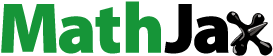
ABSTRACT
We tested the efficacy of standard soil removal and bacterial reduction from textile. A life cycle analysis for different washing cycles was also performed. The results show that washing at 40 °C and 10 g/L was the most effective and resulted in good removal of standard soiling. However, bacteria reduction was highest at 60 °C, 5 g/L and 40 °C, 20 g/L (> 5 log CFU/carrier). With the 40 °C, 10 g/L scenario, we approached the standard requirements for household laundry of ~ 4 log CFU/carrier reduction and good soil removal. Howsoever, life cycle analysis shows that washing at 40 °C and 10 g/L has a higher environmental impact than 60 °C and 5 g/L due to the significant contribution of the detergent. Reducing energy consumption and reformulation of detergents needs to be implemented in the household laundry to achieve sustainable washing without compromising the quality.
Introduction
Household laundry consumes a considerable amount of energy, chemicals and water to ensure good hygiene practices and consumer safety. However, with population growth and the increasing demand for clean and fresh clothes, the impact on the environment has become a major issue in both developing and developed countries (Morgan et al. Citation2018). It was estimated that 39% of greenhouse gas emissions from the textile and apparel industry worldwide are due to energy consumed in product care through washing, drying, and ironing. Accordingly, in recent years, household laundry has been the subject of several legislations, policies and initiatives in the EU, the US and the UK to reduce energy and material consumption per wash cycle and thus environmental impacts (Yates and Evans Citation2016; Sohn et al. Citation2021; Tavčer et al. Citation2022). Environmental impacts occur throughout the life cycle of laundry equipment, but the washing phase is by far the most burdensome. The environmental impact depends on washing practices, socioeconomic factors, washing facilities, detergents and other factors (Abney et al. Citation2021). The product-related parameters including detergent formulation, dose as well as washing machine-related parameters, such as wash program, temperature, load size and energy efficiency will contribute impact on the environment (Shahmohammadi et al. Citation2018). The research by Sa et al. (Citation2020) even showed that the carbon footprint for household laundry per amount of textile washed is higher than for professional laundry services. Reducing energy, water and chemical consumption are a win-win for the environment. Eberle et al. (Citation2007), for example, analysed low-temperature laundry washing using a life cycle assessment and found that energy consumption can be reduced by up to 55%, which has a positive impact on global warming potential, acidification and photochemical formation. Also, Chen et al. (Citation2021) reported that reducing the water temperature in the wash cycle reduces the environmental impact in terms of life cycle assessment results. The laundry detergent formulation has a significant impact on LCA. For example Dewaele et al. (Citation2006) assessed the LCA of reformulation of commercial liquid laundry detergent and found a reduced impact on eutrophication, acidification and ozone layer depletion. Furthermore, Rebello et al. (Citation2020) made an LCA comparison of conventional and natural detergents and found a lower impact on the environment with benefits on eutrophication and toxicity. All this indicates that detergents represent an important role in LCA and must thus be assessed. Either way, washing functionally removes soils, body fluids and microorganisms from textiles to ensure proper hygiene (Abney et al. Citation2021). The effectiveness of removing dirt and microorganisms is related to the variables of Sinner’s circle, which consists of temperature, time, chemistry and mechanical action and their interaction. The reduction of one of the factors must be compensated by others (Schages et al. Citation2020). The “eco” programmes of the modern washing machines offer a lower washing temperature and a longer programme duration. This concept indeed helps to save energy, and it has been shown to work very well in terms of soil removal, together with the use of modern detergents. Accordingly, carefully selected chemicals are added to the detergent to facilitate soil removal. These can be divided into four different groups: Surfactants to remove greasy stains (e.g. oil, butter, mayonnaise); Bleaches to remove oxidisable stains (e.g. red wine, tea, coffee); Builders to neutralise water hardness, enhancing the cleaning action of surfactants and soil dispersing/suspending and finally Enzymes to remove enzymatic stains (e.g. blood, grass, milk); (Johansson and Somasundaran Citation2007). Bleaching agents are added in the washing detergents in the form of perborates or percarbonates, from which reactive oxygen species (ROS) are released during washing. It is worth noting that perborates or percarbonates are added only in solid detergent powders and their amount have been greatly reduced since 2010 due to EU environmental regulations. The benefits of adding enzymes to detergents are also controversial. Although these highly effective biocatalysts help to remove stains effectively at low temperatures and mild pH values, they also have significant drawbacks, such as the antagonistic activity of protease to other added enzyme types and thus their reduced shelf life, skin irritation caused by protease enzyme residues on laundry after washing, and their negative effect on wash water (grey water) due to an imbalance in the organic environment of the wastewater treatment plant. Therefore, in certain detergents, both bleaching agents and enzymes are compensated by adding a higher concentration of surfactants.
Considering the concept of low-temperature washing, the doubts about achieving efficient washing hygiene are justified. Namely, stains derived from bodily secretions such as sweat, urine and even faeces are frequently present on clothing and are an important source of microbial contamination. In addition, clothing contaminated with microorganisms, such as underwear or shirts, can serve as a source for the spread of microorganisms and bacterial resistance (Bockmühl et al. Citation2019). Recent research has also found that the global burden of bacterial resistance is approximately 5 million deaths worldwide, and E. coli, P. aeruginosa and S. aureus are among the most prevalent resistant pathogens (Murray et al. Citation2022). The reduction of microorganisms on textiles is highly dependent on washing practices such as water temperature, choice of detergent and additives such as sodium hypochlorite and hydrogen peroxide (Honisch et al. Citation2014). A study by Riley et al. (Citation2017) showed that S. aureus and E. coli contamination on healthcare textiles can be removed by washing at 60°C for 10 minutes. Another study by Shin et al. (Citation2020) tested the effectiveness of bacterial reduction on the cotton surface and found that E. coli, P. aeruginosa and S. aureus can be removed at 60°C. Monleón-Getino and Cavalleri (Citation2022) studied the reduction of E. coli, P. aeruginosa and S. aureus from textiles at 40°C and demonstrated a reduction between 2–3.3 log CFU cm−2, with only the addition of perborate increasing efficacy to over 5 log CFU cm−2 when washing at low temperatures. This indicates that despite the low-washing temperature, washing hygiene can be well achieved just by the presence of bleaching agents in the detergents, but is compromised due to their decreased concentration in the modern powder detergents, while they are completely absent in the liquid detergents, which are however more popular among consumers (Ferri et al. Citation2016). The aim of the study was to evaluate the sustainability of the Sinner’s Circle principle, i.e. to determine the environmental impact of increased detergent concentration at low-temperature washing (i.e. 40°C) compared to high-temperature washing (i.e. 60°C). For this purpose, the standard phosphate-free detergent ECE without bleach or enzymes was used at various increased concentrations until both the standard soiling and the reference bacterial reduction were comparable to those achieved at 60°C. The environmental impact of the washing scenarios studied was determined and further analysed in terms of eco-points. This approach allows us to assess whether it is more sustainable to increase the washing temperature or to maintain washing at lower temperatures and adding more detergent.
Methods
Standard soil removal
The effectiveness of soil removal was tested according to the standard SIST EN 60,456:2005 with four different Empa standard soil fabrics: Empa 101–100% cotton soiled with carbon black/olive oil, Empa 114–100% cotton soiled with red wine, Empa 116–100% cotton soiled with blood/milk/ink and Empa 160–100% cotton soiled with chocolate (Materials Research Products, UK). The standard soiled textiles were washed in a Gorenje household washing machine, model Asko (Gorenje, Slovenia), using ECE reference detergent 98 (composition: 75 g linear sodium alkylbenzene sulfonate, 40 g ethoxylated fatty alcohol, 28 g sodium soap, 50 g antifoaming agent DC −42485, 250 g sodium aluminosilicate zeolite, 91 g sodium carbonate, 40 g sodium salt of a copolymer of acrylic and maleic acid, 26 g sodium silicate, 10 g carboxymethyl cellulose, 6 g diethylenetriamine penta(methylene phosphoric acid), 60 g sodium sulphate). The standard soil fabric samples were placed in the washing machine and washed together with the ballast load of 100% cotton fabric with a mass area of 170–190 g/m2 and a total weight of 2.45 kg. The programme “Universal without prewash” was used for all wash cycles. The volume of the wash bath was 10 L and the washing time was 30 minutes. Four different washing scenarios were performed ().
Table 1. Life cycle inventory for each washing scenario.
After washing, the samples were rinsed with 3 L of water for 10 minutes and air dried. To determine the removal of standard soil, colour measurements were made on unwashed and washed samples of standard soil using a Datacolour Spectraflesh 600 spectrophotometer PLUS-CT operated with Datacolour Datamaster software with the following settings: Illuminant D65, large area view, specular light excluded, UV included and standard observation angle of 10°. The soil removal efficiency was determined by the difference in brightness between washed and unwashed standard soils, ΔLs*, calculated as follows:
where and
stands for CIE L* coordinate values of the washed and unwashed standard soil samples. For each studied washing scenario two parallels of washing were performed and ten measurements per sample of standard soil were obtained.
Standard strain bacterial reduction
Bacteria reduction was tested according to the standard EN 16,616:2015 with some modifications as follows. Standard bacterial strains of cultures E. coli ATCC 35,218, P. aeruginosa ATCC 27,853 and S. aureus ATCC 25,923 were obtained from Sigma Aldrich (Virginia, USA). The bacteria from the collection were transferred to nutrient agar and incubated at 37°C for 24 hours. A single colony of the strain was transferred from nutrient agar to nutrient broth (Biolife, Italy) and incubated under the same conditions. For recovery, the bacterial suspension was centrifuged at 3000 gN for 15 minutes. The supernatant was removed and the pellet was mixed with an appropriate amount of physiological solution (0.9% NaCl) to obtain a cell concentration of 1.5 × 109 CFU mL−1. The suspension was centrifuged again and resuspended with defibrinated sheep blood (EOLABS, UK) in the same volume.
The cotton carriers were cut into 1 × 1 cm pieces, boiled in distilled water and then autoclaved. The sterilised cotton carriers were exposed to the bacterial suspension in defibrinated sheep blood for 15 minutes at room temperature. After drying at room temperature in a safety cabinet, the carriers were transferred to sterile cotton bags and the disinfection process was carried out in the washing machine (Asko, Gorenje). The washing machine was filled with the ballast and cotton carriers in the bags and, depending on the washing scenario, 12.5 ml of defibrinated sheep blood per kg of ballast and ECE reference detergent 98 were added (). At the end of the wash cycle, the bags containing the carriers were removed. Each treated carrier (Ct) was transferred to a separate tube containing 5 mL neutraliser (0.25 mol/L phosphate buffer) and glass beads. The bacterial cells were detached from the carriers by vortex shaking at 1000 rpm for 10 minutes. The sample liquid was then serially diluted and 1 ml of it was transferred to a Petri dish and poured with agar. Endo agar (Merck) was used for E. coli, cetrimide agar (Sigma Aldrich) for P. aeruginosa and mannitol salt agar (Biolife) for S. aureus. Agar plates were incubated at 37°C for 24 hours, colonies were counted, and results were expressed as log CFU/carrier. All experiments were performed with five parallel experiments and three replicates. Cotton carriers with bacteria that were not washed were considered as control (Cn). The reduction of bacterial cells was expressed as relative reduction (RL), calculated as log reduction RL.
Life cycle assessment of the washing cycle
The life cycle assessment (LCA) was carried out in accordance with ISO14040:2006 and ISO 14,044:2006 using Sima Pro 9.4.0.1 software (Pré Sustainability, Amersfoort, Netherlands). The tool allows the quantification of the environmental performance of products, taking into account the entire life cycle, starting with the production of raw materials and ending with the final disposal of the products (SimaPro Citation2016). In this study, the Ecoinvent 3.6 database was used for raw materials, manufacturing processes and processed materials. The ECE reference inventories for detergent 98 were taken from the database according to the detergent manufacturer (Methods 2.1), while water and energy were considered site-specific as Central European sites. Data on water consumption per wash cycle was taken from the washing machine manufacturer’s data sheet, while electricity consumption was measured in each wash scenario using the EMOS P5821 electricity consumption metre. The life cycle assessment is shown in . The LCA was calculated for a wash cycle without pre-washing and drying the laundry. The environmental impact results are expressed as eco-points (Pt). The unit Pt used in the eco-indicator method is defined as a dimensionless value. The value of 1 Pt means one-thousandth of the annual environmental impact of an average European inhabitant (Morsali Citation2017). We used the ReCiPe 2016 Endpoint, midpoint (H) v1.07 method. Impact categories were: Global Warming – Human Health, Global Warming – Terrestrial Ecosystems, Global Warming – Freshwater Ecosystems, Stratospheric Ozone, Ionising Radiation, Ozone Formation – Human Health, Particulate Matter Formation, Ozone Formation – Terrestrial Ecosystems, Terrestrial Acidification, Freshwater eutrophication, Marine eutrophication, Terrestrial ecotoxicity, Freshwater ecotoxicity, Marine ecotoxicity, Human carcinogenic toxicity, Human non-carcinogenic toxicity, Land use, Mineral resource scarcity, Fossil resource scarcity, Water use. This approach makes it possible to analyse combined indicators of damage to resources, ecosystems and human health (Maceno et al. Citation2022).
Statistical analysis
Statistical analysis was provided using R software version 4.1.1. (Bell Laboratories, New Jersey, U.S.). Normality was checked using the Shapiro-Wilk test (p > 0.05). One-way analysis of variance (ANOVA) and the Duncan test were used to determine the significant differences at a significance level of p < 0.05.
Results
According to Sinner, each of the four factors that affect overall cleaning performance (i.e. time, temperature, mechanical action, and chemistry) can, in principle, be offset by one of the other three. For example, a decrease in wash temperature can be offset by an increase in detergent concentration. For this reason, various increased concentrations of the ECE reference detergent were tested in the low-temperature washing scenarios studied at 40°C and the standard soil removal efficiency achieved was compared to that obtained when washing at 60°C with the addition of the prescribed concentration of the ECE reference detergent, i.e. 5 g/l. The results are shown in . As can be seen, the removal efficiency of the tested standard soils is not only influenced by the concentration of the ECE reference detergent, but also by the type of soiling. For example, compared to 60°C, the highest cleaning efficiency and thus the highest increase in ΔL* value was obtained in the low-temperature wash scenarios (40°C) for Empa 160 (chocolate), followed by Empa 101 (carbon black/olive oil), Empa 116 (blood/milk/ink) and Empa 114 (red wine). Accordingly, gradually increasing the concentration of ECE reference detergent 98 in the low-temperature washing scenarios affected increasing the removal of Empa 101, Empa 116, Empa 160 and Empa 114 (p < 0.05; Table S2). Thus, compared to 60°C and 5 g/L ECE reference detergent, a higher cleaning performance was achieved at 40°C, but by adding twice the concentration of the reference detergent. Importantly, further increasing the concentration of ECE reference detergent 98 resulted in a slight reduction of ΔL*, regardless of the standard soil tested.
Figure 1. Lightness difference, ΔLs*, of the tested standard soiled textile washed at 40°C and 60°C with ECE reference detergent (N=20) according to the studied washing scenarios. A statistically significant difference (p < 0.05 for Duncan test following different letters above column for each soil).
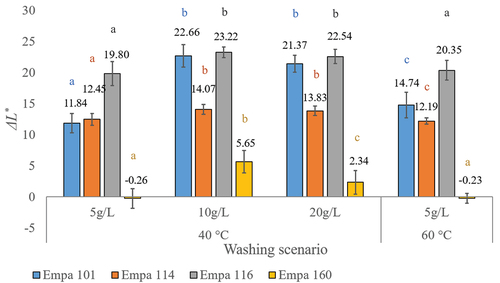
To investigate the bacterial reduction potential, different washing scenarios and bacterial strains were tested. shows the initial load (log CFU/carrier) for each bacterial strain on the cotton carrier and the remaining bacterial cells after each washing scenario (N = 15) (log CFU/carrier). shows that increasing the concentration of ECE reference detergent 98 at 40°C results in a decreasing number of cells for all bacteria tested (p < 0.05; Table S1). At 40°C and a concentration of 5 g/L, the reduction is about 2.8 log CFU/carrier (2.9 log CFU/carrier for E. coli and P. aeruginosa and 2.6 log CFU/carrier for S. aureus), while at 40°C and 20 g/L complete elimination (ND – not detected) of the bacteria from the cotton carrier were achieved. Of particular note in are the result of bacterial reduction at 60°C and the ECE reference detergent concentration 98 of 5 g/L. Here, complete bacterial elimination was demonstrated, which is comparable to the washing scenario at 40°C and a four times higher concentration (20 g/L). In detail, the results of the statistical analysis show significant differences between the washing scenarios in terms of bacteria reduction (Table S1).
Figure 2. E. coli, P. aeruginosa and S. aureus as log CFU/carrier and logarithmic reduction (numbers above columns) on cotton carriers (N=15) after washing regimes combing ECE reference detergent and temperature (ND – not detected). A statistically significant difference (p < 0.05 for Duncan test following different letters above column for each bacterial strain).
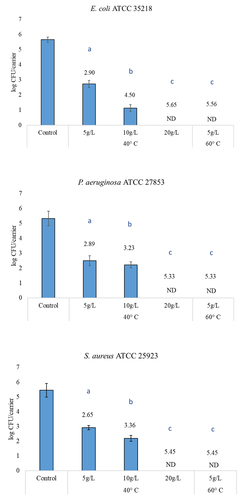
The results from LCA show that the washing scenario 40°C and 20 g/L ECE reference detergent causes the highest environmental impact (12.8 mPt), followed by the washing scenario 40°C and 10 g/L ECE reference detergent (7.65 mPt), 60°C and 5 g/L ECE reference detergent (7.41 mPt), and the lowest environmental impact for the washing scenario 40°C and 5 g/L ECE reference detergent (5.09 mPt) (). All this show that increasing the detergent concentration for balancing the reduced energy consumption will cause a higher environmental impact. A more detailed analysis shows that for the wash cycles at 40°C, the majority of the environmental impact is for the ECE reference detergent 5 g/L (2.55 mPt; 50%), 10 g/L (5.11 mPt; 67%), 20 g/L (10mPt; 80%). In the case of the washing scenario at 60°C and 5 g/L ECE reference detergent, the largest environmental impact is electricity (4.17 mPt; 56.28%) ().
Discussion
The initiative to reduce the temperature of washing household laundry can only be implemented if the quality of washing laundry is maintained compared to high temperatures. This is the first study that has assessed the balance between the quality of household laundry washing and the impact on the environment. We have confirmed that depending on the type of soiling, the removal of standard soiling is equivalent or even more efficient when washing at 40°C than when washing at 60°C, but with a higher concentration of the standard ECE detergent. In a study by Drol et al. (Citation2012), it was shown that among the factors studied (i.e. temperature, detergent and type of soil, wash cycles), the cleaning performance of a washing process is most influenced by temperature. Indeed, at a higher temperature, the kinetic energy of the surfactants and their adsorption properties on the substrate increase, facilitating the removal of soil. Therefore, to achieve the same soil removal when washing at low temperatures as when washing at 60°C, concentrated detergents must be used or the washing time should be extended. This certainly facilitates the removal of greasy or particulate soils, as a higher amount of surfactants allows them to interact with water molecules, removing the soils from the substrate surface and further emulsifying, solubilising or suspending them in the wash bath. The increase in cleaning performance by increasing the ECE reference detergent 98 is readily apparent from the results in while increasing the ECE standard detergent concentration twice (from 5 g/L to 10 g/L) results in an increase in the ΔL* value of the standard soil Empa 101, Empa 116 and Empa 160 by 2.3, 1.2 and 5.0 times, respectively. While Empa 101 contains only detergent (i.e. surfactant and builder) related soils, Empa 116 and Empa 160 are detergents and enzyme-related soils, so it would be reasonable to expect their much higher removal efficiencies during low-temperature wash. Since the ECE reference detergent does not contain enzymes, the ΔL* increase in the low-temperature wash scenario (40°C) is entirely due to the detergent. Empa 114, on the other hand, belongs to the oxidisable stains and is therefore influenced by the presence of bleach in the detergent. As the latter was not added to the ECE reference detergent, the ΔL* of Empa 114 remained approximately the same regardless of the washing scenario. The detergent industry has adapted well to the demand for washing at low temperatures, as various studies confirm the good cleaning performance of concentrated commercial detergents at 30°C and 40°C (Cameron Citation2007; Laitala and Jensen Citation2010; Ferri et al. Citation2016; Tavčer Citation2020). But it should also be noted that more detergent does not always mean more washing performance in household washing (Gülümser Citation2010). As can be seen from the results, the ΔL* of the standard soils Empa 101, 116 and 160 increased only up to a concentration of 10 g/L of the ECE reference detergent 98, while its further increase (i.e. 20 g/L) resulted in impaired removal of all standard soils tested. The reason for this can be explained as follows: As the concentration of the surfactant in the aqueous solution increases, and above a certain point known as the critical micelle concentration (CMC), the surface tension of the solvent (i.e. water; γL) reaches its lowest possible value, while the molecules of the surfactant form aggregate known as micelles, with hydrophobic interior (tail) located in the centre of the clusters and hydrophilic exterior (head) facing the solvent. The CMC is known to be the optimal concentration of surfactant in the detergent that ensures maximum washing performance. The formation of micelles improves the solubilization of organic impurities because the impurities are trapped in the hydrophobic core of the micelles by hydrophobic forces. Consequently, their solubility in aqueous solutions increases. However, further increase of detergent concentration in the wash bath beyond the CMC of the surfactant, which must have occurred at the highest detergent concentration studied, has a significant impact on various physical properties of the aqueous solution, the most important being the renewed increase in γL, which in turn reflects impaired wettability properties of the wash bath and, consequently, an impairment of the washing performance. Moreover, at higher surfactant concentrations, surfactant adsorption can also increase the hydrophobicity of soil surfaces, which promotes the readsorption of dissolved organic contaminants onto the soil surfaces and further decreases the washing efficiency (Rosen and Kunjappu Citation2012; Li et al. Citation2014). In addition, severe foaming was observed at 20 g/L, which in turn affected the interaction between the surfactant and the soil, thus also affecting the mobilization/solubilization process in the soil (Johansson and Somasundaran Citation2007). Furthermore, the reduction of E. coli, S. aureus and P. aeruginosa bacteria on the cotton carrier in the low temperature (40°C) washing scenario shows that increasing the amount (5 g/L, 10 g/L, 20 g/L) of ECE Standard Detergent 98 results in increased bacterial reduction. Similar was found by Heinzel et al. (Citation2010) when testing detergents against viruses from domestic laundry. No bacteria were detected at a concentration of 20 g/L, as in the case of the 60°C wash scenario and 5 g/L ECE standard detergent 98. These results are in agreement with the results of Schages et al. (Citation2020) who tested washing laundry in the lab-scale washing machine at 40°C and with 5.5 g/L detergent containing sodium perborate. They observed a reduction of S. aureus at 3.8 CFU/carrier and P. aeruginosa at 4 CFU/carrier. In addition, when the laundry was washed at 60°C, complete elimination (LR > 5 log CFU/carrier) of the bacteria was observed. In addition, Zinn et al. (Citation2022) analysed bacterial communities in washing machines and found that typical bacteria associated with humans were staphylococci, P. aeruginosa and faecal bacteria. However, the authors also reported that low washing temperatures cause microbial colonisation, proliferation and even biofilm formation. Another study by Honisch et al. (Citation2016) tested different washing regimes and reported that commercial detergent (4.3 g/L) caused 2.3 log CFU of P. aeruginosa at 40°C, which is comparable to our study. Furthermore, the authors emphasised that the complete elimination of bacteria at low temperatures is only possible when active oxygen bleach is added to the commercial detergent. Similarly, our previous study showed that adding 3% hydrogen peroxide to the main wash eliminated bacteria when washing at low temperatures (Tavčer et al. Citation2022). Similarly to that Amichai et al. (Citation2013) analysed the eradication of fungi and yeast from socks in domestic washing and found that yeasts were eradicated at 40°C. Contravening current trends for energy saving and environmental protection, laundering at low temperatures is not effective in eradicating fungal pathogens, which requires high-temperature laundering at 60°C. Also, Bockmühl et al. (Citation2019) reported that for the inactivation of fungi or gram-negative bacteria higher concentrations are required. In addition, Reynolds et al. (Citation2022) quantified the risk of infection from pathogens when washing household laundry through a risk assessment analysis and found that the risk of intestinal infection when washing at low temperatures is 3.38 × 10−2, while this risk decreases to 1.87 × 10−6 when washing at high temperatures, which is consistent with our results on bacterial reduction. The standard for chemical disinfection of textiles for domestic use (EN17658, 2022) specifies a bacterial reduction of ≥ 4 log CFU/carrier at a wash cycle of 40°C as a criterion. It is not considered that domestic textiles should be without microorganisms like in the case of hospital textiles (>7 log/carrier). The results of our study show that when washing at 40°C with 10 g/L ECE standard detergent 98, this criterion is achieved for E. coli (4.5 log CFU/carrier), while the reduction for S. aureus and P. aeruginosa was too low (3.4 log CFU/carrier and 3.2 log CFU/carrier). However, ensuring proper laundry hygiene at low temperatures and higher detergent or electricity consumption is necessary and has worrying environmental implications. The LCA of our four washing processes shows significant differences in terms of environmental impact and contribution of the processes. In this study, it is noticeable that increasing the washing set temperature from 40°C to 60°C increases the energy consumption from 0.340 kWh to 0.764 kWh and more than doubles the environmental impact (1.85 mPt to 4.17 mPt). Similarly, Shahmohammadi et al. (Citation2018) analysed the GHG footprint of washing machines in 23 EU countries at different temperature settings and found that washing at 40°C produces an average of 300 g CO2 equivalents per wash cycle while washing at 60°C produces 580 g CO2 equivalents per wash cycle, which is comparable to our results. In addition, Eberle et al. (Citation2007) presented an LCA analysis of laundry disinfection processes at high (70°C) and low (40°C) temperatures and found that the hurdle approach with low temperature and peracetic acid reduced energy consumption by 55% and the potential for global warming and acidification by almost half. A detailed analysis of our results shows that for washing at 40°C, the most important contributor to the total environmental impact is detergent, which accounts for 50%, 67% and 80% of the eco-points in the 5 g/L, 10 g/L and 20 g/L scenarios, respectively. A more detailed analysis shows that global warming and particulate matter are the most important impact categories, especially for the 40°C, 20 g/L scenarios (Figure S1). Our study demonstrates varying temperatures and detergents in the washing cycle have an impact on both soil removal and bacterial reduction. Increasing the temperature or detergent concentration will result in better bacterial reduction while increasing the pressure on the environment. At the same time low and high-temperature washing will not benefit soil removal and doubling the concentration (from 10 g/L to 20 g/L) will either not remove more soil. Although washing scenario 40°C and 10 g/L shows good results from a soil and bacteria management point of view, the approach is not justified from an environmental perspective. The results demonstrate that balancing the reduced energy consumption by increasing the detergent concentration while maintaining the proper washing quality will result in higher environmental impacts. Substitution of harmful substances in detergents with less hazardous ones would reduce the environmental impact per wash cycle and therefore contribute to more sustainable household laundry. For example, Rebello et al. (Citation2020) compared synthetic and bio-based detergents at LCA and found that bio-based detergents have significantly lower impacts on fossil fuel consumption, respiratory organic compounds and climate change compared to classical petroleum-based detergents. Another approach to increase laundry quality would be the application of a longer contact time of textiles with detergent (Tavčer et al. Citation2022). Howsoever since electricity consumption also represents an important part of total environmental impact, a more energy-efficient washing machine would be the next approach to more sustainable laundry. And nonetheless transition to renewable sources of electricity e.g. photovoltaic would further reduce the pressure on the environment. Managing energy and resources is one of the principles of sustainable development (Ruggerio Citation2021). However, reducing energy consumption in laundry must be done without compromising the effectiveness of soil and bacteria reduction and should not be accompanied by an increase in detergent consumption. Simultaneously developing natural, environmentally friendly and highly effective detergents and reducing energy consumption will enable humanity to move towards a sustainable, healthy and equitable society.
Conclusions
Reducing environmental impact on the one hand and maintaining household quality on the other is a demanding challenge. This study has shown that the removal of soil and bacteria reduction on textiles is detergent and temperature dependent. Increasing the detergent concentration at 40°C results in higher standard soil removal, but only up to a detergent concentration of 10 g/L, as further increases impair the detergent’s cleaning performance. In the meantime, the total bacterial reduction can be achieved either by increasing detergent consumption (20 g/L) at low temperatures (40°C) or by heating the water (60°C) at low detergent consumption (5 g/L). The LCA shows that increasing the detergent concentration as the balance for low-temperature washing while sustaining the proper washing quality is not justified from an environmental point of view. Either way, household laundry washing could reduce its environmental impact in the future by switching to less hazardous detergents and new energy sources without compromising the quality of the wash.
Supplemental Material
Download MS Word (362.4 KB)Disclosure statement
No potential conflict of interest was reported by the authors.
Supplemental data
Supplemental data for this article can be accessed online at https://doi.org/10.1080/09603123.2023.2194615.
Additional information
Funding
References
- Abney SE, Ijaz MK, McKinney J, Gerba CP. 2021. Laundry hygiene and odor control: state of the science. Appl Environ Microbiol. 87(14):e03002–03020. doi:10.1128/AEM.03002-20.
- Amichai B, Grunwald MH, Davidovici B, Farhi R, Shemer A. 2013. The effect of domestic laundry processes on fungal contamination of socks. Int J Dermatol. 52(11):1392–1394. doi:10.1111/ijd.12167.
- Bockmühl DP, Schages J, Rehberg L. 2019. Laundry and textile hygiene in healthcare and beyond. Microbial Cell. 6(7):299. doi:10.15698/mic2019.07.682.
- Cameron BA. 2007. Laundering in cold water: detergent considerations for consumers. Fam Consum Sci Res J. 36(2):151–162. doi:10.1177/1077727X07308159.
- Chen F, Ji X, Chu J, Xu P, Wang L. 2021. A review: life cycle assessment of cotton textiles. Ind Textila. 72(01):19–29. doi:10.35530/IT.072.01.1797.
- Dewaele J, Pant R, Schowanek D, Salducci N 2006. Comparative Life Cycle Assessment (LCA) of Ariel “Actif à froid”(2006), a laundry detergent that allows to wash at colder wash temperatures, with previous Ariel laundry detergents (1998, 2001). Procter & Gamble, Brussels Innovation Center, Central Product Safety-Environmental: Brussels.
- Drol P, Kert M, Simoncic B, Hladnik A. 2012. Evaluation of the influence of different parameters in removing standard soil from cotton fabric using multi-factorial analysis of variance. Tekstilec. 55(3):194–205.
- Eberle U, Lange A, Dewaele J, Schowanek D. 2007. LCA study and environmental benefits for low temperature disinfection process in commercial laundry (12 pp). Int J Life Cycle Assess. 12(2):127–138. doi:10.1065/lca2006.05.245.
- Ferri A, Osset M, Abeliotis K, Amberg C, Candan C, Owens J, Stamminger R. 2016. Laundry performance: effect of detergent and additives on consumer satisfaction. Tenside Surfactants Deterg. 53(4):375–386. doi:10.3139/113.110451.
- Gülümser T. 2010. Soil releasing effect of concentrated detergents compared with the ordinary ones. Textile and Apparel. 20(4):329–335.
- Heinzel M, Kyas A, Weide M, Breves R, Bockmühl DP. 2010. Evaluation of the virucidal performance of domestic laundry procedures. Int J Hyg Environ Health. 213(5):334–337. doi:10.1016/j.ijheh.2010.06.003.
- Honisch M, Brands B, Weide M, Speckmann H-D, Stamminger R, Bockmühl DP. 2016. Antimicrobial efficacy of laundry detergents with regard to time and temperature in domestic washing machines. Tenside Surfactants Deterg. 53(6):547–552. doi:10.3139/113.110465.
- Honisch M, Stamminger R, Bockmühl DP. 2014. Impact of wash cycle time, temperature and detergent formulation on the hygiene effectiveness of domestic laundering. J Appl Microbiol. 117(6):1787–1797. doi:10.1111/jam.12647.
- Johansson I, Somasundaran P. 2007. Handbook for Cleaning/decontamination of Surfaces. Amsterdam: Elsevier.
- Laitala K, Jensen HM. 2010. Cleaning effect of household laundry detergents at low temperatures. Tenside Surfactants Deterg. 47(6):413–420. doi:10.3139/113.110096.
- Li H, Chen J, Jiang L. 2014. Elevated critical micelle concentration in soil–water system and its implication on PAH removal and surfactant selecting. Environ Earth Sci. 71:3991–3998. doi:10.1007/s12665-013-2783-3.
- Maceno MMC, João S, Voltolini DR, Zattar IC. 2022. Life cycle assessment and circularity evaluation of the non-medical masks in the Covid-19 pandemic: a Brazilian case. Environ Dev Sustainability. 1–28. doi:10.1007/s10668-022-02388-2.
- Monleón-Getino T, Cavalleri M. 2022. International ring trial to validate a new method for testing the antimicrobial efficacy of domestic laundry products. Plos One. 17(6):e0269556. doi:10.1371/journal.pone.0269556.
- Morgan E, Foxon TJ, Tallontire A. 2018. ‘I prefer 30°’?: business strategies for influencing consumer laundry practices to reduce carbon emissions. J Clean Prod. 190:234–250. doi:10.1016/j.jclepro.2018.04.117.
- Morsali S. 2017. Damage assessment of bitumen refineries using simapro (LCA) inventory data. J Pet Environ Biotechnol. 8(1):317. doi:10.4172/2157-7463.1000317.
- Murray CJ, Ikuta KS, Sharara F, Swetschinski L, Aguilar GR, Gray A, Han C, Bisignano C, Rao P, Wool E, et al. 2022. Global burden of bacterial antimicrobial resistance in 2019: a systematic analysis. Lancet. 399(10325):629–655. doi:10.1016/S0140-6736(21)02724-0.
- Rebello S, Anoopkumar A, Sindhu R, Binod P, Pandey A, Aneesh EM. 2020. Comparative life-cycle analysis of synthetic detergents and biosurfactants—an overview. Refining Biomass Residue Sustainable Energy Bioprod. 511–521.
- Reynolds KA, Verhougstraete MP, Mena KD, Sattar SA, Scott EA, Gerba CP. 2022. Quantifying pathogen infection risks from household laundry practices. J Appl Microbiol. 132(2):1435–1448. doi:10.1111/jam.15273.
- Riley K, Williams J, Owen L, Shen J, Davies A, Laird K. 2017. The effect of low-temperature laundering and detergents on the survival of Escherichia coli and Staphylococcus aureus on textiles used in healthcare uniforms. J Appl Microbiol. 123(1):280–286. doi:10.1111/jam.13485.
- Rosen MJ, Kunjappu JT. 2012. Surfactants and interfacial phenomena. New Jersey: John Wiley & Sons.
- Ruggerio CA. 2021. Sustainability and sustainable development: a review of principles and definitions. Sci Total Environ. 786:147481. doi:10.1016/j.scitotenv.2021.147481.
- Sa Z, Da P, Hb L, Ma L. 2020. The application of home-made Achilles tendon teaching aids combined adhesive tape in explaining Achilles tendon teaching. Asian J Surg. 44(2):494–495. doi:10.1016/j.asjsur.2020.11.024.
- Schages J, Stamminger R, Bockmühl DP. 2020. A new method to evaluate the antimicrobial efficacy of domestic laundry detergents. J Surfactants Deterg. 23(3):629–639. doi:10.1002/jsde.12401.
- Shahmohammadi S, Steinmann Z, Clavreul J, Hendrickx H, King H, Huijbregts MA. 2018. Quantifying drivers of variability in life cycle greenhouse gas emissions of consumer products—a case study on laundry washing in Europe. Int J Life Cycle Assess. 23(10):1940–1949. doi:10.1007/s11367-017-1426-4.
- Shin Y, Park J, Park W. 2020. Sterilization efficiency of pathogen-contaminated cottons in a laundry machine. J Microbiol. 58(1):30–38. doi:10.1007/s12275-020-9391-1.
- SimaPro. 2016. Introduction to LCA with SimaPro. Retrived from https://wwwpresustainabilitycom/legacy/download/SimaPro8IntroductionToLCApdf.
- Sohn J, Nielsen KS, Birkved M, Joanes T, Gwozdz W. 2021. The environmental impacts of clothing: evidence from United States and three European countries. Sustainable Prod Consumption. 27:2153–2164. doi:10.1016/j.spc.2021.05.013.
- Tavčer PF. 2020. Influence of bleach activators in removing different soils from cotton fabric. Fibres Text East Eur. 28(3(141)):74–78. doi:10.5604/01.3001.0013.9023.
- Tavčer PF, Brenčič K, Fink R, Tomšič B. 2022. Influence of hydrogen peroxide on disinfection and soil removal during low-temperature household laundry. Molecules. 27(1):195. doi:10.3390/molecules27010195.
- Yates L, Evans D. 2016. Dirtying Linen: re-evaluating the sustainability of domestic laundry. Environ Policy Governance. 26(2):101–115. doi:10.1002/eet.1704.
- Zinn M-K, Flemming H-C, Bockmühl D. 2022. A comprehensive view of microbial communities in the laundering cycle suggests a preventive effect of soil bacteria on malodour formation. Microorganisms. 10(7):1465. doi:10.3390/microorganisms10071465.