Abstract
Green infrastructure (GI) has been identified as a promising approach to help cities adapt to climate change through the provision of multiple ecosystem services. However, GI contributions to urban resilience will not be realized until it is more fully mainstreamed in the built environment and design professions. Here, we interrogate five key challenges for the effective implementation of GI: (1) design standards; (2) regulatory pathways; (3) socio-economic considerations; (4) financeability; and (5) innovation. Methods include a literature review, case studies, and interviews with resilience managers. We propose a people-centred and context-dependent approach to advance effective implementation of GI in urban planning. We highlight two underlying currents that run across all of the challenges – (1) the role of political will as a pre-condition for tackling all challenges holistically; and (2) the role of stakeholder engagement in achieving public support, harnessing funding, and maintaining and monitoring GI in the long term.
Highlights:
• The effective implementation of GI is context-specific and should adhere to the basic principles of appropriate technology.
• Continuous community engagement is needed to ensure the inclusivity and multi-functionality of GI.
• Challenges to successful GI are intersectional and therefore cannot be addressed singly in isolation.
1. Introduction
Cities and their inhabitants around the world are becoming more vulnerable as a consequence of rapid urbanization combined with climate change impacts (Petroski Citation2016). As storm events become more frequent and severe as a function of climate change (IPCC Citation2013), cities across the world become more vulnerable to floods, and droughts and to all the cascading effects that follow (e.g., damage to urban infrastructure, impacts on health), in context-specific situations (Oulahen et al. Citation2017). Likewise, as temperatures rise, urban residents become more vulnerable to often quite localized heat waves (such as the one in 2003 in France that may have killed as many as 15,000 people; Poumadère et al. Citation2005). But sudden climatic shocks are not the only challenge for twenty-first century cities; there are also underlying stresses. Air pollution increasingly threatens the health of residents. Automobile traffic is intensifying and making urban public spaces more dangerous. Diminution and fragmentation of urban green space robs communities of much-needed space for recreation and leisure. These processes are also harmful to the non-human environment, further undermining the urban ecosystem services upon which we all depend. Addressing the above challenges whilst also creating a platform for economic growth is a key priority for urban managers around the world.
In a step-change from previous attempts to deal with these urban challenges singly and in isolation, urban professionals and city leaders are increasingly adopting “resilience” as a framing concept, although the question then arises as to which definition of resilience should be adopted and how can it be operationalised (Ahern Citation2011). In the field of landscape and urban planning, the concept of sustainability is usually envisioned as a static state that, once achieved, will last for many generations (e.g., New Urbanism, Smart Growth; Ahern Citation2011). In contrast, resilience is increasingly understood as a fundamentally dynamic property of complex socio-technical systems: “the capacity of individuals, communities, institutions, businesses, and systems within a city to survive, adapt, and grow, no matter what kinds of chronic stresses and acute shocks they experience” (100 Resilient Cities Citation2018). Since this type of resilience clearly depends on nature-based, as well as other, solutions, city managers need to consider how they may improve resilience through nature-based solutions that optimise the ecosystem services potentially available to a greater or lesser degree in all cities. Learning how to protect critical infrastructure, human life and property through better urban planning and design is fundamental to reduce risk and enhance resilience (Pitt Citation2008). This represents a multi-stranded, and even intersectional, endeavour that therefore requires interdisciplinary and transdisciplinary approaches (Ahern Citation2011; Portman Citation2018).
Though often explored for its contributions to urban aesthetics and public health, urban green infrastructure (GI) also shows promise in enhancing urban resilience, through specifically promoting and enhancing ecosystem services linked to reduced flooding risk, urban heat island reduction, improved air quality, reduced energy consumption in buildings, carbon storage, conservation of wildlife habitat, and the provision of recreation and leisure amenities that improve the wellbeing of urban residents (Pennino, McDonald, and Jaffe Citation2016; Saleh and Weinstein Citation2016; Sutton-Grier, Wowk, and Bamford Citation2015). Given the existing built environment, these will often necessarily be hybrid approaches that combine “green,” “blue,” and “grey” infrastructures to achieve greater wellbeing benefits and more resilient communities (Jeong et al. Citation2016; Klenzendorf et al. Citation2016; Liu et al. Citation2016), particularly in cities located along the coast (Sutton-Grier, Wowk, and Bamford Citation2015). Urban GI can therefore be seen as “the creative combination of natural and artificial (green + grey + blue) structures intended to achieve specific resilience goals (e.g., flood/drought management, public health, etc.) with broad public support and attention to the principle of appropriate technology” (Staddon et al. Citation2017, 1). GI examples vary in scale of operation, from raingardens, vegetated swales, green roofs/walls and urban parks in cities, to ecological networks and coastal areas. In this study, we focused on urban GI.
Urban design professions have long celebrated the greening of cities, usually linked to utopian notions of “garden cities” and with a view to public health and the moral and spiritual impacts on citizens, rather than the functional resilience of urban systems (Howard and Osborn Citation1965). We contend, however, that the linking of GI with systemic approaches to building resilience in urban systems is something new and different.
Often cities around the world have initiated efforts toward greening after experiencing a severe event. Weather-related crises have often been the motivators for adopting GI-based approaches to enhancing resilience. For example, after Superstorm Sandy hit the east coast of the United States in 2012, New York City launched a campaign to plant and care for one million trees (Million Trees NYC Citation2018). After enduring flooding in 1997–1998, the City of Los Angeles (LA) adopted the Green Alley Development project, which aims to revitalize alleys into usable public space that features GI (The Trust for Public Land and UCLA Luskin Center for Innovation – TPL and LCI Citation2015). In the wake of severe flooding in summer 2007, authorities in the United Kingdom declared that nature-based solutions would be key to improving urban resilience (Pitt Citation2008). In 2014, after severe flooding events affected 137 cities in China, the Chinese government decided to invest heavily in GI and create “sponge cities” through pilot construction programs (Jiang, Zevenbergen, and Fu Citation2017). Though flooding is often a driver of GI uptake, other drivers include droughts (France, India), acid mine drainage (South Africa) and safety of poorer communities (Brazil, India).
2. Methods
In this study, we build upon previous research examining the challenges associated with the implementation of GI in cities around the world that resulted in a report submitted to Arup’s Resilience Shift initiative (Staddon et al. Citation2017). For this study, we identified five challenge domains that we used as a framework for this paper: (1) design standards; (2) regulatory pathways; (3) the socio-economic challenges; (4) financeability; and (5) innovation. For example, Li et al. (Citation2017), conducted a survey of 30 pilot “sponge cities” in China and found that the three main challenges for the implementation of GI are physical-technical, financial, and regulatory. Similarly, a review study by the National Research Council (NRC Citation2009) identified three types of barriers, (institutional, technological, and perceptual), to the implementation of GI. The socioeconomic and innovation challenges of urban resilience have also been identified by Ahern (Citation2011), and Gould and Lewis (Citation2016), who suggest that the lack of attention to social inclusion in GI programmes risks visiting a form of what they call “green gentrification” on cities. Financial challenges are also important barriers to GI implementation, as GI and other resilient measures do not easily match the most common methods of development finance (Gersonius et al. Citation2016).
In preparing the initial dataset to support the 2017 report (Staddon et al. Citation2017), we sought to ensure that we had case studies from all world regions, representing each of the five challenge domains. In , we present the 64 peer-reviewed articles from different regions of the world, published in the last 5 years (2013–2018), according to the five challenges to mainstreaming GI. Achievement of these objectives was affected through secondary literature, case studies of projects currently underway with Arcadis (a Netherlands-based consultancy with projects worldwide) and interviews with Resilience Managers at a selection of “100 Resilient Cities” and “C40” cities. We are not saying that all case studies of urban GI will always manifest all five challenge domains, but rather that, from a global perspective, these five challenge domains seem more or less present in all the case studies we observed through the literature and all the interviews we conducted.
Table 1. Place-based studies that examine the five challenges for implementing GI across the world.
In this paper, we explore each of these challenges based on our knowledge of urban GI projects worldwide. Whilst all five challenge areas are important, we are particularly keen to demonstrate that the socio-economic domain is both under-appreciated and yet critical to the success of urban GI schemes. We contend that, in most cases, good engineering and management, whilst important, are not in themselves sufficient to guarantee success of urban GI. A key to success is attention to the equitable distribution of benefits and social inclusion in the entire GI project life cycle. To accomplish this, we draw on cases from around the world that have made efforts to implement GI as a strategy to enhance urban resilience to better understand how to address the key challenges for implementation. We present an overview of each challenge and highlight some efforts underway to address or mitigate them. In the concluding section, we extract lessons that can direct urban design and planning efforts towards mainstreaming the use of GI approaches to make our cities and their inhabitants safer and more secure.
2.1. Challenge #1: Design standards
The design standards challenge reflects the significant uncertainty around how best to plan, design, implement, and maintain GI (Baptiste, Foley, and Smardon Citation2015; Campbell, Svendsen, and Roman Citation2016; Sinnett et al. Citation2018). From our interviews with resilience managers, we found that there is limited capacity in the design phase. Technological barriers that speak to design include deficiency of data about performance characteristics and insufficient technical knowledge and experience (NRC Citation2009). Design guidelines that are tailored to local conditions of cities and respond to their specific threats and availability of resources are essential for the successful planning, design, implementation, operation, maintenance, and evaluation of urban GI (Li et al. Citation2017).
Although GI alone may not be able to completely restore the functioning of the ecosystems, it can certainly buffer its negative effects. These buffer effects are context-specific and depend, for example, on the infiltration rate of the soil, as the case of Santa Cruz, Bolivia illustrates (Castelli et al. Citation2017). Design standards have practical and technical challenges that are also context-specific. For example, the greening of boulevards in Melbourne, Australia is very difficult because there are multiple regulations involved relating to road and electricity wire clearance (Furlong, Phelan, and Dodson Citation2018). Another design challenge is the unclear health benefits of urban nature, which are often assumed but more rarely demonstrated. It is unclear which elements of nature produce which health benefits. Some plant species may even have negative health effects (e.g., allergens; Shanahan et al. Citation2015).
We found a tension in this challenge, because GI performance and its benefits are context- and site-specific and yet, design standards imply a one-size-fits-all approach. In addition, it is not only about the physical and environmental performance of GI but also about social benefits and the need for public support, which are subjective and may vary in time and space. Yet, it is critical to consider people’s needs and beliefs during the design and planning of GI projects to achieve adaptation with public support (Derkzen, van Teeffelen, and Verburg Citation2017). Is it then about design standards, or about a standardized design process? We argue in favour of the latter. Cities must follow a standardized design process in which they devise their own context-specific technical guidelines based on performance data while considering people’s needs and beliefs.
Some cities are working to address this challenge through partnerships between public, private, non-profit, and academic research organizations. For example, in the United Kingdom, the Gloucestershire Wildlife Trust in partnership with planners and developers from the West of England and researchers from the University of the West of England recently launched the “Building with Nature” design standards for GI (Sinnett et al. Citation2017). Structured as a third-party organization, Building with Nature aims to define what ‘good green infrastructure’ is, providing a certification service through a set of publicly available standards. Building with Nature includes a maintenance commitment and must be in line with the prevailing “Working with Nature” national policy context established in the United Kingdom after the droughts and floods of the 2000s (Pitt Citation2008; Staddon Citation2010). Others argue for a hybrid certification system. For example, Castelli et al. (Citation2017) identify a green and blue certification system for new development as part of the Aguacruz Project in Santa Cruz, Bolivia. Likewise, Voskamp and Van de Ven (Citation2015) find that the integration of blue and green measures is critical, because green (vegetation) depends on blue (water) in the dry season or extreme heat conditions.
In India, there are also important efforts being undertaken to address this challenge. In mid-2017, the Delhi-based Centre for Science and the Environment published “Green Infrastructure: A Practitioner’s Guide,” which outlines methods and strategies for water sensitive urban design and planning. This guide is targeted at practitioners working at multiple scales – from individual parcels to neighbourhoods, zones, and cities – throughout the Global South (Rohilla, Jainer, and Matto Citation2017).
The case of Amaravati, India illustrates the benefits of having available GI design standards. A new capital for Andhra Pradesh state, Amaravati, is being developed with ‘smart cities’ and GI principles (Ghadei Citation2018). The state government is using a comprehensive network of urban waterways and a robust flood management system in a blue-green master plan (). The network of blue and green infrastructure covers 30% of the city and is intended to offset temperatures and mitigate flooding risk, while providing transportation networks and amenities for their residents. The Indian design standards (Ghadei Citation2018; Rohilla, Jainer, and Matto Citation2017) are being used in the design of GI. However, because there are no blue infrastructure guidelines available in India, they are drawing from the International Dutch Standard on Waterway Navigation (Staddon et al. Citation2017).
In the United States, GI design guidance and modelling assistance are provided by the US Environmental Protection Agency (USEPA) through the “Green Infrastructure Modeling Toolkit” (to manage stormwater with GI); the “Green Infrastructure Flexible Model” (a computer program that evaluates GI performance) (USEPA Citation2018a); and the “National Stormwater Calculator” (that estimates the urban water cycle – annual rainwater and runoff frequency – anywhere in the country) (USEPA Citation2018b). Similar to the Building with Nature initiative in the United Kingdom, this effort is linked to a certification process, although by a separate organization. If practitioners use the National Stormwater Calculator in the design process, they can obtain credits in the Leadership in Energy and Environmental Design (LEED) certification (Rohilla, Jainer, and Matto Citation2017). This sort of integration of GI within existing certification schemes (cf. BREEAM in the United Kingdom and Gold Star in South Africa) can be a very useful way of introducing GI to local or national design practitioner communities.
Figure 1. Blue-green master plan of Amaravati, India showing planned “greenways” and “blueways”. ©Arcadis. Reproduced by permission of Arcadis.
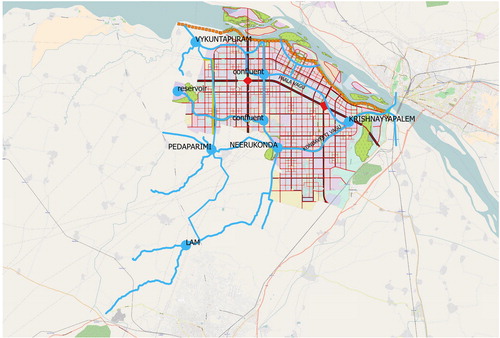
The ecological benefits of connecting GI projects at the city scale are increasingly recognized and should be considered in frameworks for design standards. In a case study of the central Nanjing district of Pukou in China, Wei et al. (Citation2018), integrate the ecological principles of landscape connectivity to their analysis of urban sustainability. The authors propose a framework for urban GI that identifies priority areas that allow functionally optimal linkages between green spaces. Likewise, in a resilience study of the Island of Dordrecht in the Netherlands, Gersonius et al. (Citation2016) found that blue-green corridors that partially remove conventional flood control defenses can actually improve flood resilience and also contribute to landscape connectivity with the Biesbosch – a conservation area that is part of Europe’s Natura 2000 network.
It is important to consider the multi-functionality of GI including, in particular, social dimensions. For example, in addition to stormwater management, GI can provide many recreational amenities during dry days, such as Rotterdam’s urban parks that can also serve – in extremis – as floodwater attenuation structures (Derkzen, van Teeffelen, and Verburg Citation2015). Likewise, GI can not only serve as wastewater treatment facilities in marginalized neighbourhoods of Lima, Peru, but also as community parks (Eisenberg et al. Citation2014). In cities in the Global South, where resources are limited, it is essential to identify priority areas for action. Addressing this challenge in Santiago, Chile, Fernández and Wu (Citation2018) proposed the “Environmental Improvement Priority Index” to identify areas with the highest level of social need, areas with the highest levels of environmental problems, and areas with both.
2.2. Challenge #2: Regulatory pathways
Further mainstreaming of urban GI faces the challenge of finding a suitable regulatory environment. Unlike, say, fire protection, few jurisdictions around the world have clear processes for regulating GI and its assumed benefits. Even in the Netherlands, a GI trailblazer in so many ways, there are no legal arrangements that give water authorities enough confidence in the longer-term benefits of resilience measures, including GI (Gersonius et al. Citation2016). Legal arrangements need to have clarity in the new distribution of responsibilities and the sustained maintenance commitment in the long term (Gersonius et al. Citation2016).
Relatively poor integration of regulatory bodies into a system that fully appreciates the multidimensional benefits of urban GI remains a key challenge (Kremer et al. Citation2016). In the United Kingdom, separate regulatory bodies see even well-planned GI only in terms of their regulatory remits; the Environment Agency sees GI in terms of water benefits in the natural environment, the Health and Safety Executive in terms of impacts on human health, etc. This has greatly hampered the mainstreaming of sustainable drainage systems (SuDS; or GI) as part of urban GI in the United Kingdom (Ellis and Lundy Citation2016). In China too, national regulations related to stormwater infrastructure systems consider only grey infrastructure systems (Li et al. Citation2017).
There are other regulatory challenges too, besides integration, including regulating a network of GI by coordinating multiple jurisdictions, as the case of Santiago, Chile demonstrates. The failed “Santiago Verde” (“Green Santiago”) Plan (2006–2011) proposed an interconnected network of GI at the regional level but remained at the conceptual stage as a consequence of the need to coordinate multiple jurisdictions and several functional sectors, all with different capacities and resources (Vásquez et al. Citation2016). In some cases, GI implementation has been possible by orchestrating bespoke planning systems from different layers of government, as the “Sponge City” case from China illustrates. After experiencing severe flooding in 2012, with damage that amounted to $1.6 billion USD, the Central Government of China launched the Sponge City Pilot Programme (Jiang, Zevenbergen, and Fu Citation2017; Staddon et al. Citation2017). This initiative is supported by the Ministry of Finance, the Ministry of Housing and Rural-Urban Development, and the Ministry of Water Resources (Dai et al. Citation2018; Li et al. Citation2017).
In addition to regulatory aporia, in some countries there is a need for collaborative action on many fronts – social, economic, political, and regulatory (Campbell, Svendsen, and Roman Citation2016). In the United States, for example, there is a need for stakeholder engagement for urban GI implementation in private property at the household level. Although there are federal regulations that mandate GI in certain vulnerable areas, there is no regulation for less vulnerable urban areas. Federal regulations mandate the use of GI in coastal areas (e.g., Coastal Zone Management Act), in areas directly linked to water bodies (e.g., USEPA’s new stormwater regulation) (Ekness and Randhir Citation2015), and in areas that provide critical habitat to endangered species (e.g., Endangered Species Act) (Martin-Mikle et al. Citation2015). In addition, new development can be regulated through building codes or through homeowner association rules, but an amendment to the US Constitution protects property rights for existing developed areas, making it impossible to enforce GI retrospectively (Baptiste, Foley, and Smardon Citation2015). For this reason, it is common to seek the voluntary participation of the community in greening projects in cities of the United States.
Property rights in the United States make it challenging to implement top-down GI initiatives in cities. However, there are other creative ways to do so, and afforestation projects are one example (e.g., the New York City’s Million Trees program; Pierre et al. Citation2016). Planting trees initiatives do not require dealing with property rights and other regulations. However, while tree-planting initiatives are growing in some cities, protecting existing trees remains a challenge. In Melbourne, there are planning regulations that mandate the inclusion of trees in new developments, but there are no regulations that protect existing trees from being removed (Furlong, Phelan, and Dodson Citation2018). Moreover, it remains unclear who is responsible for the liabilities created by trees themselves (falling trees that damage property or injure people).
Mainstreaming GI in cities in the United States not only requires the voluntary participation of citizens, but also a change in governance paradigms. Dhakal and Chevalier (Citation2016) suggest that a change of governance paradigms, from centralized to decentralized (and participatory) is necessary to effect a transition from grey to green – or even hybrid – infrastructure. The authors propose a spatial unit that is delineated by hydrological boundaries as a ‘local hydrological district’. Similar to school or fire districts, the new local hydrological district will identify, promote and regulate GI opportunities and schemes when completed. However, a regional level vision is still needed for hydrological units to function coherently amidst competing interests. However, translating theory into practice is a huge challenge. From our interviews with resilience managers we learned that there can be issues with slack technologies; there is a strong reluctance to decentralise wastewater systems, or the implementation of other technologies… this is linked to financial issues, as some technologies are costly, at least upfront.
Some cities have developed regulatory mechanisms to promote GI adoption. In South Africa, the Department of Water and Sanitation (DWS) created the National Green Drop Certification Programme to improve the performance of green infrastructure, particularly with respect to the management of water quality (Ntombela et al. Citation2016). Green Drop certification can apply to anything from a single streetscape to constructed wetlands, to much larger and more complex geographical units. In fact, the 20,000 km2 Krueger National Park (KNP) adopted Green Drop certification as a way of coordinating its efforts to better manage its complex web of ecosystem services (Water Research Commission Citation2014). The park uses constructed wetlands combined with oxidation pond systems to treat their wastewater, which complies with DWS regulations. However, the park does not exist in isolation and faces serious water quality challenges imported from neighbouring municipalities that discharge sewage and untreated industrial effluents to rivers that run through the park. KNP hopes that the Green Drop process will assist it in working with adjacent communities to better manage all waste water in the region.
Although GI regulations in some cities may be scarce or difficult to enforce, greening efforts may still happen through urban planning. Urban development in Pakistan is mostly unguided and uncontrolled, but there are some greening initiatives. Planning regulations in the Punjab province of Pakistan mandate housing development to allocate 7% of the total area for parks, and the minimum street width of 30 feet to include street landscape (Arshad and Routray Citation2018). In addition, the area under high-tension electricity lines in Punjab must be green space (Arshad and Routray Citation2018).
2.3. Challenge #3: Socioeconomic
The Urban Land Institute (ULI) (Citation2018) lists equity as one of the Ten Principles for Building Resilience, which calls for assisting the most vulnerable communities. Although promoting social equity is present in most resilience initiatives, in practice, it is a different story. Vulnerability to floods is usually greater in low-lying regions of cities, but often times, topography does not determine the distribution of risk. Instead, in many cities, it is common to find that low-income neighbourhoods have less vegetation and access to green space than wealthy neighbourhoods (Hoang and Fenner Citation2016; Willemse Citation2015). Because of the important ecosystem services that nature provides, this disproportional access to green space results in an environmental justice issue (Smiley et al. Citation2016). Green space in cities has been associated with restorative effects on wellbeing, that is, the physical, mental, and social health of the residents (Hordyk, Hanley, and Richard Citation2015; Zuniga-Teran Citation2015). In different locations around the world, marginality can arise as a function of income, age, religion, density, caste or education, and in all cases, marginality usually means lack of access to the benefits of GI (Arshad and Routray Citation2018; Escobedo et al. Citation2015). The public or private notion of green space along with its quality are important aspects to consider when assessing access to the wellbeing benefits of GI (Fernández-Alvarez Citation2017).
The timeline for shifting from grey to green infrastructure in a city also has an intrinsic equity implication. Wealthier areas are usually the first to receive the benefits of GI, while poor areas lag behind. GI projects usually happen when neighbourhoods that already have grey infrastructure in place shift to GI – in that order – leaving poor neighbourhoods behind on the timeline (Parr et al. Citation2016). For example, the wealthier and older neighbourhoods in Cape Town, South Africa, are shifting from grey to green technology, while poor neighbourhoods are still striving to build grey infrastructure (Parr et al. Citation2016). Furthermore, once the greening occurs, gentrification processes often perpetuate inequity by squeezing poorer citizens out of recently improved neighbourhoods (Gulsrud, Hertzog, and Shears Citation2018). As greening projects enhance the aesthetics and liveability in neighbourhoods, property prices rise, displacing low-income families elsewhere in a twenty-first century version of the infamous “rent gap” problem (Smith Citation1984).
At the global level, cities in the Global South face greater challenges. Cities in developing countries face severe pressures (weak, inappropriate, or absent planning, population growth, poverty), so the benefits of ecosystem services from GI are increasingly important (Lindley et al. Citation2018). Cities in Latin America, for example, have the highest growth rates, are rarely planned, and show high levels of environmental and socioeconomic inequalities (unequal distribution of hazards, risks, as well as goods and services) and inequities (unfair distribution; Fernández and Wu Citation2018). In Mexico City, GI is disproportionately distributed against density, poverty, education, and age (Fernández-Alvarez Citation2017). Globalization may contribute to this inequity by secluding production and management operation to certain pockets of the city (Lara-Valencia and Garcia-Perez Citation2015; Vásquez et al. Citation2016). Informal settlements in cities require careful planning considerations that are difficult to implement. A resilience manager reported that there are many ways in which illegal settlements interact and are part of the natural space or are particularly vulnerable near the river – so you have to plan for informality.
In Santiago, Chile, there have been some efforts to address inequities around GI. The public is demanding green spaces that include cycle lanes along the river. This demand has been addressed by local NGOs, which have engaged the community and developed GI in vulnerable neighbourhoods of Santiago (Vásquez et al. Citation2016).
Public participation has been identified as a critical aspect for implementing decisions that could affect urban residents (Petts Citation2008; Webler and Tuler Citation2006) such as GI projects. However, in some cases, people may not associate GI with climate adaptation. For example, participants of a study by Derkzen and colleagues (Citation2017) in Rotterdam, the Netherlands, recognize the impacts of climate change, but do not associate GI with an adaptation strategy. Nevertheless, people's needs and beliefs in relation to GI benefits need to be considered in any GI project to achieve legitimate adaptation, behavioural change, and public support (Derkzen, van Teeffelen, and Verburg Citation2017). The public are more likely to accept policies that affect them when they are actively involved in their definition (Giupponi and Sgobbi Citation2008). The successful implementation of GI, therefore, requires inclusive public participation and continued involvement (Baptiste, Foley, and Smardon Citation2015). It is important to recognize and protect the cultural and spiritual links between people and nature (Lindley et al. Citation2018).
Community participation, along with citizen-led knowledge, have acquired increasing attention. The Rockefeller Foundation’s review of 100 Resilient Cities’ strategies includes an important public participation component. The City of Bristol’s resilience strategy explicitly aims to build capacity in vulnerable and marginalized communities with a specific reference to GI (100 Resilient Cities and Bristol City Council Citation2016). Mexico City also places equity at the centre of their resilience strategy; and one of the pillars in their five-pillar framework is geared towards equal access to urban amenities including green space (100 Resilient Cities and Ciudad de Mexico Citation2016).
Public participation is important at both the organization and community levels. The City of Los Angeles (LA) shows an example of partnerships between local organizations and community participation in GI projects. Sadeghi et al. (Citation2016) examine the Avalon Green Alley Network, an area of poor surface water quality that constantly floods. The network is composed of six alleys, which are being renovated with underground interlocking trenches. The project is the result of a joint partnership between the Trust for Public Land, the City of LA Bureau of Sanitation, the Council for Watershed Health, the Coalition for Responsible Community Development, and the LA Conservation Corps. Support from the local community was a critical factor for the project’s success, because it could have caused major discontent in the neighbourhood. Residents were exposed to noise, dust and the prohibition of traffic on certain roads for several months. The project was possible through continuous engagement activities with the help of the “Avalon Green Team” formed of local residents, community-based groups, and school representatives. Engagement activities (community events and meetings with City council members, where residents were informed of the project and its objectives) occurred way prior to the project – they started in 2009 and construction of the project in 2015. The alleys are not only reducing flooding, but they are being transformed into liveable and safe public spaces (Sadeghi et al. Citation2016).
Although essential for effective GI implementation, public engagement may also be more difficult for low-income residents, who may not have leisure time to participate in community projects. Some people may even have negative views of trees and vegetation, which may hinder greening efforts, particularly on private land (Furlong, Phelan, and Dodson Citation2018). For others, GI can translate into ecosystem ‘disservices’ (e.g., nature can kill you), as is the case in some areas of Sub Saharan African cities (Lindley et al. Citation2018); or GI can be associated with crime and reduced wellbeing benefits, as a study in Tehran, Iran unveils (Ambrey and Shahni Citation2017). Safety concerns may drive removal of vegetation where crime rates are high (Shanahan et al. Citation2015). In Kumasi, Ghana, there is a history of neglect for green space caused by its association with habitat for snakes as well as places where criminals can hide (Nero Citation2017).
2.4. Challenge #4: Financeability
The cost of not investing in resilience initiatives – or the cost of no action – is increasing as climate change unfolds (ULI Citation2018). Cities face significant economic challenges after a flooding event because of damage to the urban infrastructure. The United States spends about $2 billion USD, on average, annually on floods (Subramanian Citation2016), while China has experienced losses that amount to about $15.77 billion USD (Li et al. Citation2017). Therefore, the flood reduction benefit of GI may be an economic incentive for cities. However, it remains unclear how to reliably estimate the costs and benefits of GI technology, and how to translate these cost/benefit calculations into financing models to fund capital and operational expenditure.
Numerous studies have found that GI is actually cheaper than traditional grey infrastructure (Levy et al. Citation2014; Li et al. Citation2017; Tayouga and Gagné Citation2016; Wild, Henneberry, and Gill Citation2017). A study by Yang et al. (Citation2015) measured the performance goal of retaining 100% of stormwater on-site and found savings of $40 million USD in stormwater infrastructure using GI strategies in Daybreak, Utah. In a life cycle analysis, Wang, Eckelman, and Zimmerman (Citation2013) compared the environmental and economic cost-effectiveness of GI compared with grey infrastructure and combined green and grey systems. Focusing on water quality, the authors found that a combined system of GI with a separate stormwater sewer system is the most cost-effective solution (Wang, Eckelman, and Zimmerman Citation2013).
Although GI may be cheaper, regulations may offset this economic advantage, particularly when the design parameters have not been institutionalized. In a cost-benefit analysis, Levy et al. (Citation2014) found that although constructed wetlands are the most economical solution to stormwater management in the United States, regulations that mandate water quality goals may hinder this economic advantage. Constructed wetlands, the authors explain, are a GI technology at the “end of the pipe” (p. 6) and therefore considered a point source for discharge; and this condition puts constructed wetlands under the US National Pollution Discharge Elimination Systems regulations. Because quality-based standards for point source discharges were developed for grey infrastructure systems, authorities use their professional judgement to evaluate compliance of GI technologies, which results in very stringent evaluations.
In contrast, in Europe, regulations for constructed wetlands are less stringent and monitoring is minimal because of institutionalized design parameters (Levy et al. Citation2014). Constructed wetlands have been operating for a long time in Europe (since the 1990s, mostly in Germany, Denmark, and Belgium); therefore, the design parameters and the resulting treatment efficiencies have been proven, or institutionalized. Therefore, there is no need to regulate a wetland that follows approved design parameters.
Fines and economic incentives can help to implement GI at the household level in cities. In the United States, federal fines for non-compliance with water quality standards can be substantial and avoiding such fees is a good incentive to implement GI. Consequently, many cities in the United States have launched economic incentives to promote GI projects, which may come from the government (via grants, loans, and fees) or from the private sector (Vogel et al. Citation2015). For example, Cleveland offers free or reduced cost rain barrels to its residents and technical support to install them correctly (Baptiste, Foley, and Smardon Citation2015). Subsidies is another economic instrument that can be used to promote GI. Rostad, Foti, and Montalto (Citation2016) recommend subsidies (e.g., tax rebates) to install rainwater harvesting systems based on the reductions in treatment costs associated with less runoff. Moreover, indirect economic incentives may also help in the wide implementation of GI. For example, the City of Chicago launched a $100,000 USD grant program to incentivize green roofs because this strategy not only reduces runoff, but also reduces energy costs (Tayouga and Gagné Citation2016).
Another financeability challenge lies in the difficulty to quantify the multi-functionality of GI (McRae Citation2016). Tucson, Arizona shows an example of GI at the end of the pipe that also functions as an amenity to the residents and a wildlife habitat. The Sweetwater Wetlands are located within the boundaries of the City of Tucson, along the Santa Cruz River, and are an integral component of a carefully planned reclaimed water system. Partially treated water from an adjacent conventional treatment works is released into the wetlands for final treatment and release into the natural environment. Effluent is also used to irrigate parks and other green space in the city, decreasing water demand, while providing a social recreational space and wildlife habitat (Sweetwater Wetlands Citation2018).
It is clear that GI is a cost-effective solution for stormwater management, but it is the wide array of ecosystem services that GI provides that makes it challenging to quantify. Therefore, it is critical to consider these benefits in any cost-analysis because they can offset the large installation costs (McRae Citation2016). Even vacant land that contains trees may have significant GI value, and this value can be calculated by considering the cost of planting the same number of similar trees elsewhere (Kim, Miller, and Nowak Citation2015). However, there is not yet a market ready to support the economic valuation of ecosystem services. Researchers recommend urban biodiversity studies to understand the relationship between ecosystem services and biodiversity in cities, but these can be very expensive to conduct (Kremer et al. Citation2016). Empirical studies, data collection, and monitoring would require heavy investment from the federal and local governments – and citizen science may be a way to do this (Kremer et al. Citation2016).
Who finances GI is another challenge. One of the interviewed resilience managers reported that team(s) are also thinking about GI, but the key problem is how do we fund that? There isn’t a pot of money to invest in GI. We found that combinations of funding sources have resulted in successful cases. In China, the Sponge City Pilot Programme was possible because the central government allocated funds that amounted to 400–600 million Yuan (about $63–94.6 million USD) for three consecutive years, while cities raised funds through public-private partnerships and other mechanisms (Li et al. Citation2017). Likewise, combined sources of private and public funding for GI projects have been available for coastal cities in the United States after disasters, as the case of Sandy Supplemental’s competition Rebuild by Design 2013 illustrates (Sutton-Grier, Wowk, and Bamford Citation2015). Similarly, international funding organizations are also investing in GI at the city scale in collaboration with state governments. In India, the cost of the “green and smart” new City of Amaravati is estimated to be $715 million USD, and is feasible through a variety of funding sources that include the World Bank, the Asian Infrastructure Investment Bank, and the Government of Andhra Pradesh.
Because GI is more effective when it is widely implemented – on public and private land – people’s willingness to accept and pay for GI is crucial. People are used to governments paying for stormwater management. However, GI projects shift the responsibility from the local government to property owners – something many people are not willing to accept (Parr et al. Citation2016). Without a clear explanation of the underlying rationale, such charges will look like a new tax and – worse still – a new tax for benefits that are hard to quantify. In addition, maintenance responsibilities and their associated costs, which go way beyond the completion of the project, further deters residents from investing in this technology (Furlong, Phelan, and Dodson Citation2018; Hoang and Fenner Citation2016). However, the benefits of parks are better understood – having access to green space is something people might be willing to pay a premium for. In a study in the United Kingdom, Wild, Henneberry, and Gill (Citation2017) found that people are willing to pay higher rent or take out a bigger mortgage for dwelling units with access to green space. Therefore, funding for maintenance of GI may be possible by drawing resources from property taxes.
Even if the funding for maintenance challenge is addressed, there is still a need to find the responsible party and this may require extra funding. In a study in Syracuse, New York, Baptiste, Foley, and Smardon (Citation2015) found that aesthetics, efficacy and cost are key factors that influence the willingness of people to adopt GI. The authors recommend targeting initiatives at low income communities who are interested in improving the aesthetics of their neighbourhood. Once the responsibility is determined, there is still a need to build capacity, which requires additional funding. A case in Africa shows that there is a need to invest in capacity building for stakeholders to ensure continuity after project completion (Lindley et al. Citation2018).
2.5. Challenge #5: Innovation
Resilience can be strengthened by harnessing innovation in infrastructure (ULI Citation2018). Because GI is a relatively new concept in terms of its relationship to urban resilience, it is necessary to find innovative mechanisms that combine grey, blue, and green infrastructure to provide a wide array of ecosystem services to urban residents. In a study on coastal communities in the United States, Sutton-Grier, Wowk, and Bamford (Citation2015) found critical opportunities for innovation in hybrid approaches. For example, the “living shorelines” – or restored ecosystems (green) protected from erosion by built infrastructure (grey) – have been adopted and even regulated in several states.
Learning from pilot projects is essential for the successful implementation of GI at a larger scale. The Sponge City case from China shows that starting with pilot projects while incorporating learning can expedite GI implementation through carefully planned milestones. The initial stage involved 16 cities in China and was set up as a 3-year learning programme to be applied on pilot districts (Li et al. Citation2018). In 2016, a second batch of 14 cities joined the pilot programme, thus propelling the Chinese urban design and management community up the learning curve. In 2017, the central government issued a new target, namely that by 2030 all big cities in China should be sponge cities. In 2018, a national evaluation was carried out to assess the performance of the first 16 pilots. By 2020, the government wants 20% of the built area of each sponge city pilot to be capturing and reusing at least 70% of stormwater runoff. By 2030, 80% of each pilot city is supposed to meet this requirement.
From the practitioner’s perspective, however, we found that learning may be hindered by staff turnover. A resilience manager reported changes and staff turnover creates problems with historical records of past experiences. Therefore, it is critical to ensure that lessons from pilot projects are well documented and that these are periodically embedded as part of a standardized design process.
It is through innovation that cities can learn from each other, reduce risk, and identify future opportunities (Li et al. Citation2017). Boston, for example, adopted the “Designing with Water” effort based on the Dutch model “Living with Water” that envisions dual purpose infrastructure – one that makes room for floods within the city in times of need and provides other benefits when flooding is not an issue (Sutton-Grier, Wowk, and Bamford Citation2015).
The City of Lima, Peru provides an interesting case study for innovation. As part of the Future Megacities research program and the LiWa project, researchers developed the Lima Ecological Infrastructure Strategy (LEIS) (Eisenberg et al. Citation2014). LEIS is a comprehensive planning and design approach for GI that integrates urban ecosystems, rivers, valleys, mountains, wetlands and the coastline. LEIS is also a participatory planning tool, which has strengthened relationships and collaboration between local stakeholders, academics and authorities. It integrates urban water management and wastewater treatment into the design of open spaces. This way, parks can serve multiple functions, including treating polluted water (through constructed wetlands) and using it for irrigation of green areas, while providing recreation opportunities for the residents. The children’s park located in La Florida II, Chuquintanta serves as a demonstration project (Eisenberg et al. Citation2014).
A close collaboration between planners, developers, politicians and scientists is needed for the successful implementation of GI. It is by working together that they can engage stakeholders, empower individuals, and produce knowledge that can result in the wide implementation of GI (Tayouga and Gagné Citation2016). The City of LA provides an example of voluntary participation to implement GI. In 1997–1998, severe flooding in LA highlighted the need to change the existing stormwater infrastructure system (LA Times Citation2010). Therefore, the city launched the “Green Alley Program” to mitigate flooding through GI implementation. But greening just a single street such as Elmer Avenue in the North Hollywood district was a huge challenge. It required the coordinated effort of multiple levels of government to implement GI uniformly in the street that involved only 24 residents, three non-profit organizations, six municipal and city agencies, one state agency, and one federal bureau (LA Times Citation2010). Financial resources and engagement activities convinced property owners to donate part of their front yards, and in return they gained curved sidewalks with rain gardens, along with solar street lamps and permeable driveways (LA Times Citation2010). Not only do the enhancements reduce flooding, but the street is now greener, cooler, and prettier.
It is also important to acknowledge that urban ecosystem services do not happen in isolation and that they are related to the hinterlands. Therefore, GI in cities should be considered as a component of a broader network of green space required for both ecological and social systems, as the case of Biesbosch in the Netherlands illustrates (Gersonius et al. Citation2016). During our interviews, one resilience manager reported that it is necessary to operate across the whole spectrum, not only in the built environment – it’s a continuum. Managing and regulating GI can be part of transportation networks and commodity chains, where the exchange of resources happens at larger scales (Kremer et al. Citation2016).
3. Discussion and conclusion
In this paper, we have articulated five major challenges for the effective implementation of GI – design standards, regulatory pathways, socio-economic trends, financeability, and innovation. We examined multiple efforts around the world that seek to address these challenges and help inform next steps. In terms of design standards, we argue for the need for a standardized design process for hybrid infrastructure that aligns with the regulatory framework and includes a maintenance commitment. GI design must consider the multi-functionality aspect that includes social uses (recreation) and ecological uses (habitat and landscape connectivity). For a successful design, the input of users and specialists must be considered. We find that some countries have addressed this challenge in the form of a certification system (as is the case in the United Kingdom and India) or as a government effort to improve practices (in the United States). Certification systems that periodically integrate learning can help practitioners enhance urban resilience.
In terms of the regulatory challenge, we find that cities need regulatory pathways in place that capture GI’s multidimensional benefits. Integrating different levels of government is problematic; therefore, we recommend creating a new spatially-defined regulatory body that overrides other branches of the complex regulatory apparatus. Stakeholder engagement and afforestation initiatives can help to implement GI in cases where regulatory pathways are not viable. Certification programmes developed by the local authorities can improve performance at different scales. Finally, at the very least, urban planning policies must preserve green space from development to allow some ecological processes to occur.
Regarding the socioeconomic challenge, we see equity issues involved around GI, where low income communities are disproportionately affected, particularly in the Global South that faces severe challenges. We find an increasing awareness on the need to address equity and most resilience initiatives have an equity component. However, gentrification, a time lag between installing grey infrastructure and shifting to GI, and lack of public participation still perpetuate inequities.
For the financeability challenge, the cost of no action makes a convincing argument for investing in GI and other resilience initiatives. GI is cheaper than grey infrastructure and economic instruments are useful for the implementation of GI. However, it is unclear who pays for this investment, who maintains GI, and how to quantify all GI benefits. Drawing from property taxes for maintenance may be accepted by the public who live close to green space.
Finally, we find that GI provides an opportunity for innovation, where grey, blue, and green infrastructure can be combined to provide multiple ecosystem services with dual purposes, depending on the need. Pilot projects with established milestones show promise in expediting learning and implementation at the national level. Stakeholder engagement and close collaboration between different groups can result in the wide implementation of GI with public support and agreed maintenance commitment.
There are two underlying currents that run across all of the challenges and that are apparent from the many cases examined here. The first is the role of political will as an underlying factor for successful implementation of GI. Rather than including political will as a standalone challenge, we concur with the mainstream literature on Environmental Politics, including scholarship on the politics of climate change and sustainable development that demonstrate political will as a distal pre-condition for tackling all the five challenges in a coherent and holistic way (e.g., Carter Citation2018; Bauer and Steurer Citation2014; Oulahen et al. Citation2017). Political will is, for example, instrumental for setting up new regulations and it determines the overall direction for more technical discussions about new standards. Similarly, a political commitment to invest (or not) in GI can be an enabler (and, conversely, a barrier in case there is a lack of commitment) to overcoming the financial challenge and support innovation; as well as to redistributing the socio-economic benefits of GI to the wider society.
The second underlying current we observe is the role of stakeholder engagement in GI implementation. We argue that a people-centred approach is needed for the successful implementation of GI, which aligns with previous studies (Petts Citation2008; Webler and Tuler Citation2006). Community engagement is not only needed to bring the community on board with the project, but also to harness funding opportunities from a diversity of funders, as the case of LA illustrates (Sadeghi et al. Citation2016). In addition, inclusive processes where stakeholders at both the organization and the community levels are engaged is crucial for the successful implementation of GI and the subsequent functioning of the system (Campbell, Svendsen, and Roman Citation2016). The public can also be involved in the monitoring through citizen science, which can improve response to crises (ULI Citation2018) and can lead to innovation.
Although we have presented them as individual challenges, we argue that challenges need to be considered in an integrative manner. For example, design standards have to align with the local policy context, as the case of Building with Nature in the United Kingdom demonstrates (Sinnett et al. Citation2018). Regulations are more stringent when design parameters have not been institutionalized, which can lead to increased costs. In the case of constructed wetlands, design standards should be developed by the authorities that evaluate water quality compliance to ensure standards address operational concerns and reduce the likelihood of paying fines (Levy et al. Citation2014).
The interdependence between the challenges also relies on the economic assessment of GI. While GI projects may be cheaper than installing grey infrastructure, there are many factors that may reduce the economic benefit, including regulations, the difficulty of quantifying ecosystem services, the challenge to incorporating the long-term savings, the added and extended cost of maintenance that may affect low-income families, capacity building, and the people’s willingness to adopt GI. In contrast, the economic value of GI may increase if we consider the cost of no action and the co-benefits of GI that can improve the quality of life of the residents (ULI Citation2018).
Not only do challenges have to be addressed in coordination with each other, but synergies can be found by linking one challenge to another. In cases where regulations do not allow the mandate of GI, economic incentives can be used to promote its adoption. As the LA case study shows, the effective collaboration of funding organizations, as well as a priori community engagement, are key factors to channel funds to local residents and this can result in the wide implementation of GI (Sadeghi et al. Citation2016; TPL and LCI Citation2015).
Ultimately, because of the necessary integration of the five challenges, the implementation of GI is context-specific. Each city faces unique challenges in terms of their regulations, their financial capacity, the type of stakeholders, level of development, land availability, climatic and soil conditions, and their potential for innovation. Therefore, context-specific solutions will likely emerge in cities throughout the world, with appropriate technologies. Data sharing and collaboration between cities can facilitate learning and expedite successful implementation. Equity issues should be at the centre of every effort, so a people-centred approach that considers the input of all stakeholders throughout the process must be adopted (Sinnett et al. Citation2017). Finally, GI projects at the neighbourhood scale should be considered part of a larger network of green space and transportation systems, at the watershed scale (following the hydrological unit), with an appropriate governance paradigm (Dhakal and Chevalier Citation2016).
We acknowledge some limitations of this study that include lack of case studies in certain regions of the world (Central America and the Caribbean, New Zealand, Pacific Islands, Central and Southeast Asia), which would provide additional insights. In addition, we recognize that this study can be considered a snapshot in time, so we recommend periodic global reviews to identify trends and potential innovations. Future research is needed to provide data on the multifunctional performance of GI that can inform design standards, and citizen science may be the way to do this more efficiently (Kremer et al. Citation2016), while big data can expedite the sharing and learning process. Research that captures lessons from participatory projects can help to expand networks and empower other communities to follow. Finally, research that explores intra-agency collaborations and innovation in funding for GI can help expedite GI implementation, enhance urban resilience, and strengthen communities. Only then can we better understand the true potential for GI approaches to make our cities and their inhabitants safer and more secure.
Disclosure statement
No potential conflict of interest was reported by the authors.
References
- 100 Resilient Cities. 2018. Accessed May 10 2018. http://www.100resilientcities.org/.
- 100 Resilient Cities and Bristol City Council. 2016. “Bristol Resilience Strategy” Accessed May 14, 2018. http://www.100resilientcities.org/wp-content/uploads/2017/07/Bristol_Strategy_PDF.compressed.pdf.
- 100 Resilient Cities and Ciudad de Mexico. 2016. “CDMX Resilience Strategy: Adaptive, Inclusive and Equitable Transformation” Accessed May 14, 2018. http://www.100resilientcities.org/strategies/mexico-city/.
- Ahern, J. 2011. “From Fail-Safe to Safe-to-Fail: Sustainability and Resilience in the New Urban World.” Landscape and Urban Planning 100 (4): 341–343. doi:10.1016/j.landurbplan.2011.02.021.
- Albers, R. A. W., P. R. Bosch, B. Blocken, A. A. J. F. van den Dobbelsteen, L. W. A. van Hove, T. J. M. Spit, F. van de Ven, T. van Hooff, and V. Rovers. 2015. “Overview of Challenges and Achievements in the Climate Adaptation of Cities and in the Climate Proof Cities Program.” Building and Environment 83: 1–10. doi:10.1016/j.buildenv.2014.09.006.
- Ambrey, C. L., and T. J. Shahni. 2017. “Greenspace and Wellbeing in Tehran: A Relationship Conditional on a Neighbourhood’s Crime Rate?” Urban Forestry & Urban Greening 27: 155–161. doi:10.1016/j.ufug.2017.08.003.
- Arshad, H. S. H., and J. K. Routray. 2018. “From Socioeconomic Disparity to Environmental Injustice: The Relationship Between Housing Unit Density and Community Green Space in a Medium City in Pakistan.” Local Environment 23 (5): 536–548. doi:10.1080/13549839.2018.1442424.
- Baptiste, A. K., C. Foley, and R. Smardon. 2015. “Understanding Urban Neighborhood Differences in Willingness to Implement Green Infrastructure Measures: A Case Study of Syracuse, NY.” Landscape and Urban Planning 136: 1–12. doi:10.1016/j.landurbplan.2014.11.012.
- Bauer, A., and R. Steurer. 2014. “Innovation in Climate Adaptation Policy: Are Regional Partnerships Catalysts or Talking Shops?” Environmental Politics 23 (5): 818–838. doi:10.1080/09644016.2014.924196.
- Bhaskar, A. S., D. M. Hogan, and S. A. Archfield. 2016. “Urban Base Flow with Low Impact Development: Urban Base Flow with Low Impact Development.” Hydrological Processes 30 (18): 3156–3171. doi:10.1002/hyp.10808.
- Campbell, L. K., E. S. Svendsen, and L. A. Roman. 2016. “Knowledge Co-Production at the Research–Practice Interface: Embedded Case Studies from Urban Forestry.” Environmental Management 57 (6): 1262–1280. doi:10.1007/s00267-016-0680-8.
- Carlet, F. 2015. “Understanding Attitudes Toward Adoption of Green Infrastructure: A Case Study of US Municipal Officials.” Environmental Science and Policy 51: 65–76. doi:10.1016/j.envsci.2015.03.007.
- Carter, N. 2018. The Politics of the Environment: Ideas, Activism, Policy. Cambridge, UK: Cambridge University Press.
- Castelli, G., C. Foderi, B. Guzman, L. Ossoli, Y. Kempff, E. Bresci, and F. Salbitano. 2017. “Planting Waterscapes: Green Infrastructures, Landscape and Hydrological Modeling for the Future of Santa Cruz de la Sierra, Bolivia.” Forests 8 (11): 437. doi:10.3390/f8110437.
- Dai, L., H. F. M. W. van Rijswick, P. P. J. Driessen, and A. M. Keessen. 2018. “Governance of the Sponge City Programme in China with Wuhan as a Case Study.” International Journal of Water Resources Development 34 (4): 578–596. doi:10.1080/07900627.2017.1373637.
- Derkzen, M. L., A. J. A. van Teeffelen, and P. H. Verburg. 2015. “REVIEW: Quantifying Urban Ecosystem Services Based on High-Resolution Data of Urban Green Space: An Assessment for Rotterdam, The Netherlands.” Journal of Applied Ecology 52 (4): 1020–1032. doi:10.1111/1365-2664.12469.
- Derkzen, M. L., A. J. A. van Teeffelen, and P. H. Verburg. 2017. “Green Infrastructure for Urban Climate Adaptation: How Do Residents’ Views on Climate Impacts and Green Infrastructure Shape Adaptation Preferences?” Landscape and Urban Planning 157: 106–130. doi:10.1016/j.landurbplan.2016.05.027.
- Dhakal, K. P., and L. R. Chevalier. 2016. “Urban Stormwater Governance: The Need for a Paradigm Shift.” Environmental Management 57 (5): 1112–1124. doi:10.1007/s00267-016-0667-5.
- Eisenberg, B., E. Nemcova, R. Poblet, and A. Stokman. 2014. Lima Ecological Infrastructure Strategy: Integrated Urban Planning and Design Tools for a Water-Scarce City (Future Megacities). Stuttgart, Germany: Institute of Landscape Planning and Ecology. University of Stuttgard. Accessed May 10, 2018. https://issuu.com/ilpe/docs/lima_ecological_infrastructure_stra_9c435aba38df2f
- Ekness, P., and T. O. Randhir. 2015. “Effect of Climate and Land Cover Changes on Watershed Runoff: A Multivariate Assessment for Storm Water Management: Determinants of Watershed Runoff.” Journal of Geophysical Research: Biogeosciences 120 (9): 1785–1796. doi:10.1002/2015JG002981.
- Ellis, J. B., and L. Lundy. 2016. “Implementing Sustainable Drainage Systems for Urban Surface Water Management Within the Regulatory Framework in England and Wales.” Journal of Environmental Management 183: 630–636. doi:10.1016/j.jenvman.2016.09.022.
- Escobedo, F. J., N. Clerici, C. L. Staudhammer, and G. T. Corzo. 2015. “Socio-Ecological Dynamics and Inequality in Bogotá, Colombia’s Public Urban Forests and Their Ecosystem Services.” Urban Forestry and Urban Greening 14 (4): 1040–1053. doi:10.1016/j.ufug.2015.09.011.
- Fernández, I. C., and J. Wu. 2018. “A GIS-Based Framework to Identify Priority Areas for Urban Environmental Inequity Mitigation and Its Application in Santiago de Chile.” Applied Geography 94: 213–222. doi:10.1016/j.apgeog.2018.03.019.
- Fernández-Alvarez, R. 2017. “Inequitable Distribution of Green Public Space in the Mexico City: An Environmental Injustice Case.” Economía Sociedad y Territorio 18 (54): 399. doi:10.22136/est002017697.
- Furlong, C., K. Phelan, and J. Dodson. 2018. “The Role of Water Utilities in Urban Greening: A Case Study of Melbourne, Australia.” Utilities Policy 53: 25–31. doi:10.1016/j.jup.2018.06.005.
- Gersonius, B., A. van Buuren, M. Zethof, and E. Kelder. 2016. “Resilient Flood Risk Strategies: Institutional Preconditions for Implementation.” Ecology and Society 21 (4): 28. https://doi.org/10.5751/ES-08752-210428
- Ghadei, M. 2018. “Amaravati: A City Reborn, Journey Towards a World-Class Smart City.” In Towards Sustainable Cities in Asia and the Middle East, edited by J. Calautit, F. Rodrigues, H. Chaudhry, and H. Altan, 15–29. New York: Springer International Publishing.
- Giupponi, C., and A. Sgobbi. 2008. “Models and Decisions Support Systems for Participatory Decision Making in Integrated Water Resource Management.” In Coping with Water Deficiency, 165–118. Dordrecht: Springer.
- Gould, K. A., and T. L. Lewis. 2016. Green Gentrification: Urban Sustainability and the Struggle for Environmental Justice. New York: Routledge
- Gulsrud, N. M., K. Hertzog, and I. Shears. 2018. “Innovative Urban Forestry Governance in Melbourne?: Investigating ‘Green Placemaking’ as a Nature-Based Solution.” Environmental Research 161: 158–167. doi:10.1016/j.envres.2017.11.005.
- Hoang, L., and R. A. Fenner. 2016. “System Interactions of Stormwater Management Using Sustainable Urban Drainage Systems and Green Infrastructure.” Urban Water Journal 13 (7): 739–758. doi:10.1080/1573062X.2015.1036083.
- Hordyk, S. R., J. Hanley, and É. Richard. 2015. “Nature Is There; Its Free”: Urban Greenspace and the Social Determinants of Health of Immigrant Families.” Health and Place 34: 74–82. doi:10.1016/j.healthplace.2015.03.016.
- Howard, E., and F. J. Osborn. 1965. Garden Cities of To-Morrow. Cambridge, MA: MIT Press.
- IPCC 2013. “Summary for Policymakers.” In Climate Change 2013: The Physical Science Basis. Contribution of Working Group I to the Fifth Assessment Report of the Intergovernmental Panel on Climate Change, edited by T. F. Stocker, 3–32. Cambridge: Cambridge University Press.
- Jeong, H., O. A. Broesicke, B. Drew, D. Li, and J. C. Crittenden. 2016. “Life Cycle Assessment of Low Impact Development Technologies Combined with Conventional Centralized Water Systems for the City of Atlanta, Georgia.” Frontiers of Environmental Science and Engineering 10 (6): 01. doi:10.1007/s11783-016-0851-0.
- Jiang, Y., C. Zevenbergen, and D. Fu. 2017. “Understanding the Challenges for the Governance of China’s ‘Sponge Cities’ Initiative to Sustainably Manage Urban Stormwater and Flooding.” Natural Hazards 89 (1): 521–529. doi:10.1007/s11069-017-2977-1.
- Kim, G., P. A. Miller, and D. J. Nowak. 2015. “Assessing Urban Vacant Land Ecosystem Services: Urban Vacant Land as Green Infrastructure in the City of Roanoke, Virginia.” Urban Forestry and Urban Greening 14 (3): 519–526. doi:10.1016/j.ufug.2015.05.003.
- Klenzendorf, B., M. Kelly, A. Poresky, and M. Christman. 2016. Evaluation of a Hybrid Green/Grey Approach to Address Neighborhood Flooding and Other Watershed Issues: Closure from a Texas Case Study. In Water, Wastewater, and Stormwater and Urban Watershed Symposium, edited by C. S. Pathak and Reinhart, 77–86. West Palm Beach, FL: American Society of Civil Engineers.
- Kremer, P., Z. Hamstead, D. Haase, T. McPhearson, N. Frantzeskaki, E. Andersson, N. Kabisch, et al. 2016. “Key Insights for the Future of Urban Ecosystem Services Research.” Ecology and Society 21 (2): 29. doi:10.5751/ES-08445-210229.
- Lara-Valencia, F., Garcia-Perez H. 2015. “Space for Equity: Socioeconomic Variations in the Provision of Public Parks in Hermosillo, Mexico.” Local Environment 20 (3): 350–368. doi:10.1080/13549839.2013.857647.
- Levy, Z., R. Smardon, J. Bays, and D. Meyer. 2014. “A Point Source of a Different Color: Identifying a Gap in United States Regulatory Policy for ‘Green’ CSO Treatment Using Constructed Wetlands.” Sustainability 6 (5): 2392–2412. doi:10.3390/su6052392.
- Li, H., L. Ding, M. Ren, C. Li, and H. Wang. 2017. “Sponge City Construction in China: A Survey of the Challenges and Opportunities.” Water 9 (9): 594. doi:10.3390/w9090594.
- Li, Z., M. Dong, T. Wong, J. Wang, A. J. Kumar, and R. P. Singh. 2018. “Objectives and Indexes for Implementation of Sponge Cities: A Case Study of Changzhou City, China.” Water 10 (5): 623. doi:10.3390/w10050623.
- Lindley, S., S. Pauleit, K. Yeshitela, S. Cilliers, and C. Shackleton. 2018. “Rethinking Urban Green Infrastructure and Ecosystem Services from the Perspective of Sub-Saharan African Cities.” Landscape and Urban Planning 180: 328–338. doi:10.1016/j.landurbplan.2018.08.016.
- Liu, Y., L. O. Theller, B. C. Pijanowski, and B. A. Engel. 2016. “Optimal Selection and Placement of Green Infrastructure to Reduce Impacts of Land Use Change and Climate Change on Hydrology and Water Quality: An Application to the Trail Creek Watershed, Indiana.” Science of the Total Environment 553: 149–163. doi:10.1016/j.scitotenv.2016.02.116.
- Los Angeles Times (LA Times). 2010. “The Dry Garden: Elmer Avenue Becomes Green Street, a Water-Wise and Solar-Lighted Community Effort.” LA at Home, Los Angeles Times. Accessed April 21, 2017. http://latimesblogs.latimes.com/home_blog/2010/07/elmer-avenue-sun-valley.html
- Martin-Mikle, C. J., K. M. de Beurs, J. P. Julian, and P. M. Mayer. 2015. “Identifying Priority Sites for Low Impact Development (LID) in a Mixed-Use Watershed.” Landscape and Urban Planning 140: 29–41. doi:10.1016/j.landurbplan.2015.04.002.
- McRae, A. M. 2016. “Case Study: A Conservative Approach to Green Roof Benefit Quantification and Valuation for Public Buildings.” The Engineering Economist 61 (3): 190–206. doi:10.1080/0013791X.2016.1186255.
- Million Trees NYC. 2018. Accessed March 30, 2018. http://www.milliontreesnyc.org/html/about/about.shtml.
- National Research Council. 2009. Urban Stormwater Management in the United States. Washington, DC: National Academies Press. https://doi.org/10.17226/12465
- Nero, B. F. 2017. “Urban Green Space Dynamics and Socio-Environmental Inequity: Multi-Resolution and Spatiotemporal Data Analysis of Kumasi, Ghana.” International Journal of Remote Sensing 38 (23): 6993–7020. doi:10.1080/01431161.2017.1370152.
- Ntombela, C., N. Funke, R. Meissner, M. Steyn, and W. Masangane. 2016. “A Critical Look at South Africa’s Green Drop Programme.” Water SA 42 (4): 703. doi:10.4314/wsa.v42i4.21.
- Oulahen, S. E., Chang, J. Z. K. Yip, T. Conger, M. and Marteleira C. Carter. 2017. “Contextualizing Institutional Factors in an Indicator-Based Analysis of Hazard Vulnerability for Coastal Communities.” Journal of Environmental Planning and Management 61 (14): 2491–2511.
- Parr, T. B., N. J. Smucker, C. N. Bentsen, and M. W. Neale. 2016. “Potential Roles of Past, Present, and Future Urbanization Characteristics in Producing Varied Stream Responses.” Freshwater Science 35 (1): 436–443. doi:10.1086/685030.
- Pennino, M. J., R. I. McDonald, and P. R. Jaffe. 2016. “Watershed-Scale Impacts of Stormwater Green Infrastructure on Hydrology, Nutrient Fluxes, and Combined Sewer Overflows in the Mid-Atlantic Region.” Science of the Total Environment 565: 1044–1053. doi:10.1016/j.scitotenv.2016.05.101.
- Petroski, H. 2016. The Road Taken: The History and Future of America’s Infrastructure. New York, NY: Bloomsbury USA.
- Petts, J. 2008. “Public Engagement to Build Trust: False Hopes?” Journal of Risk Research 11 (6): 821–835. doi:10.1080/13669870701715592.
- Pierre, S., P. M. Groffman, M. E. Killilea, and E. E. Oldfield. 2016. “Soil Microbial Nitrogen Cycling and Nitrous Oxide Emissions from Urban Afforestation in the New York City Afforestation Project.” Urban Forestry and Urban Greening 15: 149–154. doi:10.1016/j.ufug.2015.11.006.
- Pitt, M. 2008. “The Pitt Review Learning Lessons from the 2007 Floods.” Accessed February 20, 2018. http://webarchive.nationalarchives.gov.uk/20100702215619/ http://archive.cabinetoffice.gov.uk/pittreview/thepittreview/final_report.html.
- Portman, M. 2018. “Policy Options for Coastal Protection: Integrating Inland Water Management with Coastal Management for Greater Community Resilience.” SSRN Electronic Journal 144 (4): 05018005. doi:10.2139/ssrn.3046193.
- Poumadère, M., C. Mays, S. Le Mer, and R. Blong. 2005. “The 2003 Heat Wave in France: Dangerous Climate Change Here and Now.” Risk Analysis 25 (6): 1483–1494. doi:10.1111/j.1539-6924.2005.00694.x.
- Rohilla, S., S. Jainer, and M. Matto. 2017. Green Infrastructure: A Practitioner’s Guide. Delhi, India: Centre for Science and Environment.
- Rostad, N., R. Foti, and F. A. Montalto. 2016. “Harvesting Rooftop Runoff to Flush Toilets: Drawing Conclusions from Four Major US cities.” Resources, Conservation and Recycling 108: 97–106. doi:10.1016/j.resconrec.2016.01.009.
- Sadeghi, M., S. Kharaghani, W. Tam, N. Gaerlan, and H. Loaiciga. 2016. “Avalon Green Alley Network: Low Impact Development (LID) Demonstration Project in Los Angeles.” Paper presented at the World Environmental and Water Resources Congress 2016. West Palm Beach Florida, US, May 22–26.
- Saleh, F., and M. P. Weinstein. 2016. “The Role of Nature-Based Infrastructure (NBI) in Coastal Resiliency Planning: A Literature Review.” Journal of Environmental Management 183: 1088–1098. doi:10.1016/j.jenvman.2016.09.077.
- Safransky, S. 2014. “Greening the Urban Frontier: Race, Property, and Resettlement in Detroit.” Geoforum 56: 237–248. doi:10.1016/j.geoforum.2014.06.003.
- Scharenbroch, B. C., J. Morgenroth, and B. Maule. 2016. “Tree Species Suitability to Bioswales and Impact on the Urban Water Budget.” Journal of Environment Quality 45 (1): 199–206. doi:10.2134/jeq2015.01.0060.
- Sinnett, D., G. Jerome, S. Burgess, N. Smith, and R. Mortlock. 2017. “Building with Nature: A New Benchmark for Green Infrastructure.” Town and Country Planning 86 (10): 427–431.
- Sinnett, D., G. Jerome, N. Smith, S. Burgess, and R. Mortlock. 2018. “Raising the Standard: Developing a Benchmark for Green Infrastructure.” International Journal of Sustainable Development and Planning 13 (02): 226–236. doi:10.2495/SDP-V13-N2-226-236.
- Shanahan, D. F., B. B. Lin, R. Bush, K. J. Gaston, J. H. Dean, E. Barber, and R. A. Fuller. 2015. “Toward Improved Public Health Outcomes from Urban Nature.” American Journal of Public Health 105 (3): 470–477.
- Smiley, K. T., T. Sharma, A. Steinberg, S. Hodges-Copple, E. Jacobson, and L. Matveeva. 2016. “More Inclusive Parks Planning: Park Quality and Preferences for Park Access and Amenities.” Environmental Justice 9 (1): 1–7. doi:10.1089/env.2015.0030.
- Smith, N. 1984. Uneven Development: Nature, Capital, and the Production of Space (Third). Athens: The University of Georgia Press. doi:10.1086/ahr/90.2.480.
- Staddon, C. 2010. Managing Europe’s Water Resources: 21st Century Challenges. Farnham, UK: Ashgate Press.
- Staddon, C., Vito, D. L. Zuniga-Teran, A. Schoeman, Y. Hart, A., and Booth G. 2017. “Contributions of Green Infrastructure to Enhancing Urban Resilience.” Accessed May 10, 2018. http://resilienceshift.org/wp-content/uploads/2017/10/043_Contributions-of-Green-Infrastructure-to-Enhancing-Urban-Resilience.pdf.
- Subramanian, R. 2016. “Rained Out: Problems and Solutions for Managing Urban Stormwater Runoff.” Ecology LQ 43: 421–511.
- Sutton-Grier, A. E., K. Wowk, and H. Bamford. 2015. “Future of Our Coasts: The Potential for Natural and Hybrid Infrastructure to Enhance the Resilience of Our Coastal Communities, Economies and Ecosystems.” Environmental Science and Policy 51: 137–148. doi:10.1016/j.envsci.2015.04.006.
- Sweetwater Wetlands. 2018. “Official Website of the City of Tucson.” Accessed April 1, 2018. https://www.tucsonaz.gov/water/sweetwater-wetlands.
- Tayouga, S., and S. Gagné. 2016. “The Socio-Ecological Factors That Influence the Adoption of Green Infrastructure.” Sustainability 8 (12): 1277. doi:10.3390/su8121277.
- The Trust for Public Land, and UCLA Luskin Center for Innovation (TPL and LCI). 2015. The Avalon Green Alley Network Demonstration Project: Lessons Learned from Previous Projects for Green Alley Development in Los Angeles and Beyond. Los Angeles, CA: UCLA Luskin Center for Innovation. https://www.tpl.org/sites/default/files/files_upload/ca-green-alley-avalon-green-alleys-demo-project.pdf
- Urban Land Institute (ULI). 2018. The Ten Principles for Building Resilience. Washington, DC: Urban Land Institute. https://americas.uli.org/wp-content/uploads/sites/125/ULI-Documents/10P_BuildingResilience.pdf
- US Environmental Protection Agency (USEPA). 2018a. “Green Infrastructure Modeling Toolkit.” Accessed January 11, 2018. https://www.epa.gov/water-research/green-infrastructure-modeling-toolkit.
- US Environmental Protection Agency (USEPA). 2018b. “National Stormwater Calculator.” Accessed January 11, 2018. https://www.epa.gov/water-research/national-stormwater-calculator.
- Vásquez, A., C. Devoto, E. Giannotti, and P. Velásquez. 2016. “Green Infrastructure Systems Facing Fragmented Cities in Latin America: Case of Santiago, Chile.” Procedia Engineering 161: 1410–1416. doi:10.1016/j.proeng.2016.08.602.
- Vogel, J. R., T. L. Moore, R. R. Coffman, S. N. Rodie, S. L. Hutchinson, K. R. McDonough, A. J. McLemore, and J. T. McMaine. 2015. “Critical Review of Technical Questions Facing Low Impact Development and Green Infrastructure: A Perspective from the Great Plains.” Water Environment Research 87 (9): 849–862. doi:10.2175/106143015X14362865226392.
- Voskamp, I. M., and F. H. M. Van de Ven. 2015. “Planning Support System for Climate Adaptation: Composing Effective Sets of Blue-Green Measures to Reduce Urban Vulnerability to Extreme Weather Events.” Building and Environment 83: 159–167. doi:10.1016/j.buildenv.2014.07.018.
- Wang, R., M. J. Eckelman, and J. B. Zimmerman. 2013. “Consequential Environmental and Economic Life Cycle Assessment of Green and Gray Stormwater Infrastructures for Combined Sewer Systems.” Environmental Science and Technology 47 (19): 11189–11198. doi:10.1021/es4026547.
- Water Research Commission 2014. Achieve Green Drop Status: Krueger National Park Chooses Green Technology to Achieve a Green Drop (Lesson Series). Republic of South Africa: Department of Water Affairs. http://www.wrc.org.za/Knowledge%20Hub%20Documents/WINSA/Green%20Drop%20Lesson%20-%20Kruger%20National%20Park.pdf
- Webler, T., and S. Tuler. 2006. “Four Perspectives on Public Participation Process in Environmental Assessment and Decision Making: Combined Results from 10 Case Studies.” Policy Studies Journal 34 (4): 699–722. doi:10.1111/j.1541-0072.2006.00198.x.
- Wei, J., J. Qian, Y. Tao, F. Hu, and W. Ou. 2018. “Evaluating Spatial Priority of Urban Green Infrastructure for Urban Sustainability in Areas of Rapid Urbanization: A Case Study of Pukou in China.” Sustainability. 10 (2): 327. doi:10.3390/su10020327.
- Willemse, L. 2015. Some Perceptions and Preferences of Residents’ Use of Community Neighbourhood Parks in Mitchells Plain. Cape Town: Town and Regional Planning.
- Wild, T. C., J. Henneberry, and L. Gill. 2017. “Comprehending the Multiple ‘Values’ of Green Infrastructure: Valuing Nature-Based Solutions for Urban Water Management from Multiple Perspectives.” Environmental Research 158: 179–187. doi:10.1016/j.envres.2017.05.043.
- Yang, B., S. Li, H. A. Wall, P. Blackmore, and Z. Wang. 2015. “Green Infrastructure Design for Improving Stormwater Quality: Daybreak Community in the United States West.” Landscape Architecture Frontiers 3 (4): 12–22.
- Zuniga-Teran, A. 2015. “From Neighborhoods to Wellbeing and Conservation: Enhancing the Use of Greenspace Through Walkability.” PhD Thesis, University of Arizona.