Abstract
Purpose
This systematic review and meta-analysis aimed to evaluate the effectiveness of inspiratory muscle training (IMT) on respiratory muscle strength, lung function and quality of life (QOL) in adults with spinal cord injuries (SCI).
Methods
Databases were searched up to June 2022; CENTRAL, CINAHL, MEDLINE, PEDRo, and PubMed. Following PRISMA reporting guidelines, two independent reviewers selected studies and extracted data. Study quality and levels of evidence were assessed.
Results
Following selection from 624 initial search results, six randomised controlled trials were identified, comprising 124 participants. Quality of Evidence was very low to moderate. Meta-analysis showed that post intervention, IMT significantly improved maximal inspiratory pressure (MD 15.72 cmH2O, 95% CI 5.02, 26.41, p = 0.004) when compared with a control intervention. There was no significant benefit for physical QOL (SMD 0.12, 95% CI −1.01, 1.25, p = 0.84), mental QOL (SMD −0.2, 95% CI −1.72, 1.33, p = 0.80), maximal expiratory pressure (MD 5.19 cmH2O, 95% CI −4.16, 14.55, p = 0.80), or FEV1 (MD 0.26 L, 95% CI −0.19, 0.7, p = 0.26). Sensitivity analyses found larger effects for studies with 8 week interventions (MD 17.5 cmH2O (95% CI 3.36 to 31.66)) and spring loaded devices alone (MD 21.18 cmH2O, 95% CI 9.65 to 32.72).
Conclusion
Moderate quality evidence suggests IMT improves respiratory strength in adults with an SCI. The mental and physical QOL outcomes provided very low quality of evidence, with considerable heterogeneity between study results, leading to inconsistency. Further research is warranted to investigate medium and long-term impact of robust IMT protocols, accounting for patient motivation and adherence to IMT.
Inspiratory muscle training (IMT) significantly improves respiratory muscle strength in adults with spinal cord injuries—irrespective of time since injury, or degree of injury completeness.
IMT is a feasible, safe, and worthwhile intervention to implement with adults with spinal cord injuries and can be utilized in a variety of settings.
IMT interventions that are 8 weeks long and utilize a spring-loaded inspiratory threshold device may generate the largest improvements in respiratory strength.
IMPLICATIONS FOR REHABILITATION
Introduction
A traumatic spinal cord injury (SCI) refers to damage to the spinal cord, resulting in a lesion that causes impairments and/or paralysis to sensory, motor and autonomic functions below the level of injury [Citation1]. Respiratory complications remain the most common cause of mortality following SCI, as respiratory function and airway clearance is impaired to varying degrees depending on the level and completeness of the injury [Citation2,Citation3,p.668]. This dysfunction has the potential to greatly affect an individual’s physical and psychological well-being [Citation1,Citation4].
Research suggests that strengthening the respiratory muscles may improve pulmonary function during initial recovery post SCI, and lower the risk of excessive decline in lung function and cough capacity during the chronic phase [Citation5,Citation6]. Inspiratory muscle training (IMT) is strength training that specifically targets the respiratory muscles, to improve inspiratory muscle strength—and subsequently—respiratory function [Citation7]. Methods such as abdominal weights [Citation7], incentive spirometers [Citation8], inspiratory resistance devices [Citation5,Citation9–14], and normocapnic hyperpnea training [Citation15] are examples of ways researchers have attempted to strengthen the inspiratory muscles. Although these methods are diverse, similar outcome measures are used to monitor changes in respiratory function and lung volumes. The most commonly reported outcome measures are lung volumes and flows, such as maximal inspiratory pressure, maximal expiratory pressure, forced expiratory volume in 1 s, peak expiratory flow rate, maximal voluntary ventilation, and functional outcomes such as quality of life, dyspnoea, and rate of perceived exertion [Citation9,Citation10]. Maximal inspiratory pressure is the most widely used measure of respiratory muscle strength [Citation9], and may be a pre-cursor for the development of pneumonia in individuals with levels below lesion-specific reference values [Citation16]. Considering respiratory dysfunction accounts for 28% of deaths within the first year after an SCI [Citation17], utilizing IMT may lower an individual’s risk of mortality—potentially impacting quality of life.
To date, various methodological limitations, small sample sizes and heterogeneity in participant characteristics and outcome measures have prevented various systematic reviews [Citation4,Citation9,Citation10,Citation15,Citation18,Citation19] from determining an overall effect of IMT on respiratory strength and quality of life. A Cochrane review by Berlowitz and Tamplin [Citation9] synthesized nine studies performing respiratory muscle training interventions. Meta-analyses for maximal inspiratory pressure (n = 147) and maximal expiratory pressure (n = 151) found a statistically significant difference in favour of the intervention over control groups, however meta-analyses for forced expiratory volume in 1 s (n = 97) determined no significant difference between groups. Berlowitz and Tamplin [Citation9] state these findings were conservative as only five randomised control trials were included in the analysis. This bias contributed to their certainty in the evidence—as maximal inspiratory pressure, maximal expiratory pressure, forced expiratory volume in 1 s and quality of life were given a Grading of Recommendations, Assessment, Development and Evaluation (GRADE) [Citation20] of low certainty.
Berlowitz and Tamplin [Citation9] concluded that additional research is needed to determine whether IMT has an effect on lung function, respiratory strength and any carryover effects to quality of life. They also state the need to discern an optimum method and treatment length for IMT [Citation9]. This gap in literature contributed to the overall aim of this systematic review. Additionally, the findings from Raab et al. [Citation16] may assist in understanding the impact IMT has at reducing respiratory complications, as a means to improve quality of life. However, whether IMT provides enough of a training effect on maximal inspiratory pressure to improve respiratory strength needs to be explored. Given this, investigating the impact of IMT on respiratory strength in adults with SCI is an important and worthwhile area of research because of the potential to improve patient care.
Therefore, the research questions for this systematic review are:
Does IMT improve respiratory muscle strength, lung function values and influence quality of life?
Is there an optimal length of intervention and resistance device for performing IMT?
Methods
Identification of studies and search strategy
A search was performed using the following databases: CENTRAL, CINAHL, MEDLINE, PEDRo, and PubMed. Searches were conducted on June 16th, 2022. See Supplemental File Appendix 1 on the eAddenda for the full search strategy and search terms. Following the database searches, grey literature concerning the Conference Proceedings Citation Index (CPCI-S), Current Controlled Trials (ISRCTN Registry), National Institute of Health Clinical Trials Database (ClinicalTrials.gov), the WHO International Clinical Trials Registry Platform and System for Information on Grey Literature in Europe, and the Science Citation Index Expanded (SCI-E) through ISI Web of Science, as well as the reference lists of included papers were examined for additional relevant articles. Two reviewers (AW and RS) independently performed initial screening of titles and abstracts against the eligibility criteria (Box 1). After non-relevant articles were discarded, a second screening involved reading full texts and analysing whether studies met the eligibility criteria. Final decisions regarding article selection were then made.
Box 1. Inclusion criteria.
Design
Randomised controlled trial
Participants
Adults ( >18 years old)
Traumatic spinal cord injuries, acute or chronic
American Spinal Injury Association (ASIA) levels A, B, C, D, incomplete or complete injuries
Intervention
Inspiratory muscle training with a device that provides resistance on inspiration
Intervention at least 2 weeks long, frequency of sessions at least 2 days per week
Training can include a warm-up and cool down, but no adjunct therapies combined with inspiratory muscle training
Based in hospital or community settings
Duration, frequency and intensity of training must be recorded
Outcome measures
Primary: maximal inspiratory pressure (MIP), quality of life (QOL)
Secondary: maximal expiratory pressure (MEP), forced expiratory volume in 1 second (FEV1)
Comparisons
Inspiratory muscle training versus no training, sham intervention or usual care
Assessment of characteristics of studies
Quality
Methodological quality of included studies was assessed using the Cochrane Risk of Bias tool, assessing each domain of bias as high, low or unclear. Criteria for making judgements about risk of bias was provided from the Cochrane Handbook for Systematic Reviews [Citation21]. The GRADE [Citation20] tool was used to grade the overall body of evidence gathered in this review, dictating the certainty of each outcome as high, moderate, low and very low.
Participants
Studies were included if participants were >18 years old, had suffered a traumatic spinal cord injury, at ASIA levels A, B, C, or D. Individuals could be in the acute (<3 months) or chronic (>3 months) stage of their injury. Data was extracted regarding participants age, sex, injury type (i.e., Level and ASIA classification), and time since diagnosis.
Intervention
The experimental intervention was IMT, using an inspiratory training device (PowerBreathe®, HaB International ltd, Southam, UK; Philips Threshold® IMT, Respironics Inc, Parsippany, New Jersey; Respifit S® electronic, Eumedics GmbH, Purkersdorf, Austria; DHD Medical Products, Diemolding healthcare Division, Canatota NY) that provides resistance on inspiration. Data was extracted about the type of device used, the duration, resistance and frequency of training, as well as location where training took place. Control interventions were sham treatments, no training or usual care.
Outcome measures
The primary outcome measures were inspiratory muscle strength, and quality of life. Inspiratory muscle strength was measured via maximal inspiratory pressure, and assessed using a manometer, with values expressed in cmH2O. Quality of life measures were the Medical Outcomes Short Form Survey (SF-36 or SF-12)—with data regarding the physical and mental health components extracted.
The secondary outcome measures were lung function, expiratory muscle strength, and likelihood of developing pneumonia. The measure for lung function was forced expiratory volume in 1 s—assessed by spirometry and expressed in litres. Expiratory muscle strength was measured via maximal expiratory pressure using a manometer and expressed in cmH2O.
Data analysis
Two reviewers (AW and RS) used standard forms to extract data from the studies. Post-intervention data was deemed continuous, and pooled using meta-analysis. Negative values were standardised. Data was expressed as a mean difference (MD) or standardised mean difference (SMD). A 95% confidence interval (CI) was set and statistical significance at p < 0.05. Meta-analysis was performed with the Review Manager (RevMan 5.4.1, The Cochrane Collaboration, 2020) (RevMan) statistical software using the Generic Inverse Variance Method, with a random-effects model. A sensitivity analysis was also planned to address the secondary research question around the impact of duration of intervention and type of device. Selected studies were pooled or sub-grouped together using RevMan. Data was expressed as a mean difference, with 95% CI and statistical significance at p < 0.05.
Results
Study selection
The search yielded 624 articles from databases and grey literature sources, with 70 full-text articles assessed for eligibility after initial screening of titles and abstracts, and removal of duplicates. After reading full-text articles, 14 were excluded as they did not perform IMT, 4 did not use an inspiratory resistance device, 31 were not a randomised control trial, 6 performed both expiratory muscle training and IMT, 6 did not possess outcome measures of interest, and 3 consisted of participants who had other neurological conditions. The remaining six studies were included in this systematic review ().
Figure 1. Flow of studies through the review.
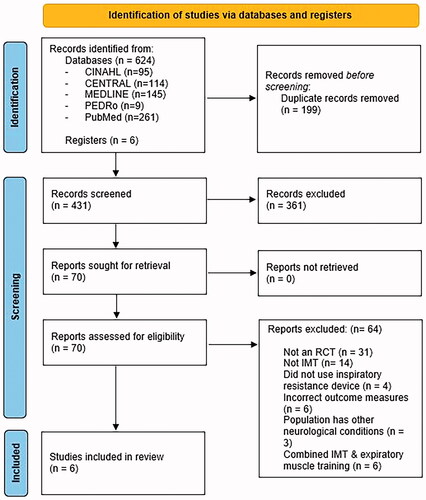
Study characteristics
The characteristics of included studies are presented in .
Table 1. Summary of included studies (n = 6).
Participants
The six included studies had a total of 124 participants, with mean ages between 27 and 46 years old. Participants had SCI’s spanning C4 to T9, with ASIA classifications A, B, C, and D. Time since diagnosis ranged from two days to 15 years. Included studies had small sample sizes, ranging between 1021 to 40 participants [Citation5].
Intervention
Three studies used inspiratory training devices that provided a resistance and were spring loaded (PowerBreathe®, HaB International ltd, Southam, UK; Philips Threshold® IMT, Respironics Inc, Parsippany, New Jersey), while the other three studies used devices that were not spring loaded (Respifit S® electronic, Eumedics GmbH, Purkersdorf, Austria; DHD Medical Products, Diemolding healthcare Division, Canatota NY). Duration of IMT interventions ranged from four to eight weeks, with a training frequency of four to seven sessions a week. Three studies trained for 15–20 min, or 30 repetitions twice a day [Citation8,Citation11,Citation14], while other studies trained for 10–15 min once a day [Citation5,Citation12,Citation13]. Levels of resistance participants trained at varied from 40% of their baseline maximal inspiratory pressure to 80%. Studies using the Diemolding Healthcare Division (DHD) medical products inspiratory muscle trainer (DHD Medical Products, Diemolding healthcare Division, Canatota NY) began training at the smallest resistance, which was measured as the diameter of the hole they inspired through (7 mm). Follow-up was immediately after interventions ended, excluding Postma et al. [Citation5] who performed an additional follow-up at 16 weeks and one year post intervention.
Outcome measures
All studies measured inspiratory muscle strength using a manometer through maximal inspiratory pressure [Citation5,Citation8,Citation11–14], with five studies also assessing maximal expiratory pressure [Citation5,Citation8,Citation12–14]. Quality of life was assessed in two studies [Citation5,Citation12], using the 12-item version of the Medical Outcomes Short-form Health Survey [Citation22], and the 36-item version of the Medical Outcomes Short-form Health Survey [Citation23]. Both studies presented results of the physical and mental health components of the measure separately. For change in lung function, forced expiratory volume in 1 s was assessed via spirometry [Citation5,Citation8,Citation12,Citation14]. To determine whether IMT improved respiratory muscle strength enough to prevent the development of pneumonia, the MD generated for MIP was applied to strength discriminators for pneumonia [Citation16].
Risk of bias
The highest source of bias was due to incomplete outcome data, as participants were lost to follow-up, or missed a large proportion of treatment sessions. Of the studies where there was incomplete outcome data [Citation5,Citation8,Citation11,Citation12], none completed an intention-to-treat analysis. However, Postma et al. [Citation5] completed a per protocol analysis. All studies analysed outcomes they had stated a priori, giving a low risk for reporting bias. Two studies provided no information regarding the blinding of participants and personnel [Citation8,Citation11], while two others provided insufficient information to ensure participants and personnel were not aware of the experimental conditions [Citation5,Citation13]. Regarding detection bias, three studies did not state whether participants and personnel were blinded to outcome assessment [Citation8,Citation11,Citation14], creating an unclear risk of bias. The method of allocation concealment was not described in Derrickson et al. [Citation11] or Liaw et al. [Citation8], and the consecutive randomisation approach used by Mueller et al. [Citation12] is not adequate to assume participants were unaware of upcoming group allocations. Random sequence generation was implemented for all interventions, however Mueller et al. [Citation12] did not mention their method for doing so, creating an unclear risk of bias ().
Figure 2. Risk of bias of included studies assessed using the Cochrane Risk of Bias Tool.
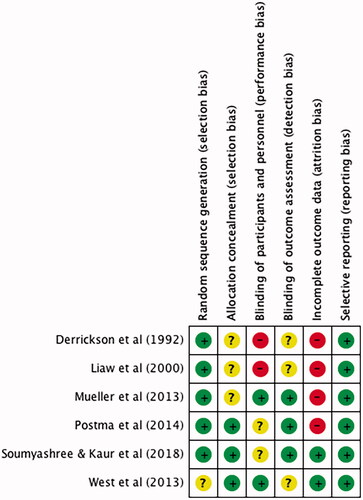
Effect of inspiratory muscle training
Respiratory muscle strength
All six studies [Citation5,Citation8,Citation11–14] totalling 124 participants reported the effect of IMT on maximal inspiratory pressure immediately post intervention. All respiratory pressures were expressed as absolute values. Compared to control (sham or no intervention), IMT significantly improved maximal inspiratory pressure by an average of 15.72 cmH2O (95% CI 5.02 to 26.41) ().
Figure 3. Mean difference (95% CI) in maximal inspiratory pressure (cmH2O) due to inspiratory muscle training, estimated by pooling data from six studies (n = 124).
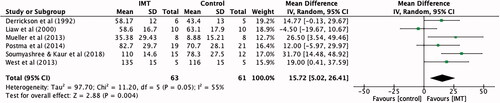
Five studies [Citation5,Citation8,Citation12–14] totalling 113 participants reported the effect of IMT on maximal expiratory pressure. When compared to control, maximal expiratory pressure improved by an average of 5.19 cmH2O (95% CI −4.16 to 14.55), however this was not statistically significant ().
Figure 4. Mean difference (95% CI) in maximal expiratory pressure (cmH2O) due to inspiratory muscle training, estimated by pooling data from five studies (n = 113).

Quality of life
Two studies [Citation5,Citation12] provided data on 56 participants, regarding the effect of IMT on physical and mental quality of life. There was a non-significant standardised mean difference in physical quality of life of 0.12 (95% CI −1.01 to 1.25) for IMT when compared to control groups (). IMT did not improve the mental component of quality of life compared to control groups, producing a standardised mean difference of −0.20 (95% CI −1.72 to 1.33) ().
Figure 5. Standardised mean difference (95% CI) in quality of life: physical component due to inspiratory muscle training, estimated by pooling data from two studies (n = 56).

Figure 6. Standardised mean difference (95% CI) in quality of life: mental component due to inspiratory muscle training, estimated by pooling data from two studies (n = 56).

Lung function
Lung function measured via forced expiratory volume in 1 s was reported in four of the studies [Citation5,Citation8,Citation12,Citation14] totalling 86 participants. IMT improved forced expiratory volume in 1 s compared to control by an average of 0.26 L (95% CI −0.19 to 0.7)—however there was not a significant difference in experimental over control groups (p = 0.26) ().
Figure 7. Mean difference (95% CI) in Forced expiratory volume in 1 s (L) due to inspiratory muscle training, estimated by pooling data from four studies (n = 86).
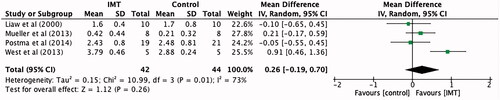
Sensitivity analyses
Changes in maximal inspiratory pressure for spring-loaded and non-spring-loaded devices
Three studies [Citation5,Citation13,Citation14] totalling 77 participants used a spring-loaded device (PowerBreathe®, HaB International ltd, Southam, UK; Philips Threshold® IMT, Respironics Inc, Parsippany, New Jersey) for IMT. Analysing only these studies, IMT significantly improved maximal inspiratory pressure compared to control groups, with an average of 21.18 cmH2O (95% CI 9.65 to 32.72).
Three studies [Citation8,Citation11,Citation12] totalling 47 participants used a non-spring-loaded threshold device (Respifit S® electronic, Eumedics GmbH, Purkersdorf, Austria; DHD Medical Products, Diemolding healthcare Division, Canatota NY) for IMT. IMT did not significantly improve maximal inspiratory pressure compared to control groups, producing a MD of 10.86 cmH2O (95% CI −6.22 to 27.94) ().
Figure 8. Mean difference (95% CI) in maximal inspiratory pressure due to inspiratory muscle training, estimated by pooling data from six studies, sub-grouped into studies utilizing non-spring-loaded and spring-loaded threshold devices (n = 124).
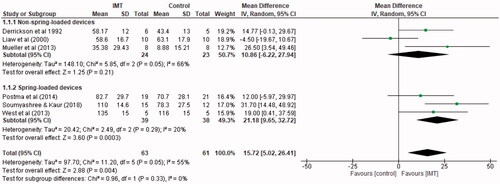
Changes in maximal inspiratory pressure for six and eight week interventions
Four studies [Citation5,Citation8,Citation12,Citation14] with a sample of 86 participants performed IMT interventions which lasted six and eight weeks. When compared to control, six week interventions produced a non-significant effect for IMT, with an average maximal inspiratory pressure of 6.61 cmH2O (95% CI −16.39 to 29.61). For eight week interventions, IMT had a significant effect over control, producing an average maximal inspiratory pressure of 17.5 cmH2O (95% CI 3.36 to 31.66) ().
Figure 9. Mean difference (95% CI) in maximal inspiratory pressure due to inspiratory muscle training, estimated by pooling data from four studies, sub-grouped into six and eight week interventions (n = 86).
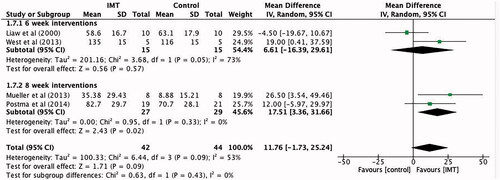
Certainty of evidence
Using the GRADE assessment, the outcome maximal inspiratory pressure showed moderate quality evidence, due to risk of bias concerning blinding of participants, outcome assessment and attrition bias. Maximal expiratory pressure and forced expiratory volume in 1 s had low quality of evidence as small sample sizes and wide confidence intervals included both no effect and appreciable benefit, leading to imprecision. The mental and physical quality of life outcomes gave very low quality of evidence, as there was considerable heterogeneity between study results, leading to inconsistency ().
Table 2. Quality of evidence using the GRADE approach (inspiratory muscle training compared to control for adults with spinal cord injuries).
Discussion
The aim of this systematic review was to determine if IMT is effective at improving respiratory muscle strength, lung function and quality of life, and whether any improvements in strength were enough to lower an individual with SCI’s risk of developing pneumonia. A secondary aim was to explore whether intervention, duration or type of device impacted on effectiveness. IMT was found to have a statistically significant effect on inspiratory muscle strength. However, there was a lack of sufficient evidence to reach a clear conclusion on quality of life, maximal expiratory pressure, or forced expiratory volume in 1 s. Therefore, maximal inspiratory pressure may be a better measure of improvement for respiratory muscle strength, especially in people with lesions where the inspiratory muscles innervate (C2-T11).
The meta-analyses for maximal inspiratory pressure had the largest sample size (n = 124), and the largest effect size to favour the intervention (MD 15.72 cmH2O, 95% CI 5.02–26.41). This is consistent with several other systematic reviews that examined IMT in SCI [Citation4,Citation9,Citation10,Citation19]. The sensitivity analysis comparing maximal inspiratory pressure scores for six and eight week interventions showed a significant effect favouring eight week interventions (MD 17.5 cmH2O, 95% CI 3.36 to 31.66) over six week (MD 6.61 cmH2O, 95% CI −16.39 to 29.61). Skeletal muscle fibre hypertrophy can occur with strength training sessions performed two to three times per week, with resistance progressively increased [Citation24]. Participants in the eight week intervention group had more total IMT sessions than the six week, allowing them more time to train under increasing resistance. This extra time and training sessions may explain why the Mean Difference for maximal inspiratory pressure was significantly improved compared to results for six week interventions.
To further analyse which experimental protocols were most conducive to generating improvements in maximal inspiratory pressure, a sensitivity analysis considering only IMT devices that were spring-loaded was performed. The Mean Difference improved from an original maximal inspiratory pressure of 15.72 cmH2O (95% CI 5.02–26.41) to 21.18 cmH2O (95% CI 9.65–32.72), with a significance of p = 0.0003. The statistical heterogeneity also improved from 55% to 20%. Conversely, the sensitivity analysis for maximal inspiratory pressure considering non-spring-loaded devices found a non-significant Mean Difference and greater heterogeneity between studies. These results suggest that ideal experimental conditions for IMT may involve at least eight week interventions, using spring-loaded inspiratory threshold devices (PowerBreathe®, HaB International ltd, Southam, UK; Philips Threshold® IMT, Respironics Inc, Parsippany, New Jersey).
A 2009 update of the American Thoracic Society and the European Respiratory Society’s statement on respiratory muscle testing proposes reference equations for both the mean clinically important difference (MCID) and lower limit of normal (LLN) values for maximal inspiratory and maximal expiratory pressure, according to age and sex [Citation25]. This would assist in determining clinical relevance of our results for these outcomes. However, due to variability in weight, lesion-level and ASIA classification, there is a broad spectrum of respiratory strength and lung volumes one may expect to find clinically in individual’s with SCI’s. Given this, a study by Mueller et al. [Citation26] developed regression coefficients to better determine the lesion-specific reference values for maximal inspiratory pressure in individuals with SCI’s ().
Figure 10. Regression coefficients and 95% confidence intervals to determine lesion- specific MIP and MEP values, using constants developed by Mueller et al. [Citation26].
![Figure 10. Regression coefficients and 95% confidence intervals to determine lesion- specific MIP and MEP values, using constants developed by Mueller et al. [Citation26].](/cms/asset/af24ad45-894e-4649-95d2-14dd11c07b8f/idre_a_2107085_f0010_b.jpg)
Using the regression coefficients, a 15% increase in maximal inspiratory pressure is needed for individuals with ASIA A and B lesions to obtain enough respiratory strength to avoid developing pneumonia [Citation16]. For individuals with ASIA C and D lesions, a maximal inspiratory pressure of 93.5 cmH2O has been identified as the discrimination between individuals with and without pneumonia [Citation16]. An example of the equation is provided below using mean participant characteristics from Postma et al. [Citation5], along with the MD for maximal inspiratory pressure determined in this review ().
Table 3. Example equation to determine lesion-specific MIP value.
The average pre-intervention lesion-specific maximal inspiratory pressure value is 56.8 cmH2O. Therefore, an increase of 8.52 cmH2O is needed to avoid developing pneumonia. Since the MD for maximal inspiratory pressure is 15.72 cmH2O (95% CI 5.02–26.41), using IMT to improve maximal inspiratory pressure may decrease an individual's chances of developing pneumonia—depending on the pre-intervention lesion-specific maximal inspiratory pressure level (). This is an important clinical consideration—since pneumonia is one of the most common risk factors for mortality in SCI [Citation2].
Results of the quality of life meta-analyses were not significant, however improvements in physical quality of life favoured the IMT group (SMD 0.12, 95% CI −1.01 to 1.25, p = 0.84). This is continuous with the results found in the systematic review by Van Houtte et al. [Citation15], as significant improvements in respiratory strength were coupled with improvements in health-related quality of life. However this was not the same case for mental quality of life, as improvements were not mutually exclusive to the experimental group participants were in (SMD −0.2, 95% CI −1.72 to 1.33, p = 0.8). This may be attributable to several factors such as time since injury, and coping with the impact of a new disability and its effect on social participation, dependency, acceptance, social relations and completion of daily tasks [Citation27].
Postma et al. [Citation5] used the SF-36 version of the Medical Outcomes Short-Form Health Survey [Citation23], while Mueller et al. [Citation12] used the SF-12 [Citation22] version. Both outcomes are proven reliable and valid in people with SCI [Citation22,Citation28,Citation29]. However, assessment of the mental component for the SF- 36 was based on groups of individuals one and five years post SCI [Citation29]. Since Postma et al. [Citation5] recruited participants two to four months post SCI, this measure may not be sensitive enough to detect initial changes an SCI has on mood and emotional wellbeing.
A strategy to more accurately assess participants quality of life could be performing IMT in group sessions. This was done by Roberts et al. [Citation30], who found patients were more motivated to complete IMT when done as a group. However, they state a focus group or patient satisfaction questionnaire needs to be undertaken to obtain qualitative feedback on factors that may influence patient’s adherence and motivation to compliance with IMT long-term.
Meta-analyses for forced expiratory volume in 1 s found the mean difference crossed the line of null effect, and there was no statistically significant difference for IMT compared to control groups (MD 0.26 L, 95% CI −0.2 to 0.7, p = 0.26). Other systematic review’s on IMT were inconclusive as to the effect on forced expiratory volume in 1 s [Citation4,Citation18], although Lemos et al. [Citation19] did find a significant improvement in forced expiratory volume in 1 s concerning RMT interventions (through narrative synthesis). The participants had injuries spanning C2-L3—meaning some individuals did not lose innervation to their expiratory muscles. This may explain the discrepancy in results for forced expiratory volume in 1 s compared to this review.
The meta-analyses for maximal expiratory pressure did not produce a statistically significant effect for IMT, however the pooled effect favoured the intervention compared to control group (MD 5.19 cmH2O, 95% CI −4.16 to 14.55). The expiratory muscles are innervated through levels T1-T12 [Citation3,p.669], therefore IMT is unlikely to make a difference in maximal expiratory pressure as most of the individuals in the included studies had cervical spine injuries. Patients with these injuries are likely to need manual assistance to perform any forceful expiration e.g., an assisted cough [Citation3, p.673].
In an attempt to minimize methodological bias, the GRADE approach was implemented. This took into consideration the large effect size present in each meta-analyses—allowing for more accurate recommendations to be made. Berlowitz and Tamplin [Citation9] performed a GRADE analysis across similar outcomes, with comparable reasons for downgrading evidence. However, the recommendations for maximal inspiratory pressure differed in that this systematic review graded the quality of the evidence higher. This could be attributable to more recent randomised controlled trials that were included in this review, which gathered significant results using newer threshold training devices with a spring-loaded component.
Another aspect that threatened methodological quality of this review is combining articles that are considerably heterogenous. This was found concerning devices used to deliver IMT, training protocols of studies, participant characteristics and comparison control groups. While some of these differences allowed for conclusions to be drawn concerning ideal training length or more effective device—an increase in homogeneity would have improved the certainty of this evidence. Sheel et al. [Citation4] addressed participant heterogeneity as an “essential consideration” due to the different effect IMT will have on participants with cervical compared to thoracic injuries. Given this, Sheel et al. [Citation4] and Van Houtte et al. [Citation15] narratively synthesized results of their systematic reviews. Berlowitz and Tamplin [Citation9] identified similar factors for heterogeneity, but performed a meta-analysis as they had enough studies consisting of similar outcome measures. However, they limited subgroup analysis to outcomes with data for three or more trials, to increase internal validity. This was also performed by this systematic review, in an effort to deliver trustworthy results and minimize reporting bias. However, subgroup analysis considering intervention effect on treatment intensity and time since injury was not performed, as studies had high variability in training intensities and injury duration.
Moderate to high risk of bias was observed across the included studies. Many articles reported participants withdrew after studies had begun, and did not use an intention-to-treat analysis to account for missing outcome data. This created high-risk for attrition bias in four [Citation5,Citation8,Citation11,Citation12] of the six studies included, as they did not obtain enough participants to achieve an adequately powered sample size (of those that stipulated on this before-hand). Therefore, losing participants threatened statistical power of each study, making it difficult to detect a true difference between experimental groups that was due to the intervention itself.
Blinding of participants and personnel as well as outcome assessment was another source of bias amongst included studies. A recently published protocol concerning the use of IMT and postoperative pulmonary complications [Citation31] is attempting to minimize blinding and allocation bias by including a low resistance and high resistance IMT group. They stated including a low resistance group is likely to blind participants with respect to their allocation, as they are likely to be unfamiliar with IMT, so are unlikely to have expectations of benefit from being in one group rather than another. Blinding and allocation bias has also been found in other systematic reviews involving IMT with participants who have asthma [Citation32], cystic fibrosis [Citation33] and SCI [Citation9]. However, a systematic review analysing IMT with people who have COPD included multiple randomised controlled trials that tried to minimize allocation bias using sham inspiratory training devices [Citation34]. It is difficult to achieve blinding in rehabilitation and inpatient settings, but the strategies highlighted above may help to mitigate these effects.
The small sample sizes and heterogeneity of participants in the included studies also limited the generalizability of results from this review, as confounding factors such as age, ASIA classification, and time since injury were not balanced across studies. However, even if studies do achieve an adequately powered sample size, participant withdrawal is highly likely due to the nature of SCI’s e.g., pressure sores, early discharge, complications not related to intervention, or declining further participation. There are also limitations concerning the method for outcome assessment on individuals with SCI’s. The American Thoracic Society and the European Respiratory Society have adapted guidelines for respiratory assessments involving pressure manometers and the use of spirometry [Citation35], however discrepancy in the position participants were measured in may have attributed to statistical and clinical heterogeneity across the included studies.
In recent years, IMT has grown in popularity, bringing about changes to its design. Two of the devices used in the included studies [Citation8,Citation11,Citation12] involve flow dependent resistance (Respifit S® electronic, Eumedics GmbH, Purkersdorf, Austria; DHD Medical Products, Diemolding healthcare Division, Canatota NY). A limitation to these devices is that the load is generated by airflow, i.e.; if a participant does not inspire forcefully enough, there is no training load [Citation36]. This parameter is difficult to control for, and could have impacted the training effect on participants in these studies.
The other two devices used in the included studies [Citation5,Citation13,Citation14] work via a one-way spring-loaded threshold valve [Citation36]. This type of device is advantageous, as the valve closes during inspiration and requires participants to inhale hard enough to open the valve and let air enter [Citation36]. Therefore the inspiratory pressure remains constant, regardless of how quickly or slowly participants breathe in. A study on individuals with COPD demonstrated that inspiratory pressure load is an important variable in IMT. They found that training at higher pressure loads, using a pressure-threshold device significantly improved respiratory muscle strength, endurance and exercise tolerance compared to patients with COPD who trained at lower pressure loads [Citation37]. A similar effect was illustrated in this systematic review, as the sensitivity analysis for spring-loaded devices (PowerBreathe®, HaB International ltd, Southam, UK; Philips Threshold® IMT, Respironics Inc, Parsippany, New Jersey) showed greater improvement in respiratory muscle strength over flow-dependent devices (Respifit S® electronic, Eumedics GmbH, Purkersdorf, Austria; DHD Medical Products, Diemolding healthcare Division, Canatota NY). This tells us that including both spring-loaded and non-spring loaded devices contributes to clinical heterogeneity, and lower estimates of treatment effect. This information will allow researchers to better understand the parameters that create a true intervention effect for IMT.
IMT has been proven effective in intensive care settings on non-SCI mechanically ventilated patients as part of their physiotherapy [Citation38]. It’s proven feasible and safe for adults with acute, complete cervical and thoracic SCI whose respiratory status was stable [Citation7], and increases respiratory strength irrespective of time since injury, or degree of injury completeness [Citation6]. Participants in the included studies of this systematic review handled the device independently, with little supervision, and in many different settings—as the device is small and portable. IMT can be implemented alongside regular physiotherapy, and is not time intensive—with benefits found between 10 and 20 min a day from the studies included in this review. Additionally, none of the included studies reported adverse outcomes as a result of using IMT, and those that included patient satisfaction scores reported higher levels of satisfaction and motivation [Citation5,Citation14]. Considering these factors, IMT should be implemented as a routine therapy for people with SCI [Citation6].
Previous randomised controlled trials and systematic reviews have provided a strong case for IMT in SCI—however few considered evidence of carryover beyond the training period. Performing IMT as a group may help influence long-term adherence [Citation30], however further analysis needs to be done before any conclusions are drawn. Motivation for people to continue with IMT may be based on changes in outcome measures that are perceived by them to be important, such as reductions in dyspnoea or improvements in exercise capacity, social participation and decreased hospital admissions [Citation39]. Given this, studies which consider obtaining qualitative data from patient focus groups or semi-structured interviews would help with understanding the factors behind motivation and adherence to IMT. This data could inform the creation of guidelines for the use of IMT, so it can be implemented in various clinical settings of individuals with SCI’s.
The results of this review indicate IMT can improve respiratory muscle strength in people with an SCI, no matter their level of injury or completeness. However, outcomes such as quality of life, maximal expiratory pressure, and forced expiratory volume in 1 s show no clear evidence of a benefit from IMT itself. Further evidence is therefore needed as to the benefit of IMT on these outcomes, in addition to MIP. Interventions that last eight weeks and utilize spring-loaded pressure-threshold devices are the most ideal experimental conditions, and have potential to bring about the greatest improvements in respiratory strength. Furthermore, IMT may help decrease an individual with SCI’s risk of mortality and morbidity due to respiratory complications such as pneumonia. However, these results should be interpreted with caution due to the heterogeneous nature of included studies, and small sample sizes. Although IMT is feasible and safe to implement in clinical practice, future trials are needed to determine more robust recommendations at this time.
Provenance
Not invited. Peer-reviewed.
Supplemental_File_Appendix.docx
Download MS Word (14.2 KB)Disclosure statement
No potential conflict of interest was reported by the author(s).
Additional information
Funding
References
- Fehlings M, Tetreault L, Wilson J, et al. A clinical practice guideline for the management of acute spinal cord injury: introduction, rationale, and scope. Global Spine J. 2017;7(3 Suppl):84S–94S.
- DiMarco A, Dawson N. Risk factors for mortality in spinal cord injury. J Spinal Cord Med. 2014;37(6):670–671.
- Main E, Denehy L. Cardiorespiratory physiotherapy: adults and paediatrics, 5th ed. UK: Elsevier, 2016. pp. 667–673.
- Sheel W, Reid W, Townson A, et al. Effects of exercise training and inspiratory muscle training in spinal cord injury: a systematic review. J Spinal Cord Med. 2008;31(5):500–508.
- Postma K, Haisma J, Hopman M, et al. Resistive inspiratory muscle training in people with spinal cord injury during inpatient rehabilitation: a randomised controlled trial. Phys Ther. 2014;94(12):1709–1719.
- Boswell-Ruys C, Lewis C, Wijeysuriya N, et al. Impact of respiratory muscle training on respiratory muscle strength, respiratory function and quality of life in individuals with tetraplegia: a randomised clinical trial. Thorax. 2020;75(3):279–288.
- McDonald T, Stiller K. Inspiratory muscle training is feasible and safe for patients with acute spinal cord injury. J Spinal Cord Med. 2019;42(2):220–227.
- Liaw M, Lin M, Cheng P, et al. Resistive inspiratory muscle training: its effectiveness in patients with acute complete cervical cord injury. Arch Phys Med Rehabil. 2000;81(6):752–756.
- Berlowitz D, Tamplin J. Respiratory muscle training for cervical spinal cord injury. Cochrane Database Syst Rev. 2013;7:CD008507. 10.1002/14651858.CD008507.pub2.
- Tamplin J, Berlowitz D. A systematic review and meta-analysis of the effects of respiratory muscle training on pulmonary function in tetraplegia. Spinal Cord. 2014;52(3):175–180.
- Derrickson J, Ciesla N, Simpson N, et al. A comparison of two breathing exercise programs for patients with quadriplegia. Phys Ther. 1992;72(11):763–769.
- Mueller G, Hopman M, Perret C. Comparison of respiratory muscle training methods in individuals with motor and sensory complete tetraplegia: a randomised controlled trial. J Rehabil Med. 2013;45(3):248–253.
- Soumyashree S, Kaur J. Effect of inspiratory muscle training (IMT) on aerobic capacity, respiratory muscle strength and rate of perceived exertion in paraplegics. J Spinal Cord Med. 2020;43(1):53–59.
- West C, Taylor B, Campbell I, et al. Effects of inspiratory muscle training on exercise responses in paralympic athletes with cervical spinal cord injury. Scand J Med Sci Sports. 2014;24(5):764–772.
- Van Houtte S, Vanlandewijck Y, Gosselink R. Respiratory muscle training in persons with spinal cord injury: a systematic review. Respir Med. 2006;100(11):1886–1895.
- Raab AM, Krebs J, Perret C, et al. Maximum inspiratory pressure is a discriminator of pneumonia in individuals with spinal cord injury. Respiratory Care. 2016;61(12):1636–1643.
- DeVivo MJ, Krause JS, Lammertse DP. Recent trends in mortality and causes of death among persons with spinal cord injury. Arch Phys Med Rehabil. 1999;80(11):1411–1419.
- Brooks D, O’Brien K, Geddes E, et al. Is inspiratory muscle training effective for individuals with cervical spinal cord injury? A qualitative systematic review. Clin Rehabil. 2005;19(3):237–246.
- Lemos J, da Cunha F, Lopes A, et al. Respiratory muscle training in non-athletes and athletes with spinal cord injury: a systematic review of the effects on pulmonary function, respiratory muscle strength and endurance, and cardiorespiratory fitness based on the FITT principle of exercise prescription. BMR. 2020;33(4):655–613.
- Guyatt GH, Oxman AD, Vist GE, et al. GRADE: an emerging consensus on rating quality of evidence and strength of recommendations. BMJ. 2008;336(7650):924–926.
- Higgins JPT, Green S. Cochrane Handbook for Systematic Reviews of Interventions Version 5.1.0 updated March 2011. The Cochrane Collaboration. 2011. http://www.handbook.cochrane.org.
- Dudley-Javoroski S, Shields RK. Assessment of physical function and secondary complications after complete spinal cord injury. Disabil Rehabil. 2006;28(2):103–110.
- Ware JE, Snow KK, Kosinski M, et al. SF-36 health survey: manual and interpretation guide. The health institute. Boston, MA: New England Medical Center; 1993.
- Garber C, Blissmer B, Deschenes M, et al. Quantity and quality of exercise for developing and maintaining cardiorespiratory, musculoskeletal, and neuromotor fitness in apparently healthy adults. Med Sci Sports Exerc. 2011;43(7):1334–1359.
- Evans JA, Whitelaw WA. The assessment of maximal respiratory mouth pressures in adults. Respir Care. 2009;54(10):1348–1359.
- Mueller G, Groot S, Woude L, et al. Prediction models and development of an easy to use open-access tool for measuring lung function of individuals with motor complete spinal cord injury. J Rehabil Med. 2012;44(8):642–647.
- Jespersen L, Michelsen S, Tjornhoj-Thomsen T, et al. Living with a disability: a qualitative study of associations between social relations, social participation and quality of life. J Disabil Rehabil. 2019;41(11):1275–1286.
- Forchheimer M, McAweeney M, Tate D. Use of the SF-36 among persons with spinal cord injury. Am J Phys Med Rehabil. 2004;83(5):390–395.
- Van Leeuwen C, Van der Woude L, Post M. Validity of the mental health subscale of the SF-36 in persons with spinal cord injury. Spinal Cord. 2012;50(9):707–710.
- Roberts K, Morgan SE, Chalke HL, et al. A service evaluation of the effectiveness of an inspiratory muscle training group for spinal cord injured patients, assessing the change in maximal inspiratory pressure and maximum phonation time. J Assoc Chartered Physiotherap Respirat Care. 2018;50:31–39.
- Pufulete M, Levett D, Rogers C, et al. Effectiveness and cost-effectiveness of INSPIRatory musclE training (IMT) for reducing postoperative pulmonary complications (PPC): a sham-controlled randomised controlled trial (RCT) (INSPIRE). 2019. University of Bristol, Protocol.
- Silva I, Fregonezi G, Dias F, et al. Inspiratory muscle training for asthma. Cochrane Database Systemat Rev. 2013;2013:CD003792.
- Reid W, Geddes E, O’Brien K, et al. Effects of inspiratory muscle training in cystic fibrosis: a systematic review. Clin Rehabil. 2008;22(10–11):1003–1013.
- Geddes E, O’Brien K, Reid W, et al. Inspiratory muscle training in adults with chronic obstructive pulmonary disease: an update of a systematic review. Respir Med. 2008;102(12):1715–1729.
- Kelley A, Garshick E, Gross ER, et al. Spirometry testing standards in spinal cord injury. Chest. 2003;123(3):725–730.
- Menzes KKP, Nascimento LRN, Avelino PR, et al. A review on respiratory muscle training devices. J Pulm Respir Med. 2018;08(02):1000451.
- Larson J, Kim M, Sharp J, et al. Inspiratory muscle training with a pressure threshold breathing device in patients with chronic obstructive pulmonary disease. Am Rev Respir Dis. 1988;138(3):689–696.
- Bissett B, Leditschke I, Green M, et al. Inspiratory muscle training for intensive care patients: a multidisciplinary practical guide for clinicians. Aust Crit Care. 2019;32(3):249–255.
- Hill M, Noonan V, Sakakibara B, et al. Quality of life instruments and definitions in individuals with spinal cord injury: a systematic review. Spinal Cord. 2010;48(6):438–450.