Abstract
Purpose of the article
Learning to use an exoskeleton is time consuming and diverse between users. Knowledge about trainability of exoskeleton skills is relevant for planning and expectation management. The objective was to assess predictors of exoskeleton skill performance during and after exoskeleton training.
Materials and methods
Twenty-four participants with a motor complete spinal cord injury were given 24 training sessions in 8 weeks. Nine potential predictors were identified: lesion level, age, gender, age at injury, time since injury, BMI, sport, active lifestyle, and anxiety. Univariate and multivariate linear regression analyses were performed to examine predictors of skill performance after 2, 4, 6, and 8 weeks.
Results and conclusions
Twenty participants completed the training. Univariate analysis revealed that positive predictors were: low lesion level and more active lifestyle after 2 weeks, whereas low age at injury, low BMI, and more active lifestyle were positive predictors after 6 weeks. Multivariate regression model explained 65% of the performance after 2 weeks (predictors: lesion level, anxiety, active lifestyle) and 66% after 6 weeks (predictors: BMI, active lifestyle, age). Lesion level was a predictor during the first 4 weeks, but did not influence participants' final skill level. BMI, age, and active lifestyle were predictors toward the end of the training period.
With the help of wearable exoskeletons people with a complete spinal cord injury can regain their standing and walking mobility.
Learning to use an exoskeleton is time consuming and the number of training sessions required to walk independently differs greatly between users.
This study shows that lesion level was an important predictor of exoskeleton motor learning in the first 4 weeks of training.
BMI, age, and active lifestyle were predictors of exoskeleton skill performance toward the end of the 8 week training period.
Implications for rehabilitation
Introduction
The use of robotics for rehabilitation is a relatively new field, that received a lot of attention in the last decade [Citation1–3]. In the ‘00, commercial available rehabilitation gait robotics, such as the Lokomat, were introduced in clinical setting. The Lokomat is mainly being used for restoration of gait in patients with spinal cord injury (SCI) [Citation4] and stroke [Citation5] and is always used in combination with a treadmill and body-weight support system. Although to a lesser extent, the Lokomat has also been used to prevent secondary health complications in SCI, such as pain and spasticity [Citation6]. A disadvantage of such a gait robot is that it is mainly used in a clinical setting. In the last decade, wearable gait robots the so-called exoskeletons have been introduced as an alternative.
Wearable exoskeletons can enable people with motor complete SCI to support their standing and walking ability outside the clinical setting. Due to the possibility to train in an upright position, exoskeletons may have a beneficial effect on preventing secondary health complications [Citation7,Citation8]. Previous studies have shown that the use of wearable exoskeletons in people with SCI is safe and feasible [Citation8,Citation9], but an intensive training is required before users can safely and independent use an exoskeleton [Citation10–13] outside the clinical setting. Although the majority of subjects with a complete SCI were able to walk independently with an exoskeleton, the number of training session required to reach this level of independent walking differed between participants ranging between 6 and 23 training sessions [Citation10].
The diversity between users raised the question: why do some users acquire more exoskeleton skills than others? Knowledge about trainability of exoskeleton skills during and after a training period is relevant for rehabilitation planning and expectation management. In learning to use other assistive devices, such as a manual wheelchair, relationships between personal and injury characteristics and wheelchair skill performance have been found [Citation14]. For wheelchair skill training, it was found that a lower lesion level, lower age, male gender, low body mass index (BMI) and more time spent with endurance exercise were relevant predictors for wheelchair skill performance [Citation14,Citation15]. The same predictors may also be important for learning to perform exoskeleton skills. From sport psychology studies, it is known that motor performance is also influence by anxiety [Citation16,Citation17]. An increased level of anxiety overloads the attentional capacity, resulting in a decreased motor performance [Citation17]. Finally, we expect that a shorter time since injury and a lower age at injury onset could influence exoskeleton skills learning.
The objective of this study was to evaluate the potential predictors for exoskeleton skill performance during and after an intensive training period in participants with motor complete SCI. The following personal and injury characteristics are hypothesized as potentially positive predictors for exoskeleton skill performance: low lesion level, low age, low BMI, male gender, large physical activity level, low anxiety level, short time since injury onset and a low age at injury onset.
Materials and methods
Participants
Patients with motor complete SCI who were interested in testing an exoskeleton contacted the Sint Maartenskliniek to participate in the study. Patients were first screened by telephone and second scheduled for a complete screening with the rehabilitation physician. Patients were eligible to enter the study if they were 18 years or older, at least 6 months since injury, and were classified as A or B on the American Spinal Injury Association (ASIA) impairment scale between Thoracic 1 (Th1) and Lumbar 1 (L1). Participants had to weigh less than 100 kg and have a height between 1.60 and 1.90 m for fitting in the ReWalk exoskeleton device. Exclusion criteria were: severe spasticity (Modified Ashworth Scale > 3); restricted range of motion in the hip, knee, or ankle joint; unable to control crutches; unable to make a transfer from a chair to a wheelchair without the use of external support; osteoporosis; fractures of the lower extremities in the last 2 years; pregnant or lactating; balance disorders; neurogenic heterotopic ossification; history of other conditions that could interfere with the motor learning process (e.g., stroke). All participants gave written informed consent in accordance with the Declaration of Helsinki. The study was approved by the regional medical ethics committee of Arnhem-Nijmegen (2016-2418) and by the internal review board of the Sint Maartenskliniek.
Device and training
The exoskeleton training sessions and measurements were performed in the sports hall at the Sint Maartenskliniek Rehabilitation Centre in Nijmegen. Participants were given 24 training sessions of 1.5-h over an 8 week period with a ReWalk exoskeleton. Two wearable exoskeletons were used in the study; the ReWalk™ Rehabilitation System and the ReWalk™ Personal 6.0. All participants started with the ReWalk™ Rehabilitation System and the first training session was used to adjust the exoskeleton to the patient’s body composition. Per training session at least two physical therapist guided the training and a maximum of four physical therapists were involved in the training sessions. All four physical therapists were certified ReWalk trainers. Each training was individualized, as the physical therapists chose the specific goal based on the current level of the patient. The goal and practiced skills were notated by the physical therapist after each training session.
Measures
Performance of exoskeleton skills
The exoskeleton skills were measured with the Intermediate-skills-test and the Final-skills-test. The development and assessment of the skills-tests have been described earlier, and the reliability and ability to discriminate across participants’ skill level was found to be good [Citation18]. These skills-tests consisted of standardized tests in which the capacity to perform basic and advanced exoskeleton skills with varying levels of assistance were measured. Participants' skill-level was tested every 2 weeks (total of four times), the first three times with the Intermediate-skills-test and the last time with the Final-skills-test. The Intermediate-skills-test consists of 27 skills measured separately of each other in an ascending order of difficulty, until two skills were not achieved. The order of difficulty was from standing skills (e.g., weight shifting and sit-to-stand) to walking skills (e.g., walk 10 m with and without assistance and arrest gait) to advanced skills (e.g., walk curves, passing a sloping doorstep and walk up and over a martial arts mat). Participants who could walk without assistance of the exoskeleton trainer between the 18th and 23th training session, performed the Final-skills-test in the last (24th) training session. The Final-skills-test consists of 20 skills measured in a fixed sequence and was performed twice with a 5-min break in between. The Final-skill-test aimed to resemble daily life situations in which skills are often performed in sequence (e.g., make a curve after passing a doorstep). During all tests, the physical therapist walked behind the participant to assure safety, but did not intervene unless necessary to prevent a fall.
Personal and injury characteristics
Nine potential predictors for exoskeleton skills performance were selected; neurological lesion level, age, gender, age at injury onset, time since injury, physical activity level (sport and active lifestyle), level of anxiety and BMI. Neurological lesion level was assessed by the rehabilitation physician and registered in the patient registration system prior to enrolment. Before the start of the exoskeleton training participants filled out their age, gender, age at injury onset, and time since injury on a form. In addition, participants filled out a questionnaire regarding their physical activity level and level of anxiety. Physical activity level was assessed through two questions: (1) Are you currently engaged in a sport? And if so, how many hours do you on average spend on it per week? (physical activity level: sport). (2) In addition to your sport, how many times a week do you usually perform a moderate to intensive physical activity that increases your heart rate or speeds up your breathing (e.g., leisurely hand biking) for at least 30 min? (physical activity level: active lifestyle). The former question addresses the Dutch physical activity guidelines, which is the minimum amount of physical activity required for a healthy and active lifestyle [Citation19]. Level of anxiety was calculated from the seven-item anxiety subscale of the Dutch version of the Hospital Anxiety and Depression Scale (HADS) [Citation20]. A higher anxiety sum score (range 0–21) represents more anxiety. During the first exoskeleton session participants height and weight were registered for adjustment of the exoskeleton. BMI was calculated as weight divided by the square of the height of the participant.
Data and statistical analysis
Only participants who performed all three measurement (Intermediate-skills-test 1, 2, and 3) were included in the analysis of the performance of the Intermediate-skills-tests. For the analysis of the Final-skills-test, only participants who met the criteria to perform the Final-skills-test were included. The outcome of the performance of the Final-skills-test was the average of the achieved final skills in both rounds.
Descriptive statistics were used to present the personal, injury characteristics and the number of achieved skills at the three Intermediate-skills-tests and the Final-skills-test. Univariate and multivariate linear regression analyses were performed to examine the personal and injury characteristics that can predict exoskeleton skill performance. The dependent variable was exoskeleton skill performance defined as the achieved basic and advanced skills at Intermediate-skills-test 1, 2, 3 and the Final-skills-test. The independent variables were the personal and injury characteristics (neurological lesion level, age at injury onset, time since injury onset, age, BMI, gender, sport, active lifestyle, and anxiety). All independent variables were univariately tested for their relationship with dependent variable (the achieved exoskeleton skills at the Intermediate-skills-tests and Final-skills-test). Independent variables were subsequently included in the multivariate analysis. A stepwise regression analysis was used to assess the predictors for the performance at Intermediate-skills-test 1, 2, 3, and the Final-skills-test. Variables with a p value of F (probability of F) ≤.05 were entered in the stepwise regression analysis. Variables that were already in the equation and added less than 0.10 (probability of F > 0.10) were removed from the regression equation. A Shapiro–Wilk test was performed to check if the residuals were normally distributed.
Results
Participants
Twenty-four participants were enrolled in the study and 20 (83%) completed the training program and performed all three Intermediate-skills-tests. At the end of the training program, 15 (75%) participants were able to walk without assistance of the physical therapist and therefore performed the Final-skills-test during the 24th training session. From one participant, the data of the active lifestyle question were missing. This missing value was replaced with the mean of the active lifestyle questions of the other 19 participants in the stepwise multiple linear regression analysis. An overview of the personal and injury characteristics is given in .
Table 1. Personal and injury characteristics.
Intermediate-skills-test
The univariate linear regression between the independent variables and the achieved intermediate skills at Intermediate-skills-test 1, 2, and 3 is presented in . Various personal and injury characteristics were significantly correlated to exoskeleton skill performance at the Intermediate-skills-tests. A lower lesion level (R2 = 0.35, p = 0.006) and a larger active lifestyle (R2 = 0.22, p = 0.041) were associated with a higher exoskeleton skill performance at Intermediate-skills-test 1 (see ). For Intermediate-skills-test 2, only the neurological level showed a significant correlation (R2 = 0.30, p = 0.012). For Intermediate skills-test 3, a higher exoskeleton skill performance was associated with a lower age at injury onset (R2 = 0.26, p = 0.023), lower age at enrolment (R2 = 0.26, p = 0.021), lower BMI (R2 = 0.26, p = 0.021), and a more active lifestyle (R2 = 0.22, p = 0.041).
Figure 1. Explained variance (Pearson’s correlation R2) at Intermediate-skills-test 1, 2, and 3 for the 9 predictors. An R2 above .20 is significant (p < 0.05), N = 20.
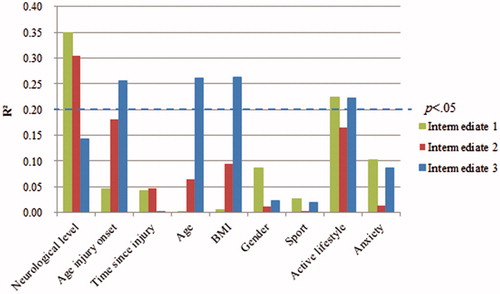
Figure 2. Scatterplot for the predictors with a significant correlation with exoskeleton skill performance (p < 0.05) at Intermediate-skills-test 1, 2, and 3.
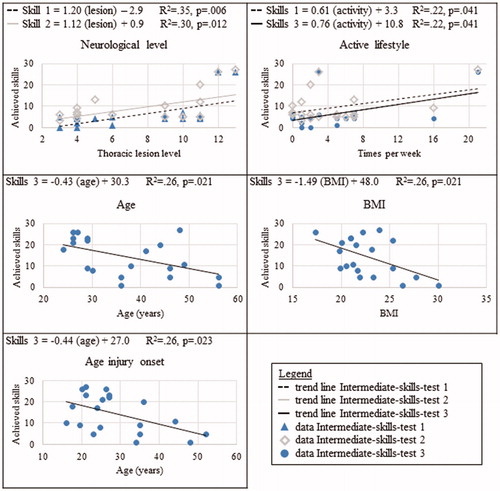
Lesion level, anxiety, and active lifestyle were included in the stepwise multiple linear regression analysis for the performance at Intermediate-skills-test 1. The model had an explained variance (R2) of 0.65 (F(3,16) = 9.99, p = 0.001). Lesion level (β = 0.59, p = 0.002), anxiety (β = 0.45, p = 0.009), and active lifestyle (β = 0.34, p = 0.040) significantly predicted performance at Intermediate-skills-test 1. For Intermediate-skills-test 2, no additional variable to lesion level (β = 0.55, p = 0.012) was added in the stepwise regression analysis. The predictors of the performance at Intermediate-skills-test 3 were BMI (β = –0.36, p = 0.031), active lifestyle (β = 0.51, p = 0.003), and age (β = –47, p = 0.008). A significant regression equation was found (F(3,16) = 10.23, p = 0.001), with an explained variance (R2) of 0.66. Shapiro–Wilk’s test revealed that the distribution of the residuals from the regression analysis were normally distributed at all three Intermediate-skills-test (p = 0.224, p = 0.156, and p = 0.539). An overview of the outcome of the stepwise regression is presented in .
Table 2. Multiple forward stepwise regression.
Final-skills-test
The univariate relationship between the independent variables and the achieved final skills is presented in . None of the independent variables were significantly related with the achieved final skills. In addition, no variables were entered in the stepwise regression analysis for performance at the Final-skills-test.
Discussion
The aim of the present study was to assess which personal and injury characteristics could predict exoskeleton skill performance during and after an 8 week intensive training program. After 2 weeks of training, lesion level, anxiety, and active lifestyle explained 65% of the skill performance. BMI, active lifestyle, and age explained 66% of skill performance after 6 weeks of training. Active lifestyle appeared to be the most predictive variable for the exoskeleton skill level at the end of the 8 week training program.
Exoskeleton skill performance was measured at four moments during the training program. As a result, different predictive variables were identified at these moments. Moreover, some variables explained increasingly less during the training program, whereas other variables explained increasingly more indicating a change in the importance of personal and injury characteristics over the training period. The injury characteristic lesion level was the only variable that explained increasingly less during the training program (see ). In the first 4 weeks, lesion level was the most important predictor (highest standardized beta coefficient at Intermediate-skills-test 1 (β = 0.59) and Intermediate-skills-test 2 (β = 0.55)) (see ). The relation between lesion level and functional outcomes has been previously described [Citation21,Citation22] and is often explained by the (im)possibility to (voluntary) control the trunk muscles involved in sitting and standing position (e.g., active core stability). Remarkably, lesion level was not a significant predictor of exoskeleton skill performance after 6 and 8 weeks, both in the univariate and in the multivariate analysis. Important to note with respect to core stability, is that seven out of 10 participants with an impaired active core stability (lesion level above Th6) used a (plaster) corset during the exoskeleton use. These results suggest that an active core stability is beneficial for learning to perform exoskeleton skills in the first period of exoskeleton training, but has minimal influence on the skill level toward the end of the training period.
In contrast to lesion level, the more personal characteristics age, age at injury onset and BMI showed an increase in prediction of the exoskeleton skill performance over the training period. These variables had a significant explained variance after 6 weeks of training, a very low explained variance at 2 weeks and in between explained variance at 4 weeks (see ). The importance of age and BMI was also supported by their inclusion in the multivariate regression model after 6 weeks of training. The most important predictors of exoskeleton skill performance at 6 weeks in the multivariate analysis were active lifestyle (β = 0.51), age (β = –0.47), and to a lesser extent BMI (β = –0.36) (see ). These variables were in accordance with learning to perform skills with other assistive technologies, such as manual wheelchairs. Age and BMI were found to be the best predictors of wheelchair skill performance after inpatient rehabilitation [Citation14] and physical capacity was highly correlated to wheelchair skill performance at the end of inpatient rehabilitation [Citation23]. This supports the hypotheses that similar predictors are involved in learning to perform wheelchair skills and exoskeleton skills.
At the end of the training program, only participants who could walk independently performed the Final-skills-test and were included in the analysis. The Final-skills-test is part of a bigger study and was designed to assess whether participants were skilled enough to use the exoskeleton safe in their home environment. Therefore, only a subgroup of more skilled participants (e.g., who could walk independent) performed the Final-skills-test. As a consequence, a smaller and homogenous group with respect to injury and personal characteristics were used in the multivariate analysis. Furthermore, the distribution of the mean achieved final skills was small, ranging between 13.5 and 19.5 final skills of which half of the participants achieved at least 19 out of 20 final skills. Therefore, the explained variance at the Final-skills-test by the injury and personal characteristics was low.
Active lifestyle was the only significant or nearly significant predictor of exoskeleton skills in all three Intermediate-skills-tests and the Final-skills-test. This variable should be addressed carefully, because one participant had an extremely active lifestyle (score of 21 times per week) and was already very skilled after 2 weeks of training (achieved all 27 intermediate skills). This participant can be seen as outlier with a high influence in the regression analysis. One additional participants achieved 26 out of 27 intermediate skills after 2 weeks of training. Both highly skilled participants had used a Reciprocating Gait Orthosis (RGO, such as the ARGO walker) and indicated that they experienced benefits from this. There are similarities between RGO and exoskeleton use, such as balance in stance, anterior–posterior weight shifting and sometimes the use of crutches. Therefore, former experience with an RGO is likely an important predictor, but this was not registered in this study.
Two crucial steps can be identified in the learning process of independent exoskeleton use. First, weight shifting in stance and standing up are important exoskeleton skills that can be seen as prerequisites for walking. Independent weight shifting and sit-to-stand were achieved by 85% and 60% of the participants after 2 weeks of training, and 95% and 90% after 4 weeks of training. The second important step in the learning process is independent walking. When independent walking was achieved, most participants were able to achieve several advanced exoskeleton skills within two weeks. Independent walking was achieved by 15 participants at the end of training program. Compared to other studies with the same number of total training sessions (14% (one out of 7) [Citation12] or 57% (four out of seven) [Citation13]), we found a higher percentage of 75% people who were able to walk independently with the exoskeleton. Despite this higher percentage, still five participants were unable to walk independently after 24 sessions. Due to the fixed training period in this study, we do not know how many additional training sessions these participants would have needed to achieve the skill independent walking. In addition to independent walking, donning the exoskeleton is an important skill for independent use. Independent donning was possible by 40% of the participants after 2 weeks of training, and 55% after 4 and 6 weeks of training. During the Final-skills-test, 87% of the participants were able to don the exoskeleton independently. The participants who did not achieve the donning skill required assistance with putting on the shoes. The remaining part of donning the exoskeleton, such as fixating the straps around the thigh and trunk, could be done independently by all 20 participants.
With 24 participants enrolled, this is the exoskeleton study with the largest sample size investigating the independent use of an exoskeleton. Despite that, the sample size of 24 is small for multivariate regression analysis limiting the number of predictors that could be tested. Other characteristics may also have been of interest to predict exoskeleton skill performance, such as previous experience with other technologies, balance capacity and general motor competence. While walking with a (ReWalk) exoskeleton, the user has to shift the body weight continuously to allow for a good swing foot clearance challenging the users balance capacity. Although there are suitable measures to assess balance capacity (e.g., ability to control someone’s center of mass while seated or during stance [Citation24]), outcome measures to assess general motor competence in people with SCI are lacking. Lesion level can be seen as an exploratory measure of participants functioning, but might not be sufficient to describe the participants general motor competence. As the important predictors for wheelchair and exoskeleton skills performance show similarities, a wheelchair skills test may be a good alternative for measuring general motor competence in chronic SCI patients. Also measures related to motor learning capacity, such as the variability in performance of motor tasks, might be related to the learning rate of new motor skills [Citation25,Citation26]. Future research should investigate the influence of other potential predictors of exoskeleton skills performance, such as experience with other technologies, balance capacity, general motor competence, and motor learning capacity.
Conclusions
Knowledge about predictors of exoskeleton skill performance is relevant for potential user and can be used by the rehabilitation specialist during patient counseling. Lesion level appeared to be an important predictor during the first 4 weeks of training, but did not influence participants’ final skill level. BMI, age, and active lifestyle were predictors of exoskeleton skill performance toward the end of the training period.
Disclosure statement
The authors declare that the research was conducted in the absence of any commercial or financial relationships that could be construed as a potential conflict of interest.
Additional information
Funding
References
- Díaz I, Gil JJ, Sánchez E. Lower-limb robotic rehabilitation: literature review and challenges. J Robot. 2011;2011:1–11.
- Chen G, Chan CK, Guo Z, et al. A review of lower extremity assistive robotic exoskeletons in rehabilitation therapy. Crit Rev Biomed Eng. 2013;41(4–5):343–363.
- Calabrò RS, Cacciola A, Bertè F, et al. Robotic gait rehabilitation and substitution devices in neurological disorders: where are we now? Neurol Sci. 2016;37(4):503–514.
- Nam KY, Kim HJ, Kwon BS, et al. Robot-assisted gait training (Lokomat) improves walking function and activity in people with spinal cord injury: a systematic review. J Neuroeng Rehab. 2017;14:24.
- Bruni MF, Melegari C, De Cola MC, et al. What does best evidence tell us about robotic gait rehabilitation in stroke patients: a systematic review and meta-analysis. J Clin Neurosci. 2018;48:11–17.
- Alcobendas-Maestro M, Esclarín-Ruz A, Casado-López RM, et al. Lokomat robotic-assisted versus overground training within 3 to 6 months of incomplete spinal cord lesion: randomized controlled trial. Neurorehabil Neural Repair. 2012;26(9):1058–1063.
- Baunsgaard C, Nissen U, Brust A, et al. Exoskeleton gait training after spinal cord injury: an exploratory study on secondary health conditions. J Rehabil Med. 2018;50(9):806–813.
- Miller LE, Zimmermann AK, Herbert WG. Clinical effectiveness and safety of powered exoskeleton-assisted walking in patients with spinal cord injury: systematic review with meta-analysis. Med Dev. 2016;9:455–466.
- Bach Baunsgaard C, Vig Nissen U, Katrin Brust A, et al. Gait training after spinal cord injury: safety, feasibility and gait function following 8 weeks of training with the exoskeletons from Ekso Bionics article. Spinal Cord. 2018;56:106–116.
- van Dijsseldonk RB, Rijken H, van Nes IJW, et al. A framework for measuring the progress in exoskeleton skills in people with complete spinal cord injury. Front Neurosci. 2017;11:1–12.
- Kozlowski A, Bryce T, Dijkers M. Time and effort required by persons with spinal cord injury to learn to use a powered exoskeleton for assisted walking. Top Spinal Cord Inj Rehabil. 2015;21(2):110–121.
- Platz T, Gillner A, Borgwaldt N, et al. Device-training for individuals with thoracic and lumbar spinal cord injury using a powered exoskeleton for technically assisted mobility: achievements and user satisfaction. Biomed Res Int. 2016;2016:1–10.
- Spungen AM, Asseslin PK, Fineberg DB, et al. Exoskeletal-assisted walking for persons with motor-complete paraplegia. NATO Sci Technol Org. 2013;6(1):6–14.
- Kilkens OJ, Dallmeijer AJ, Angenot E, et al. Subject- and injury-related factors influencing the course of manual wheelchair skill performance during initial inpatient rehabilitation of persons with spinal cord injury. Arch Phys Med Rehabil. 2005;86(11):2119–2125.
- Teeter L, Gassaway J, Taylor S, et al. Relationship of physical therapy inpatient rehabilitation interventions and patient characteristics to outcomes following spinal cord injury: the SCIRehab project. J Spinal Cord Med. 2012;35(6):503–526.
- Hardy L, Mullen R, Martin N. Effect of task-relevant cues and state anxiety on motor performance. Percept Mot Skills. 2001;92(3):943.
- Mullen R, Hardy L, Tattersall A. The effects of anxiety on motor performance: a test of the conscious processing hypothesis. J Sport Exerc Psychol. 2005;27(2):212–225.
- van Dijsseldonk RB, van Nes IJW, Rijken H, et al. RehabMove 2018: a framework of exoskeleton-skills-tests in patients with complete spinal cord injury. 6th International RehabMove State-Of-The-Art Congress 2018, 12–14 December, Groningen, The Netherlands. 2019. p. 6–8.
- Weggemans RM, Backx FJG, Borghouts L, et al. The 2017 Dutch physical activity guidelines. Int J Behav Nutr Phys Act. 2018;15(1):58–69.
- Spinhoven PH, Ormel J, Sloekers PPA, et al. A validation study of the Hospital Anxiety and Depression Scale (HADS) in different groups of Dutch subjects. Psychol Med. 1997;27(2):363–370.
- Schönherr MC, Groothoff JW, Mulder GA, et al. Functional outcome of patients with spinal cord injury: rehabilitation outcome study. Clin Rehabil. 1999;13(6):457–463.
- Dahlberg A, Kotila M, Kautiainen H, et al. Functional independence in persons with spinal cord injury in Helsinki. J Rehabil Med. 2003;35(5):217–220.
- Kilkens OJ, Dallmeijer AJ, Nene AV, et al. The longitudinal relation between physical capacity and wheelchair skill performance during inpatient rehabilitation of people with spinal cord injury. Arch Phys Med Rehabil. 2005;86(8):1575–1581.
- Altmann VC, Groen BE, Groenen KH, et al. Construct validity of the trunk impairment classification system in relation to objective measures of trunk impairment. Arch Phys Med Rehabil. 2016;97(3):437–444.
- Dhawale AK, Smith MA, Ölveczky BP. Adaptive process in motor learning: the role of variability. Annu Rev Neurosci. 2017;40(1):479–498.
- Wu HG, Miyamoto YR, Castro LNG, et al. Temporal structure of motor variability is dynamically regulated and predicts motor learning ability. Nat Neurosci. 2014;17(2):312–321.