io/ks3mq). Performance was averaged together to give a mean score for memory performance in the recall learning condition (videos 1 & 4) and the recognition learning condition (videos 2 & 3). The single-case data are compared to controls using a Bayesian test for single-case analysis (Crawford & Garthwaite, Citation2007) using the “psycho” package for R (Makowski, Citation2018).
Results
Learning trials
During the learning trials, Patient H underperformed relative to controls on all recall tests but performed well on the recognition tests. The results are plotted in .
As well as underperforming on the recall test, the pattern of responses of Patient H was remarkably inconsistent. Rather than gaining knowledge incrementally through repeated presentations of the material, Patient H remembered different answers on each trial. For example, to the question “What kind of Viking home has recently been discovered?” Patient H recalled “a farm” correctly on the first two learning trials but did not recall that detail again for the rest of the test. In response to the question, “how did the weather change when the Vikings lived in Greenland?” Patient H was alternated his response over the six learning trials so that he recalled “it got hotter” and “it got colder” three times each. However, he always correctly recalled that the animals were brought inside to protect them from the cold, indicating some memory that cold weather became an issue for the Viking settlers. Generally, the pattern of responses indicates that Patient H had a qualitatively different memory of the learning material to the typically developing children. This pattern is similar to the inter-trial forgetting observed by (Gardiner et al., Citation2008).
15-Minute delayed test
In the 15-minute delayed test, there was no clear effect of learning condition. Patient H underperformed relative to controls in the Free Recall and the Cued Recall tests but performed well in the recognition memory test (see ).
Figure 5. Memory performance in the 15-Minute Delayed Test following recall learning (left panel) and recognition learning (right panel). The outcomes of the case-control statistical tests are indicated with symbols, *p < 0.05, **p < 0.01, ***p < 0.001.
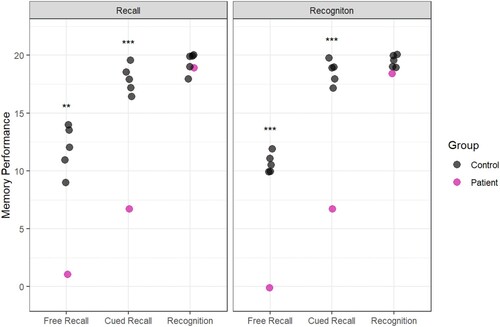
1-Week delayed test
The crucial test was conducted one week later when we assessed whether recall-based learning or recognition-based learning was associated with better performance after a long delay. The results show that Patient H was able to recall more than twice as much information that was presented via recognition-based learning (15.25) than recall-based learning (7.00). Importantly, this was true when the learning was assessed with a cued recall test, indicating that recognition during learning facilitated the formation of a memory that could be recalled after a delay ().
Figure 6. Memory performance in the 1-week Delayed Test. The outcomes of the case-control statistical tests are indicated with symbols, *p < 0.05, **p < 0.01, ***p < 0.001.
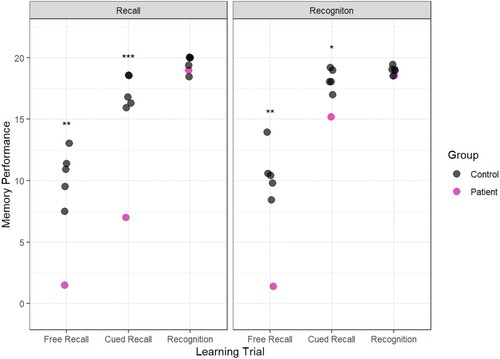
To compare learning across the two conditions, a difference score was computed between Patient H’s score in the cued recall test and each of the controls. In the recall testing condition, Patient H scored an average of 10.2 points lower than the control group (Standard Deviation = 1.2) but in the recognition testing condition, Patient H scored only 3 points below the control group (Standard Deviation = 0.90). A Revised Standard Difference Test (Crawford & Garthwaite, Citation2005) confirmed Patient H’s performance was significantly reduced in the recognition learning condition compared to the recall learning condition t (4) = 2.96, p < 0.05.
Discussion
These results build on the findings from Limond and colleagues that recognition testing can support semantic learning in DA (as reported in Elward & Vargha-Khadem, Citation2018). The previous studies showed impressive learning in adults with DA following repeated recognition testing, however, the interpretation of the previous findings was limited by changes in methodology across the experiments. Here, the methodology was controlled to allow a direct comparison of recognition testing and recall testing. The paradigm was employed with a patient and controls who had not been exposed to this material before. Although this is a single case of DA, it provides evidence that recognition testing can support semantic learning in children with DA.
In the introduction, we posited that the key to supporting semantic learning in DA may be to consider how a new semantic memory can be strengthened so that it is available for delayed recall. One mechanism by which new memories are strengthened and stabilized into semantic memory is via reconsolidation (Dudai, Citation2004, Citation2012; Squire et al., Citation2015). In typically developing people, new declarative memories are thought to be labile and vulnerable to forgetting (Squire et al., Citation2015). Reconsolidation occurs when the memory trace is reactivated (or replayed during sleep). Each reactivation of the prior memory is thought to create a new memory representation in the cortex which overlaps with the original memory (Nadel & Moscovitch, Citation1997). The process of consolidation enables commonalities across events to be extrapolated and an acontextual semantic memory to form (Winocur & Moscovitch, Citation2011). This acontextual memory is more stable and resistant to forgetting than the episodic memory for the individual learning events.
Reactivation and consolidation are thought to be crucially dependent on the hippocampal complex which is severely compromised in patients with DA (Squire et al., Citation2015). However, recent research from our laboratory indicates that patients with DA can reactivate prior events in memory even when that information is unavailable to recall (Elward et al., Citation2021). This is consistent with several other accounts that have demonstrated that hippocampal processes may not be strictly necessary for reinstatement of a prior event in memory (Gagnon et al., Citation2019) and that reinstatement can occur without the phenomenological experience of “remembering” the prior event (Thakral et al., Citation2017). If hippocampal processing and episodic memory is not crucial for the reinstatement of a prior learning event, then patients with DA may make use of reconsolidation to strengthen semantic memories even without conscious memory of the previous event. Further research combining reinstatement and semantic learning in patients with DA may test this hypothesis.
The phenomenon that retrieval practice supports learning has been referred to as “the testing effect” (Roediger & Butler, Citation2011). In typically-developing people, active and elaborative re-processing of newly learned information, such as taking a revision test, is associated with better performance than restudying the information. This is because the process of memory retrieval is thought to support reconsolidation. However, the type of retrieval test is important for testing to facilitate learning. In research with typically-developing young adults, taking a multiple-choice revision test did not support learning any more than restudying the information (Kang et al., Citation2007). The benefit of testing only became apparent with more elaborative tests, including short answer questions. Kang and colleagues interpret that the more demanding the retrieval process, the greater the benefit to learning. Unfortunately, elaborative tests and short answer questions are too demanding for patients with DA and are ineffective for learning in this patient group. Short answer questions require recall and patients with DA have particular difficulty with recall which is dependent on the integrity of the hippocampus (Patai et al., Citation2015). Patients with DA, however, have a remarkably preserved ability to recognize familiar items (Adlam et al., Citation2009). The data presented here indicate that recognition may be used to support semantic consolidation in this patient group. Moreover, our findings can be interpreted within the hierarchical model of memory whereby episodic memory and recall are crucially dependent on the hippocampus, but recognition memory and semantic memory are supported by cortical structures (Mishkin et al., Citation1997). Learning in DA is optimal when patients can utilize cortical processing of the learned information (i.e., recognition) and avoid processing the information via the episodic memory system (i.e., recall) which is damaged in patients with DA.
This report is not the first to demonstrate that semantic processing of studied material benefits learning in DA. In a previous study with patient Jon, an adult patient with DA, a more elaborative, semantic encoding strategy (rating the words for their pleasantness) was associated with better memory performance than perceptual processing (counting the number of syllables in the words) (Gardiner et al., Citation2006). Therefore, patients with DA may benefit from semantic processing of the studied material in multiple-choice tests. This is more demanding than passive re-exposure to the studied materials, but not so demanding that it is impossible for the patients to succeed with the test (viz recall testing or short answer questions).
Interestingly, there was no benefit of recognition testing in the 15-minute delayed test (see ). The benefits of recognition testing emerged after one one-week delay. This is consistent with previous research indicating that consolidation can aid semantic learning in typically-developing people (see Squire et al., Citation2015). However, previous research in DA indicates that sleep consolidation had no improvement in learning semantic from news clips (Baddeley et al., Citation2001) or semantic facts from the encyclopaedia (Gardiner et al., Citation2008). In fact, the key finding from a review of the literature is that typically-developing people tend to forget some newly acquired semantic information over a delay, but patients with DA tend to retain the little information that they have learned. In this report, however, we saw memory improve over time, presumably due to a consolidation mechanism that took place over the delay. Similarly, Green et al. (Citation2014) report that a spaced learning technique improved paired-associate memory in a patient with DA. The mechanism of spaced learning is unclear, but one suggestion is that spaced learning promotes consolidation. More research is needed to understand the mechanism by which consolidation supports learning in amnesia.
We also reported some qualitative observations of learning in our patient with DA. We described that he did not learn information incrementally such that he maintained some information that he had learned on previous trials and added additional information with more learning experiences. Instead, we report a highly variable set of responses where some information was forgotten, and other information was recalled on each recall learning trial. A similar pattern can be seen in the CAVLT-2 (see : Neuropsychological Assessment) where Patient H fails to learn new items on each learning trial but consistently recalls around 5 items each time. This pattern is not seen in typically developing people. Gardiner et al. (Citation2008) described this phenomenon as “intertrial-forgetting” and demonstrated that controls rarely forgot items between learning trials, but this was significantly more common in the DA patient, Jon. Gardiner et al. interpret that this indicates that the control participants have a greater ability to use episodic memory to scaffold semantic learning. Therefore, although semantic memory is a strength in individuals with DA, the learning of new semantic information is inconsistent and piecemeal. Children with DA experience learning in a qualitatively different way to typically developing people and must be supported differently in order to succeed in education.
Although encouraging, these results should be interpreted with caution. We report a single-case study in which a child who is struggling in education shows a clear benefit to learning from recognition testing. However, as this is a single-case, more research should be conducted to establish that this pattern holds in other children with DA. Furthermore, research with such patients should be conducted over longer delays than one week and in more ecologically valid conditions (e.g., a classroom environment). We report that the patient’s memory improved markedly in the recognition testing condition, and we also reported that his learning deficit compared to a control group was reduced. This comparison to the control group should also be treated with caution as our control group was a small opportunity sample that was not tightly matched to the patient. The controls were mostly girls and were slightly older than the patient (controls ranged from 8 to 10 years, the patient was aged 8). Therefore, although the patient’s memory scores improved during recognition testing, it is not clear how his performance would compare to boys of his own age.
At the outset of this paper, we described that children with DA have learning difficulties that impact their education and limit their ability to live independent lives. One goal of our work has been to provide evidence-based approaches to supporting children with DA in education. An early diagnosis of DA is crucial to establish the pattern of learning difficulty that is characterized by the disorder at the outset of formal education. That is, a pattern of poor episodic memory and recall in the presence of good general cognitive ability, age-appropriate vocabulary, and recognition. Following the diagnosis, educational professionals have a requirement to produce a needs-driven plan to support each individual child’s education. This plan may include evidence-based interventions or reasonable adjustments to classroom teaching to support children with this type of learning difficulties. The data reported here indicate that multiple choice tests can have a large effect on learning outcomes in children with DA. Multiple choice tests and quizzes can be readily incorporated into the classroom environment and are widely available in revision guides and educational websites. Once an appropriate test has been identified, the child may take the same test multiple times, and this recognition practice may support learning and improve the child’s ability to keep up with peers in the classroom. Educational professionals who are supporting a child with amnesia may choose to make this reasonable adjustment to homework or classroom quizzes so that children with DA can participate more fully in education.
NSL_Paper_SupplementaryMaterials.docx
Download MS Word (22.1 KB)Disclosure statement
No potential conflict of interest was reported by the author(s).
Data availability
Data and analysis code are available on the Open Science Framework. https://doi.org/10.17605/OSF.IO/KS3MQ.
Additional information
Funding
References
- Adlam, A. L. R., Malloy, M., Mishkin, M., & Vargha-Khadem, F. (2009). Dissociation between recognition and recall in developmental amnesia. Neuropsychologia, 47(11), 2207–2210. https://doi.org/10.1016/j.neuropsychologia.2009.01.038
- Baddeley, A., Vargha-Khadem, F., & Mishkin, M. (2001). Preserved recognition in a case of developmental amnesia: Implications for the acquisition of semantic memory? Journal of Cognitive Neuroscience, 13(3), 357–369. https://doi.org/10.1162/08989290151137403
- Bindschaedler, C., Peter-Favre, C., Maeder, P., Hirsbrunner, T., & Clarke, S. (2011). Growing up with bilateral hippocampal atrophy: From childhood to teenage. Cortex, 47(8), 931–944. https://doi.org/10.1016/j.cortex.2010.09.005
- Blumenthal, A., Duke, D., Bowles, B., Gilboa, A., Rosenbaum, R. S., Köhler, S., & McRae, K. (2017). Abnormal semantic knowledge in a case of developmental amnesia. Neuropsychologia, 102(January), 237. https://doi.org/10.1016/j.neuropsychologia.2017.06.018
- Brandt, K. R., Gardiner, J. M., Vargha-Khadem, F., Baddeley, A. D., & Mishkin, M. (2006). Using semantic memory to boost ‘episodic’ recall in a case of developmental amnesia. Neuroreport, 17(10), 1057–1060. https://doi.org/10.1097/01.wnr.0000220134.09221.04
- Chareyron, L. J., Chong, W. K. K., Banks, T., Mishkin, M., Burgess, N., Saunders, R. C., & Vargha-Khadem, F. (2023). Greater hippocampal atrophy in developmental amnesia is associated with better recall and working memory performance. BioRxiv, https://doi.org/10.1101/2023.01.23.525152
- Cooper, J. M., Gadian, D. G., Jentschke, S., Goldman, A., Munoz, M., Pitts, G., Banks, T., Chong, W. K., Hoskote, A., Deanfield, J., Baldeweg, T., de Haan, M., Mishkin, M., & Vargha-Khadem, F. (2015). Neonatal hypoxia, hippocampal atrophy, and memory impairment: Evidence of a causal sequence. Cerebral Cortex, 25(6), 1469–1476. https://doi.org/10.1093/cercor/bht332
- Crawford, J. R., & Garthwaite, P. H. (2005). Testing for suspected impairments and dissociations in single-case studies in neuropsychology: Evaluation of alternatives using Monte Carlo simulations and revised tests for dissociations. Neuropsychology, 19(3), 318–331. https://doi.org/10.1037/0894-4105.19.3.318
- Crawford, J. R., & Garthwaite, P. H. (2007). Comparison of a single case to a control or normative sample in neuropsychology: Development of a Bayesian approach. Cognitive Neuropsychology, 24(4), 343–372. https://doi.org/10.1080/02643290701290146
- Dudai, Y. (2004). The neurobiology of consolidations, or, how stable is the engram? Annual Review of Psychology, 55(1), 51–86. https://doi.org/10.1146/annurev.psych.55.090902.142050
- Dudai, Y. (2012). The restless engram: Consolidations never end. Annual Review of Neuroscience, 35(1), 227–247. https://doi.org/10.1146/annurev-neuro-062111-150500
- Elward, R. L., Dzieciol, A. M., & Vargha-Khadem, F. (2019). Little evidence for fast mapping in adults with developmental amnesia. Cognitive Neuroscience, 10(4), 215–217. https://doi.org/10.1080/17588928.2019.1593123
- Elward, R. L., Rugg, M. D., & Vargha-Khadem, F. (2021). When the brain, but not the person, remembers: Cortical reinstatement is modulated by retrieval goal in developmental amnesia. Neuropsychologia, 154, 107788. https://doi.org/10.1016/j.neuropsychologia.2021.107788
- Elward, R. L., & Vargha-Khadem, F. (2018). Semantic memory in developmental amnesia. Neuroscience Letters, 680(October 2017), 23–30. https://doi.org/10.1016/j.neulet.2018.04.040
- Gadian, D. G., Aicardi, J., Watkins, K. E., Porter, D. A., Mishkin, M., & Vargha-Khadem, F. (2000). Developmental amnesia associated with early hypoxic-ischaemic injury. Brain, 123(Pt 3), 499–507. https://doi.org/10.1093/brain/123.3.499
- Gagnon, S. A., Waskom, M. L., Brown, T. I., & Wagner, A. D. (2019). Stress impairs episodic retrieval by disrupting hippocampal and cortical mechanisms of remembering. Cerebral Cortex, 2947–2964. https://doi.org/10.1093/cercor/bhy162
- Gardiner, J. M., Brandt, K. R., Baddeley, A. D., Vargha-Khadem, F., & Mishkin, M. (2008). Charting the acquisition of semantic knowledge in a case of developmental amnesia. Neuropsychologia, 46(11), 2865–2868. https://doi.org/10.1016/j.neuropsychologia.2008.05.021
- Gardiner, J. M., Brandt, K. R., Vargha-Khadem, F., Baddeley, A., & Mishkin, M. (2006). Effects of level of processing but not of task enactment on recognition memory in a case of developmental amnesia. Cognitive Neuropsychology, 23(6), 930–948. https://doi.org/10.1080/02643290600588442
- Gilboa, A., Winocur, G., Rosenbaum, R. S., Poreh, A., Gao, F., Black, S. E., Westmacott, R., & Moscovitch, M. (2006). Hippocampal contributions to recollection in retrograde and anterograde amnesia. Hippocampus, 16(11), 966–980. https://doi.org/10.1002/hipo.20226
- Green, J. L., Weston, T., Wiseheart, M., & Rosenbaum, R. S. (2014). Long-term spacing effect benefits in developmental amnesia: Case experiments in rehabilitation. Neuropsychology, 28(5), 685–694. https://doi.org/10.1037/neu0000070
- Isaacs, E. B., Vargha-Khadem, F., Watkins, K. E., Lucas, A., Mishkin, M., & Gadian, D. G. (2003). Developmental amnesia and its relationship to degree of hippocampal atrophy. Proceedings of the National Academy of Sciences, 100(22), 13060–13063. http://dx.doi.org/10.1073/pnas.1233825100
- Jonin, P.-Y., Besson, G., La Joie, R., Pariente, J., Belliard, S., Barillot, C., & Barbeau, E. J. (2018). Superior explicit memory despite severe developmental amnesia: In-depth case study and neural correlates. Hippocampus, https://doi.org/10.1002/hipo.23010
- Kang, S. H. K., McDermott, K. B., & Roediger, H. L. (2007). Test format and corrective feedback modify the effect of testing on long-term retention. European Journal of Cognitive Psychology, 19(4–5), 528–558. https://doi.org/10.1080/09541440601056620
- Makowski, D. (2018). The psycho package: An efficient and publishing-oriented workflow for psychological science. The Journal of Open Source Software, 3(22), 470. https://doi.org/10.21105/joss.00470
- Mishkin, M., Suzuki, W. A., Gadian, D. G., & Vargha-Khadem, F. (1997). Hierarchical organization of cognitive memory. Philosophical Transactions of the Royal Society of London. Series B: Biological Sciences, 352(1360), 1461–1467. https://doi.org/10.1098/rstb.1997.0132
- Nadel, L., & Moscovitch, M. (1997). Memory consolidation, retrograde amnesia and the hippocampal complex. Current Opinion in Neurobiology, 7(2), 217–227. https://doi.org/10.1016/S0959-4388(97)80010-4
- Patai, E. Z., Gadian, D. G., Cooper, J. M., Dzieciol, A. M., Mishkin, M., & Vargha-Khadem, F. (2015). Extent of hippocampal atrophy predicts degree of deficit in recall. Proceedings of the National Academy of Sciences, 12830–12833. https://doi.org/10.1073/pnas.1511904112
- Picard, L., Mayor-Dubois, C., Maeder, P., Kalenzaga, S., Abram, M., Duval, C., Eustache, F., Roulet-Perez, E., & Piolino, P. (2013). Functional independence within the self-memory system: New insights from two cases of developmental amnesia. Cortex, 49(6), 1463–1481. https://doi.org/10.1016/j.cortex.2012.10.003
- Roediger, H. L., & Butler, A. C. (2011). The critical role of retrieval practice in long-term retention. Trends in Cognitive Sciences, 15(1), 20–27. https://doi.org/10.1016/j.tics.2010.09.003
- Rosenbaum, R. S., Cassidy, B. N., & Herdman, K. A. (2015, May). Patterns of preserved and impaired spatial memory in a case of developmental amnesia. Frontiers in Human Neuroscience, 9(1), 1–15. https://doi.org/10.3389/fnhum.2015.00196
- Squire, L. R., Genzel, L., Wixted, J. T., Morris, R. G. M., Wisted, J. T., & Morris, R. G. M. (2015). Memory consolidation. Cold Spring Harbor Perspectives in Biology, 7(8), a021766. https://doi.org/10.1101/cshperspect.a021766
- Thakral, P. P., Wang, T. H., & Rugg, M. D. (2017). Decoding the content of recollection within the core recollection network and beyond. Cortex, 91, 101–113. https://doi.org/10.1016/j.cortex.2016.12.011
- Vargha-Khadem, F., Gadian, D. G., Watkins, K. E., Connelly, A., Van Paesschen, W., & Mishkin, M. (1997). Differential effects of early hippocampal pathology on episodic and semantic memory. Science, 277(5324), 376–380. https://doi.org/10.1126/science.277.5324.376
- Vargha-Khadem, F., Limond, J. A., Belton, E., & Miskin, M. (2006). Society for Neuroscience. Atlanta, GA: New Semantic Learning in Developmental Amnesia.
- Vicari, S., Menghini, D., Di Paola, M., Serra, L., Donfrancesco, A., Fidani, P., Milano, G. M., & Carlesimo, G. A. (2007). Acquired amnesia in childhood: A single case study. Neuropsychologia, 45(4), 704–715. https://doi.org/10.1016/j.neuropsychologia.2006.08.004
- Winocur, G., & Moscovitch, M. (2011). Memory transformation and systems consolidation. Journal of the International Neuropsychological Society, 17(5), 766–780. https://doi.org/10.1017/S1355617711000683