ABSTRACT
An information gap exists in the construction industry, where stakeholders possess information that is not effectively shared or sufficiently detailed. The information gap increases uncertainty in decision-making processes, leading to design conservatism, project delays and errors. Reducing over-design and waste in concrete practice is critical to achieving greater sustainability. Bridging the information gap, therefore, supports sustainable change and can be reinforced by digital transformation that aids information availability. Nevertheless, the complexity and current ambiguity in the information requirements of stakeholders in the supply chain pose a substantive challenge to effective implementation. To this end, this study develops a process flow model for the concrete sector constructed using a mixed method approach of a questionnaire, interviews, and an industry workshop. The information requirements and actions of all stakeholders in the system are collectively mapped for the first time. By developing process understanding, the model demonstrates that the current document-centric approach adopted by the sector contributes to the information gap. Furthermore, the developed process understanding aids in targeted digital transformation to tackle the information gap in practice.
Introduction
Concrete use is responsible for around 8% of global greenhouse gas emissions, primarily due to the production of ordinary Portland cement (OPC) (Miller et al., Citation2018). Despite a 20% reduction in the embodied carbon of concrete since 1990 through clinker substitution and fuel switching, the rapid increase in production has caused a threefold rise in concrete-related emissions from 1990 to 2020 (Scrivener et al., Citation2018). With the escalating consumption of concrete, there is an urgent need to improve lifecycle efficiency, from design and construction to usage and waste management, to mitigate emissions (Watari et al., Citation2023). Studies suggest that nearly 50% of emissions can be curtailed through material efficiency (Kazaz et al., Citation2015; Shanks et al., Citation2019).
The construction industry strives to enhance resource utilization and implement circular economy principles to improve environmental sustainability. However, progress is hindered by challenges such as insufficient knowledge transfer and limited cross-functional cooperation (Sawhney et al., Citation2020). Digital transformation, aimed at improving information flow across the supply chain so that the necessary information is available at the right time to make informed decisions, is seen as an opportunity for achieving sustainability goals (Bastos et al., Citation2023). However, current digital initiatives often result in data overload, hindering effective decision-making and underscoring the need for a fundamental understanding of the process to be developed before building digital systems for information transfer (Lawrenz et al., Citation2021).
Process flow models, which diagrammatically represent sequences of actions, have proven effective in other industries for simplifying complex processes and identifying issues with information flow (Heher & Chen, Citation2017). However, their application in the construction sector remains limited. This research aims to develop a process flow model that maps key stakeholder actions and information flows at various project stages. To do so, the information requirements of each stakeholder in the system must first be identified. By addressing current deficiencies, the study will provide a comprehensive understanding of large-scale concrete processes, guiding more effective digital transformation efforts in the sector.
Literature review
Worldwide cement use is dominated by the ready-mix concrete sector, governed by several large producers (Cave et al., Citation2014; Syverson, Citation2008). This area of practice is referred to as large-scale concrete activity. Given increasing concrete consumption, there is a growing focus on improving the efficiency of design, construction, use and waste throughout the lifecycle of concrete structures, and it is estimated that around 25% potential material savings are available via the reduction of over-design and waste (Kazaz et al., Citation2015; Shanks et al., Citation2019). Some of the key aspects driving over-design and waste in the concrete sector are described in .
Table 1. Causes of over-design and waste (Favier et al., Citation2018; Kazaz et al., Citation2015; Shanks et al., Citation2019).
Concrete sector
The concrete sector is a complex system with several stakeholders, including clients, designers, site contractors, concrete and admixture producers, and procurement professionals. At a high level, the concrete producer is responsible for delivering concrete that conforms to the specifications and requirements of the site contractor and designer. The concrete producer may work closely with an admixture producer who can offer products to add to the concrete mix to meet requirements in a cost-effective and sustainable manner. The site contractor will be responsible for placing the concrete. The client will set the project’s overall aims, which are to be adhered to by all other stakeholders. Procurement representatives will primarily appoint project teams, such as the concrete producer, negotiate contracts, and respond to cost queries.
These stakeholders depend on one another to contribute to efficient, high-quality and timely information flow to reduce uncertainty in decision-making and enhance final product quality (Badenhorst-Weiss et al., Citation2013; Vaidyanathan et al., Citation2020). suggests that while concrete materials flow in one direction along the concrete supply chain, information flow should ideally be bidirectional between stakeholders to prevent material and information waste over the product lifecycle (Bishop, Citation1972). Various authors suggest this is not the case within the construction industry, and numerous information gaps exist (Joseph & Chandran, Citation2023; Lawrenz et al., Citation2021; Noche & Elhasia, Citation2013). These information gaps contribute to uncertainty, delays, and mis-ordered materials (Lawrenz et al., Citation2021; Man-Shi & Ochsendorf, Citation2005). Each stakeholder has limited knowledge about how others use shared information and the level of detail required (Joseph & Chandran, Citation2023). Currently, the lack of information linkages between stakeholders has contributed to divergent and conflicting sustainability approaches (Miller, Citation2021; Titus & Bröchner, Citation2005). In the absence of information, overly cautious decisions are often made based on worst-case scenarios. This leads to lower material efficiency as these conditions rarely occur. Effective information flow ensures stakeholder coordination that reduces rework and waste and aids accurate material tracking for circular construction (Joseph & Chandran, Citation2023; Lawrenz et al., Citation2021; Stowe et al., Citation2020).
Figure 1. Traditional concrete supply chain, material flow adapted from Noche and Elhasia (Citation2013).
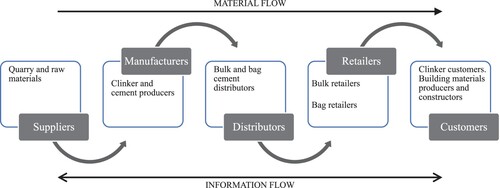
Ambiguity in information requirements between stakeholders poses a substantive challenge to digital methods attempting to bridge these information gaps (Graham et al., Citation2005; Stowe et al., Citation2020; Titus & Bröchner, Citation2005). Furthermore, establishing information requirements within construction projects is becoming more challenging due to the growing complexity of projects and supply chains (Royal Institute of British Architects, Citation2021). The RIBA Plan of Work, commonly used in the United Kingdom, sets out the critical stages to plan and manage construction projects. The Plan of Work requires stakeholders to specify information needs at each project stage but does not provide detailed examples. The RIBA Plan of Work promotes stakeholders to provide information in a document-centric manner at formal stage milestones rather than a free-flowing data-centric approach within project stages. This is because it is currently unclear what information is to be produced, what tasks underpin the information requirements and for what purposes the information will be used (Royal Institute of British Architects, Citation2021). A crucial consideration, therefore, is which information to transfer and which to disregard throughout the process, reflecting a more mature approach to the management of data (Hossain et al., Citation2020; Joseph & Chandran, Citation2023; Kaipia, Citation2009).
Some work to develop an understanding of construction processes has been completed. maps the integration dimensions of time, function, and stage for construction projects. The dimensions also align with the RIBA stages, as shown in (b). Current research on developing an understanding of concrete industry processes is divided into four distinct areas, mapped to the integration dimensions in (a). The first area is research that has considered material management alongside vertical integration of the supply chain (Chen et al., Citation2022; Graham et al., Citation2005; Noche & Elhasia, Citation2013). The second area is the optimization of concrete production plants, including digitalization (Miller, Citation2021; Zhang et al., Citation2021). The third area (and largest body of research) focuses on optimizing the delivery process, including the simulation of dispatch and pouring methods incorporating schedule-orientated supply chain coordination (Chen et al., Citation2021; Maghrebi et al., Citation2014; Park et al., Citation2011; Phan & Athigakunagorn, Citation2022; Sholeh et al., Citation2018). A final research area focuses on material and information mapping to capture the information flow in precast manufacturing plants (Ergen & Akinci, Citation2008; Wang et al., Citation2020). The vast differences between the actions and stakeholders present in ready mix and precast concrete processes mean that the research within this final area leaves large gaps in transferring knowledge across to large-scale activity. Research in these distinct areas has mainly focused on specific stages and stakeholders, overlooking more than half of the system, as illustrated in . When combined, these existing process research areas offer an incomplete understanding of the overall process, with a limited stakeholder representation. Breaking the system into smaller sections leads to a loss of knowledge about the information transferred both within and between project stages, which is a key consideration for identifying and bridging information gaps.
Figure 2. (a) the integration dimensions of large-scale concrete activity, with highlighted current research areas, adapted from (Fergusson & Teicholz, Citation1996) (b) relationship between RIBA stages and planning, design and construction.
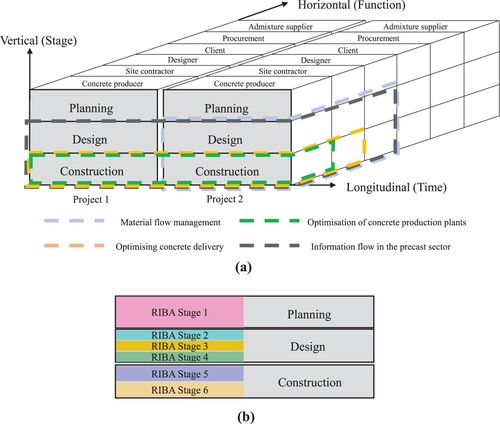
Process flow models have been deployed in other sectors as the first step on the process efficiency improvement journey, and these methods have been successfully applied in large supply chains to map and redesign complex processes (NHS Modernisation Agency, Citation2002). Despite this, the use of process flow models is underutilized in the construction industry and concrete sector. demonstrates one of the earliest published process mapping exercises completed for the wider construction sector. While this work highlights the roles of multiple stakeholders across multiple stages, it does not detail materials. It is mainly concerned with the commercial aspects of the project, including costing and payment. and provide a more detailed view of concrete production and construction. However, again, these contributions only cover a small part of the system, which results in an incomplete representation of stakeholders and their roles over time. While some process understanding at an industry level has been developed, notably by The Department of the Environment (Citation1971), authors have championed frameworks to be used within specific disciplines to capture sufficient detail (Bishop, Citation1972). In this study, a process flow model for the concrete sector is set out in detail; the following section describes the research methodology adopted to develop the model.
Figure 3. Idealized construction process, adapted from Bishop (Citation1972).
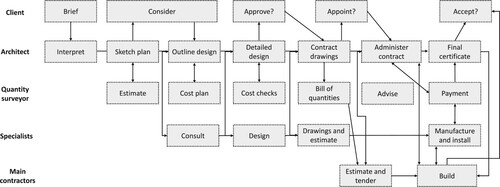
Figure 4. Ready mix concrete value chain, adapted from Graham et al. (Citation2005).
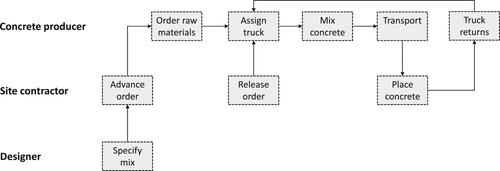
Figure 5. Process flow for the concrete sector, adapted from Man-Shi and Ochsendorf (Citation2005).
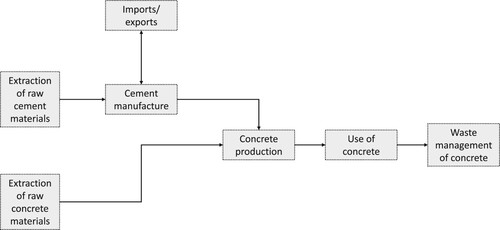
Research methodology
As described in , a mixed-method approach was adopted to construct the process flow model. The approach was designed with each element complementing the others to ensure that the process flow model is comprehensive and robust. The data-gathering approach focussed on participants within the UK. Methods to expand the application of the model for other regions are discussed later. To gain a wider range of insights into the stakeholder information requirements, an industry questionnaire was used. To obtain more in-depth insights into the actions of each stakeholder and the flow of the information requirements over time, a focused set of interviews was conducted. The use of surveys and interviews is encouraged in operational and process research, particularly during the early stages, where preliminary insights are sought with a range of potential viewpoints (Forza, Citation2002). Finally, an industry workshop was used to validate the robustness of the developed process flow model. Workshops with experts are an established mechanism to validate research findings (Ørngreen & Levinsen, Citation2017). Each of these approaches is discussed in detail herein.
The questionnaire aimed to identify stakeholder’s critical information requirements rather than solely focusing on the process itself. The information requirements obtained from the questionnaire are provided as a list of discrete pieces of information called information points in the following section. To obtain the appropriate information points, each survey participant was asked to highlight which information points were necessary for completing their associated actions (see Supplementary material for details of survey questions). Participants were also asked which stakeholders they exchanged information with, which offered high-level indications of the level of interaction between the different stakeholders in the system. Representatives from concrete industry bodies, such as the UK Mineral Products Association, were consulted to aid survey distribution. These key contacts helped to distribute the questionnaire across key stakeholder groups: clients, concrete producers, designers, site contractors, procurement professionals, and admixture suppliers. summarizes the characteristics of the 63 survey respondents. The goal was not to achieve statistically significant results but to ensure the process flow model had representative input across the key stakeholder groups.
Table 2. Characteristics of the 63 survey respondents.
Following the identification of the stakeholder information points from the questionnaire, 13 interviews were held to interrogate the flow of information in more depth. Interviews were utilized for this purpose as they facilitated a deeper dive into the time elements of the stakeholder actions and of the information transfer, which was unable to be robustly captured using the questionnaire. summarizes the characteristics of the 13 interview participants. This demonstrates that the participants worked across multiple sectors on projects of different values. Six of the interview participants had taken part in the previous questionnaire.
Table 3. Characteristics of the 13 interview participants.
The structure of the interviews was divided into two main sections. The first section explored the key information points gathered from the questionnaire. The participants were asked to list which stakeholders this information was shared with at which time point and for which actions each information point was used. Each information transfer was categorized based on whether it was necessary for taking immediate action or for information-sharing purposes without immediate action. The second section of the interview focused on how the information points were currently recorded and how this could be improved, i.e. how the information gap could be bridged. The findings from the questionnaire and interviews informed the first draft of the process flow model as the key actions and information transfers over time were now identified.
A workshop was then held to validate the accuracy and robustness of the draft process flow model, with representatives from each stakeholder group present. Twenty-two participants participated in the two-hour online workshop, including five site contractors, five concrete producers, four designers, two procurement professionals, two admixture producers, and four client representatives. Some of the participants in the workshop had participated in the questionnaire, but many of the participants had not been previously involved in the project. Across the questionnaire, interviews and workshop, a total of 82 different participants were consulted. In the first part of the workshop, three breakout groups were set up, with the participants approximately evenly divided between these groups. The process flow model was hosted on a dynamic platform that facilitated live editing of the model. Each group independently worked to validate the model by ‘walking’ through the model to validate that the information points and actions accurately depict those occurring in practice. Participants would highlight sections that appeared to be inaccurate. In the second part of the workshop, the comments were consolidated, and each proposed change was considered. Each change required agreement from at least two of the three groups until the final model was set.
Process flow model
Stakeholder information requirements
Identifying the information requirements of each stakeholder is a critical step in bridging the information gap and creating a process flow model. A summary of the top ten information points highlighted by each stakeholder in the questionnaire, and therefore adopted in the developed process flow model, is presented in . More than ten key information points are presented where the same number of stakeholders selected the information point, and a singular 10th ranking could not be recognized. An inadequate number of responses were received from procurement professionals (less than 5% of responses) to confidently derive the key information points from the questionnaire. Nevertheless, the in-depth interviews validated the role of procurement professionals in the process and the information points and actions are presented in the developed process flow model accordingly. The information points are presented in order of priority, with the most mentioned information points occurring higher up the list. Although there is a commonality in the stakeholders’ information requirements, each stakeholder has distinct aims and aspects required to facilitate their role.
Table 4. Stakeholder information points.
Two main themes emerged within the information requirements presented by the study participants. The first group of requirements relates to higher-level aspects such as cost, environmental conditions, material availability or target concrete strength. This group of information requirements is referred to as key information points herein. Compressive strength and durability are the key information points for the concrete and admixture producer. For the designer, durability and embodied carbon content are vital. For the client, cost and durability are key information points, while the site contractor concentrates on concrete quantity and testing, particularly while concrete remains in a fresh state.
The second group of information requirements is technical information related to the details of the concrete mix design, such as the water/cement ratio and the aggregate size adopted. Concrete producers and site contractors highlighted the mix proportions and water/cement ratio as critical aspects. There was generally a significant crossover between the technical information points selected by the concrete producer and the technical information points chosen by the site contractor. The admixture supplier was most concerned about the water/cement ratio and cement content and type. The client appeared to link the technical information points and carbon, selecting cement content and replacement as key technical information points for their role. Finally, the designer was most concerned with cement type, aggregate size and working life.
Stakeholder information interactions
The nature of the cross-stakeholder information exchange relationships was assessed following the research methodology outlined previously. Each questionnaire participant was asked to highlight the stakeholders with whom they directly interacted. This high-level interaction analysis guided the later interview discussions about which stakeholders were responsible for transferring which information points. Here, direct interaction indicates that the stakeholder initiates interaction with another stakeholder.
The questionnaire responses indicatively demonstrated that stakeholders within the same role regularly interacted with one another, for example, site contractors with other site contractors or designers with other designers. While the cross-stakeholder interaction between site contractors and designers was reported to be strong, the concrete producers were seen to interact much less with the other stakeholders. The indicated strength of the cross-stakeholder relationships had implications for information sharing across the supply chain. Although all concrete producers were aware of the carbon content of the delivered concrete mixes, only half of the site contractors and designers claimed to have knowledge of this. Responses to a further survey question indicated that a lack of information surrounding the carbon content of the concrete mix led to a general dissatisfaction with the stakeholder’s ability to control the mix’s carbon emissions. These indicative results suggest that stakeholders are not effectively sharing information despite its availability in the supply chain, highlighting the presence and significance of the information gap in practice.
The exchange of specific information points was examined during the interviews. Interview participants were asked to identify which stakeholders were responsible for sending and receiving the information points identified from the questionnaire in . The results of this process are presented in and . Each technical information point is assigned a reference, e.g. T1 for cement type, and each key information point is assigned a reference, e.g. I1 for quantity of concrete. These references are carried forward to the process flow model. There appears to be a primary highway transferring information between the concrete producer, designer, and contractor. These results also suggest that the site contractor has a unique role in sending and receiving the key information points, such as concrete quantities (I1), most commonly between the designer and concrete producer. Target compressive strength (I9) is a further example of this information highway where the information is sent from the designer and received by the site contractor, who then sends it forward to the concrete producer. This highway for information exchange reflects the interactions between stakeholders presented in . outlines the transfer mechanisms for the technical information points. For the technical information points, the concrete producer takes a more active role in sending and receiving the information points, often between the designer and the contractor, such as cement type (T1) and target water/cement ratio (T4).
Table 5. Transfer of key information points.
Table 6. Transfer of technical information points.
Once and were obtained, the information points within the system and the stakeholders associated with sending and receiving this information were known. Given the established RIBA time stages, the interview participants were asked about the timing of the information transfer, and hence, the information points could be preliminarily placed within the appropriate time stages. Knowledge of the transfer of the key information points over time facilitated the development of a draft process flow model, which was expanded to include the full stakeholder set and validated using the workshop process described previously.
A process flow model for large-scale concrete activity
The developed process flow model is summarized in the schematic presented in (a). All main stakeholders are represented in the model, with each stakeholder possessing a horizontal stream, and time is along the longitudinal direction of the process flow model. The model follows the journey of concrete use, from the brief being set by the client to the delivery of concrete on site and the creation of the operation and maintenance manual for the ongoing upkeep of the concrete asset. maps the process flow of large-scale concrete activity according to four stages. These four stages involve some aggregation of the RIBA stages 1–6, and an approximate mapping between the stages is presented in (c). Segmenting large-scale concrete activity into the plan, source, produce and deliver stages was found to represent the actual process more accurately, given the feedback received from the project interviews and workshop.
Figure 7. (a) process flow model schematic (b) process understanding encompassing the integration dimensions (c) process sections mapped onto the RIBA stages.
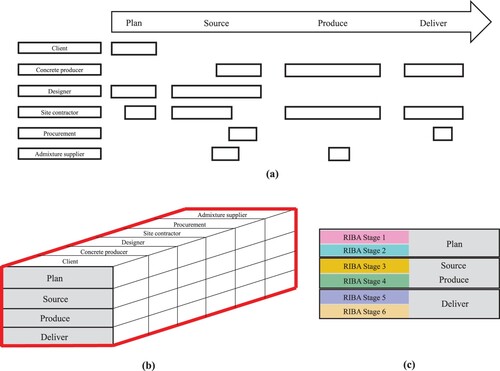
display the complete process flow model for large-scale concrete activity. In the developed process flow model, each stakeholder has a horizontal stream. In the stakeholder streams, a portion is allocated to actions, and a portion is allocated to information transfer. All of the formal actions undertaken by each stakeholder at different stages along the project lifecycle are highlighted. For most of these actions, information points are transferred between supply chain stakeholders. The same notation for the information points, e.g. I1 for concrete quantity, set out in and , is adopted in the process flow model. The project workshop identified several occasions where informal interactions took place within the project timeframe. The workshop participants were keen that such processes were represented in the model and are discussed further in the following model description as they have significant implications for the digital transformation of large-scale concrete activity.
The first stage in the process has been termed ‘Plan’ (). This stage begins with the client’s specification of project location (I18) and programme (I19), environmental conditions (I13) and, recently, any early carbon targets (I15). These aspects are transferred when the project brief and environmental impact assessment are created and shared across the stakeholders. The designer then takes the next step to develop the engineering design. As part of the structural engineering design works, a Building Information Model and preliminary drawings are created, which give insights into estimates of concrete quantities (I1). The site contractor then adopts this for preliminary cost and programme estimates. The key documents created at this stage, e.g. project brief, drawings and programme, may be disseminated to other stakeholders, such as the procurement team, but no immediate actions are undertaken. Informal discussions are held in this stage to introduce the range of stakeholders working on the project and how they will contribute to the remainder of the stages. The focus of this stage is on the actions of the client and designer. This stage broadly aligns with RIBA stages 1–2 (Preparation, Briefing and Concept design).
The second stage has been termed ‘Source’ (). At this point, the preliminary date of concrete delivery (I2) is set by the site contractor, allowing the procurement team to begin forming a requisition for the concrete. This stage progresses to the detailed engineering design phase, whereby the designer sets a preliminary concrete specification, including information points such as its target compressive strength (I9), and appearance (I34), among other aspects. Many of the key technical information points are also specified at this stage, including the cement content (T3) and maximum aggregate size (T8). At this point, the site contractor will review the preliminary mix design and communicate many of the fresh state requirements for successful placement on site, such as the workability (I28) and the approximate rate of strength development (I20) required. The procurement team engages supply partners via enquiries with the concrete and admixture producers, and the supplier details (I3), cost (I16) and embodied carbon (I15) are transferred. Once, engaged, the concrete producer begins the preliminary concrete mix design, selecting suitable materials for the project location and brief, feeding in the earlier aspects set out by the designer and contractor team. Many of the technical information points set out in are transferred at this point, including the target water/cement ratio (T4) and raw material sources (I5), given the concrete producer’s early formation of the concrete mix design. Admixture producers often propose products (T6) for the concrete mix and input on the mix design, and the information related to such products is transferred to the concrete producer. This stage focuses on the actions of the designer and concrete producer, with input from the site contractor. At the end of this stage, the designer typically issues the concrete specification, distributing it to other stakeholders for adoption within the next stages.
The third stage has been termed ‘Produce’ (). At this stage, the concrete producer produces the final mix design, using the reinforcement and construction requirements of the designer and contractor, respectively. Then, the focus shifts to the actions of the site contractor and the concrete producer and the revalidation of the key information points, collecting any changes in the structural design or specification. After detailed reinforcement drawings are made, which transfer information on the reinforcement type (I35) and details on the pours to be made (I7), construction requirements are set, including details of requested sampling and testing (I12), and the concrete producer often compiles trial mixes to ensure conformity. The concrete producer regularly interacts with the admixture producer to update the admixture information (T6). Informal discussions reviewing contract requirements and efforts to lower embodied carbon take place at this stage. A change order process updating concrete quantities (I1) and project programme (I19) is conducted if required. At the end of this stage, the procurement team will likely place the purchase order for an overall estimated concrete quantity. The second and third stages broadly align with the RIBA stages 3 and 4 (Spatial Coordination and Technical Design).
The final stage in the process is termed ‘Deliver’ (), and the focus remains on the actions and collaboration between the site contractor and the concrete producer. The concrete producer and the site contractor liaise on delivery dates (I2), and a route plan and delivery plan are created. The mix details, such as the water/cement ratio (T4), are adjusted for the local weather conditions. Other information points are set to produce the batching ticket, such as the mixing method and time (I25 and I26). Post-delivery, there is a period of monitoring and testing compiled in a quality assurance and post-pour check sheet pack to ensure the strength specifications (I10 and I20) are met. The procurement, contractor and concrete producers teams are included in billing discussions. Finally, the as-built drawings and the operation and maintenance manual are compiled for the constructed asset (I36). This final stage broadly aligns with the RIBA stages 5 and 6 (Manufacturing, Construction, and Handover).
Discussion
Each project stage is currently broadly defined by the release of key documents that collate many of the information points highlighted in the process flow model, as guided by the RIBA Plan of Work. Developing an information model from process understanding is considered best practice for the implementation of effective digital transformation. Key documents in the current process were identified using the stakeholder participation and the developed process flow model. The client is responsible for the project brief and environmental impact assessment documents (‘Plan’ stage – ). The designer is responsible for the concrete specification document (‘Source’ stage – ), the drawing and modelling pack (‘Source’ and ‘Produce’ stages – and ) and the operation and maintenance manual (‘Deliver’ stage – ). The contractor is responsible for the certificate of mix composition and EPD documents (‘Produce’ stage – ) and the batching ticket (‘Delivery’ stage – ). Finally, the procurement team is responsible for the requisition terms and conditions (‘Source’ stage – ) and the purchase order documents (‘Produce’ stage – ). By identifying and collating the key documents in the current process, a current information model is presented in and is discussed in detail herein.
The developed information model adopts Unified Modelling Language (UML) as it embodies best practices for process modelling and provides a standard way to visualize a system (Köller et al., Citation2002). represents a class diagram for the system, describing the types of objects and the various relationships that exist between them. Classes represent groups of objects with similar roles; they are displayed as boxes in . The concrete asset is one class, as are the groups of different stakeholders, such as designers or concrete producers. Different types of documents also each have their own class. Relationships between classes can also be displayed; a line connecting the classes shows that there is a structural link (association) between the two classes. A filled arrowhead indicates a directed association.
The direction arrows indicate which items call other items; for example, rather than the designer’s concrete specification leading to the project client, it is shown that the client can call the designer’s concrete specification. Furthermore, a notation such as 1 … * is used where the concrete asset and contractor are linked; this is read that one or more concrete assets are built by the contractor. Another example is that the site contractor produces a Site Quality Assurance (QA) pack, and concrete producers produce one or more batching tickets.
The concrete asset is the focus of the information system, surrounded by the different actors (stakeholders). The relationships of how the key documents are exchanged between stakeholders are carried over from the process flow model. The number of stakeholder relationships highlights the designer’s concrete specification as a crucial information source. However, for many stakeholders, the concrete specification will include additional information they do not require. Therefore, this represents an inefficient information transfer mechanism for these stakeholders and contributes to the information gap in practice, as described by the study participants. Several of the interviewed stakeholders mentioned waiting for one or more critical documents before making progress or decisions. In some cases, a single piece of information was required from a large document not yet released, causing significant delays. Sometimes, the impact of the information gap was more profound, where assumptions were made about the required information to keep the process flowing despite more accurate information being held by other stakeholders. An example was provided at the ‘Source’ stage in the process, where the designer assumes workability requirements due to a lack of input of the fresh requirements from the contractor before issuing the specification. Incorrectly estimating requirements can lead to later changes. A conservative approach is adopted to prevent such changes, which induces inefficiency, which is often not retrospectively rectified.
The realization of the process flow model has significant implications for the digitalization of the concrete sector, which can be adopted by the industry when attempting to improve current digitalization efforts. First, identifying the information points required by each stakeholder unlocks the potential transfer of discrete information points, offering an improvement over the current document-centric approach. Second, the process flow model highlights several informal interactions that need to be considered when applying digital transformation within the sector. Finally, the identification of key actions and decision points facilitates the development of technologies that automate or better inform decisions at such points. Each of these areas of progression for digital transformation is discussed in detail herein.
The increase in available data resulting from current digital transformation efforts fails to address the issue of information availability at the point of decision-making, and stakeholders may be overwhelmed by the sheer volume of the provided information. To bridge the information gap, the transfer of individual information points is suggested, freeing up the transfer of information when it is required across the supply chain. Since the information requirements and subsequent actions for each stakeholder have been laid out in this study, the transfer of individual information points is now unlocked. A digital system should be created where the information points set out in are embedded and automatically transferred across stakeholders according to the mechanisms described in and . An example of digitalization supporting effective information transfer is the implementation of collaborative tools and platforms such as BIM and Blockchain to aid coordination (Darabseh & Martins, Citation2020; Hunhevicz et al., Citation2022; Wang & Wegrzyn, Citation2021). The standardization of information-sharing practices can be achieved here through digital product data templates (El Sibaii et al., Citation2023).
The process flow model has highlighted further implications for the digital transformation of the concrete sector and, in particular, of information exchange. Industry stakeholders were keen to highlight informal interactions as a key part of the process. For instance, early discussions to introduce stakeholders and form the design brief, as well as the change management and later contract requirements discussions, will not be easy to digitalize. Digital systems for transforming information flow in the sector should, therefore, be flexible and adaptable to these effects and for different project teams and setups.
In addition to technologies that promote timely information transfer, the targeted application of digital technologies at critical decision points identified in the process flow model is likely to have a significant impact on bridging the information gap. Examples of how specific digital levers, underpinned by process understanding, can help reduce inefficiency in the areas set out in are shown in . In the diagram, each arc represents a relationship between the levers. For example, actioning levers 2 and 4 both facilitate a reduction in quantity specification inefficiency and error. Improving quality control is an important lever to tackle overdesign and waste (). At the point of concrete delivery (), a decision is made whether to accept or reject the delivered mix. This represents a critical turning point for the supply chain as it dramatically impacts progress, significantly delaying the project in terms of time and cost if rejected or if errors and performance deficiencies are found later. Digital tools can support this process via BIM delivery schedules and digital batching sheets, which can cross-check design and mix data to identify discrepancies, aiding the decision to accept or reject the delivered mix (Begić et al., Citation2023; Tommelein & Li, Citation1999). Furthermore, the process flow model identified testing (I12) as a key information point at the point of delivery. Various technologies have been developed to increase the performance insights offered by fresh-state testing via digitization, such as digital slump reconstruction (White & Lees, Citation2023).
Figure 12. Arc diagram representing potential digital levers to tackle causes of over-design and waste.
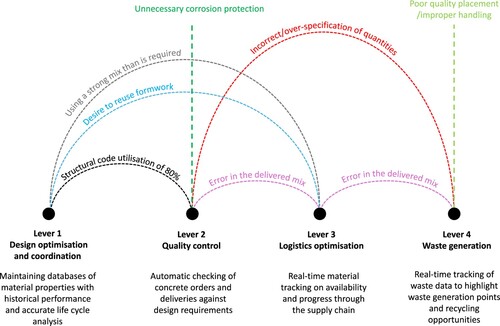
Although this work adopts the United Kingdom market as a case study, the process flow model can be applied to other markets across the world while accounting for the differences in local practices. To accomplish this, the authors recommend conducting a workshop in the country where the process flow model is required. A similar workshop process to the one followed in this study should be undertaken, in which a diverse range of participants representing various stakeholders involved in the practice are invited to review the model and agree on alterations suitable for the country being considered. Since the key stakeholder groups and information points are likely not specific to any country, it is unlikely that the process flow model will require significant changes.
Conclusions and future work
As growth in concrete production continues, there is an increasing focus on promoting efficient practices due to the embodied carbon emissions in the production process. Potential savings of 25% of embodied carbon emissions may be unlocked by addressing over-design and waste. Information is critical to reducing uncertainty and conservativism in decision-making, and as such, there is increasing interest in achieving effective and efficient information flow. The information gap, where stakeholders hold information not shared effectively, leads to uncertainty, delays, and error. The quality and timeliness of shared information should be improved to bridge the information gap. Nonetheless, there is a lack of research to highlight stakeholders’ information requirements and their actions in large-scale concrete activity. Expanding knowledge in this area is crucial, as the key to bridging the information gap is effectively considering which data to transfer and which to disregard. This provides a sound foundation to best enact digital transformation to reduce the climate impact of large-scale concrete activity.
This study has highlighted the specific information requirements for all stakeholders in the concrete sector for the first time. Furthermore, a process flow model was developed to demonstrate the key activities and information transfer. The process flow model highlights that the current document-centric approach contributes to the information gap. Stakeholders are not receiving adequate and timely information according to their requirements as the process is primarily defined by sharing key documents rather than the information required. This finding has significant implications for the application of digitalization in the sector, which has, to date, attempted to transform the current system using digital platforms promoting total information transfer. Instead, targeted digital transformation is encouraged. Such transformation can include the transfer of discrete information points according to the information requirements of each stakeholder. Furthermore, it can include the informal stakeholder interactions prevalent across the sector as well as technologies that can automate and improve insights at decision-making points. While this work has considered the UK concrete sector as a case study, future work is planned to apply the approach and expand the process flow model’s applicability to countries around the world. Ultimately, this work provides a mechanism to bridge the information gap within the large-scale concrete sector.
Supplementary Material.docx
Download MS Word (3.6 MB)Acknowledgements
For the purpose of open access, the authors have applied a Creative Commons Attribution (CC BY) licence to any Author Accepted Manuscript version arising from this submission. The University of Cambridge Department of Engineering Ethics Review Committee granted ethical approval for this study.
Disclosure statement
No potential conflict of interest was reported by the authors.
Additional information
Funding
References
- Badenhorst-Weiss, J. A., Maurer, C., Brevis-Landsberg, T., & Badenhorst-Weiss, H. (2013). Developing measures for the evaluation of information flow efficiency in supply chains. Journal of Transport and Supply Chain Management, 7(1), 13.
- Bastos, T., Teixeira, L. C., Matias, J. C. O., & Nunes, L. J. R. (2023). Agroforestry biomass recovery supply chain management: A more efficient information flow model based on a web platform. Logistics, 7(3), 56. https://doi.org/10.3390/logistics7030056
- Begić, H., Galić, M., & Klanšek, U. (2023). Active BIM system for optimized multi-project ready-mix-concrete delivery. Engineering, Construction and Architectural Management. https://doi.org/10.1108/ECAM-11-2022-1064
- Bishop, D. (1972). Information flow in the construction industry: A study and the development of its recommendations. Aslib Proceedings, 24(2), 79–95. https://doi.org/10.1108/eb050323
- Cave, M., Davis, R., Evan, P., Hoehn, T., & Nicholson, M. (2014). Aggregates, cement and ready-mix concrete market investigation final report. Competition Commission.
- Chen, Q., Adey, B. T., Haas, C. T., & Hall, D. M. (2022). Exploiting digitalization for the coordination of required changes to improve engineer-to-order materials flow management. Construction Innovation, 22(1), 76–100. https://doi.org/10.1108/CI-03-2020-0039
- Chen, Q., García de Soto, B., & Adey, B.T. (2021). Supplier-contractor coordination approach to managing demand fluctuations of ready-mix concrete. Automation in Construction, 121, 103423. https://doi.org/10.1016/j.autcon.2020.103423
- Darabseh, M., & Martins, J. P. (2020). Risks and opportunities for reforming construction with blockchain: Bibliometric study. Civil Engineering Journal, 6(6), 1204–1217. https://doi.org/10.28991/cej-2020-03091541
- Department of the Environment. (1971). Information system for the construction industry: Final report of working party on data co-ordination.
- El Sibaii, M., Rocha Ribeiro, R., Dias, R., Pinto, J. R., Granja, J., & Azenha, M. (2023). Towards standardization of data for structural concrete: Product data templates. In A. Jędrzejewska, F. Kanavaris, M. Azenha, F. Benboudjema, & D. Schlicke (Eds.), International RILEM Conference on Synergising Expertise towards Sustainability and Robustness of Cement-based Materials and Concrete Structures (Vol. 43, pp. 263–275). Springer.
- Ergen, E., & Akinci, B. (2008). Formalization of the flow of component-related information in precast concrete supply chains. Journal of Construction Engineering and Management, 134(2), 112–121. https://doi.org/10.1061/(ASCE)0733-9364(2008)134:2(112)
- Favier, A., De Wolf, C., Scrivener, K., & Habert, G. (2018). A sustainable future for the European cement and concrete industry. ETH Zurich.
- Fergusson, K. J., & Teicholz, P. M. (1996). Achieving industrial facility quality: Integration is key. Journal of Management in Engineering, 12(1), 49–56. https://doi.org/10.1061/(ASCE)0742-597X(1996)12:1(49)
- Forza, C. (2002). Survey research in operations management: A process-based perspective. International Journal of Operations & Production Management, 22(2), 152–194. https://doi.org/10.1108/01443570210414310
- Graham, D., Tommelein, I., & Smith, S. (2005). Cost reduction through vertical integration of the in-situ concrete supply chain. In ARCOM 21st Annual Conference (pp. 1005–1014). Association of Researchers in Construction Management.
- Heher, Y. K., & Chen, Y. (2017). Process mapping: A cornerstone of quality improvement. Cancer Cytopathology, 125(12), 887–890. https://doi.org/10.1002/cncy.21946
- Hossain, M. A., Yeoh, J. K. W., Abbott, E. L. S., & Chua, D. K. H. (2020). Domain-knowledge enriched BIM in Construction 4.0: Design-for-safety and crane safety cases. In A. Sawhney, M. Riley, & J. Irizarry (Eds.), Construction 4.0 (pp. 326–349). Routledge.
- Hunhevicz, J. J., Motie, M., & Hall, D. M. (2022). Digital building twins and blockchain for performance-based (smart) contracts. Automation in Construction, 133, 103981. https://doi.org/10.1016/j.autcon.2021.103981
- Joseph, N., & Chandran, R. (2023). Information flow in reuse prospects of construction materials analysis of information flow in the Swedish construction industry [Masters]. Uppsala Universitet, Uppsala.
- Kaipia, R. (2009). Coordinating material and information flows with supply chain planning. The International Journal of Logistics Management, 20(1), 144–162. https://doi.org/10.1108/09574090910954882
- Kazaz, A., Ulubeyli, S., Er, B., Arslan, V., Arslan, A., and Atici, M., 2015. Fresh ready-mixed concrete waste in construction projects: A planning approach. Procedia Engineering, 123, 268–275. https://doi.org/10.1016/j.proeng.2015.10.088
- Köller, J., Belaud, J. P., Jarke, M., Kuckelberg, A., & Teague, T. (2002). Chapter 4.1 - Methods & tools for software architecture. In B. Braunschweig & R. Gani (Eds.), Computer aided chemical engineering (pp. 229–266). Elsevier.
- Lawrenz, S., Nippraschk, M., Wallat, P., Rausch, A., Goldmann, D., & Lohrengel, A. (2021). Is it all about information? The role of the information gap between stakeholders in the context of the circular economy. Procedia CIRP, 98, 364–369. https://doi.org/10.1016/j.procir.2021.01.118
- Maghrebi, M., Sammut, C., & Waller, T. S. (2014). Predicting the duration of concrete operations via artificial neural network and by focusing on supply chain parameters. Building Research Journal, 61(1), 1–14. https://doi.org/10.2478/brj-2014-0001
- Man-Shi, L., & Ochsendorf, J. A. (2005). Material flow analysis of concrete in the United States [Master of Science]. Massachusetts Institute of Technology, Boston.
- Miller, S.A., 2021. The role of data variability and uncertainty in the probability of mitigating environmental impacts from cement and concrete. Environmental Research Letters, 16(5), 054053. https://doi.org/10.1088/1748-9326/abe677
- Miller, S. A., John, V. M., Pacca, S. A., & Horvath, A. (2018). Carbon dioxide reduction potential in the global cement industry by 2050. Cement and Concrete Research, 114, 115–124. https://doi.org/10.1016/j.cemconres.2017.08.026
- NHS Modernisation Agency. (2002). Process mapping, analysis and redesign.
- Noche, B., & Elhasia, T. (2013). Approach to innovative supply chain strategies in cement industry; Analysis and model simulation. Procedia - Social and Behavioral Sciences, 75, 359–369. https://doi.org/10.1016/j.sbspro.2013.04.041
- Ørngreen, R., & Levinsen, K. T. (2017). Workshops as a research methodology. The Electronic Journal of e-Learning, 15, 70–81.
- Park, M., Kim, W. Y., Lee, H. S., & Han, S. (2011). Supply chain management model for ready mixed concrete. Automation in Construction, 20(1), 44–55. https://doi.org/10.1016/j.autcon.2010.07.005
- Phan, T.-D., & Athigakunagorn, N. (2022). Discrete-event simulation-based decision making of Just-In-Time strategies for precast concrete supply chain using batch delivery and offsite inventory level. Uncertain Supply Chain Management, 10(3), 679–692. https://doi.org/10.5267/j.uscm.2022.5.007
- Royal Institute of British Architects, 2021. RIBA plan of work.
- Sawhney, A., Riley, M., & Irizarry, J. (2020). Construction 4.0: Introduction and overview. In A. Sawhney, M. Riley, & J. Irizarry (Eds.), Construction 4.0 (pp. 3–22). Routledge.
- Scrivener, K. L., John, V. M., & Gartner, E. M. (2018). Eco-efficient cements: Potential economically viable solutions for a low-CO2 cement-based materials industry. Cement and Concrete Research, 114, 2–26. https://doi.org/10.1016/j.cemconres.2018.03.015
- Shanks, W., Dunant, C. F., Drewniok, M. P., Lupton, R. C., Serrenho, A., & Allwood, J. M. (2019). How much cement can we do without? Lessons from cement material flows in the UK. Resources, Conservation and Recycling, 141, 441–454. https://doi.org/10.1016/j.resconrec.2018.11.002
- Sholeh, M. N., Fauziyah, S., Dharmo, B., Widodo, P., & Christiawan, I. (2018). The development of construction supply chain model on ready mix concrete with value stream mapping method. Advanced Science Letters, 24(12), 9695–9697. https://doi.org/10.1166/asl.2018.13116
- Stowe, K., Lépinoy, O., & Khanzode, A. (2020). Innovation in the construction project delivery networks in Construction 4.0. In A. Sawhney, M. Riley, & J. Irizarry (Eds.), Construction 4.0 (pp. 62–88). Routledge.
- Syverson, C. (2008). Markets: Ready-mixed concrete. Journal of Economic Perspectives, 22(1), 217–234. https://doi.org/10.1257/jep.22.1.217
- Titus, S., & Bröchner, J. (2005). Managing information flow in construction supply chains. Construction Innovation, 5(2), 71–82. https://doi.org/10.1108/14714170510815186
- Tommelein, I., & Li, A. (1999, July 26–28). Just-In-Time concrete delivery: Mapping alternatives for vertical supply chain integration. Conference of the International Group for Lean Construction, Berkeley.
- Vaidyanathan, K., Varghese, K., & Devkar, G. (2020). Cloud-based collaboration and project management. In A. Sawhney, M. Riley, & J. Irizarry (Eds.), Construction 4.0 (pp. 370–394). Routledge.
- Wang, S., Tang, J., Zou, Y., & Zhou, Q. (2020). Research on production process optimization of precast concrete component factory based on value stream mapping. Engineering, Construction and Architectural Management, 27(4), 850–871. https://doi.org/10.1108/ECAM-10-2018-0455
- Wang, E., & Wegrzyn, K. (2021). The pros and cons of blockchain in supply chain. JD Supra. Retrieved September 30, 2022, from https://www.jdsupra.com/legalnews/the-pros-and-cons-of-blockchain-in-8158255/
- Watari, T., Cao, Z., Serrenho, A. C., & Cullen, J. (2023). Growing role of concrete in sand and climate crises. iScience, 26(5), 106782. https://doi.org/10.1016/j.isci.2023.106782
- White, C., & Lees, J. M. (2023). Yield stress prediction from 3D reconstruction of fresh concrete slump. Cement and Concrete Research, 174, 107331. https://doi.org/10.1016/j.cemconres.2023.107331
- Zhang, Z., Nielsen, M. K., Hørsholt, S., Muralidharan, G., & Jørgensen, J. B. (2021). Digitalization, control and optimization for cement plants. Computer Aided Chemical Engineering, 50, 1319–1324. doi:10.1016/B978-0-323-88506-5.50203-5