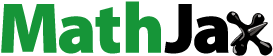
ABSTRACT
Pattern separation is the process that minimises interference between memory representations with similar features and is suggested to be associated with hippocampus-related recollection. We tested this hypothesis using the incidental Mnemonic Similarity Task with old (target), similar (lure), and new (foil) items presented on a recognition test, which is widely used for detecting individual differences in behavioural pattern separation performance. In Experiment 1, participants made old/similar/new decisions and rated decision confidence on a scale ranging from “not at all sure” to “very sure”. In Experiment 2, participants made recognition confidence judgments on a scale ranging between “sure it was new” and “sure it was old”. In Experiment 3, subjects gave old/similar/new decisions and made a secondary Remember/Know/Guess judgment. In Experiment 1, confidence ratings were higher for targets compared to lures when we analysed correct responses (old for targets and similar for lures). Additionally, we found a symmetrical ROC curve and a linear zROC curve for target-lure discrimination in Experiment 2. Finally, we found a bias toward Know responses when we analysed behavioural pattern separation performance (i.e., the rate of similar responses given to the lures). These findings suggest that familiarity, rather than recollection, contributes to behavioural pattern separation performance.
Pattern separation
Episodic memory has been conceptualised as a memory system that allows the conscious retrieval of unique events (Tulving, Citation1972, Citation1985). Recently, it has been suggested that a process, called pattern separation, plays a key role in episodic remembering (Yassa & Stark, Citation2011). Pattern separation refers to the reduction of interference between memory representations with similar features. In other words, it allows the encoding and storage of a set of similar information in the form of unique memory representations (Hunsaker & Kesner, Citation2013).
Importantly, episodic remembering has its own characteristics. Specifically, when event-specific (contextual) details of unique memories are accessed during retrieval, the person has a feeling of the self in the past, a phenomenon termed recollective experience (Brewer, Citation1996; Conway, Citation2005; Tulving, Citation1985). Since pattern separation is suggested to be a hallmark feature of episodic memory, then one might expect that pattern separation is accompanied by recollection. This is not the case, however. In fact, pattern separation is associated with, but not restricted to, recollection (Yassa & Stark, Citation2011; see also Kim & Yassa, Citation2013). First, recollection not always requires pattern separation such as when there is no overlap between the features of two or more memory representations. Second, whereas pattern separation is a neural computational mechanism, recollection is a psychological construct, and we have no reason to assume a specific neural mechanism associated with recollection. Therefore, even if interference resolution is needed, it can occur without the phenomenological experience of recollection.
Nevertheless, confirming the relationship between episodic memory and pattern separation, a long line of research has demonstrated that the hippocampus is essential to both episodic remembering (Tulving, Citation2002; Tulving & Markowitsch, Citation1998) and pattern separation (Yassa & Stark, Citation2011). In the past few years, several computational models have been developed to describe how the hippocampus supports interference resolution between representations with similar features (e.g., McClelland, McNaughton, & O'Reilly, Citation1995; Treves & Rolls, Citation1994; for an overview, see also Levy, Citation1989). There has been a growing body of evidence that specific subregions of the hippocampus, including the dentate gyrus (DG) and the CA3, perform domain-general pattern separation on overlapping sensory inputs enabling the formation of distinct memory representations (Bakker, Kirwan, Miller, & Stark, Citation2008; Kirwan et al., Citation2012; Yassa et al., Citation2011). Since both structural and functional changes affect specific subregions of the hippocampus (including the DG and the CA3) in various psychiatric and neurological disorders (e.g., Kheirbek, Klemenhagen, Sahay, & Hen, Citation2012; Stark, Yassa, Lacy, & Stark, Citation2013) as well as in pathological and normal ageing (e.g., Stark et al., Citation2013; Stark, Yassa, & Stark, Citation2010), the investigation of pattern separation has been of special scientific interest in the past few years to neuroscientists and psychologists as well.
From a behavioural perspective, the correct rejection of an item that is only similar to but not the same as a previously studied (target) item is assumed to be the manifestation of successful interference resolution and has been used as a reliable measure of pattern separation (Bakker et al., Citation2008). Therefore, in humans, pattern separation is usually assessed by recognition memory tasks where subjects are presented with critical lure items that are similar to ones presented at encoding (Segal, Stark, Kattan, Stark, & Yassa, Citation2012; Stark et al., Citation2013; Yassa et al., Citation2011). The Mnemonic Similarity Task (MST), developed by Stark and colleagues (Citation2013), is a frequently used method to assess the behavioural manifestation of putative neural mechanisms enabling pattern separation. In the original paradigm, subjects are typically engaged in an incidental encoding phase while they are presented with photographs of everyday objects. This phase is followed by a recognition test with no delay between encoding and retrieval. On the recognition test, participants see exact repetitions of images that were presented at encoding and completely new items (targets and foils, respectively). Crucially, subjects are also presented with critical lures that are visually similar items to ones that were presented at encoding. Participants have three response options: old, new, and similar, and a reliable index of behavioural pattern separation is the rate of similar responses given to the lures (see also Stark, Kirwan, & Stark, Citation2019).
Familiarity and recollection in recognition memory
As it was described in detail, behavioural pattern separation is typically measured by using some variation of recognition memory tasks. Importantly, one approach to investigate process(es) that contribute(s) to memory performance is the analysis of receiver operating characteristics (ROC) in recognition tasks measuring confidence levels (Egan, Citation1958). In these tasks participants study a list of items, followed by a test where subjects are presented with a mixture of old (studied) and new (unstudied) items. Participants are typically asked to make a recognition confidence judgment on a scale ranging between “sure it was new” and “sure it was old”. The ROC curve is a plot of hits (correct old responses given to the old items) against false alarm rate (incorrect old responses given to the new items).
Early studies showed that the ROC curve tends to be curvilinear and symmetrical indicating that one single process contributes to performance in recognition memory tasks (Murdock & Dufty, Citation1972). The signal detection theory assumes that recognition memory decisions are based on a response criterion. The principal idea is that previously studied old items are more familiar than new items, and recognition memory performance reflects this single process of familiarity-based decision. These single-process models assume that recognition decision depends on the strength of a memory signal, and once the strength exceeds a criterion, individuals accept the item as having been studied (for an overview, see e.g., Wixted, Citation2007).
Other models, on the other hand, state that the ROC curve is asymmetrical when correct recognition memory judgments are based not only on familiarity, but also on recollection. It has been demonstrated that when recollection contributed to performance, the ROC curve was asymmetrical, such as in case of correct source memory judgments (Yonelinas, Citation1999) or when retrieval was accompanied by the feeling of “remembering” (Yonelinas, Citation2001a). The two-criterion model proposes that a familiarity-based correct old decision is made when the level of an old item’s familiarity falls above a criterion value; otherwise, a search process starts that could lead to a recollection-based correct old decision (Atkinson & Juola, Citation1973). Another version of the dual-process models also assumes two independent processes and suggests that while familiarity depends on a response criterion, recollection reflects a threshold process (Yonelinas, Citation1999, Citation2001b, Citation2002). In this model, recollection is suggested to be a categorical process that occurs when the correct recognition of an item is associated with the highest confidence level (“sure it was old”) or does not occur when successful recognition is associated with low or medium confidence level (but see Mickes, Wixted, & Wais, Citation2007; Wixted, Citation2007; Wixted, Mickes, & Squire, Citation2010 for an opposite view).
Other authors formulated a more moderate viewpoint assuming that recollection is associated with high level but not necessarily the highest level of confidence (Gardiner & Java, Citation1990; Tulving, Citation1985). Moreover, it seems that familiarity-based responses are not only given whenever a recognition memory decision is associated with low level of confidence (Gardiner & Java, Citation1990). This finding suggests that the difference between familiarity-based recognition memory judgments and recollection is not limited to confidence levels associated with retrieval (see also Rajaram, Citation1993). Somewhat in line with this viewpoint, the dual-process model also states that sometimes familiarity is also associated with the highest confidence (Yonelinas, Citation2001b).
Another way to investigate processes that contribute to recognition memory is the Remember/Know paradigm. In this task subjects are first asked to make an old/new decision. Additionally, following each old response, participants are required to decide whether they remember the item (together with some aspects of the context of its original presentation) or just know that the stimulus has been presented previously. Originally, Endel Tulving (Citation1985) developed this procedure to separate episodic from semantic memory by assessing the conscious experience (i.e., state of awareness) that accompanies memory retrieval. A long line of research did show a dissociation between Remember and Know responses, i.e., some experimental manipulations affected only Remember and not Know responses and vice versa (e.g., Gardiner & Java, Citation1990; Gardiner & Parkin, Citation1990) indicating that remembering and knowing are indeed functionally independent. Later studies, however, suggest that whereas Remember responses are assumed to reflect recollection, Know judgments are assumed to be associated with familiarity-based recognition (see Jacoby, Yonelinas, & Jennings, Citation1997; but see e.g., Wais, Mickes, & Wixted, Citation2008; Wixted & Mickes, Citation2010).
Regarding the neural background of recognition memory, the traditional idea is that recollection and familiarity do not rely on identical brain regions. While the hippocampus selectively supports recollection, the perirhinal cortex is critical for familiarity-based decisions (Brown & Aggleton, Citation2001; see also e.g., Fortin, Wright, & Eichenbaum, Citation2004). Later studies, however, introduced a more moderate viewpoint suggesting that the hippocampus is more important to recollective remembering, when compared to familiarity-based recognition (Yonelinas, Citation2001b). Moreover, some authors propose that the hippocampus is critical for both recollection and familiarity and that these two processes always contribute to recognition memory decisions (Manns, Hopkins, Reed, Kitchener, & Squire, Citation2003; Squire, Wixted, & Clark, Citation2007; Wais, Wixted, Hopkins, & Squire, Citation2006).
Beside the assumption that behavioural pattern separation is associated with recollection at least to some extent (Yassa & Stark, Citation2011), the empirical investigation of the topic is largely missing. Therefore, we designed a study to examine processes that contribute to pattern separation by measuring decision/recognition confidence levels and by using the Remember/Know paradigm. We used the MST (Stark et al., Citation2013) and we made crucial modifications compared to the original paradigm. In the recognition test phase of Experiment 1, subjects were not only instructed to give old/similar/new responses, but to make decision confidence judgments as well on a scale ranging from “not at all sure” to “very sure”. In Experiment 2, participants were not asked to give old/similar/new responses, but to make recognition confidence judgments on a scale ranging between “sure it was new” and “sure it was old”. In Experiment 3, subjects gave an old/similar/new response (just as in Experiment 1), and following each old/similar decision, they made a secondary Remember/Know/Guess judgment.
The dual-process model of recognition memory allows the separation between processes contributing to recognition memory decisions (for a detailed overview, see Dobbins, Citation2015), therefore, we analysed the data on the basis of the dual-process signal detection (DPSD) model (see Koen, Barrett, Harlow, & Yonelinas, Citation2017). Since this model states that recollection is always associated with the highest level of confidence (Yonelinas, Citation2001a, Citation2001b), we focused on decision confidence frequencies associated with behavioural pattern separation as measured by the rate of similar responses given to the lure items in Experiment 1. Furthermore, for data of Experiment 2, we constructed ROC and z-transformed ROC (zROC) curves by plotting hit rates for the targets against false alarm rate for the lures to investigate whether the shape of the ROC curve is asymmetrical and the zROC is curvilinear that the dual-process model would predict for recollection-based decisions (Yonelinas, Citation2001b). Finally, since Remember responses are suggested to reflect recollection and Know responses are assumed to reflect familiarity (e.g., Jacoby et al., Citation1997), when we analysed the data of Experiment 3, we compared the ratios of Remember, Know, and Guess judgments for successful behavioural pattern separation as measured by similar responses given to the lures.
Experiment 1
Materials and methods
Participants
In all experiments, subjects were undergraduate students and received course credit for participation. They had no history of psychiatric and neurological disorders and had normal (or corrected-to-normal) vision. All participants gave written informed consent. The study was approved by the Hungarian United Ethical Review Committee for Research in Psychology and has been carried out in accordance with the Code of Ethics of the World Medical Association (Declaration of Helsinki) for experiments involving humans.
There were 25 participants in Experiment 1 (4 men; age range: 20–32 years, M = 22.5, SD = 2.6). No subject was excluded from the sample.
Stimuli, experimental design, and procedure
We used a modified version of the MST, which was originally developed by Stark and colleagues (Citation2013) to tax behavioural pattern separation. Stimuli were colour photographs of objects and animals. The task consisted of two phases (with no delay between them): an incidental encoding phase and a recognition test. The experimental procedure is illustrated in .
Figure 1. The procedure of Experiment 1, 2, and 3. Note(s). In all experiments, participants were presented with photographs of everyday objects followed by a surprise recognition test. In Experiment 1, subjects were instructed to make an old/similar/new decision and to judge how confident they were that they had made the right decision (1 = not at all sure, 6 = very sure). In Experiment 2, participants were not asked to make an old/similar/new decision but to make a recognition confidence judgment (1 = sure it was new, 6 = sure it was old). In Experiment 3, subjects gave an old/similar/new response, and following each old/similar response, they made a Remember/Know/Guess judgment. PSI = pre-stimulus interval.
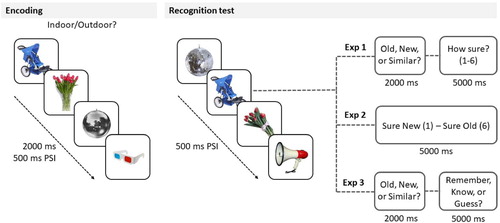
In the encoding phase, subjects were presented with 128 images (for 2000 ms each with a pre-stimulus interval [PSI] of 500 ms). The images were presented in the middle of the computer screen, and participants were instructed to make an indoor/outdoor judgement. Response options (F = indoor, K = outdoor) remained at the top of the computer screen during the whole encoding phase of the task.
Immediately after encoding, subjects participated in a surprise recognition task while they saw 192 images in the middle of the computer screen. Sixty-four images were targets, i.e., exact repetitions to ones that were presented at encoding. Sixty-four images were foils, i.e., new images that were not presented at all at encoding. Crucially, 64 images were visually similar, but not identical lures of the images that were presented at encoding.
As in the original paradigm, subjects were required to decide whether they had seen the image before (old), or not (new), or just saw a similar picture to one they had been presented with during encoding (similar). The stimulus together with the response options (F = old, H = similar, K = new) remained on the screen for 2000 ms preceded by a 500-ms PSI. Importantly, as a modification compared to the original paradigm, immediately after making each old/similar/new decision, participants were required to judge on a 6-point scale how confident they were that they had made the right old/similar/new decision (where 1 = not at all sure, 6 = very sure). The stimulus remained on the screen for 5000 ms together with the response options, i.e., the confidence scale. Therefore, together with the old/similar/new response, each picture remained on the screen for 7000 ms.
The recognition test was preceded by a 1.5-min practice phase, while subjects saw the labels of the confidence scale (e.g., “not at all sure”) and were required to press the corresponding response button. During the practice phase, the labels remained on the screen for 5000 ms.
Results
illustrates the rate of old/similar/new responses for each stimulus type. Rates of responses were in the similar range as they were in the sample with a comparable age range (20–39 years) in Stark et al. (Citation2013).
Table 1. The rate of old, similar, and new responses given to the target, lure, and foil items (Experiment 1). Note(s). Values represent the means. Standard errors of the means are shown in parentheses.
a shows mean confidence ratings per response type per stimulus type. Since old responses given to the targets is suggested to form a stronger association with familiarity and similar responses given to the lures is assumed to form a stronger association with recollective remembering (Stark et al., Citation2013), we focused on these two response types when we analysed the data of Experiment 1. We analysed mean confidence ratings by conducting a repeated measures analysis of variance (ANOVA) with the two crucial Response types (old and similar) and the two crucial Stimulus types (target and lure) as independent factors. While the main effect of Response type was significant, F(1, 24) = 72.343, p < .001, = 0.751, Stimulus type had no main effect on confidence ratings, F(1, 24) = 2.260, p = .146,
= 0.086. Importantly, the interaction between these variables was significant, F(1, 24) = 69.605, p < .001,
= 0.744.
Figure 2. Confidence ratings in Experiment 1. Note(s). Mean confidence ratings for each response type and for each stimulus type (“A”) and confidence frequencies of correct responses (Old | Target and Similar | Lure) (“B”). Error bars represent the standard error of the mean.
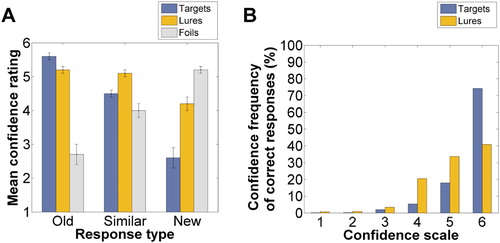
As post hoc tests, a list of difference contrast analyses was conducted, first to compare ratings between response types (old vs. similar) within the two stimulus types separately. For targets, confidence rating was higher for the old responses (Old | Target) than it was for the similar responses (Similar | Target), F(1, 24) = 52.612, p < .001, = 0.687. In contrast, there was no significant difference between confidence ratings given to the lures (Old | Lure vs. Similar | Lure), F(1, 24) = 0.721, p = .404,
= 0.029. Most importantly, we analysed confidence ratings for the correct responses as well (Old | Target vs. Similar | Lure), and we found that rating was higher for the targets than it was for the lures, F(1, 24) = 84.896, p < .001,
= 0.780.
Finally, we analysed the distributions of confidence rating frequencies of correct responses (Old | Target and Similar | Lure) with a special focus on the highest confidence rating category (6 = very sure), because the dual-process model of recognition memory suggests that recollection is associated with high confidence responses, when compared to familiarity-based responses (see Yonelinas, Citation1999, Citation2001a, Citation2001b). As can be seen in b, a high proportion (74.3%) of correct responses given to the targets was associated with the highest confidence rating. For the lures, 40.9% of the correct responses were associated with the highest confidence rating category.
In this experiment, however, we only assessed decision confidence levels and we could not make a clear conclusion on the underlying processes associated with behavioural pattern separation, because the dual-process model proposes that sometimes familiarity-based decisions are also associated with high confidence ratings (Yonelinas, Citation2001b). Therefore, in a second experiment, following the methodological tradition of recognition memory research, participants were not asked to make an old/similar/new decision, just to make a recognition confidence judgment on a scale ranging between “sure it was new” and “sure it was old”. This instruction allowed us to examine the discrimination between different types of stimuli, specifically, the separation between targets and foils as well as the separation between targets and critical lures.
Experiment 2
Materials and methods
Participants
There were 25 subjects in Experiment 2. One participant was excluded from the sample, because this subject gave no response in 75.6% of the trials in the test phase of the memory task. Therefore, we analysed the data of 24 subjects (6 men; age range: 19–41 years, M = 22.5, SD = 4.3).
Stimuli, experimental design, and procedure
The experimental procedure is illustrated in . The stimulus sets and the encoding phases of the tasks were identical in Experiment 1 and 2 (and were identical to ones used in the original paradigm developed by Stark and colleagues, Citation2013).
As in Experiment 1, subjects saw 192 images (64 targets, 64 foils, and 64 lures) in the middle of the computer screen on the recognition test. As a modification, participants were not asked to make an old/similar/new decision, but to make a recognition judgment on a 6-point confidence scale (where 1 = sure it was new, 6 = sure it was old). Each picture remained in the middle of the computer screen for 5000 ms (with a PSI of 500 ms), and the response options (i.e., the confidence scale) remained at the top of the computer screen during the whole recognition test. Just as in the first experiment, the recognition task was preceded by a 1.5-min practice phase, while subjects saw the labels of the confidence scale (e.g., “not at all sure”). They were instructed to press the corresponding response button. During the practice phase, the labels remained on the screen for 5000 ms.
Data analysis
We performed ROC analyses using the ROC Toolbox (The Regents of the University of California, Oakland, California, US) developed in the Matlab computing environment (R2014a, The MathWorks, Inc., Natick, Massachusetts, US) by Koen and colleagues (Citation2017). The DPSD model was fitted to our data set to analyse recognition confidence response frequencies and to separate recollection- and familiarity-based decisions. We used the general version of the model with the default constrains defined in the Toolbox.
An ROC curve was constructed by plotting confidence ratings given to the targets against false alarm rate for the foils (when participants gave incorrect old responses to foils). Furthermore, an ROC curve was generated by plotting confidence ratings given to the targets against false alarm rate for the lures (when participants gave old responses to lures). Finally, we transformed response proportions to z-scores as well to analyse whether zROCs are linear (see Macmillan & Creelman, Citation2005; Starns, Rotello, & Hautus, Citation2014; for an overview, see also Heathcote, Citation2003). ROC and zROC curves were fitted to data of all participants (i.e., we cumulated response frequencies for all subjects within each stimulus type) and to individual data as well.
Results
For aggregated subject confidence response frequencies, the DPSD model fit the data set well, for both the target-foil and the target-lure discriminations, both R2 s = .999, both SSEs = 10−4. shows the observed and the model predicted ROCs and zROCs.
Figure 3. ROC and zROC curves for target-foil and target-lure discriminations (Experiment 2). Note(s). The blue (dark) and the yellow (light) points represent the observed data for target-foil and target-lure discriminations, respectively. The black lines represent the model predicted ROCs and zROCs. The first row shows the ROC curves for target-foil (“A”) and target-lure (“B”) discriminations, whereas the second row shows the zROC curves again for target-foil (“C”) and target-lure (“D”) discriminations. ROC = receiving operating characteristic; zROC = z-transformed receiving operating characteristic.
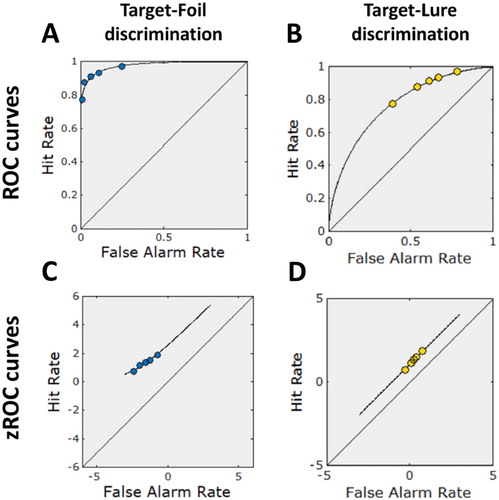
Since area under curve (AUC) is suggested to be a reliable measure of performance (sensitivity) without any model assumptions (see e.g., Green & Moses, Citation1966; Macmillan & Creelman, Citation2005; Pollack & Hsieh, Citation1969), we used this index to compare target-foil and target-lure discriminations when we analysed individual data. Paired-samples t-test showed that, as expected, accuracy was better for target-foil discrimination (MAUC = 0.965, SEM = 0.007), than it was for target-lure discrimination (MAUC = 0.715, SEM = 0.021), t(23) = 13.207, p < .001, d = 2.696.
When we analysed ROC curves fitted to individual data, the recollection (Ro) parameter was compared between target-foil and target-lure discriminations by conducting a Wilcoxon signed-rank test. The results showed that Ro was higher for the target-foil discrimination than it was for the target-lure discrimination (Mdtarget-foil = 0.720; Mdtarget-lure = 0.300), Z = 3.823, p < .001, r = .780.
To determine whether the zROC curves have significant linear components, or an additional quadratic component would result in a better fit, we performed a linearity analysis. First, a linear regression was performed on the zROC curve followed by a separate fit of a line with and additional quadratic component (with a quadratic coefficient c). The curve is considered linear if the addition of a quadratic term does not result in a significantly better fit, and curvilinear if the quadratic term improves the fit of the equation over the linear fit (Hilford, Glanzer, Kim, & DeCarlo, Citation2002; Slotnick & Dodson, Citation2005; Yonelinas, Citation1999).
The cumulative zROC curve of target-foil discrimination was best described by a linear model, R2 lin = .982, Flin(1, 3) = 163.980, MSElin = 4.3 × 10−3, plin = .001, and an additional quadratic component did not improve the fit of the curve, cquad = −0.062, Fquad(1, 2) = 0.321, MSEquad = 5.5 × 10−3, pquad = .628. Linearity analysis performed on the cumulative zROC curve of target-lure discrimination showed a significant linear relationship between target and lure z-scores, R2 lin = 1.000, Flin(1, 3) = 6445.899, MSElin = 1.1 × 10−4, plin < .001, where an additional quadratic component did not improve the fit of the curve significantly, cquad = 0.008, Fquad(1, 2) = 0.028, MSEquad = 1.6 × 10−4, pquad = .882. In brief, for target-lure discrimination, the symmetrical ROC curve (b) together with the linear zROC curve (d) suggest that familiarity, rather than recollection, contributed to task performance.
Experiment 3
Materials and methods
Participants
There were 25 subjects in Experiment 3. One participant was excluded from the sample, because this subject gave no response in 19.3% of the trials on the recognition test, which was more than 3 SDs from the mean of the sample (M = 6.9%, SD = 4.0). Therefore, we analysed the data of 24 participants (8 men; age range: 19–27 years, M = 21.8, SD = 2.1).
Stimuli, experimental design, and procedure
The experimental procedure is illustrated in . The stimulus sets and the encoding phases of the tasks were identical in Experiment 1, 2, and 3.
As in Experiment 1 and 2, participants saw 192 images (64 targets, 64 foils, and 64 lures) in the middle of the computer screen on the recognition test. Just as in Experiment 1, subjects were required to make an old/similar/new decision and they had 2000 ms to respond. Crucially, as a modification, after “old” and “similar” responses, participants were asked to give a secondary Remember/Know/Guess response. They had 5000 ms to respond (as in e.g., Wais et al., Citation2008). Subjects were asked to use the number row on a standard keyboard (and not the numeric pad); the response buttons were as follows: 1 = Remember, 2 = Know, and 3 = Guess. The stimulus remained on the screen for 5000 ms together with the response options. Therefore, together with the old/similar/new response, each picture remained on the screen for 7000 ms.
We used the instruction published in Rajaram (Citation1993). To avoid inter-individual differences in time until subjects understand the instructions, we used a 10-min delay between the encoding phase and the recognition test. During this delay (following Rajaram’s description) participants were asked to read the instructions and then explain to the experimenter how they would make the Remember/Know/Guess judgments. If it was necessary, the experimenter further clarified the instructions. If subjects still had time until the end of the 10-min delay, we asked them to wait for the recognition test.
The rationale for using a Guess response option was that sometimes individuals recognise items while their subjective experience meets the criteria for neither a Remember or a Know response. Even so, when they are motivated to perform well, they tend to choose the Remember or the Know response option. Their only other option is to give a “new” response resulting in reduced overall memory performance (for detailed overviews, see Dunn, Citation2004; Gardiner, Ramponi, & Richardson-Klavehn, Citation2002). For the Guess response option, we used the instruction of Dunn (Citation2004). Subjects were asked to respond Guess if the retrieval of an item did not meet the criteria for either a Remember or a Know decision, but the stimulus was judged to be old.
Results
As for data of Experiment 1, we analysed correct responses (old for targets and similar for lures). We analysed the ratio of Remember, Know, and Guess responses for targets and lures, separately (see ).
Figure 4. Remember, Know, and Guess responses in Experiment 3. Note(s). Error bars represent the standard error of the mean.
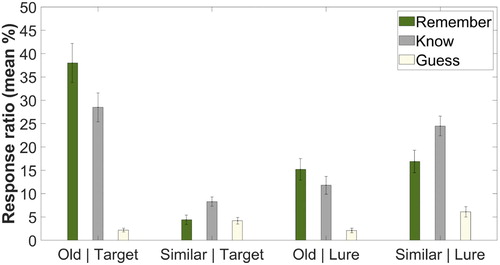
For old responses given to the targets, the repeated measures ANOVA (with three levels: Remember, Know, and Guess) indicated a significant difference, F(2, 46) = 28.036, p < .001, = 0.549. According to the contrast analyses, this difference was due to the reduced rate of Guess responses as compared to the Remember, F(1, 23) = 65.148, p < .001,
= 0.739, and Know judgments, F(1, 23) = 71.528, p < .001,
= 0.757. The rates of Remember and Know responses did not differ significantly, F(1, 23) = 2.070, p = .164,
= 0.083.
For similar responses given to the lures, the ANOVA indicated a significant difference as well, F(2, 46) = 19.909, p < .001, = 0.464. The rate of Guess responses was lower as compared to the Remember, F(1, 23) = 13.365, p = .001,
= 0.368, and Know judgments, F(1, 23) = 69.777, p < .001,
= 0.752. Most importantly, we found a bias toward Know responses, as compared to the ratio of Remember decisions, F(1, 23) = 4.745, p = .040,
= 0.171.
For further support, we performed a follow-up analysis where we analysed the two crucial stimulus types (targets and lures) and the two crucial secondary judgment types (Remember and Know) together. Only correct responses were analysed (old for targets and similar for lures). We conducted a 2 × 2 ANOVA with Stimulus type (target and lure) and Judgment type (Remember and Know) with independent factors. While the main effect of Stimulus type was significant, F(1, 23) = 31.873, p < .001, = 0.581, the main effect of Judgment type was not, F(1, 23) = 0.049, p = .826,
= 0.002. Most importantly, the interaction between these variables was significant, F(1, 23) = 8.324, p = .008,
= 0.266, indicating that the ratios of Remember and Know responses differed between targets and lures.
Discussion
The aim of the present study was to investigate processes that contribute to behavioural pattern separation measuring decision/recognition confidence levels and the conscious experience that accompanies memory retrieval. Crucially, on the recognition test, subjects were not only presented with old (target) and new (foil) items, but also with critical lures that were visually similar items to ones that had been presented at encoding. Our main finding is that successful pattern separation seemed to be based on familiarity, rather than on recollection.
The dual-process model proposes that when correct recognition is accompanied by recollection, responses are associated with the highest confidence level (“very sure”), whereas familiarity-based decisions are associated with a wide range of confidence responses (Yonelinas, Citation2001a, Citation2001b). In Experiment 1, we found that pattern separation (as measured by the rate of similar responses given to the lures) was accompanied by the highest confidence level in less than half (approximately 40%) of the trials. Based on these results, however, there are research questions that remain open. First, it was suggested that sometimes familiarity-based responses are associated with the highest confidence as well (Yonelinas, Citation2001b). Therefore, although 40% of the similar responses given to the lure items were associated with the highest confidence category, we could not be sure that this proportion of responses reflects only recollection, and not familiarity. Second, according to several theorists, recollection is associated with high level but not necessarily the highest level of confidence (Gardiner & Java, Citation1990; Tulving, Citation1985). That is, the remaining 60% of the responses might reflect recollection as well, and not only familiarity. Therefore, we conducted a second experiment, where due to the methodological modifications, we could plot ROC and zROC curves.
The dual-process model states that the ROC curve (plot of hits against false alarm rate) is asymmetrical when both recollection and familiarity contribute to memory performance and symmetrical when decisions are based solely on familiarity (Yonelinas, Citation1999, Citation2001b). In Experiment 2, we found an asymmetrical ROC curve when we plotted hits (old responses given to the targets) against false alarm rate for the foils (incorrect old responses given to the foils). In contrast, a symmetrical ROC curve was found when we plotted hits against incorrect old responses given to the lures. The conclusion follows from this latter finding that the correct discrimination between old items and critical lures is based on the level of item familiarity.
Plotting z-transformed hit rate against z-transformed false alarm rate across multiple levels of bias is another way to analyse confidence ratings (see e.g., Macmillan & Creelman, Citation2005; Starns et al., Citation2014). The dual-process model predicts a linear zROC when only familiarity contributes to performance and a curvilinear zROC when recognition memory decisions are accompanied by recollection, such as for correct source memory judgments (Yonelinas, Citation1999). We found a linear zROC for target-lure discrimination. This finding further corroborates that the discrimination between target items and lures depends on item familiarity.
Our findings, on the other hand, could be interpreted within the framework of the single-process model of recognition memory as well. The original version of the signal detection theory assumes two equal-variance normal distributions, one representing the memory strength of the old items and one representing the memory strength of the new items (for an overview, see e.g., Ratcliff, Sheu, & Gronlund, Citation1992). It has also been suggested, however, that the variance of the old items’ distribution could exceed the variance of the new items’ distribution (Egan, Citation1958; Ratcliff et al., Citation1992; Wixted, Citation2007). According to the unequal-variance signal detection theory, when the variance of the old items’ distribution is higher than that of the new items’ distribution, the ROC curve is asymmetrical, and when the two distributions is equal, the ROC curve is symmetrical (Mickes et al., Citation2007; Wixted, Citation2007). And this is what we found for the target-lure discrimination, specifically, a symmetrical ROC curve. It has also been shown that the two distributions are unequal for strong memories and equal for weaker memories (Squire et al., Citation2007; Wais et al., Citation2006). Therefore, it seems plausible that the distribution of the lures is similar and is closer to that of the targets (rather than to the distribution of the foils), resulting in weaker relative target strength compared to the lures. Nevertheless, whether our data is interpreted according to the single- or the dual-process model of recognition memory, it suggests that interference resolution between visually similar memory representations is based on the level of item familiarity.
Recently, Loiotile and Courtney (Citation2015) also assessed decision confidence frequencies in a modified version of the MST. Stimuli were presented either once or three times at encoding. On the recognition test, subjects had only two response options (old and new), and were asked to make a confidence judgment following each old/new decision. A superior target-lure discrimination was found in the 3-repeat condition, as compared to the 1-repeat condition, when confidence levels were analysed by plotting ROC curves. The authors concluded that behavioural pattern separation improves as a function of memory strength that was manipulated by the repetition of the stimuli. Importantly, however, in this study, the original paradigm was modified at two key points (repetitive stimulus presentation at encoding and two-choice response options at retrieval), and this study did not aim at investigating whether behavioural pattern separation is based on recollection or familiarity (or both).
In Experiment 3, we investigated the subjective experience that accompanied retrieval, and we found a bias toward Know responses (as compared to the rate of Remember and Guess responses) when we analysed behavioural pattern separation performance as measured by the rate of similar responses given to the lures. While Remember responses are suggested to reflect recollection, Know responses are suggested to be associated with familiarity-based recognition memory judgments (see e.g., Jacoby et al., Citation1997; Yonelinas, Citation2001b). Therefore, these findings indicate that successful pattern separation relies more on familiarity than on recollection. These results are consistent with the findings of our first two experiments where we measured decision/recognition confidence frequencies.
Another study also combined the MST with the Remember/Know paradigm (Kim & Yassa, Citation2013). Contrary to our results, the authors found a bias toward Remember judgments for similar responses given to the lures, when compared to the rate of Know responses (32% and 20%, respectively). When the authors interpreted these results within the framework of the dual-process model (assuming that Remember responses reflect recollection), they concluded that pattern separation is associated with, but not restricted to, recollection. It should be highlighted, that our paradigm and the task used in the study of Kim and Yassa differed in several aspects. First, we used a Guess response option to overcome a potential limitation of the original paradigm with two response options (Remember and Know); see Dunn (Citation2004) and Gardiner et al. (Citation2002) for detailed overviews. Second, we used a fixed delay of 10 min between encoding and the recognition test to avoid inter-individual differences in time until participants understand the Remember/Know/Guess instruction, and it has been demonstrated that the delay between study and test has differential effects on the forgetting rate of Remember and Know responses (see e.g., Sadeh, Ozubko, Winocur, & Moscovitch, Citation2016). Finally, we applied a frequently used Remember/Know instruction (Rajaram, Citation1993) that is not the same as Kim and Yassa used.
In sum, albeit we suggest that the separation between target and lure items is based on familiarity, we accept that pattern separation is a more complex concept and is not restricted to the ability to discriminate between studied items and similar lures. Therefore, further studies are needed to map the complex relationship between behavioural pattern separation and episodic remembering. Nevertheless, we suggest what Yassa and Stark (Citation2011) did, namely, pattern separation and recollection are not interchangeable terms and can occur independently from each other. The ratio of high confidence frequencies in Experiment 1 together with the ratio of Remember responses in Experiment 3 indicate that successful pattern separation is mostly associated with, but not restricted to, familiarity-based decisions.
Previously it was suggested that “like many tests of recognition memory, old/new recognition scores from the BPS-O task relies more on gist and familiarity than processes such as recollection” (Stark et al., Citation2013, page 2447; here BPS refers to the Behavioural Pattern Separation Task that was later renamed to Mnemonic Similarity Task). In line with this assumption, we found a linear zROC for target-foil discrimination in Experiment 2. However, in Experiment 3, old responses given to the targets were associated not only with Know decisions, but with Remember judgments as well, indicating that standard recognition memory score of the MST (similar to standard recognition memory scores of many tests of recognition memory) reflects recollective remembering as well.
Acknowledgment
We thank Karina Cozma, Anita Lencsés, and Fanni Takátsy for their help in data collection. We thank Ádám Markója for his help in preparing the experiments.
Disclosure statement
No potential conflict of interest was reported by the authors.
Data availability
Datasets related to this article are available at Open Science Framework (https://osf.io/nma7d/).
Additional information
Funding
References
- Atkinson, R. C., & Juola, J. F. (1973). Factors influencing speed and accuracy of word recognition. In S. Kornblum (Ed.), Attention and performance IV (pp. 1–58). New York: Academic Press.
- Bakker, A., Kirwan, C. B., Miller, M., & Stark, C. E. (2008). Pattern separation in the human hippocampal CA3 and dentate gyrus. Science, 319, 1640–1642. doi: 10.1126/science.1152882
- Brewer, W. F. (1996). What is recollective memory? In D. C. Rubin (Ed.), Remembering our past: Studies in autobiographical memory (pp. 19–66). Cambridge: Cambridge University Press.
- Brown, M. W., & Aggleton, J. P. (2001). Recognition memory: What are the roles of the perirhinal cortex and hippocampus? Nature Reviews Neuroscience, 2, 51–61. doi: 10.1038/35049064
- Conway, M. A. (2005). Memory and the self. Journal of Memory and Language, 53, 594–628. doi: 10.1016/j.jml.2005.08.005
- Dobbins, I. G. (2015). Forecasting versus fitting, dissociating versus describing. Celebrating Larry Jacoby’s methodological approach to understanding recognition. In D. S. Lindsay, C. M. Kelley, A. P. Yonelinas, & H. L. Roediger (Eds.), Remembering: Attributions, processes, and control in human memory (pp. 112–132). New York: Psychology Press.
- Dunn, J. C. (2004). Remember-know: A matter of confidence. Psychological Review, 111, 524–542. doi: 10.1037/0033-295X.111.2.524
- Egan, J. P. (1958). Recognition memory and the operating characteristic, (Tech. Note AFCRC-TN-58-51). Hearing and Communication Laboratory. Bloomington, IN: Indiana University.
- Fortin, N. J., Wright, S. P., & Eichenbaum, H. (2004). Recollection-like memory retrieval in rats is dependent on the hippocampus. Nature, 431, 188–191. doi: 10.1038/nature02853
- Gardiner, J. M., & Java, R. I. (1990). Recollective experience in word and nonword recognition. Memory and Cognition, 18, 23–30. doi: 10.3758/BF03202642
- Gardiner, J. M., & Parkin, A. J. (1990). Attention and recollective experience in recognition memory. Memory and Cognition, 18, 579–583. doi: 10.3758/BF03197100
- Gardiner, J. M., Ramponi, C., & Richardson-Klavehn, A. (2002). Recognition memory and decision processes: A meta-analysis of remember, know, and guess responses. Memory, 10, 83–98. doi: 10.1080/09658210143000281
- Green, D. M., & Moses, F. L. (1966). On the equivalence of two recognition measures of short-term memory. Psychological Bulletin, 66, 228–234. doi: 10.1037/h0023645
- Heathcote, A. (2003). Item recognition memory and the receiver operating characteristic. Journal of Experimental Psychology: Learning, Memory, and Cognition, 29, 1210–1230. doi: 10.1037/0278-7393.29.6.1210
- Hilford, A., Glanzer, M., Kim, K., & DeCarlo, L. T. (2002). Regularities of source recognition: ROC analysis. Journal of Experimental Psychology: General, 131, 494–510. doi: 10.1037/0096-3445.131.4.494
- Hunsaker, M. R., & Kesner, R. P. (2013). The operation of pattern separation and pattern completion processes associated with different attributes or domains of memory. Neuroscience and Biobehavioral Reviews, 37, 36–58. doi: 10.1016/j.neubiorev.2012.09.014
- Jacoby, L. L., Yonelinas, A. P., & Jennings, J. M. (1997). The relation between conscious and unconscious (automatic) influences: A declaration of independence. In J. D. Cohen & J. W. Schooler (Eds.), Scientific approaches to consciousness (pp. 13–47). Mahwah, NJ: Lawrence Erlbaum Associates.
- Kheirbek, M. A., Klemenhagen, K. C., Sahay, A., & Hen, R. (2012). Neurogenesis and generalization: A new approach to stratify and treat anxiety disorders. Nature Neuroscience, 15, 1613–1620. doi: 10.1038/nn.3262
- Kim, J., & Yassa, M. A. (2013). Assessing recollection and familiarity of similar lures in a behavioral pattern separation task. Hippocampus, 23, 287–294. doi: 10.1002/hipo.22087
- Kirwan, C. B., Hartshorn, A., Stark, S. M., Goodrich-Hunsaker, N. J., Hopkins, R. O., & Stark, C. E. (2012). Pattern separation deficits following damage to the hippocampus. Neuropsychologia, 50, 2408–2414. doi: 10.1016/j.neuropsychologia.2012.06.011
- Koen, J. D., Barrett, F. S., Harlow, I. M., & Yonelinas, A. P. (2017). The ROC Toolbox: A toolbox for analyzing receiver-operating characteristics derived from confidence ratings. Behavior Research Methods, 49, 1399–1406. doi: 10.3758/s13428-016-0796-z
- Levy, W. B. (1989). A computational approach to hippocampal function. Psychology of Learning and Motivation, 23, 243–305. doi: 10.1016/S0079-7421(08)60113-9
- Loiotile, R. E., & Courtney, S. M. (2015). A signal detection theory analysis of behavioral pattern separation paradigms. Learning and Memory, 22, 364–369. doi: 10.1101/lm.038141.115
- Macmillan, N. A., & Creelman, C. D. (2005). Detection theory: A user’s guide (2nd ed.). New York: Psychological Press.
- Manns, J. R., Hopkins, R. O., Reed, J. M., Kitchener, E. G., & Squire, L. R. (2003). Recognition memory and the human hippocampus. Neuron, 37, 171–180. doi: 10.1016/S0896-6273(02)01147-9
- McClelland, J. L., McNaughton, B. L., & O'Reilly, R. C. (1995). Why there are complementary learning systems in the hippocampus and neocortex: Insights from the successes and failures of connectionist models of learning and memory. Psychological Review, 102, 419–457. doi: 10.1037/0033-295X.102.3.419
- Mickes, L., Wixted, J. T., & Wais, P. E. (2007). A direct test of the unequal-variance signal detection model of recognition memory. Psychonomic Bulletin and Review, 14, 858–865. doi: 10.3758/BF03194112
- Murdock, B. B., & Dufty, P. O. (1972). Strength theory and recognition memory. Journal of Experimental Psychology, 94, 284–290. doi: 10.1037/h0032795
- Pollack, I., & Hsieh, R. (1969). Sampling variability of the area under the ROC-curve and of d'e. Psychological Bulletin, 71, 161–173. doi: 10.1037/h0026862
- Rajaram, S. (1993). Remembering and knowing: Two means of access to the personal past. Memory and Cognition, 21, 89–102. doi: 10.3758/BF03211168
- Ratcliff, R., Sheu, C. F., & Gronlund, S. D. (1992). Testing global memory models using ROC curves. Psychological Review, 99, 518–535. doi: 10.1037/0033-295X.99.3.518
- Sadeh, T., Ozubko, J. D., Winocur, G., & Moscovitch, M. (2016). Forgetting patterns differentiate between two forms of memory representation. Psychological Science, 27, 810–820. doi: 10.1177/0956797616638307
- Segal, S. K., Stark, S. M., Kattan, D., Stark, C. E., & Yassa, M. A. (2012). Norepinephrine-mediated emotional arousal facilitates subsequent pattern separation. Neurobiology of Learning and Memory, 97, 465–469. doi: 10.1016/j.nlm.2012.03.010
- Slotnick, S. D., & Dodson, C. S. (2005). Support for a continuous (single-process) model of recognition memory and source memory. Memory and Cognition, 33, 151–170. doi: 10.3758/BF03195305
- Squire, L. R., Wixted, J. T., & Clark, R. E. (2007). Recognition memory and the medial temporal lobe: A new perspective. Nature Reviews Neuroscience, 8, 872–883. doi: 10.1038/nrn2154
- Stark, M. S., Kirwan, C. B., & Stark, C. E. (2019). Mnemonic Similarity task: A tool for assessing hippocampal integrity. Trends in Cognitive Sciences, 23, 938–951. doi: 10.1016/j.tics.2019.08.003
- Stark, S. M., Yassa, M. A., Lacy, J. W., & Stark, C. E. (2013). A task to assess behavioral pattern separation (BPS) in humans: Data from healthy aging and mild cognitive impairment. Neuropsychologia, 51, 2442–2449. doi: 10.1016/j.neuropsychologia.2012.12.014
- Stark, S. M., Yassa, M. A., & Stark, C. E. (2010). Individual differences in spatial pattern separation performance associated with healthy aging in humans. Learning and Memory, 17, 284–288. doi: 10.1101/lm.1768110
- Starns, J. J., Rotello, C. M., & Hautus, M. J. (2014). Recognition memory zROC slopes for items with correct versus incorrect source decisions discriminate the dual process and unequal variance signal detection models. Journal of Experimental Psychology: Learning, Memory, and Cognition, 40, 1205–1225. doi: 10.1037/a0036846
- Treves, A., & Rolls, E. T. (1994). Computational analysis of the role of the hippocampus in memory. Hippocampus, 4, 374–391. doi: 10.1002/hipo.450040319
- Tulving, E. (1972). Episodic and semantic memory. In E. Tulving & W. Donaldson (Eds.), Organization of memory (pp. 382–402). New York: Academic Press.
- Tulving, E. (1985). Memory and consciousness. Canadian Psychology/Psychologie Canadienne, 26, 1–12. doi: 10.1037/h0080017
- Tulving, E. (2002). Episodic memory: From mind to brain. Annual Review of Psychology, 53, 1–25. doi: 10.1146/annurev.psych.53.100901.135114
- Tulving, E., & Markowitsch, H. J. (1998). Episodic and declarative memory: Role of the hippocampus. Hippocampus, 8, 198–204. doi: 10.1002/(SICI)1098-1063(1998)8:3<198::AID-HIPO2>3.0.CO;2-G
- Wais, P. E., Mickes, L., & Wixted, J. T. (2008). Remember/know judgments probe degrees of recollection. Journal of Cognitive Neuroscience, 20, 400–405. doi: 10.1162/jocn.2008.20041
- Wais, P. E., Wixted, J. T., Hopkins, R. O., & Squire, L. R. (2006). The hippocampus supports both the recollection and the familiarity components of recognition memory. Neuron, 49, 459–466. doi: 10.1016/j.neuron.2005.12.020
- Wixted, J. T. (2007). Dual-process theory and signal-detection theory of recognition memory. Psychological Review, 114, 152–176. doi: 10.1037/0033-295X.114.1.152
- Wixted, J. T., & Mickes, L. (2010). A continuous dual-process model of remember/know judgments. Psychological Review, 117, 1025–1054. doi: 10.1037/a0020874
- Wixted, J. T., Mickes, L., & Squire, L. R. (2010). Measuring recollection and familiarity in the medial temporal lobe. Hippocampus, 20, 1195–1205. doi: 10.1002/hipo.20854
- Yassa, M. A., Lacy, J. W., Stark, S. M., Albert, M. S., Gallagher, M., & Stark, C. E. (2011). Pattern separation deficits associated with increased hippocampal CA3 and dentate gyrus activity in nondemented older adults. Hippocampus, 21, 968–979. doi: 10.1002/hipo.20808
- Yassa, M. A., & Stark, C. E. (2011). Pattern separation in the hippocampus. Trends in Neurosciences, 34, 515–525. doi: 10.1016/j.tins.2011.06.006
- Yonelinas, A. P. (1999). The contribution of recollection and familiarity to recognition and source memory judgments. Journal of Experimental Psychology: Learning, Memory, and Cognition, 25, 1415–1434. doi: 10.1037/0278-7393.25.6.1415
- Yonelinas, A. P. (2001a). Consciousness, control, and confidence: The 3 Cs of recognition memory. Journal of Experimental Psychology: General, 130, 361–379. doi:10.1037//0096-3445.130.3.361 doi: 10.1037/0096-3445.130.3.361
- Yonelinas, A. P. (2001b). Components of episodic memory: The contribution of recollection and familiarity. Philosophical Transactions of the Royal Society B, 356, 1363–1374. doi:10.1098/rstb.2001.093 doi: 10.1098/rstb.2001.0939
- Yonelinas, A. P. (2002). The nature of recollection and familiarity: A review of 30 years of research. Journal of Memory and Language, 46, 441–517. doi: 10.1006/jmla.2002.2864