ABSTRACT
This study describes blood plasma concentrations of PCBs and p,p’-DDE in the Canadian population aged 20–79 years. PCBs and p,p’-DDE were measured in 1668 participants in the Canadian Health Measures Survey, Cycle 1 (2007–2009). We investigated how concentrations vary by sociodemographic, anthropometric, and lifestyle variables, identified factors associated with exposures, and evaluated concentrations against health-based guidance values. Congeners of PCB most commonly detected were PCB-138, PCB-153, and PCB-180. p,p’-DDE was detectable in > 99% of the samples. Factors associated with ∑PCBs were age, region of birth, frequency of fish consumption, and liver intake (R2 = 58.1%). For p,p’-DDE, significant factors were sex, age, region of birth, household education, and ethnic origin (R2 = 47.0%). PCB concentrations in Canadians were similar to those in the United States, and lower than those reported in Europe. A small percentage equalled or exceeded the Human Biomonitoring value of 3.5 µg/L for PCBs. Few exceedances of the p,p’-DDE biomonitoring equivalent were observed.
KEYWORDS:
Introduction
Polychlorinated biphenyls (PCBs) and dichlorodiphenyltrichloroethane (DDT) are among the most well studied persistent organic pollutants (POPs) that bioaccumulate and biomagnify in the environment ([ATSDR] Agency for Toxic Substances and Disease Registry Citation2000, Citation2002). To study the body burden of these POPs in human populations, biomonitoring studies have reported PCBs, p,p’-DDT (a major isomeric constituent of DDT) and metabolites of p,p’-DDT in human blood (Becker et al. Citation2002; [CDC] Centers for Disease Control and Prevention Citation2009; Huetos et al. Citation2014; Saoudi et al. Citation2014). Generally, populations living at lower latitudes are exposed to PCBs and DDT primarily through consumption of fish, meat, dairy products, and breast milk ([ATSDR] Agency for Toxic Substances and Disease Registry Citation2000, Citation2002). In the Arctic, consumption of fish and marine mammals are the primary sources of exposure to PCBs and many other organochlorine compounds such as DDT and their metabolites. The most abundant PCB congeners are PCB-138, 153, and 180, and their body burden is generally representative of total PCBs ([ATSDR] Agency for Toxic Substances and Disease Registry Citation2000, Citation2011; [AFSSA] Agence Francaise de Sécurité Sanitaire des Aliments. Citation2010). In the liver, p,p’-DDT is metabolized to p,p’-dichlorodiphenyldichloroethylene (p,p’-DDE) ([ATSDR] Agency for Toxic Substances and Disease Registry Citation2002).
The Stockholm Convention of 2004 is an internationally binding agreement to phase out POPs (Stockholm Convention Citation2008). PCBs are listed under Annex A of the Convention, which requires measures to eliminate their production and use. The listing of p,p’-DDT is under Annex B, which restricts production but allows for continued use to control disease vectors. In Canada, action has been taken on PCBs and DDT under the Canadian Environmental Protection Act, 1999 (CEPA Citation2004), in response to concerns about environmental and health effects. Despite these regulations, PCBs, DDT and DDE continue to be detected in environmental media, biota, and human beings in Canada, primarily due to their long-range atmospheric transport, environmental persistence and bioaccumulative potential (Donaldson et al. Citation2010).
Occupational exposures to PCBs in humans and unintentional overdoses, as in the Yusho and Yu-Cheng poisoning incidents, have resulted in a broad spectrum of health effects, including effects on the liver, skin, and lung ([ATSDR] Agency for Toxic Substances and Disease Registry Citation2000; Li et al. Citation2013; Ruder et al. Citation2014; Putschögl et al. Citation2015; Yang et al. Citation2015). Potential adverse effects of low-level environmental exposures are complex and still need further validation. The United States Environmental Protection Agency’s Integrated Risk Information System (IRIS) is conducting a systematic review of non-cancer effects of PCBs. An initial scoping review of in vitro, animal, and human studies has identified several health effects of interest, such as effects on the cardiovascular and endocrine systems ([U.S. EPA] United States Environmental Protection Agency Citation2015). In human populations, p,p’-DDE has also been associated with adverse health effects, such as prostate cancer, diabetes, hypertension, and reduced lung function (Emeville et al. Citation2015; Van Larebeke et al. Citation2015; Ye et al. Citation2015). The International Agency for Research on Cancer classifies PCBs, including dioxin-like congeners, as carcinogenic to humans (Group 1) ([IARC] International Agency for Research on Cancer Citation2016ba), and DDT as probably carcinogenic to humans (Group 2A) ([IARC] International Agency for Research on Cancer Citation2016b).
Given the potential for adverse effects on human health, national biomonitoring surveys are critical for monitoring on-going population exposures and to evaluate the effectiveness of regulatory controls on the manufacture, import, and use of hazardous chemicals. The Canadian Health Measure Survey (CHMS) is an on-going, nationally representative, cross-sectional, direct health measures survey conducted every two years, starting in 2007 (Health Canada Citation2010). It provides nationally-representative data on the health of Canadians, including urine and blood concentrations of environmental contaminants.
In this study, data on blood concentrations of PCBs and p,p’-DDE in the Canadian population were obtained from the first cycle (2007–2009) of the CHMS, which contains the most recent data available and the first nationally representative dataset on individual exposures. p,p’-DDE was studied because of its higher potential for accumulation and persistence in the body compared to p,p’-DDT. Since PCBs and p,p’-DDE are lipophilic, their concentrations in serum and plasma are affected by lipid content; accordingly, lipid-adjusted serum or plasma concentrations represent cumulative past exposure ([ATSDR] Agency for Toxic Substances and Disease Registry Citation2000, Citation2002). The primary objectives of this paper are to assess plasma concentrations of PCBs and p,p’-DDE in population subgroups, to elucidate factors associated with exposures, and to compare the concentrations with international and historical levels as well as previously established levels of concern for PCBs and the metabolites of DDT.
Methods
Data source
Data collected as part of the CHMS Cycle 1 (2007–09) were analysed in this study. CHMS used a complex, multi-stage sampling strategy across 15 collection sites in five standard regions. In Cycle 1, 5604 respondents between 6 and 79 years of age were surveyed. They represented 96.3% of the Canadian population in this age range in the 10 provinces and three territories. Excluded from the survey were full-time members of the Canadian Forces, institutionalized individuals, persons living on reserves and other Aboriginal settlements, and those in certain remote regions, and in areas with low population density (Health Canada Citation2010). Ethics approval for the CHMS was from Health Canada’s Research Ethics Board and participation in the survey was voluntary (Health Canada Citation2010). Respondents were actively provided the results for lead, mercury, and cadmium and could receive the results of other environmental contaminants by request to Statistics Canada (Health Canada Citation2010).
The CHMS survey included a household interview component addressing sociodemographic characteristics, nutrition, physical activity, smoking, health conditions, as well as several other lifestyle and health risk factors. Physical measures were assessed at a mobile examination centre (MEC) located within a defined radius of each collection site.
As part of the survey, the concentrations (µg/L) of 24 PCB congeners (PCB-28, 52, 66, 74, 99, 101, 105, 118, 128, 138, 146, 153, 156, 163, 167, 170, 178, 180, 183, 187, 194, 201, 203, and 206) and p,p’-DDE were measured in the plasma of 1668 fasting respondents aged 20–79 years. Laboratory measurements for plasma PCBs and p,p’-DDE were conducted at the Centre de toxicologie du Québec (CTQ) of L’Institut national de santé publique du Québec (INSPQ), Québec City. Measurements of lipid content in serum samples were conducted by Health Canada. Details of the measurement methodology, including quality assurance and control measures, have been described previously (Health [INSPQ] Institut national de santé publique du Québec Citation2009; Canada Citation2010). The limits of detection (LOD) for PCBs ranged from 0.01 to 0.05 μg/L, with the exception of PCB-52, which had a LOD of 0.30 μg/L. The LOD for p,p’-DDE was 0.09 μg/L (Health Canada Citation2010).
Data treatment
The Cycle 1 CHMS 2007–2009 database was accessed at the Carleton, Ottawa, Outaouais Local Research Data Center (COOL-RDC). Values below the LOD were imputed as LOD/2. After imputation of values below the LOD, lipid-adjusted PCB and p,p’-DDE concentrations were calculated by dividing plasma concentrations by total serum lipid content. Total lipid was derived from total cholesterol and triglycerides using the equation (Bernert et al. Citation2007):
total lipids (mg/dL) = 2.27*total cholesterol + triglycerides + 62.3
If cholesterol or triglyceride measurements were missing, then lipid-adjusted values were not calculated for those observations. Two observations were excluded for this reason. The concentration of plasma lipid has been found to be lower than serum lipid by 1.3–6% (Health Canada Citation2010). The reported adjusted contaminant concentrations based on serum lipids, therefore, may underestimate actual concentrations. An additional factor that may contribute to an underestimation of concentrations is the use of fasted samples. In a study by Phillips et al. (Citation1989), serum samples from non-fasted individuals contained higher concentrations of PCBs and p,p’-DDE compared with samples from fasted subjects. Although the authors showed that adjustment for serum lipids reduced the differences in the concentrations of PCBs and p,p’-DDE between fasted and non-fasted samples, the non-fasted samples still had 11% and 8% higher concentrations of PCBs and p,p’-DDE, respectively (Phillips et al. Citation1989).
The total PCB plasma concentration (∑PCBs) was calculated as the sum of plasma concentrations of individual congeners (PCB-28, PCB-66, PCB-74, PCB-99, PCB-101, PCB-105, PCB-118, PCB-128, PCB-138, PCB-146, PCB-153, PCB-156, PCB-163, PCB-167, PCB-170, PCB-178, PCB-180, PCB-183, PCB-187, PCB-194, PCB-201, PCB-203, PCB-206). PCB-52 was excluded from the sum because all concentration observations were below the LOD.
Sociodemographic, anthropometric and lifestyle factors
Concentrations of individual PCB congeners and p,p’-DDE by sex and age group (20–39, 40–49, 60–79 years) have been reported previously (Health Canada Citation2010). Here, we additionally report concentrations by sex and age group for ∑PCBs. Other sociodemographic factors analysed in this study were province of residence (Ontario, Quebec, rest of Canada; only Ontario and Quebec had sufficient number of participants to analyse individually), region of birth (North America, Europe, South Asia, East Asia/Southeast Asia, other), ethnic origin (Caucasian and other), highest level of household education (less than secondary school graduation, secondary school graduation, some post-secondary education, post-secondary graduation), occupational status in last 12 months, and household income quartile. Anthropometric factors assessed were body mass index (BMI) category and BMI quartile. BMI was calculated from measurements of weight and standing height. Respondents were classified as underweight (BMI < 18.50 kg/m2), normal weight (18.50 ≤ BMI ≤ 24.99 kg/m2), overweight (25.00 ≤ BMI ≤ 29.99 kg/m2), obese (30.00 ≤ BMI ≤ 34.99 kg/m2), very obese (35.00 ≤ BMI ≤ 39.99 kg/m2), and severely obese (BMI ≥ 40.00 kg/m2), based on the World Health Organization international standard (Statistics Canada Citation2014). These BMI categories were further aggregated into the following three categories: normal weight, overweight, and obese/very obese/severely obese.
Lifestyle factors included having breastfed at least one baby, current smoking status (daily, occasionally, not at all), and diet. The dietary factors were weekly consumption frequencies of fish (saltwater, freshwater, and shellfish – examined as individual types of fish and aggregated as total fish), egg and egg dishes, dairy products (milk, cottage cheese, yogurt, ice cream/frozen yogurt), red meat (beef, hamburger, pork, lamb), and liver (all types of liver such as beef, veal, pork, or chicken, excluding liverwurst and liver pâté). The weekly food consumption frequencies were calculated by adding the annual frequencies of individual food items within a group and dividing the sum by 52. Fish, egg, dairy, and red meat consumption were categorized as low, medium, or high based on tertiles of frequency of weekly consumption The range of weekly food intake frequencies captured by the different tertiles are shown in . Liver intake was classified as ‘none’ or ‘yes’.
Table 1. Description of study population (CHMS, Cycle 1).
Supplementary tables S1-S7 contain data for variables categorized in alternative ways (ie household income category, household income adequacy, and work in last week) and for variables that were statistically non-significant relative to the reference category.
Statistical analysis
Geometric means (GM) and 95% confidence intervals (CIs) were calculated for individual PCB congeners with less than 40% of observations below the LOD. To produce estimates that were representative of the Canadian population covered by the survey, all analyses incorporated the survey sample weights generated by Statistics Canada. These weights adjust for the unequal probability of being selected into the survey and for non-response. In addition, to account for the complex survey design, bootstrap weights provided by Statistics Canada were used to estimate 95% CIs. As per Statistics Canada guidelines, estimates based on small samples (< 10) have not been reported.
GMs and 95% CIs of lipid-adjusted ∑PCBs and p,p’-DDE were calculated for population subgroups in univariate analyses. Statistical differences between categories of subgroups were examined by t tests or analysis of variance (ANOVA). Pairwise comparisons with Bonferroni correction were made if ANOVA was statistically significant (p < 0.05). The univariate subgroup analyses were used to inform the construction of multiple linear regression models to identify factors associated with exposures, adjusted for other covariates. Lipid-adjusted natural log transformed ∑PCBs or p,p’-DDE concentrations were the dependent variables.
Subgroups with significantly different lipid-adjusted concentrations of ∑PCBs or p,p’-DDE were considered for inclusion in the statistical models. Due to the limited number of degrees of freedom, the models were constructed in two stages. In Stage 1, variables that were statistically significant in univariate analyses, excluding food variables, were included in a model that incorporated survey sample weights, and a manual backward elimination procedure was performed. For ∑PCBs, the Stage 1 model included the following variables: age, region of residence, region of birth, ethnic origin, household education (or household income quartile), occupational status in last 12 months, and having breastfed at least one baby. For p,p’-DDE, the Stage 1 model included: sex, age, region of birth, ethnic origin, household education, household income quartile, and occupational status in last 12 months. Smoking status was not added to the p,p’-DDE model at this point because it overfitted the model, but was tested for statistical significance by adding it to the final Stage 1 model. The statistically non-significant variables were iteratively removed until all retained variables were statistically significant (p < 0.05). Each variable that was removed was entered back into the model, one by one, to assess if that variable reached statistical significance.
In Stage 2, statistically significant food variables in univariate analyses were then added to the Stage 1 model. For ∑PCBs, the variables added were frequencies of consumption of fish, red meat, and egg/egg dishes, and intake of liver. For p,p’-DDE, the variables added were frequency of consumption of freshwater fish and intake of liver. The manual backward elimination procedure was repeated to arrive at final models. Again, food variables that were removed were entered back into the model, one by one, to assess if that variable reached statistical significance. The final models were tested for correlation between variables (variation inflation factor < 5) and linear regression assumptions (eg homoscedasticity).
Statistical significance was p < 0.05. Analyses were conducted using Stata version 14.0.
Comparison with PCB levels of concern and DDE biomonitoring equivalent
We compared our results to recently established blood guidance values, the human biomonitoring (HBM) values I and II for non-carcinogenic effects of PCBs, derived by the German Federal Environment Agency (German Federal Environment Agency Citation2012). HBM values were based on wet-weight serum PCB concentrations (calculated as the sum of wet-weight serum concentrations of PCB-138, 153, and 180 multiplied by a factor of 2). As wet-weight serum concentrations were not available in the CHMS Cycle 1, comparisons with HBM I and II values were made by taking the sum of wet-weight plasma concentrations of PCB-138, 153, and 180 multiplied by a factor of 2. The GMs and percentages exceeding HBM values were calculated for the total study population (20–79 years old), and for the age groups 20–39 years, 40–59 years, and 60–79 years for men and women, as well as women of child-bearing age (20–45 years). The last was a vulnerable population sub-group considered in the development of HBM values (German Federal Environment Agency Citation2012). For p,p’-DDE, CHMS Cycle 1 concentrations were compared with a biomonitoring equivalent value of 5000 µg/kg lipid (Kirman et al. Citation2011). This value was based on the no-observed-adverse-effect-level (NOAEL) for liver lesions in rats (non-cancer effects) for the sum of DDT, DDE, and DDD, and potency estimates for liver tumours in male and female mice and hamsters (carcinogenicity) for DDE. The GMs and percentages of individuals with plasma p,p’-DDE concentrations exceeding 5000 µg/kg lipid were calculated for the total study population (20–79 years old), and for the age groups 20–39 years, 40–59 years, and 60–79 years for men and women.
Results
Description of study population
Measurements of PCBs and p,p’-DDE were taken in 1668 respondents (weighted population: 23,308,581), with equal distribution of males and females (). Most mothers breastfed at least one baby (70%). The majority of respondents were born in North America (78%) and were of Caucasian origin (84%). Nearly 90% of the population graduated from secondary school or a post-secondary institution. Most of the population fell under the normal-weight (38%) or overweight (39%) categories of BMI. Either daily or occasional smoking was reported by about 23% of the population. With respect to dietary factors, the largest percentage of the population was in the low-tertile category of consumption for fish (39%), egg and egg dishes (49%), and red meat (43%). Dairy product consumption was approximately equally distributed among the tertiles. The majority did not consume animal liver (61%).
Concentrations of PCBs and p,p’-DDE in the study population
In , wet-weight and lipid-adjusted plasma concentrations are provided for individual PCB congeners with < 40% of observations below the LOD (or detected in 60% or more of the samples), and for p,p’-DDE. These data are based on an independent analysis of biomonitoring data from CHMS Cycle 1 accessed at the COOL-RDC and matched summary results previously reported by Health Canada (Health Canada Citation2010). The congeners found at the highest concentrations were PCB-138 (GM, 95% CI = 10.1, 8.9–11.5 µg/kg lipid), PCB-153 (18.3, 15.8–21.2 µg/kg lipid), and PCB-180 (15.2, 13.5–17.1 µg/kg lipid). Both per cent detection rates and GM concentrations of individual congeners varied widely among the age groups 20–39 years, 40–59 years, and 60–79 years based on an analysis of summary data provided by Health Canada (Citation2010) (). Only four of the 24 PCB congeners analysed as part of the CHMS were detected in ≥ 60% of the plasma samples collected from participants of 20–39 years of age, contrasting with the detection of 16 of 24 congeners in ≥ 60% of samples from the 60–79 years age group (). The greater detection rates for several congeners in individuals from the older age group were also complemented by higher concentrations of the detected congeners (). p,p’-DDE was detectable in > 99% of the population (152.3, 127.2–182.2 µg/kg lipid).
Table 2. Wet-weight and lipid-adjusted plasma concentrations of PCBs and p,p’-DDE in the Canadian population (CHMS, Cycle 1).
Figure 1. Profiles of PCB congeners in the CHMS, Cycle 1.
Detection rates and concentrations of PCB congeners by age group, measured as part of CHMS Cycle 1 (based on data reported in HC (2010). A) Circles denote that the congener was detected (concentration ≥ LOD) and areas of the circles represent the per cent of CHMS samples in which a congener was detected (range from 1–100%). Shaded circles represent congeners detected in ≥ 60% of samples for which a geometric mean was calculated and shown in . B. Areas of circles represent geometric mean concentrations of the congeners ranging from 2.29 µg/kg lipid (PCB-146 in 40–59 years age group) to 41.27 µg/kg lipid (PCB-180 in 60–79 years age group).
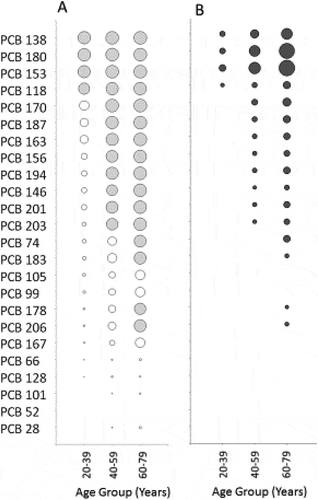
Subgroup comparisons: sociodemographic, anthropometric, and lifestyle factors
Univariate analyses showed statistically significant differences in lipid-adjusted concentrations of ∑PCBs and p,p’-DDE among categories of demographic, anthropometric, and lifestyle variables (). Concentrations of ∑PCBs increased with age and were significantly different across age categories. The concentrations were also significantly different across total household income quartiles, with lower PCB concentrations in income quartile 3 ($61,000–$99,000) compared with quartiles 1 (0–$35,000) and 2 ($36,000–$60,000). No differences were found by household income category or household income adequacy (Supplementary Material, Tables S1 and S2). Respondents who resided in Ontario had higher concentrations of ∑PCBs compared with the rest of Canada, omitting the Ontario and Quebec regions. Statistically significant differences were observed by region of birth, with respondents born in Europe having higher PCB concentrations compared with other regions. ∑PCBs concentrations in Caucasians were significantly higher compared with all other ethnicities combined. In the post-secondary graduation category, however, ∑PCBs concentration was significantly lower compared with those found in the less-than-secondary school graduation category. Similarly, PCB concentrations were significantly lower in those who worked in a job or business in the last 12 months () or in the last week (Supplementary Material, Table S3) preceding the date of the household survey, compared with those who did not. No statistically significant differences by BMI category or BMI quartile were found (Supplementary Material, Tables S4 and S5). Women who breastfed at least one baby had significantly lower ∑PCBs compared with women who had not breastfed.
Table 3. Univariate analysis of lipid-adjusted plasma concentrations of PCBs and p,p’-DDE by subgroup (CHMS, Cycle 1).
Females had significantly higher p,p’-DDE concentrations compared with males (). p,p’-DDE concentrations also increased with age. Differences in p,p’-DDE concentration by region of birth were observed. In pairwise comparisons, respondents born in Europe, South Asia, East/Southeast Asia or other region had significantly higher concentrations of p,p’-DDE than did respondents born in North America (p < 0.01). Caucasians had much lower p,p’-DDE concentrations compared with all other ethnicities combined. There were statistically significant differences by education, income, and occupational status. Concentrations of p,p’-DDE in some ‘post-secondary school’ or ‘post-secondary graduation’ respondents were lower compared with those with less than secondary school graduation. In household income quartiles 3 ($61 000-$99 000) and 4 ($100 000-$500 000), p,p’-DDE concentrations were lower compared with income quartile 1 (0-$35 000). Lower p,p’-DDE concentrations were also observed among respondents in higher household income categories and among those with higher household income adequacy (Supplementary Material, Tables S1 and S2). p,p’-DDE concentrations in respondents who worked at a job or business in last 12 months () or last week (Supplementary Material, Table S3) were significantly lower compared to respondents who had not worked. There were no statistically significant differences by BMI (Supplementary Material, Tables S4 and S5), or in women who breastfed versus those who did not. Daily smokers had significantly lower concentrations compared with non-smokers ().
Subgroup comparisons: dietary factors
Significantly higher concentrations of ∑PCBs were found among the high and medium tertiles of fish consumption compared with the low tertile (). For red meat consumption, significantly lower concentrations were found for the highest tertile compared with the medium tertile (Supplementary Material, Table S7). Respondents who consumed animal liver had significantly higher ∑PCBs compared with respondents who consumed no liver (). The highest tertile of egg/egg dish consumption was associated with lower PCB concentrations compared with the lowest tertile (). No significant differences were observed in PCB concentrations with consumption of dairy products (Supplementary Material, Table S6).
For p,p’-DDE, no statistically significant differences by consumption of total fish were observed. However, p,p’-DDE concentrations were higher in those in the highest tertile of freshwater fish compared with those in the medium tertile (data not shown). There were also no differences by dairy product, egg/egg dish, or red meat consumption (Supplementary Material, Tables S6 and S7). Liver intake was associated with higher lipid-adjusted plasma p,p’-DDE concentrations ().
Factors associated with PCB and p,p’-DDE concentrations in regression models
Age, region of birth, frequency of fish consumption, and liver intake were significantly associated with ∑PCBs concentrations (R2 = 58.1%). The age covariate had a β of 0.03 (). This translates to an increase in lipid-adjusted plasma concentration by a multiple of 1.03 (or 3%) for each one-year increase in age. Birth in a European country was associated with higher ∑PCBs concentrations compared with birth in North America (1.46 times higher, 95% CI: 1.08–1.99). The highest tertile of fish consumption was associated with an increase in ∑PCBs concentration by a multiple of 1.30 (95% CI: 1.15–1.45), relative to the lowest tertile. Eating animal liver was associated with an increase in ∑PCBs concentration by 1.12 times (95% CI: 1.03–1.21), compared to not eating animal liver.
Table 4. Factors associated with lipid-adjusted plasma concentrations of ∑PCBs and p,p’-DDE based on multiple linear regression analysis (CHMS, Cycle 1).
Sex, age, region of birth, household education, and ethnic origin were significantly associated with p,p’-DDE concentration (R2 = 47.0%). Females had 1.23 (95% CI: 1.07–1.40) times higher concentration of p,p’-DDE compared with males (). As with ∑PCBs, each one-year increase in age was associated with an increase in concentration of 3%. Birth in a region other than North America was associated with a 1.67 (95% CI: 1.27–2.18) times increase, and non-Caucasian origin was associated with a 2.80 (95% CI: 1.55–5.05) times increase compared with birth in North America and Caucasian origin, respectively. Post-secondary graduation was associated with a 0.79 (95% CI: 0.63–0.99) times lower p,p’-DDE concentration compared with less-than-secondary school graduation.
Comparison with PCB levels of concerns and DDE biomonitoring equivalent
The GMs and 95% CIs of wet-weight PCBs (∑PCB138, 153, 180 x2) and lipid-adjusted p,p’-DDE concentrations for the total CHMS population, and by male and female age groups, are plotted in relation to the levels of concern for PCBs () and the biomonitoring equivalent for DDE (). A small percentage of the population (2.5% total, 3.1% males, and 2.0% females) had PCB concentrations equal to or exceeding the HBM I value of 3.5 µg/L (99th percentile for ∑PCB138, 153, 180 × 2 = 4.7 µg/L). Cell counts were too low to release estimates of exceedances by male and female age groups. None of the population exceeded the HBM II value of 7 µg/L, although the upper 95% CI limit of the GM estimate for males aged 60–79 years was 8.1 µg/L (). Most of the population had p,p’-DDE concentrations below the biomonitoring equivalent of 5000 µg/kg (99th percentile: 2648.5 µg/kg lipid) ().
Discussion
In this study, plasma concentrations of individual PCB congeners, ∑PCBs, and p,p’-DDE in a representative sample of the Canadian population, 20–79 years of age, from the CHMS Cycle 1 (2007–2009), were assessed. When compared with other national surveys conducted during a comparable time frame, the more frequently detected and highly abundant PCB congeners in the Canadian adult population (ie PCB-138, PCB-153, and PCB-180) were similar to those measured in the United States, and considerably lower than those reported in European surveys (). The contrasts were relatively less prominent for p,p’-DDE, except for a notable difference between the American and Canadian concentrations. These differences may be partly attributable to differences in age and the fasting status of the adult populations covered in the surveys, choice of sample matrix (i.e., plasma, serum, whole blood), method to calculate total lipids for use in lipid adjustment, LOD or limit of quantification, and factors considered in the imputation of values below the LOD. However, the more substantial two- to six-fold higher concentrations of PCB-138, PCB-153, or PCB-180 in European populations compared to Canadians may relate to the extended production and trade of PCBs in Europe compared to North America before regulatory interventions, and to several European populations residing near PCB-contaminated sites (Breivik et al. Citation2002, InVS Citation2011). European countries banned the use of PCBs in open applications in 1976 and, in 1996, established guidelines for disposal and decontamination of equipment containing 500 ppm or more by 2010 (Eckley and Selin Citation2004). In support of the findings of higher PCB concentrations in European surveys, we found that birth in a European country was associated with higher ∑PCBs compared with birth in North America. Notwithstanding the regional differences, the concentrations of PCBs and DDT are generally on the decline in both European and North American populations, including Canada, where multiple regulations were adopted to reduce exposures.
Table 5. Comparison with selected international surveys.
In Canada, PCBs were added to the List of Toxic Substances under the Canadian Environmental Protection Act (CEPA, Citation2004). The import and commercial uses of PCBs were restricted in Canada in 1977 with the introduction of Chlorobiphenyls Regulations (1977). Updated PCB regulations that came into effect in 2008 under CEPA (Citation2004) aims to further minimize the environmental and health risks posed by PCBs through accelerating their phase out and eventual elimination through further restrictions on the manufacture, export, import, sale, use, processing, storage, and release of PCBs. Although it was never manufactured in Canada, DDT was registered as a pesticide in the 1940s and was widely used in pest control products until the 1960s. In response to increasing environmental and safety concerns, most uses of DDT in Canada were phased out by the mid-1970s and registration of all uses of DDT was discontinued in 1985, with the understanding that existing stocks would be sold, used or disposed of by 1990. The sale or use of DDT in Canada today is prohibited. The effect of these regulatory measures are expected to be reflected in PCB and DDT concentrations measured in the general Canadian population when compared to historical levels. Comparisons of: (1) Plasma PCB concentrations in Canadians from CHMS Cycle 1 (2007–2009) with concentrations in women from Quebec City during 1994–1997 (Demers et al. Citation2002); (2) PCBs and p,p’-DDE concentrations from CHMS Cycle 1 with concentrations among a non-fish eating population from two Ontario communities in 1992 (Kearney et al. Citation1999); and (3) PCB and p,p’-DDE concentrations from CHMS Cycle 1 with concentrations among Canadians 65+ years of age from the Canadian Study of Health and Aging 1991–2002 (Medehouenou et al. Citation2011), suggest that Canadian concentrations of these contaminants have declined over the last two decades (). In a study of adipose tissue samples collected from across Canada during the years 1969, 1972, 1976, and 1985, Mes (Citation1990) noted a continuous decline in the levels of p,p’-DDE from 1969 to 1985. A decline in PCB levels was noted only after 1976, with a nearly halving of the levels of PCBs between 1976 and 1985. These declines corresponded to restrictions in the use and trade of PCBs starting in 1977, and an earlier phase-out of DDT. This temporal decline can be further validated using pooled serum data for PCBs, p,p’-DDT, and p,p’-DDE measured as part of CHMS Cycle 3 (2012–13), Cycle 4 (2014–15), Cycle 5 (2016–17), and Cycle 6 (2018–19), expected to become available in 2020.
Table 6. Comparison with Canadian historical data.
Particular segments of the general population, such as populations living in the Arctic, which are outside the scope of the CHMS, may be more vulnerable to contaminant exposure due to long-range transport of POPs to the Arctic. For Inuit living in the Arctic, the main source of exposure to POPs is from consumption of high-trophic-level marine mammals such as seals and whales, which is part of their traditional diet. Despite a steady decline in concentrations of POPs in Arctic populations over the last 20 years, total PCB concentrations in Canadian Inuit are about four times higher than those in the general Canadian population covered by the CHMS (GM, 95% CI: 409 µg/kg lipid, 389–430), and the sum of p,p-DDE and DDT concentrations in Canadian Inuit are about two times higher than general population exposures (GM, 95% CI: 311 µg/kg lipid, 292–323) (Laird et al. Citation2013). Research and biomonitoring studies in the Arctic are still on-going through continued support from the Northern Contaminants Program, which was established in 1991 to focus on addressing the issue of human exposure to elevated levels of contaminants in wildlife species in the Arctic.
The CHMS subgroup analyses based on age showed ~ 300% higher concentrations of ∑PCBs and ~ 200% higher concentrations of p,p’-DDE in the 60–79 age group than in the 20–39 age group. Even after adjusting for other covariates in multiple regression analyses, age was associated with 232% higher concentrations for both ∑PCBs and p,p’-DDE in the 60–79 age category compared to the 20–39 age category. Notably, the magnitude of increase observed for both ∑PCBs and p,p’-DDE concentrations between these two age groups is considerably greater than the levels associated with other statistically significant covariates, such as fish consumption (∑PCBs is 30% higher in participants in the highest vs lowest tertile), liver intake (∑PCBs is 12% higher in consumers than in non-consumers), region of birth (∑PCBs is 46% higher in participants born in European countries and p,p’-DDE is 67% higher in participants born outside North America, when compared to those born in North America), sex (p,p’-DDE is 23% higher in females than in males), and ethnicity (p,p’-DDE is 180% higher in non-Caucasians than in Caucasians). The effect of age on PCB concentration is likely related to greater exposures among older Canadians during periods of peak global PCB production and use, and to the bioaccumulative potential of PCBs. It is remarkable that as many as 20 of the 24 PCB congeners assessed were not even detected in ≥ 60% of the plasma samples collected from CHMS participants 20–39 years of age. This is in contrast to the detection and higher concentrations of 16 of 24 congeners in ≥ 60% of samples from the 60–79 age group. PCB-138, PCB-153, and PCB-180 were the three most abundant congeners in each age group and were detected at increasingly higher concentrations with increasing age, as also noted in datasets from other national surveys (ie the US National Health and Nutrition Examination Survey (NHANES), the French National Nutrition and Health Survey (Etude Nationale Nutrition Sante – ENNS), the German Environmental Survey 1998 (GerES III), and the Spanish BIOAMBIENT.ES project). While we illustrate an association of age with measured concentrations of individual congeners, an assessment of the significance of the differences in congener profiles (eg in terms of known differences in toxicities of different congeners, including between dioxin-like and non-dioxin-like congeners, and their concentrations in Canadians) is outside the scope of this work.
The difference in p,p’-DDE concentrations between those born in South Asia (GM, 1639 µg/kg lipid) and in North America (116.0 µg/kg lipid) was especially striking based on sub-group analysis. Following adjustment for other covariates in multiple regression models, birth in a region other than North America was still associated with a 67% higher concentration of p,p’-DDE than birth in North America. Similarly, in a study conducted on primiparous women from five southern Canadian locations between 2005 and 2007, a two-fold higher concentration of p,p’-DDE was noted in foreign-born women compared to Canadian-born (Curren et al. Citation2014). Variation in p,p’-DDE concentrations by region of birth, as noted in the CHMS, may be reflective of the continued manufacturing and use of p,p’-DDT in some parts of the world, such as Asia, for malaria control (van Den Berg Citation2009).
The reason for higher p,p’-DDE level in females compared with males is unclear, although this has been found in other studies. In the United States NHANES, DDE was higher in women than men, however the difference was not as pronounced as the CHMS (241 vs 235 ng/g lipid) ([CDC] Centers for Disease Control and Prevention Citation2009). An older study of non-eaters of Great Lakes fish in Canada reported higher DDE in females (359.8 µg/kg lipid) than in males (292.8 µg/kg lipid) (Kearney et al. Citation1999). A study of older Canadians 65+ also found higher DDE in females than in males (828 vs 565 ng/g lipid) (Medehouenou et al. Citation2011). The differences in DDE levels by sex may be due to differences in country of birth or travel history, however these factors were not specifically examined in our study. It is expected that DDE would be excreted through breast milk due to its lipophilicity (Carrizo et al. Citation2006). When we conducted analyses for women who had breastfed at least one baby, we did not observe a statistical difference with women who had not breastfed, although geometric mean levels were lower in women who had breastfed (187 vs 215 µg/kg lipid). The breastfeeding variable was examined among a subset of respondents who had given birth, and placental transfer, or small sample size, may have had a role in masking the effect of breastfeeding.
The association of fish consumption with levels of ∑PCBs corresponds with findings from other studies. In the NHANES, the concentration of the sum of 30 PCB congeners was strongly associated with fish consumption, but was not correlated to meat or milk (Xue et al. Citation2014). In a smaller study of Montreal-area residents, participants with high consumption of sport fish from the St. Lawrence River had significantly higher levels of Aroclor 1260, 14 individual PCB congeners, and p,p’-DDE (Kosatsky et al. Citation1999). The observed association between the dietary intake of animal liver and PCB concentrations may be related to the presence of lipophilic chemicals, such as PCBs, at higher concentrations in the liver than in the muscle tissue of farm-raised and game animals used for human consumption (Wahl et al. Citation2013; Warenik-Bany et al. Citation2016).
St-Amand et al. (Citation2014) compared the CHMS Cycle 1 plasma PCB concentrations (calculated as the sum of PCB-138, PCB-153, PCB-180) to the levels of concern established by the Agence Française de Securité Sanitaire des Aliments (AFSSA), which were based on mental and motor development in children exposed in utero, and thyroid hormone levels and neurological function in adult populations ([AFSSA] Agence Francaise de Sécurité Sanitaire des Aliments. Citation2010). The AFSSA level of concern of 700 ng total PCB/g of plasma lipids was established for pregnant women, women of childbearing age, lactating women, and children under 3 years of age. The level of concern of 1800 ng total PCBs/g of plasma lipids was established for boys over three years of age, adult men, and women past child-bearing age. The former value was compared with CHMS data for women 20–45 years of age, and the latter value with CHMS data for men aged 20–79 years and women aged 45–79 years. The calculated hazard quotients (ie the ratio of biomarker concentration to the level of concern) were less than 1.0 for all CHMS age groups, suggesting the existence of a margin of safety (St-Amand et al. Citation2014).
We compared the CHMS Cycle 1 dataset to the HBM I and II guidance values for PCBs, established by the German Federal Environment Agency (German Federal Environment Agency Citation2012). In the development of HBM values for PCBs, neurotoxicity and immunotoxicity were considered critical effects in the most vulnerable population of children exposed in utero. The final HBM values were based on benchmark doses calculated for neurotoxic effects by Jacobson et al. (Citation2002). Adverse effects are not expected below 3.5 μg PCBtotal/L serum (HBM I value); however, there is an elevated risk of adverse effects above 7 μg PCBtotal/L serum (HBM II value) in susceptible populations, such as infants, young children, and women of child-bearing age. Although both the AFSSA levels of concern and German HBM values are based on health criteria, the AFFSA values are meant to be used only for population-level interpretations of PCB related health risks ([AFSSA] Agence Francaise de Sécurité Sanitaire des Aliments. Citation2010), whereas the HBM values are relevant as screening value in environmental medicine. Exceedance of HBM I confers a slightly elevated risk of subtle, but not quantitatively relevant, cognitive effects, and HBM II is intended as an intervention value. With exceedances of either HBM value, measures should be taken to identify and eliminate the sources of exposure, especially when the exceedances occur in infants, children, or women of child-bearing age (German Federal Environment Agency Citation2012). In the CHMS, a small percentage (2.5%) of the population exceeded the HBM I value. Although the number of exceedances for both males and females by age group were too small to report, the GM and 95% CI estimates clearly increase from the 20–39 to the 60–79 age groups and, therefore, the majority of exceedances will be concentrated in the elderly population.
Plasma concentration of p,p’-DDE in Canadians were well below the biomonitoring equivalent of 5000 ng/g lipid for sum of DDT and its metabolites. This biomonitoring equivalent corresponds to the exposure guidance values of the US Environmental Protection Agency (USEPA) RfD (reference dose), the Netherlands National Institute for Public Health and the Environment (RIVM) TDI (tolerable daily intake), and the Agency for Toxic Substances and Disease Registry (ATSDR) intermediate MRL (maximum residue limit), which were based on liver lesions in rats and a 1 × 10−4 cancer risk level (Kirman et al. Citation2011). In the CHMS, concentrations of p,p’-DDE among males and females of all age groups were well below the biomonitoring equivalent. In a previous comparison of the sum of DDT and DDE from CHMS, Cycle 1 to a biomonitoring equivalent of 40,000 ng/g lipid, based on an FAO/WHO pTDI (provisional tolerable daily intake) exposure guidance value, St-Amand et al. (Citation2014) calculated hazard coefficients of less than 1.0. As noted by Haines et al. (Citation2017), the PCB and DDE background exposures of Canadians, as measured by the 95th percentile of the measured pollutant concentrations (also referred to as reference values or RV95), are similar or less than the RV95 values established for the United States, France and Germany. The evidence, from Canadian datasets, of declining PCB and DDE levels in the general Canadian population due to continued regulatory measures, provides an additional layer of safety.
Chronic diseases, such as cardiovascular disease and diabetes, are emerging concerns related to PCB and p,p’-DDE exposures (Valera et al. Citation2013; Turyk et al. Citation2015), and may be relevant to future health guidance development. The carcinogenicity of population-level PCB exposures is still not well characterized, as the mechanisms of carcinogenicity are complex ([IARC] International Agency for Research on Cancer Citation2016a). Neither chronic disease, such as cardiovascular disease and diabetes, nor carcinogenicity was factored into the derivation of previously established HBM values or the AFFSA level of concern for PCBs due to insufficiency or inconsistency of human data.
With on-going monitoring of POPs in the Canadian population, the biomonitoring data collected by the CHMS are important from both research and public policy standpoints. Health Canada’s existing guidance value for PCBs is based on Aroclor 1260 (Health Canada Citation1998). This value was established for whole blood and hence not used in our interpretations of plasma concentrations from the CHMS. The many Canadian biomonitoring efforts as part of the CHMS, the Maternal Infant Research on Environmental Chemicals (MIREC) Study, the Northern Contaminants Program (NCP), and the First Nations Biomonitoring Initiative (FNBI), along with a growing literature on potential health effects associated with PCBs, provide a strong evidence base for future work on PCB guidance in Canada.
CIJE-2018-0172-File003.docx
Download MS Word (25.2 KB)Acknowledgements
The analysis presented in this paper was conducted at the COOL RDC, which is part of the Canadian Research Data Centre Network (CRDCN). The services and activities provided by the COOL RDC are made possible by the financial or in-kind support of the Social Sciences and Humanities Research Council (SSHRC), the Canadian Institutes of Health Research (CIHR), the Canada Foundation for Innovation (CFI), Statistics Canada, Carleton University, the University of Ottawa and the Université du Québec en Outaouais. The views expressed in this paper do not necessarily represent those of the CRDCN or its partners. We thank Mike Walker, Scott Blechinger, Meredith Curren, Lorraine Seed, Cheryl Khoury, Bryan Adlard, and Kim Irwin from Health Canada for their invaluable feedback.
Disclosure statement
The authors declare that they have no conflict of interest.
Supplementary Material
Supplementary data can be accessed here.
Additional information
Funding
References
- [AFSSA] Agence francaise de sécurité sanitaire des aliments. 2010. Opinion of the French food safety agency on interpreting the health impact of PCB concentration levels in the French population. [Internet]. [Accessed 2016 Mar 19]. Available from: https://www.anses.fr/en/content/opinion-french-food-safety-agency-interpreting-health-impact-pcb-concentration-levels-french
- [ATSDR] Agency for Toxic Substances and Disease Registry. 2000. Toxicological profile for polychlorinated biphenyls (PCBs). [Internet]. [Accessed 2016 Mar 20]. Available from: http://www.atsdr.cdc.gov/toxprofiles/tp17.pdf
- [ATSDR] Agency for Toxic Substances and Disease Registry. 2002. Toxicological profile for DDT, DDE, and DDD. [Internet]. [Accessed 2016 Mar 20]. Available from: http://www.atsdr.cdc.gov/toxprofiles/tp35.pdf
- [ATSDR] Agency for Toxic Substances and Disease Registry. 2011. Addendum to the toxicological profile for polychlorinated biphenyls. [Internet]. [Accessed 2016 Mar 20]. Available from: http://www.atsdr.cdc.gov/toxprofiles/pcbs_addendum.pdf
- [CDC] Centers for Disease Control and Prevention. 2009. Fourth National Report on Human Exposure to Environmental Chemicals. [ Internet]. [Accessed 2016 Mar 20]. Available from: http://www.cdc.gov/exposurereport/pdf/FourthReport.pdf
- [CEPA] Canadian Environmental Protection Act. 2004. A guide to understanding the Canadian Environmental Protection Act, 1999. [Internet]. [Accessed 2017 May 23]. Available from: https://www.ec.gc.ca/lcpe-cepa/E00B5BD8-13BC-4FBF-9B74-1013AD5FFC05/Guide04_e.pdf
- [IARC] International Agency for Research on Cancer. 2016a. Polychlorinated biphenyls and polybrominated biphenyls: IARC monographs on the evaluation of carcinogenic risks to humans. Volume 107. [Internet]. [Accessed 2016 May 10]. Available from: http://monographs.iarc.fr/ENG/Monographs/vol107/mono107.pdf
- [IARC] International Agency for Research on Cancer. 2016b. DDT (4,4ʹ-Dichlorodiphenyltrichloroethane). IARC monographs on the evaluation of carcinogenic risks to humans. [Internet]. [Accessed 2016 Dec 12]. Available from: http://monographs.iarc.fr/ENG/Classification/latest_classif.php
- [INSPQ] Institut national de santé publique du Québec. (2009). Analytical method for the determination of polychlorinated biphenyl congeners, polybrominated congeners, toxaphene congeners, and organochlorinated pesticides in plasma by GC-MS (E-446), condensed version. Laboratoire de toxicologie. 2009- 05-25.
- [INVS] French Institute for Public Health Surveillance. 2011. Exposition de la population française aux substances chimiques de l’environnement. Tome 2: polychlorobiphényles (PCB-NDL); Pesticides. [Internet]. [Accessed 2017 May 22]. Available from: http://opac.invs.sante.fr/doc_num.php?explnum_id=6864
- [U.S. EPA] United States Environmental Protection Agency. 2015. Scoping and problem formulation for the toxicological review of polychlorinated biphenyls (PCBs): effects other than cancer [CASRN 1336-36-3]. [Internet].[Accessed 2016 Aug 9]. Available from: https://cfpub.epa.gov/ncea/iris_drafts/recordisplay.cfm?deid=309645
- Becker K, Kaus S, Krause C, Lepom P, Schulz C, Seiwert M, Seifert B. 2002. German Environmental Survey 1998 (GerES III): environmental pollutants in blood of the German population. Int J Hyg Environ Health. 205:297–308.
- Bernert JT, Turner WE, Patterson DGJ, Needham LL. 2007. Calculation of serum “total lipid” concentrations for the adjustment of persistent organohalogen toxicant measurements in human samples. Chemosphere. 68:824–831.
- Breivik K, Sweetman A, Pacyna JM, Jones KC. 2002. Towards a global historical emission inventory for selected PCB congeners - a mass balance approach 1. Global Production and Consumption. Sci Total Environ.. 290:181–198.
- Canada H. 2010. Report on human biomonitoring of environmental chemicals in Canada: results of the Canadian health measures survey Cycle 1 (2007-2009). [Internet]. [Accessed 2016 Mar 19]. Available from: http://www.hc-sc.gc.ca/ewh-semt/alt_formats/hecs-sesc/pdf/pubs/contaminants/chms-ecms/report-rapport-eng.pdf
- Carrizo D, Grimalt JO, Ribas-Fito N, Sunyer J, Torrent M. 2006. Physical-chemical and maternal determinants of the accumulation of organochlorine compounds in four-year-old children. Environ Sci Technol. 40:1420–1426.
- Curren MS, Davis K, Liang CL, Adlard B, Foster WG, Donaldson SG, Kandola K, Brewster J, Potrala M, Van Oostdam J. 2014. Comparing plasma concentrations of persistent organic pollutants and metals in primiparous women from northern and southern Canada. Sci Total Environ. 479-480:306–318.
- Demers A, Ayotte P, Brisson J, Dodin S, J,And R, Dewailly É. 2002. Plasma concentrations of polychlorinated biphenyls and the risk of breast cancer: a congener-specific analysis. Am J Epidemiol. 155:627–635.
- Donaldson SG, Van Oostdam J, Tikhonov C, Feeley M, Armstrong B, Ayotte P, Boucher O, Bowers W, Chan L, Dallaire F, et al. 2010. Environmental contaminants and human health in the Canadian Arctic. Sci Total Environ. 408:5165–5234.
- Eckley N, Selin H. 2004. All talk, little action: precaution and European chemicals regulation. J Eur Public Policy. 11:78–105.
- Emeville E, Giusti A, Coumoul X, Thome JP, Blanchet P, Multigner L. 2015. Associations of plasma concentrations of dichlorodiphenyldichloroethylene and polychlorinated biphenyls with prostate cancer: a case-control study in Guadeloupe (French West Indies). Environ Health Perspect. 123:317–323.
- German Federal Environment Agency. 2012. Human Biomonitoring (HBM) values for polychlorinated biphenyls (pcbs) in blood. Position paper by the Human Biomonitoring Commission of the Federal Environmental Agency. German Federal Environment Agency (Umweltbundesamt).
- Haines DA, Khoury C, Saravanabhavan G, Werry K, Walker M, Malowany M. 2017. “Human biomonitoring reference values derived for persistent organic pollutants in blood plasma from the canadian health measures survey 2007–2011.” Int J Hyg Environ Health in press. doi:10.1016/j.ijheh.2017.03.004.
- Health Canada. 1998. The health and environment handbook for health professionals: health and the environment. In: Ottawa: Health Canada. p. 1998.
- Huetos O, Bartolome M, Aragones N, Cervantes-Amat M, Esteban M, Ruiz-Moraga M, Perez-Gomez B, Calvo E, Vila M, Es BIOAMBIENT, et al. 2014. Serum PCB levels in a representative sample of the SPANISH adult population: the BIOAMBIENT.ES. Project. Sci Total Environ.. 493:834–844.
- Jacobson JL, Janisse J, Banerjee M, Jester J, Jacobson SW, Ager JW. 2002. A benchmark dose analysis of prenatal exposure to polychlorinated biphenyls. Environ Health Perspect. 110:393–398.
- Kearney JP, Cole DC, Ferron LA, Weber JP. 1999. Blood PCB, p,p’-DDE, and Mirex levels in Great Lakes fish and waterfowl consumers in two Ontario communities. Environ Res. 80:S138–S149.
- Kirman CR, Aylward LL, Hays SM, Krishnan K, Nong A. 2011. Biomonitoring equivalents for DDT/DDE. Regul Toxicol Pharmacol. 60:172–180.
- Kosatsky T, Przybysz R, Shatenstein B, Weber JP, Armstrong B. 1999. Fish consumption and contaminant exposure among Montreal-area sportfishers: pilot study. Environ Res. 80:S150–S158.
- Laird BD, Goncharov AB, Chan HM. 2013. Body burden of metals and persistent organic pollutants among Inuit in the Canadian Arctic. Environ Int. 59:33–40.
- Li MC, Tsai PC, Chen PC, Hsieh CJ, Guo YLL, Rogan WJ. 2013. Mortality after exposure to polychlorinated biphenyls and dibenzofurans: 30 years after the “Yucheng Accident.”. Environ Res. 120:71–75.
- Medehouenou TCM, Ayotte P, Carmichael PH, Kröger E, Verreault R, Lindsay J, Dewailly E, Tyas SL, Bureau A, Laurin D. 2011. Polychlorinated biphenyls and organochlorine pesticides in plasma of older Canadians. Environ Res. 111:1313–1320.
- Mes J. 1990. Trends in the levels of some chlorinated hydrocarbon residues in adipose tissue of Canadians. Environ Pollut. 65:269–278.
- Phillips DL, Pirkle JL, Burse VW, Bernert JT, Omar Henderson L, Needham LL. 1989. Chlorinated hydrocarbon levels in human serum: effects of fasting and feeding. Arch. Environ. Contam. Toxicol.. 18:495–500.
- Putschögl FM, Gaum PM, Schettgen T, Kraus T, Gube M, Lang J. 2015. Effects of occupational exposure to polychlorinated biphenyls on urinary metabolites of neurotransmitters: a cross-sectional and longitudinal perspective. Int J Hyg Environ Health. 218:452–460.
- Ramos JJ, Huetos O, González S, Esteban M, Calvo E, Pérez-Gómez B, Castaño A. 2016. Organochlorinated pesticides levels in a representative sample of the Spanish adult population: the Bioambient.es project. Int J Hyg Environ Health. 220(2, Part A):217–226. Bioambient.es
- Ruder AM, Hein MJ, Hopf NB, Waters MA. 2014. Mortality among 24,865 workers exposed to polychlorinated biphenyls (PCBs) in three electrical capacitor manufacturing plants: a ten-year update. Int J Hyg Environ Health. 217:176–187.
- Saoudi A, Fréry N, Zeghnoun A, Bidondo ML, Deschamps V, Göen T, Garnier R, Guldner L. 2014. Serum levels of organochlorine pesticides in the French adult population: the French National Nutrition and Health Study (ENNS), 2006-2007. Sci Total Environ. 472:1089–1099.
- St-Amand A, Werry K, Aylward LL, Hays SM, Nong A. 2014. Screening of population level biomonitoring data from the Canadian Health Measures Survey in a risk-based context. Toxicol Lett. 231:126–134.
- Statistics Canada. 2014. Canadian Health Measures Survey (CHMS) Cycle 01 derived variable (DV) specifications. [Internet]. [Accessed 2016 May 10]. Available from: http://www23.statcan.gc.ca/imdb-bmdi/pub/document/5071_D3_T9_V2-eng.htm#a123
- Stockholm Convention. 2008. Listing of POPs in the Stockholm Convention. [Internet]. [Accessed 2016 Mar 20]. Available from: http://chm.pops.int/TheConvention/ThePOPs/ListingofPOPs/tabid/2509/Default.aspx
- Turyk M, Fantuzzi G, Persky V, Freels S, Lambertino A, Pini M, Rhodes DH, Anderson HA. 2015. Persistent organic pollutants and biomarkers of diabetes risk in a cohort of Great Lakes sport caught fish consumers. Environ Res. 140:335–344.
- Valera B, Jørgensen ME, Jeppesen C, Bjerregaard P. 2013. Exposure to persistent organic pollutants and risk of hypertension among Inuit from Greenland. Environmental Research. 122:65–73.
- van Den Berg H. 2009. Global status of DDT and its alternatives for use in vector control to prevent disease. Environ Health Perspect. 117:1656–1663.
- Van Larebeke N, Sioen I, Hond ED, Nelen V, Van de Mieroop E, Nawrot T, Bruckers L, Schoeters G, Baeyens W. 2015. Internal exposure to organochlorine pollutants and cadmium and self-reported health status: a prospective study. Int J Hyg Environ Health. 218:232–245.
- Wahl K, Malisch R, Kotz A, Djuchin K, Haedrich J. 2013. Levels of PCDD/Fs AND PCBs in meat and liver of different animal species and comparison with regulatory limits. Organohalogen Compounds. 75:1024–1027.
- Warenik-Bany M, Strucinski P, Piskorska-Pliszczynska J. 2016. Dioxins and PCBs in game animals: interspecies comparison and related consumer exposure. Environ. Int.89-90:21–29.
- Xue J, Liu SV, Zartarian VG, Geller AM, Schultz BD. 2014. Analysis of NHANES measured blood PCBs in the general US population and application of SHEDS model to identify key exposure factors. J Expo Sci Environ Epidemiol. 24:615–621.
- Yang CY, Chiou SL, Wang JD, Guo YL. 2015. Health related quality of life and polychlorinated biphenyls and dibenzofurans exposure: 30 years follow-up of Yucheng cohort. Environ Res. 137:59–64.
- Ye M, Beach J, Martin JW, Senthilselvan A. 2015. Association between lung function in adults and plasma DDT and DDE levels: results from the Canadian Health Measures Survey. Environ Health Perspect. 123:422–428.