Abstract
Unialgal cultures of the widespread tremalith-bearing coccolithophorid Ochrosphaera neapolitana Schussnig were investigated using light and electron microscopy. O. neapolitana exhibits a heteromorphic life cycle identical to that described for the closely related tremalith-bearing genus Hymenomonas, involving an alternation between (diploid) coccolith-bearing cells and a (haploid) non-calcifying phase, characterised by the production of layers of mucilage. Differing scale ornamentation between the two phases together with chromosome counts confirms the diplo-haplontic nature of this cycle. The coccolith-bearing phase may be non-motile or possess flagella. The coccoliths of all strains studied exhibit the typical tremalith structure, but variation in size and shape is observed (vari-monomorphism). Unusual among coccolithophorids, the coccosphere of this species is not continually renewed, and progressive extracellular calcification of the coccoliths is observed in old cultures that may result in the formation of a calcareous ‘pseudocyst’. At the ultrastructural level, the flagellar apparatus is of the Pleurochrysis type, consisting of two flagellar basal bodies linked by proximal, intermediary and distal bands, a vestigial haptonema consisting of five microtubules, and four asymmetrically arranged microtubular roots. Two roots are compound, each possessing a secondary crystalline microtubular component, and two are simple. The peripheral endoplasmic reticulum plays a role in coccolithogenesis, which occurs in the proximity of the plasma membrane. The taxonomy of the genus Ochrosphaera is discussed and suppression of the two other species in the genus, O. verrucosa Schussnig and O. rovignensis Schussnig, is suggested.
Introduction
The majority of coccolithophorids, unicellular algae in the class Prymnesiophyceae, have cells covered by microscopic calcite plates (coccoliths), and are typically found in oceanic environments where they may form extensive blooms (e.g. Balch et al., Citation1996). However, a number of genera inhabit inshore waters exclusively. These littoral coccolithophorid genera, Pleurochrysis Pringsheim, Hymenomonas Stein, Ochrosphaera Schussnig, Cruciplacolithus Hay et Mohler, and Jomonlithus Inouye et Chihara (the latter being more characteristic of brackish water environments), rarely form visible blooms, and are of limited significance in the biogeochemical context of long term carbonate fixation. However, they are relatively easy to culture and thus are the subject of a larger body of cytological information compared to the oceanic species.
In our experience Ochrosphaera is the most frequently observed coccolithophorid in littoral zone samples from diverse locations in the North Atlantic and Indian Oceans and the Mediterranean Sea (Fresnel, Citation1989). Inouye (Citation1988) also noted that Ochrosphaera is one of the most common and widely distributed microalgae of the littoral zone of the Japanese current region.
The genus Ochrosphaera was created by Schussnig (Citation1930) for a single species, Ochrosphaera neapolitana. Like the genus Hymenomonas, Ochrosphaera is characterised by the presence of tremaliths (vase-shaped coccoliths calcified only on the rim of the base-plate scale). Using Schussnig's original strain plus new material, Schwartz (Citation1932) described sexual reproduction within the life cycle of O. neapolitana and made a detailed light microscope study of the cytology of this species, which was followed up by Magne (Citation1952). This species has been the subject of previous research in our laboratory (Lepailleur, Citation1969), followed by preliminary electron microscopic observations, notably of the coccoliths (Gayral & Fresnel-Morange, Citation1971). In 1940 Schussnig (Citation1940) described two more species, O. verrucosa and Ochrosphaera rovignensis. Since these descriptions were based on material in mixed enrichment cultures, reference cultures were not available for further study. The next author to mention Ochrosphaera verrucosa was Norris (Citation1967), who cited the ‘probable’ presence of this species. Several authors (West, Citation1969; Lefort, Citation1971, Citation1975; Inouye & Chihara, Citation1980; Inouye, Citation1988) have subsequently reported O. verrucosa without, in our opinion, providing convincing arguments that exclude the possibility that some of the observed organisms may actually have been O. neapolitana. One of the objectives of our study was to clarify the ambiguity that exists about these two taxa.
The classical taxonomy of coccolithophorids has been based almost exclusively on differences in the morphology of the scales and coccoliths which cover the cells, but increasing awareness of the bi-phasic nature of coccolithophorid life cycles has added a degree of complexity to this scheme. In this paper, life cycle observations of O. neapolitana supplement those of previous authors and provide evidence for an alternation of generations of the type observed in the closely related genus Hymenomonas (Fresnel, Citation1994). It is clear that any review of the taxonomy of the coccolithophorids should integrate as much information as possible from diverse sources in order to provide a more robust systematic overview of the group. Molecular genetic analyses (Edvardsen et al., Citation2000; Fujiwara et al., Citation2001) have already produced invaluable new data to supplement classical taxonomy and have provided a basis for phylogenetic interpretation. Ultrastructural analysis of the cell itself provides another line of evidence, and a detailed analysis of the fine structure of O. neapolitana, with emphasis on the structure of the flagellar apparatus, is presented.
Materials and methods
The unialgal culture strains used in this study are detailed in . The ultrastructural study was conducted using strain AC13 and the life cycle investigation using strain AC12. Other strains were employed for comparative observations. Cultures originating from our laboratory were clonal, having been initiated from single cell isolations from mixed enrichment cultures or agar plates. All cultures were maintained in ES-Tris II medium (Cosson, Citation1987) at room temperature in daylight.
Table 1 . Culture strains employed in this study
Light microscopic observations were conducted with a Leitz Orthoplan microscope equipped with differential interference contrast (DIC) optics. For chromosome counting, freshly sub-cultured cells were fixed at different times of the day and night in ethanol:glacial acetic acid (3 : 1) for at least 1 h, and subsequently stained with lactopropionic orcein (Dyer, Citation1963).
For scanning electron microscopy, drops of cell suspension were fixed for 5–10 min with a few drops of 2% osmium tetroxide in 0.1 M sodium cacodylate buffer (pH 7.2). After centrifugation the pellet was rinsed several times with neutralized distilled water and the cells placed on glass coverslips and dried. The preparations were coated with gold/palladium and viewed with either a JEOL 100C or 100CX electron microscope. Whole mounts for transmission electron microscopy were fixed as above and placed on formvar-coated grids. In order to study non-mineralized scales, the coccoliths were dissolved with dilute acid. The grids were coated with gold/palladium at an angle of 20 to 30°.
For TEM, a cell suspension was gently centrifuged and the pellet fixed for 2 h at 4°C in a glutaraldehyde solution (3%) in 0.1 M sodium cacodylate buffer (pH 7.2) containing 0.25 M sucrose. Cells were then washed three times in 0.1 M cacodylate buffer containing decreasing concentrations of sucrose (0.25 M, 0.12 M, the last wash without sucrose) for 15 min each time, followed by post-fixation in 2% osmium tetroxide in 0.1 M cacodylate buffer for 1.5 h at 4°C. After washing in distilled water, cells were embedded in a fibrin network (Charret & Fauré-Frémiet, Citation1967), dehydrated in a graded ethanol series, and embedded in Epon resin. A slight modification to the fixation protocol was employed to conserve external coccoliths (although it resulted in poorer fixation of cell contents), using 3% glutaraldehyde in culture medium for 2 h, followed by 3 rinses in culture medium (the rest of the protocol was identical to that described above). Sections were double stained with uranyl acetate followed by Reynold's lead citrate. Shadowed preparations and thin sections were viewed with a Siemens 1A or 102 transmission electron microscope.
Observations
Light microscopy (–)
Figs 1–14. Light microscope (DIC) observations of Ochrosphaera neapolitana. . Optical section of non-motile cells showing coccoliths in profile. . Surface view of cells showing ring-shaped coccoliths in surface view. . Optical section showing thin lobed chloroplasts. . Cells fixed with osmium vapour revealing different aspects of the pyrenoid. Note the cell with separated pyrenoids (arrowhead), a pyrenoid with a long stalk (single arrow), and two pyrenoids abutting each other (double arrow). . Two and four cells in the same coccosphere in an older culture. The layer of scales thickens and the coccoliths become widely spaced as the coccosphere expands. . Division in a young culture showing one of the two cells being released from the coccosphere. The released cell is usually elongate (upper left) and possesses flagella. . Four cells in a coccosphere following vegetative division in an old culture. . Release of flagellated daughter cells from a coccosphere after immersion in new medium. –. Progressive stages in the over-calcification of coccoliths and the formation of pseudocysts. . Cultured cells after three months. . Surface view of a cell after several months in culture with individual over-calcified coccoliths still visible. . Intermediate stage in the formation of a pseudocyst (individual coccoliths no longer visible). . A heavily over-calcified pseudocyst. . Release of a naked cell from a pseudocyst. . Two cells within an envelope recently released from a pseudocyst (note newly formed coccoliths on envelope). Scale bar in represents 10 µm and applies to all figures.
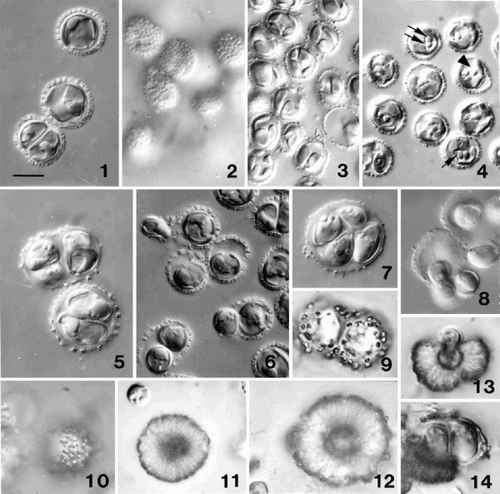
Cells measure 7–8 µm in diameter (total range of 5–12 µm) and are covered by a single layer of tremaliths (), which are more or less circular in surface view (), and vase-shaped in section (). In young cultures, tremaliths are thin and lightly calcified. As the culture ages and cells aggregate, the translucent layer of organic body scales under the coccoliths thickens, the cells increase in size and the coccoliths become more widely spaced (). Each cell has two thin parietal, often bilobed, golden-yellow chloroplasts (). One of the characteristics of Ochrosphaera is the shape of the bulging pyrenoids that are clearly visible after fixation with osmium vapour, and are situated on the internal face of each chloroplast, protruding into a vacuole (). A canal may separate the two pyrenoids, or, more often, they may be in contact with each other ().
The non-motile coccolith-bearing cells are usually solitary in young cultures (, ). Following division, daughter cells may remain within the ‘mother’ coccosphere for a short time, and packets of two (rarely four) cells were observed (, ). One of the daughter cells subsequently escapes (), acquires flagella, and rapidly forms a new covering of coccoliths. The other cell conserves the initial coccosphere, growing to fill its entire volume (). In older cultures, after cell division ceased, large coccospheres with thick coverings of scales enclosing two or four cells were observed (, ). Four or five days after transfer into fresh medium, these cells form flagella and leave the mother cell (). Cells are often naked when released, but rapidly acquire a complete coccosphere (note that Schwarz (Citation1932) reported that coccoliths only appeared after loss of the flagella). These elongate cells are 9 × 6 µm (, ), and possess two flagella of slightly unequal length (12–15 µm), but no emergent haptonema is visible. The cytological characteristics of motile cells are identical to those of non-motile cells. The motile cells exhibit strongly positive phototactism and remain motile for a few days.
O. neapolitana does not continuously regenerate its coccosphere as is common in other cultured coccolithophorid species, but exhibits extracellular over-calcification of coccoliths in old cultures. shows cells of strain LB-1722 (from J.A. West) soon after sub-culture, while shows the same strain after 3 months in culture, when the tremaliths are no longer recognisable. – show progressively more heavily calcified stages in strain AC12 and the formation of calcareous ‘pseudocysts’. Such pseudocysts may liberate one () or two () usually naked cells. While the pseudocysts may represent resistant stages, liberation of cells may occur without transfer to fresh medium; the apparently compact calcareous covering disaggregates, forming an opening through which the cell(s) can escape. It is not clear which factors are involved in regulating cell liberation.
Electron microscopy (–)
Periplast covering
The basic structure of the tremaliths of O. neapolitana remains constant, but there is considerable variability in coccolith morphology. Each coccolith is composed of 5–9 thin, lightly calcified trapezoid plates that form a vase shape with a protruding perforated base () when assembled and viewed in profile. The coccolith elements are distally truncated when compared to members of the genus Hymenomonas in which elements end in a point (Gayral & Fresnel, Citation1976). The interior of newly formed coccoliths is smooth, while some protuberances (typically 0.6 µm high × 1.0 µm diameter) may be present on the exterior surface, generally towards the base of the vase, near the suture lines between the elements (, ).
Figs 15–21. SEM observations of Ochrosphaera neapolitana coccoliths. . Group of coccoliths showing vase-shaped coccoliths with external protrusions in profile and from above (arrow) and two pulley-shaped coccoliths in surface view (arrowheads). . Detail of a vase-shaped coccolith in profile. . ‘Intermediate’ coccolith. . West's strain after one month of culture exhibiting small protrusions on the coccoliths. Pulley-shaped coccolith with a small distal flange (arrow). . Increasingly over-calcified coccolith. . Highly over-calcified coccosphere in which the central cavities of the coccoliths are almost filled. . Pseudocyst in which individual coccoliths are no longer recognisable. Scale bars represent 1 µm (), 0.5 µm (–) and 2 µm (, ).
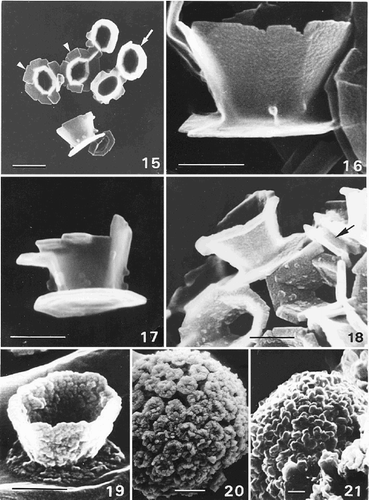
In addition to this coccolith type, which is observed most frequently and which we consider typical of O. neapolitana, coccoliths that resemble pulleys in profile (, ) are also found. These coccoliths are smaller (only 0.3 µm in height) and have a protruding distal flange that is roughly equivalent in diameter to the base. When observed distally, the rim elements overlap slightly. A complete range of intermediate morphologies has been observed between these two coccolith types. Coccoliths that could be classified as ‘intermediate’ have been observed occasionally, in which some of the distal elements have a distal flange and others are normally truncated (). Vase-shaped coccoliths with a thin distal flange are much more common (), corresponding to those described by West (Citation1969) for O. verrucosa. For clarification, West's original strain (UTEX culture collection, LB-1722) was examined. In this strain, the same heterogeneity in coccolith shape was observed, both pulley-shaped and normal vase-shaped coccoliths, as well as vase-shaped coccoliths with a distal flange, the only type described by West (Citation1969). The different coccolith types are often mixed on the same coccosphere (the TEM section in shows both extremes of coccolith shape side by side on the same cell). Both non-motile and motile cells exhibit the same range in coccolith shape.
Progressive stages in pseudocyst formation are illustrated in –. Tremaliths are thin and more or less smooth when newly formed. The earliest signs of over-calcification are visible on the interior surface of the coccolith (). The entire coccolith subsequently becomes thick and irregular (). This process occurred in all strains of O. neapolitana that were observed. In cultures that contain the coccolith-bearing stage and the mucilage producing non-calcifying stage (see below), over-calcification may continue to such an extent that individual coccoliths are no longer distinguishable and a pseudocyst is formed (, ). Whole mounts of motile cells viewed by TEM confirm the absence of a haptonema and show the sub-equal acronematic flagella ().
Figs 22–29. TEMs showing general cell ultrastructure and scale morphology of Ochrosphaera neapolitana. , , are shadowed with gold/palladium. . Coccolith-bearing flagellated cell. Note the absence of an emergent haptonema and the unequal acronematic flagella. . Section showing two extremes of coccolith shape, vase-shaped (arrow) and pulley-shaped (arrowhead), side by side on one cell. . Proximal face of a coccolith base-plate scale with radiating microfibrils in quadrants. . Distal face of coccolith base-plate scale (arrow) showing a vaguely concentric pattern of microfibrils. Circular body scales with an identical pattern of superficial, irregularly concentric microfibrils and a thin rim on each face are also visible. . Section of whole cell showing the main cell components: nucleus (N), chloroplasts (C), pyrenoid (P) (here with an enlarged base), mitochondrion (m), vacuole (V). . Detail of Golgi body showing several forming scales in the middle and distal parts of the Golgi stack (arrows). Columnar material (cm) is visible against the plasmalemma and in Golgi vesicles (arrowheads). . Stalked pyrenoid: arrows indicate the periplastidial reticulum at the base of the pyrenoid. . Pyrenoid thylakoid continuous with that of the chloroplast. Scale bars represent 2 µm (), 0.5 µm (–), and 1 µm (–).
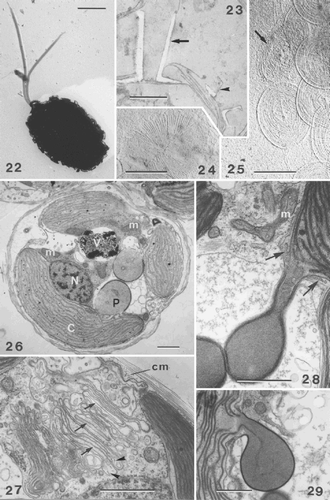
Coccolith base-plate scales are more or less oval, and measure 0.8–0.9 µm × 0.4–0.7 µm. A pattern of radiating filaments divided into four sectors (approximately 30 filaments per quadrant) is present on the proximal face of the base-plate scale (), while the distal face has a more or less uniform cover of fine filaments (). The external surface of the plasmalemma is covered by a thick layer of columnar material and by several layers of circular non-calcified body scales (). These scales measure 0.6–0.7 µm in diameter and have an identical pattern of superficial concentric microfibrils and a slightly raised rim on each face (). The smaller body scales observed in the anterior region in Hymenomonas lacuna Pienaar(Pienaar, Citation1976) and Hymenomonas globosa (Magne) Gayral et Fresnel (our observations), both of which are tremalith-bearing species that lack an emergent haptonema, have not been detected in O. neapolitana.
Cell ultrastructure
The internal organisation of the cell shows many of the features characteristic of the Prymnesiophyceae. The entire cytoplasm is enclosed by peripheral endoplasmic reticulum (PER) situated immediately beneath the plasmalemma. The nucleus is located adjacent to both chloroplasts and the nuclear envelope is continuous with the chloroplastic reticulum (, ). Mitochondria with tubular cristae are situated in the centre of the cell, often adjacent to the inner surface of the chloroplast (, ). A single Golgi body lies adjacent to the nucleus. The cisternae in the middle portion of the stack show intercalary dilations lined with densely staining material, characteristic of the class (). Organic scales are produced in Golgi derived vesicles, and several sacs, each containing a single fully formed scale, were observed towards the mature face of the Golgi stack (). Columnar material is also formed in Golgi vesicles ().
Each parietal chloroplast possesses lamellae with three thylakoids, and contains a bulging pyrenoid. The pyrenoids are mostly pyriform (), and the thin base may become elongated to form a stalk (). Girdle lamellae are not present in the chloroplast. The dense stroma of each pyrenoid encloses one or two lamellae of two thylakoids, which form a loop within the pyrenoid and are continuous with those of the chloroplast (). Numerous small vesicles are present in the space between the chloroplast envelope and the periplastidial membranes at the base of the pyrenoids ().
Coccolithogenesis
Since coccolith formation in O. neapolitana does not continue once the coccosphere is complete, developing coccoliths were rarely observed in preparations of young, actively growing cultures. Coccolithogenesis occurs in the immediate proximity of the plasmalemma (–). Each cell may contain several forming coccoliths, each in a separate vesicle. Coccolith base-plate scales are formed in Golgi stack cisternae that elongate laterally as they bud off the trans face and migrate towards the cell periphery (). The vesicle subsequently dilates when it is in close proximity to the PER (, ), with the base-plate scale variously oriented relative to the plasmalemma (, ). From this early stage and throughout coccolithogenesis, coated vesicles of Golgi origin are seen fusing with the vesicle containing the forming coccolith ().
Figs 30–33. Coccolithogenesis in Ochrosphaera neapolitana. . Oblique section of the distal part of a coccolith vesicle showing the distal tubular matrix containing densely stained material (Tm) continuous with the PER (arrow). The internal membrane of the coccolith vesicle is lined with densely staining material and a coated vesicle fusing with the coccolith vesicle (arrowhead). . Section of a vesicle containing vase-shaped coccolith at an advanced stage of development, showing interconnections of the well developed tubular matrix (Tm) and PER with the coccolith vesicle. Arrows indicate points within the coccolith vesicle, which are presumed first stages of calcification (calcite presumably dissolved during fixation). , . Advanced stages in the formation of pulley-shaped coccoliths. . Portion of a cell showing that the lining of the coccolith vesicle is denser than the central clearer zone where calcification will occur. Note reduced tubular matrix (Tm). . Cell showing that the PER follows the contours of the coccolith vesicle. Note the different orientation of the coccolith vesicle in , . Scale bars represent 0.5 µm.
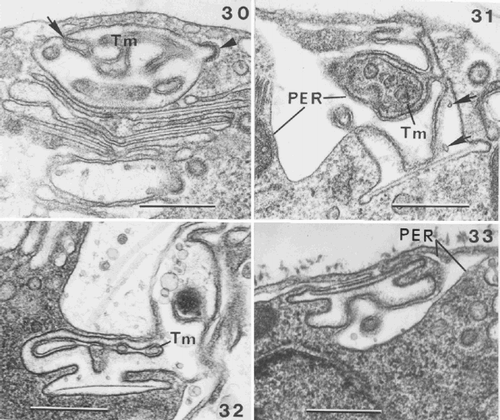
The PER appears to play an important role in coccolith development. In early stages, invaginations of the PER form a tubular matrix within the coccolith vesicle (). This matrix is less obvious during the formation of pulley-shaped coccoliths (, ) and contains densely stained material (, ). The PER and tubular matrix appear to interconnect with, and to be intimately involved in modelling the contours of, the vesicle containing the forming coccolith (–). The calcite structure of the coccolith is not visible until a late stage (), but the internal lining of the membrane appears increasingly dense, leaving a clearer region within each vesicle, which delimits the calcified zone of the future coccolith (, , ). The tremaliths of O. neapolitana are thin and lightly calcified and it is clear that calcification only occurs in this clear zone and does not involve the entire lumen of the coccolith vesicle.
Flagellar apparatus
The fine structure of the flagellar apparatus of O. neapolitana is described using the terminology of Inouye & Pienaar (Citation1985) and Beech & Wetherbee (Citation1988). The helical band characterised in Pleurochrysis carterae (Braarud et Fagerland) Christensen (Beech & Wetherbee, Citation1988) is probably present at the base of the axonemes (). O. neapolitana also possesses an axosome in the distal part of the flagellar transition zone (, ), the structure of which is apparently the same as that of P. carterae. The motile cells of O. neapolitana do not possess an emergent haptonema, but a vestigial haptonema composed of 5 microtubules is visible in section (). The microtubules of the haptonematal base form a semi-circle with the concave face oriented towards the left basal body. As pointed out by Roberts & Mills (Citation1992) this character is a useful marker for cellular orientation. The four main flagellar roots are associated with the flagellar bases in O. neapolitana and are similar to those observed in Pleurochrysis sp. (Inouye & Pienaar Citation1985). The complex roots (R1, R2), with their secondary crystalline components (CR1, CR2) (, ), as well as the simple roots (R3, R4) are present. The microtubular plate of R1 is associated with the left flagellar base and has a reduced number of microtubules relative to Pleurochrysis. In contrast, CR1 is well developed (, , ). Part of R1 extends to meet the haptonematal base (). R2, which is composed of 4 microtubules (), arises between the flagellar bases and extends in the opposite direction to the haptonema (). CR2 (, , ) is composed of a reduced number of microtubules, compared with Pleurochrysis. R3 and R4 are formed of 4 and 2 microtubules respectively (), and bifurcate on either side of the right flagellar base (, ).
Figs 34–40. Flagellar root structure in Ochrosphaera neapolitana. . Tangential section of a flagellum showing the probable presence of the helical band at the base of the axoneme (arrow). Roots (R3, R4) are also visible in transverse section (arrowheads). . Longitudinal section of a flagellar base at the level of the axosome (Ax). . Transverse section of left and right flagellar bases (L and R) and haptonematal base (H) with five microtubules. Proximal and intermediary (Pb and Ib) striated bands connect the flagellar bases, and the accessory band links the haptonematal base and the left flagellar base (Ab3). –. Serial sections of the flagellar bases in transverse section: . Section through root (R1) showing linkage via accessory band (Ab4) to the left flagellar base. The crystalline component (CR1) is visible. Roots (R3, R4) extend from either side of the right flagellar base. The contractile root (Cr) extends along the surface of the chloroplast at the periphery of the cell; the connection of the Cr with the fibrous root (Fr) emanating from the left flagellar base is visible. . Section at the level of the Db. Accessory band (Ab4) is present. . Section through one of the flagella at the level of the axosome (Ax). . Section of roots (R1, R2) and their crystalline components (CR1, CR2). Scale bars represent 0.5 µm.
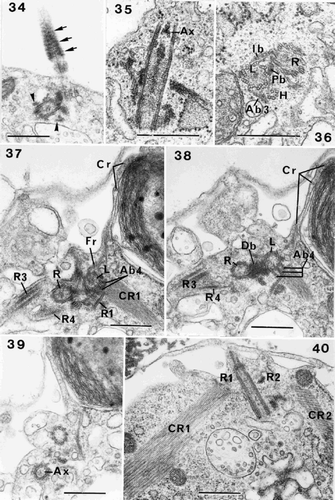
Our observations indicate the presence of three striated bands joining the flagellar bases, as described by Inouye & Pienaar (Citation1985): a proximal band (Pb) (), intermediate band (Ib) joining the flagellar bases on the side opposite to the haptonematal base (, ) and a distal band (Db) (). Accessory connecting fibres, which appear to divide into several bands, link the microtubular plate of R1 to the left flagellar base (, ). They lie at the same level as the Db, and are interpreted as representing the accessory band (Ab4). We have not clearly observed the dense accessory band (Ab1) joining the right flagellar base and the haptonematal base, but the accessory band (Ab3) between the left flagellar base and the haptonematal base (Beech & Wetherbee, Citation1988) is present in O. neapolitana (, , ).
Figs 41–46. Flagellar root structure in Ochrosphaera neapolitana. –. Serial transverse sections of flagellar bases showing partial tangential views of the contractile root (Cr) with interconnecting microfilaments (Mf). . Section showing the right and left flagellar bases linked by the intermediary striated connecting band (Ib); a mitochondrion (m) is visible. . Section nearer the cell surface showing the haptonematal base (H) linked to the left flagellar base by a small non-striated accessory band (Ab3). . Section showing root (R1) in contact with the haptonematal base. Root (R2) shown in longitudinal section with the fibrous root (Fr) also visible. . Crystalline root (CR2) is seen in transverse section, CR1 in longitudinal section, and part of the Cr is also illustrated. , . Serial tangential sections through the sheet of microtubules (Mt) of the Cr. . Section through microtubules (Mt) of Cr, showing their regular spacing and linkage by microfilaments (Mf). . Section showing Cr in close proximity to a mitochondrion. Root (R2) and its crystalline component (CR2) (in longitudinal section) are also visible. Scale bars represent 0.5 µm.
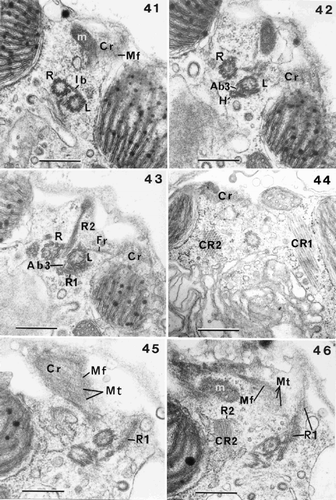
The ‘contractile root’ seen in Pleurochrysis placolithoides Fresnel et Billard (Fresnel & Billard, Citation1991) is also present in O. neapolitana. As in previous cases, it is connected with microtubules of R1, after these have fused with the fibrous root (Fr) that originates at the left flagellar base (, , , , ). The microtubules of the contractile root are regularly spaced, forming a plate that is characterised by the presence of microfilaments in the spaces between the microtubules (, , , , ). Different sections show that this root does not penetrate into the centre of the cell, but is situated just underneath the plasmalemma (, , ). Along part of its length, it runs adjacent to the surface of one of the chloroplasts (, ). Mitochondrial profiles are always visible just beside the contractile root (, ). A three-dimensional diagrammatic reconstruction of the flagellar apparatus of O. neapolitana is presented in .
Figs 47–48. Flagellar root structure in Ochrosphaera neapolitana. , . Serial sections of root (R1) and the microtubular plate, the crystalline root (CR1) and the contractile root (Cr). . Section through the junction of R1 with the fibrous root (Fr), and the localisation of the Cr under the plasmalemma close to the flagellar bases. . Section showing microfilaments joined to the microtubules after the fusion of R1 and Fr. R1 is sectioned transversely. Scale bars represent 0.5 µm.
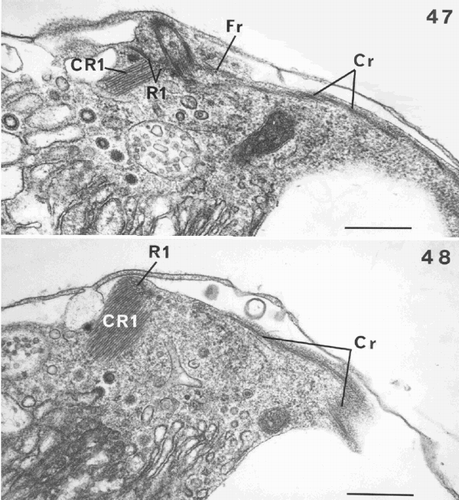
Fig. 49. Diagram showing the flagellar apparatus of Ochrosphaera neapolitana. The position of the accessory bands is approximate relative to the flagellar bases. Left and right flagellum bases (L, R); haptonematal base (H); roots (R1, R2, R3, R4); crystalline components (CR1, CR2); contractile root (Cr); fibrous root (Fr); accessory bands (Ab1, Ab3, Ab4); proximal, distal and intermediate bands (Pb, Db, Ib). Not drawn to scale.
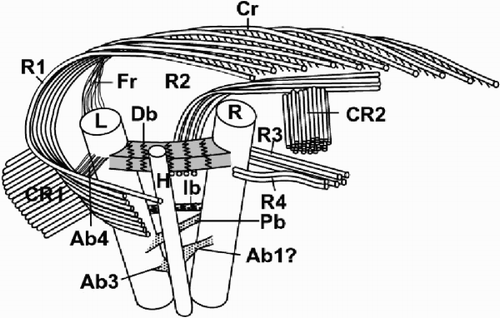
Life cycle (–)
The most common type of reproduction in all strains of O. neapolitana is vegetative division as described above. In strain AC12, which produces tremalith-bearing cells typical of O. neapolitana, the dominant form in long-term culture consists of non-motile, non-calcifying cells embedded in thick mucilage (). The coating stains with ruthenium red, and is formed of concentric mucilaginous layers surrounding each cell (). The cells typically measure 5 µm in diameter (rarely up to 10 µm), and have the same cytological characteristics as the coccolith-bearing cells. While they were originally believed to have simply lost their ability to produce coccoliths, studies on related genera have revealed the significance of scale morphology in digenetic life cycles (e.g. Fresnel, Citation1994) and prompted re-examination of the non-calcifying cells of this strain. In fact, they possess scales with a different pattern compared to the coccolith-bearing cells. Scales are present in several layers immediately outside the plasmalemma and have different patterns on each face. The proximal face has radial microfibrils divided into four sectors, while the distal face is ornamented with microfibrils in tight concentric rings (). These scales do not possess the rim observed on body scales of the coccolith bearing cells, and show greater irregularity in size and shape, being more or less oval, 0.6 – 1.0 × 0.5 – 0.7 µm. A second type of scale was rarely observed, which, by analogy with the genus Hymenomonas (Fresnel, Citation1994), must be situated distal to the first type. The second type is more regular in shape and size (0.6–0.7 µm) and differs by having a rim on both faces and a barely visible concentric pattern on the distal face. These scales resemble coccolith base-plate scales, but are more circular, slightly smaller, and with much more accentuated rims (compare with , ).
Figs 50–57. Life-cycle of Ochrosphaera neapolitana. . Aspects of the S-cell generation. Cells are naked and have the same cytological characteristics as coccolith-bearing cells. . Mucilage coating surrounding S-cells highlighted by staining with ruthenium red. . Contrasting aspects of colonies of each generation grown on agar. C-cell colonies (arrow) are compact and dark in colour; S-cell colonies are more dispersed and lighter. . Tetrad (= meiocyst; compare with ) preceding the reappearance of S-cells. . Naked S-cells released from the meiocyst. . Scales characteristic of the S-cell generation. The most abundant scales are irregular in shape and cover the plasmalemma (basal scales). The proximal faces (bspf) have radial fibres in quadrants and their distal faces (bsdf) have concentric fibres. Both faces of the superficial scales are also seen. The proximal faces (sspf) of these scales have radiating fibres and a thin rim; the distal faces (ssdf) have concentric fibres that are difficult to resolve. , . Metaphase chromosomes fixed and stained with lactopropionic orcein. . Diploid C-cell with 20 ± 1 chromosomes. . Haploid S-cell with 10 ± 1 chromosomes. Scale bars represent 10 µm (, , , ), 50 µm (), 0.5 µm () and 5 µm (, ).
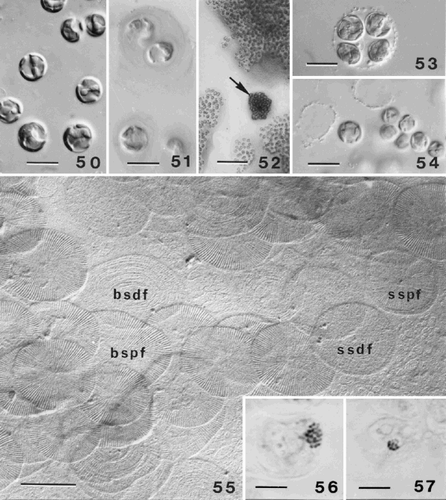
In order to study the two phases of the life cycle an earlier protocol involving cultivation on agar medium, developed to study P. pseudoroscoffensis Gayral et Fresnel (Gayral & Fresnel, Citation1983) was employed. As the culture develops, it is easy to distinguish colonies of the two types of cells. The coccolith-bearing ‘C’ stage forms dark brown colonies consisting of tightly packed cells, while the scale-bearing ‘S’ stage forms colonies of lighter colour with cells clearly separated from each other, due to dispersion of cells in the mucilage (). After isolation and transfer into liquid medium in a watch glass, the development of colonies can be followed under a binocular microscope. The C stage colonies produce swimming cells that rapidly form coccoliths and subsequently divide vegetatively. Tetrads of a different type were observed in pure cultures of C-cells after about ten days (compare and ). These coccospheres liberate small, non-motile, naked cells () that in our opinion may be the result of meiotic division. The naked cells reproduce rapidly and produce the mucilage coating typical of the S generation. The reappearance of C-cells in pure cultures of the S generation, which should be preceded by fusion of two S-cells, was not observed. After isolating the two phases into pure culture, chromosome counts of cells undergoing mitosis indicate that C-cells (20 ± 2 chromosomes) are diploid () and S-cells haploid (10 ± 1 chromosomes) (). In both generations, mitoses most frequently occur in the dark, particularly between 24.00 h and 02.00 h. Despite (or perhaps because of) their small size, the chromosomes of O. neapolitana are much more readily separated by application of gentle pressure on the coverslip than those of the much larger H. lacuna (Fresnel, Citation1994). A schematic representation of the life cycle of O. neapolitana is shown in . This life cycle has a diploid coccolith- and scale-bearing generation alternating with a haploid generation with two types of scales, different to those of the diploid generation. Both generations are capable of vegetative division.
Fig. 58. Schematic representation of the presumed life cycle of Ochrosphaera neapolitana. (a) cellular coating of diploid coccolith-bearing stage; (b) cellular coating of haploid scaly stage. 1. Motile coccolith-bearing (C) cell; 2–4. vegetative division; 5. meiocyst; 6. motile scale-bearing (S) cell; 7. palmelloid S stage and vegetative division; 8. fusion; 9. zygote; 10. formation of C cell inside zygote envelope; 11. liberation of C cell. Note: stages 6, and 8 to 11 are hypothetical.
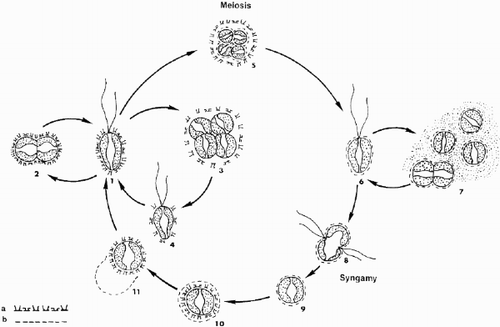
Discussion
Taxonomy
The first author to provide electron microscopic images of Ochrosphaera was West (Citation1969), who attributed the culture strain that he had isolated to O. verrucosa. He described only one type of coccolith: vase-shaped with a distal flange. Soon afterwards, Gayral & Fresnel-Morange (Citation1971) reported organisms with two types of coccoliths, vase- and pulley-shaped, on the same cell as O. neapolitana. Subsequent authors finding Ochrosphaera with two types of coccoliths (Lefort, Citation1975; Inouye & Chihara, Citation1980) should therefore have assigned them to O. neapolitana rather than O. verrucosa. Our study of West's original strain revealed the existence of vari-monomorphic (sensu Jordan et al., Citation1995) coccoliths that were identical to those described for O. neapolitana. Our observations of strains of Ochrosphaera from diverse locations have always revealed such vari-monomorphism. Among these are strains 162 and 466 (designated O. neapolitana and O. verrucosa respectively) of the Plymouth culture collection, a collection whose strains are often used as reference cultures. Both show the same coccolith vari-monomorphism in coccolith-bearing cells, the only difference being the dominance of non-calcifying mucilage-covered cells in strain 162. It seems likely that all work on Ochrosphaera since Schussnig (Citation1930, Citation1940) is on a single species, O. neapolitana. However, Schussnig (Citation1940) clearly observed other organisms when he created two new species, O. verrucosa and O. rovignensis. Considering the excellent line drawings provided (p. 318, ; p. 321, ), it seems probable that his O. verrucosa, with its thick chloroplasts, corresponds to H. globosa, and that O. rovignensis corresponds to H. lacuna. We have found both of these Hymenomonas species together with O. neapolitana in identical habitats along the Mediterranean coast. The binomial O. verrucosa could therefore be a synonym of H. globosa and O. rovignensis of H. lacuna. Since no reference strains exist for these two species, it is impossible to re-examine their coccoliths. We therefore suggest that the use of these two names be abandoned and that they be considered synonyms of H. globosa and H. lacuna, both of which have been thoroughly described in electron microscope studies (Pienaar, Citation1976; Gayral & Fresnel, Citation1976, Citation1979). The conclusion that Ochrosphaera is a monospecific genus may be contradicted by future molecular genetic analyses. If this is the case, then the currently used characters of coccolith morphology will not constitute reliable characteristics upon which species can be distinguished. Even though the two genera are closely related, Hymenomonas and Ochrosphaera should be maintained for the time being due to differences in coccolith morphology. Identical tremaliths are formed from numerous elements with distal points in Hymenomonas, whereas in Ochrosphaera vari-monomorphic tremaliths are formed from a reduced number of elements that do not end in a point (see Gayral & Fresnel, Citation1976).
Flagellar apparatus
In the Prymnesiophyceae, the flagellar basal apparatus is relatively constant, with two variably divergent basal bodies, the left basal body being distinguished from the right basal body by the proximity of the haptonematal base to the left basal body. However, the structure and arrangement of the associated microtubular root components, which were first fully reconstructed in the excellent studies on Pleurochrysis by Inouye & Pienaar (Citation1985) and Beech & Wetherbee (Citation1988), show considerable variability within the Prymnesiophyceae, and may be phylogenetically informative. Four types of flagellar roots are recognised. R1 is proximally associated with the left flagellar base and R2 arises between the left and right basal bodies. Either or both of these roots may have an associated crystalline component. CR1 and CR2 are composed of a varying number of microtubules. R3 is a simple root (i.e. with no crystalline component) that is composed of a limited number of microtubules that bifurcate to either side of the right flagellar base, and hence are usually considered as two separate roots: R3 and R4 (Inouye, Citation1993). In some species, R4 may be absent, e.g. Hymenomonas coronata mills, (Roberts & Mills, Citation1992). Kawachi & Inouye (Citation1994) defined two types of flagellar root systems based on the presence or absence of the secondary crystalline roots associated with R1 and R2. The Pleurochrysis type is characterised by two well-developed crystalline roots, and the Syracosphaera type is for species lacking CR1 and CR2. Sym & Kawachi (Citation2000) reviewed the current state of knowledge on crystalline roots in coccolithophorids. Included in the Pleurochrysis type are Jomonlithus littoralis Inouye et Chihara (Inouye & Chihara, Citation1983), Umbilicosphaera sibogae var. foliosa (Kamptner) Okada et McIntyre (Inouye & Pienaar, Citation1984), H. lacuna (Pienaar, Citation1976) and H. globosa (Fresnel, Citation1989). Intermediary types include Cruciplacolithus neohelis (McIntyre et Bé) Reinhardt that lacks a CR2 (Kawachi & Inouye, Citation1994). Two species lack a CR1: H. coronata (Fresnel, Citation1989; Roberts & Mills, Citation1992) and Calyptrosphaera radiata Sym et Kawachi (Sym & Kawachi, Citation2000). The Syracosphaera type, which at present includes only Syracosphaera pulchra Lohmann (Inouye & Pienaar, Citation1988), is more typical of non-coccolithophorid Prymnesiophycean genera and hence may indicate an early divergence during coccolithophorid evolution. O. neapolitana is clearly of the Pleurochrysis type, with well-developed CR1 and CR2 crystalline roots, although there are fewer microtubules in the R1 than in Pleurochrysis.
R2 in O. neapolitana consists of 4 microtubules, a number that is typical for coccolithophorids (Green & Hori, Citation1994), with the exception of J. littoralis with 5 microtubules (Inouye & Chihara, Citation1983). The first report of a vestigial haptonematal base in a prymnesiophyte lacking an emergent haptonema was for O. neapolitana (Gayral & Fresnel-Morange, Citation1971). The haptonematal base in coccolithophorids (and all other prymnesiophytes) with an emergent haptonema typically comprises at least 8 microtubules whereas vestigial haptonematal bases typically comprise 5 microtubules (H. globosa, Gayral & Fresnel, Citation1976; H. coronata, Fresnel, Citation1989; Roberts & Mills, Citation1992; J. littoralis, Inouye & Chihara, Citation1983; C. neohelis, Fresnel, Citation1986; Kawachi & Inouye, Citation1994). However, there are two exceptions. E. huxleyi lacks any trace of a haptonematal base (Klaveness, 1972a, b), while U. sibogae var. foliosa has a vestigial base formed of 8 microtubules (Inouye & Pienaar, Citation1984).
The notion that part of the compound root (Beech & Wetherbee, Citation1988), possibly formed by the fusion of some R1 microtubules from the fibrous root, that emanates from the left flagellar base, should be considered a ‘contractile root’ was raised by Fresnel & Billard (Citation1991). A contractile function was suggested by Gayral & Fresnel (Citation1983), based on morphological criteria such as a zig-zag structure and the presence of microfilaments linking the microtubules, together with observations of cellular contractions in Pleurochrysis pseudoroscoffensis Gayral & Fresnel. This compound root may be situated within a cytoplasmic tongue (=‘languette cytoplasmique’; Gayral & Fresnel, 1983), a term adopted by various authors, when the root is well developed and traverses the cell as in Pleurochrysis (Beech & Wetherbee, Citation1988; Fresnel & Billard, Citation1991). In many other coccolithophorids and some non-calcifying members of the Prymnesiophyceae, notably the genus Prymnesium Massart ex Conrad (Birkhead & Pienaar, Citation1994; Fresnel et al., Citation2001), this root is typically less well developed and more superficial. In C. neohelis it may nevertheless be situated within a cytoplasmic tongue (Kawachi & Inouye, Citation1994). However, there are situations in which this compound root is not located within a cytoplasmic tongue, e.g. in O. neapolitana (this study) and the closely related H. coronata, in which the structure was termed F1 (Roberts & Mills, Citation1992). An assessment of the nature of the contractile root awaits further analysis, especially in relation to its constituent proteins, and in particular the presence or absence of contractile proteins in the microfilaments that link the microtubules.
Pyrenoid
The fact that the pyrenoids may bulge to such an extent that they abut each other has already been observed in the tremalith-bearing species H. lacuna (Pienaar, Citation1976) and H. coronata (Inouye & Chihara, Citation1980). The stalked basal structure of the pyrenoids of O. neapolitana, which Schwarz (Citation1932) called a ‘pyrenoid holder’ (this was the first mention of the presence of pyrenoids in a coccolithophorid), is unusual and unique among the coccolithophorids.
Coccolithogenesis
In all heterococcolithophorids studied to date, the coccoliths are produced inside the cell within Golgi-derived vesicles. However, as an increasing number of species are examined in detail, variation is becoming apparent in this process. Variations occur in the manner in which components for the construction of the coccolith are transported to the vesicle and in the role of different cell components in shaping the forming coccolith. Morphological and biochemical aspects of coccolith production have been extensively studied in two model coccolithophorid species, Emiliania huxleyi (Lohm-) Hay et Mohler (see review by Paasche, Citation2002) and P. carterae (reviewed by Marsh, Citation1994). The organic base plate within the coccolith production compartment in E. huxleyi is closely apposed to the nuclear membrane and exhibits the same curvature as the nucleus (Klaveness, Citation1972a). A reticular body of anastomosing tubes linked directly to the coccolith vesicle (Klaveness, Citation1972a) may be involved in the transfer of precursor organic and mineral components from the Golgi body, and possibly from other vesicles, to the coccolith vesicle (Westbroek et al., Citation1984). In P. carterae, the vesicle containing the coccolith base-plate scale is moved to a more distal region between the nucleus and the plasma membrane, and numerous Golgi-derived vesicles, which contain densely staining granules termed ‘coccolithosomes’ (Outka & Williams, Citation1971), transport both calcium ions (van der Wal et al., Citation1983 a) and polysaccharides (van der Wal et al., Citation1983 b) to the coccolith vesicle. In U. sibogae var. foliosa, the ER system, nucleus and Golgi body seem to play roles in determining the shape of the coccolith vesicle (Inouye & Pienaar, Citation1984). The manner of coccolith production in O. neapolitana is interesting and currently unique, in that the coccolith vesicle is located at the periphery of the cell where the PER appears to play an important role. The reticular body of E. huxleyi and the distal tubular matrix of O. neapolitana have some morphological characteristics in common since both are tubular structures of endoplasmic reticular origin. The exact function of the PER in haptophytes has not been elucidated, but it is interesting to note that Marsh & Dickinson (Citation1997) suggested that the extensive membrane system of the reticular body in E. huxleyi may transport calcium ions into the lumen of the coccolith vesicle.
Over-calcification
To our knowledge, O. neapolitana is the only coccolithophorid that does not continually produce coccoliths to regenerate the coccosphere, and the only species in which extracellular over-calcification of coccoliths has been observed in culture. The heavily over-calcified pseudocysts observed in O. neapolitana have been reported by several authors (Lepailleur, Citation1969; Parke, Citation1971; Gayral & Fresnel-Morange, Citation1971). Pseudocysts are only formed in cultures containing both the coccolith-bearing phase and the uncalcified phase. The uncalcified phase produces copious quantities of amorphous mucilage, which, although unique among coccolithophorids, is reminiscent of the Isochrysidacean (non coccolith-bearing) genus Chrysotila Anand emend. Green et Parke, which is also characterised by a benthic phase that produces mucopolysaccharides (Green & Parke, Citation1975). Green & Course (Citation1983) considered it unlikely that extracellular calcification in C. lamellosa served the biological function of resting cyst formation, stating that cells enclosed in these structures were probably dead. However, our observations of living cells emerging from O. neapolitana pseudocysts may indicate that they do serve such a biological function, although they have never been reported under natural conditions.
Life cycle
The number of coccolithophorids maintained in culture is limited, and our knowledge of modes of reproduction is consequently fragmentary. Several types of heteromorphic life cycle have nevertheless been reported. Von Stosch (Citation1955, Citation1967) demonstrated an alternation of heterococcolith-bearing cells with scale-bearing pseudofilaments in P. carterae. The majority of cricolith-bearing species have also been shown to share the same life cycle (Leadbeater, Citation1970, Citation1971; Inouye & Chihara, Citation1979; Gayral & Fresnel, Citation1983; Fresnel & Billard, Citation1991). In E. huxleyi, a non-motile, placolith-bearing stage lacking body scales alternates with a flagellate, scale-bearing cell (Klaveness & Paasche, Citation1971; Klaveness, Citation1972b). Parke & Adams (Citation1960) showed that the holococcolithophorid Crystallolithus hyalinus Gaarder et Markali was the flagellated stage of a non-motile placolith-bearing species, Coccolithus pelagicus (Wallich) Schiller. This life cycle type has subsequently been demonstrated for a number of species by the observation of ‘combination cells’ bearing both hetero- and holococcoliths (Thomsen et al., Citation1991; Kleijne, Citation1991; Cros et al., Citation2000). Another life cycle type, involving the alternation of tremalith-bearing cells with scale-bearing cells, was observed in two species of Hymenomonas, both stages being non-motile or motile (Fresnel, Citation1994). The life cycle of O. neapolitana is summarised in . It is identical to that proposed for Hymenomonas and is also characteristic of tremalith-bearing coccolithophorids (see discussion in Fresnel, Citation1994). However, this life cycle contradicts Schwarz's (Citation1932) observations on O. neapolitana. She observed the process of fusion on several occasions, but considered the zygote to be the only diploid stage, with chromosome reduction occurring immediately after fusion. Thus the life cycle was considered to be ‘pure haploid’. Our observations show that both the diploid coccolith-bearing stage and the haploid scale-bearing stage are capable of asexual reproduction, and thus that Schwarz's anomalous conclusions were due to mis-interpretation.
Since the first description of differing scale ornamentation in the two generations of Pleurochrysis (Leadbeater, Citation1970, Citation1971), for which the cycle had previously been shown to be haplo-diplontic (Rayns, Citation1962), there has been an increasing awareness of the significance of this character in describing coccolithophorid life cycles (Fresnel, Citation1994, Billard, Citation1994). The superficial concentric microfibrils and a thin rim on both faces of the body scales of the diploid C-cell phase, and concentric and radiating microfibrils on the distal and proximal unrimmed faces, respectively, of those of the haploid S-cell phase of O. neapolitana are entirely consistent with previous observations. Both types of scale ornamentation were illustrated for O. neapolitana, but were mis-interpreted by Gayral & Fresnel-Morange (Citation1971). Scale ornamentation and chromosome counts from the two phases provide strong evidence for the proposed haplo-diplontic life-cycle of O. neapolitana, although not all stages in the sexual cycle have yet been observed.
Acknowledgements
We would like to thank Jeremy Young and two anonymous reviewers for reading the manuscript and offering many useful suggestions, and Didier Goux and Jacques Prigent for technical assistance with electron microscope studies. This research was partly funded by the EU via the CODENET (Coccolithophorid Evolutionary Biodiversity and Ecology Network) project of the FP4 TMR activity.
References
References
- Balch , WM , Kilpatrick , KA and Trees , CC . 1996 . The 1991 coccolithophore bloom in the central North Atlantic. 1. Optical properties and factors affecting their distribution . Limnol. Oceanogr. , 41 : 1669 – 1683 .
- Beech , PL and Wetherbee , R . 1988 . Observations on the flagellar apparatus and peripheral endoplasmic reticulum of the coccolithophorid Pleurochrysis carterae (Prymnesiophyceae) . Phycologia , 27 : 124 – 158 .
- Billard C 1994 Life cycles In The Haptophyte Algae (Green, J.C. & Leadbeater, B.S.C., editors) 167 186 Systematics Association Special Volume No. 51 Clarendon Press Oxford
- Birkhead , M and Pienaar , RN . 1994 . The flagellar apparatus of Prymnesium nemamethecum (Prymnesiophyceae) . Phycologia , 33 : 311 – 323 .
- Charret , R and Fauré-Frémiet , E . 1967 . Technique de rassemblement de microorganismes . J. Microscopie , 6 : 1063 – 1066 .
- Cosson , J . 1987 . Croissance des sporophytes issus d'hybridations interspécifiques et intergénériques chez les laminaires . Crypto. Algol. , 8 : 61 – 72 .
- Cros , L , Kleijne , A , Zeltner , A , Billard , C and Young , JR . 2000 . New examples of holococcolith-heterococcolith combination coccospheres and their implications for coccolithophorid biology . Mar. Micropaleontol. , 39 : 1 – 34 .
- Dyer , AF . 1963 . The use of lacto-propionic orcein in rapid squash methods for chromosome preparations . Stain. Technol. , 38 : 85 – 90 .
- Edvardsen , B , Eikrem , W , Green , JC , Andersen , RA , Moon-van-der-Staay , SY and Medlin , LK . 2000 . Phylogenetic reconstructions of the Haptophyta inferred from 18S ribosomal DNA sequences and available morphological data . Phycologia , 39 : 19 – 35 .
- Fresnel , J . 1986 . Nouvelles observations sur une coccolithacée rare: Cruciplacolithus neohelis (McIntyre et Bé) Reinhardt (Prymnesiophyceae) . Protistologica , 22 : 193 – 204 .
- Fresnel J 1989 Les coccolithophorides (Prymnesiophyceae) du littoral. Genres: Cricosphaera, Cruciplacolithus, Hymenomonas et Ochrosphaera, ultrastructure, cycle biologique, systématique Ph.D. thesis, Université de Caen 281 pp
- Fresnel , J . 1994 . A heteromorphic life cycle in two coastal coccolithophorids, Hymenomonas lacuna and Hymenomonas coronata (Prymnesiophyceae) . Can. J. Bot. , 72 : 1455 – 1462 .
- Fresnel , J and Billard , C . 1991 . Pleurochrysis placolithoides sp. nov. (Prymnesiophyceae) a new marine coccolithophorid with remarks on the status of cricolith-bearing species . Br. Phycol. J. , 26 : 67 – 80 .
- Fresnel , J , Probert , I and Billard , C . 2001 . Prymnesium faveolatum sp. nov. (Prymnesiophyceae), a new toxic species from the Mediterranean sea . Vie et Milieu , 51 : 89 – 97 .
- Fujiwara , S , Tsuzuki , M , Kawachi , M , Minaka , N and Inouye , I . 2001 . Molecular phylogeny of the Haptophyta based on the rbcL gene and sequences variation in the spacer region of the rubisco operon . J. Phycol. , 37 : 121 – 129 .
- Gayral , P and Fresnel , J . 1976 . Nouvelles observations sur deux Coccolithophoracées marines: Cricosphaera roscoffensis (P. Dangeard) comb. nov. et Hymenomonas globosa (F. Magne) comb. nov. . Phycologia , 15 : 339 – 355 .
- Gayral , P and Fresnel , J . 1979 . Révision du genre Hymenomonas Stein à propos de l'étude comparative de deux Coccolithacées: Hymenomonas globosa (Magne) Gayral et Fresnel et Hymenomonas lacuna Pienaar . Rev. Algol. , 2 : 117 – 125 .
- Gayral , P and Fresnel , J . 1983 . Description, sexualité et cycle de développement d'une nouvelle coccolithophoracée (Prymnesiophyceae): Pleurochrysis pseudoroscoffensis sp. nov. . Protistologica , 19 : 245 – 261 .
- Gayral , P and Fresnel-Morange , J . 1971 . Résultats préliminaires sur la structure et la biologie de la Coccolithacée Ochrosphaera neapolitana Schussnig . C. R. Acad. Sci. Paris , 273 : 1683 – 1686 .
- Green , JC and Parke , M . 1975 . New observations upon members of the genus Chysotila Anand, with remarks upon their relationships within the Haptophyceae . J. Mar. Biol. Ass. UK , 55 : 109 – 121 .
- Green , JC and Course , PA . 1983 . Extracellular calcification in Chrysotila lamellosa (Prymnesiophyceae) . Br. Phycol. J. , 18 : 367 – 382 .
- Green JC Hori T 1994 Flagella and flagellar roots In The Hatophyte Algae (Green J.C. & Leadbeater, B.S.C., editors) 47 71 Systematics Association Special Volume No. 51 Clarendon Press Oxford
- Inouye I 1988 Microalgae of mangroves and characteristics of the community In Biological of Mangroves A report of east Indonesian mangrove expedition, 1986 (3) (Ogino K. & Chihara M., editors) 35 43 Ehime University Matsuyama Japan
- Inouye I 1993 Flagella and flagellar apparatus of algae In Ultrastructure of Microalgae (Berner, T., editor) 99 133 CRC Press Boca Raton Florida
- Inouye , I and Chihara , M . 1979 . Life history and taxonomy of Cricosphaera roscoffensis var. haptonemofera, var. nov. (Class Prymnesiphyceae) from the Pacific . Bot. Mag. Tokyo , 92 : 75 – 87 .
- Inouye , I and Chihara , M . 1980 . Laboratory culture and taxonomy of Hymenomonas coronata and Ochrosphaera verrucosa (Class Prymnesiophyceae) from the Northwest Pacific . Bot. Mag. Tokyo , 93 : 195 – 208 .
- Inouye , I and Chihara , M . 1983 . Ultrastructure and taxonomy of Jomonlithus littoralis gen. et sp. nov. (Class Prymnesiophyceae), a coccolithophorid from the Northwest Pacific . Bot. Mag. Tokyo , 96 : 365 – 376 .
- Inouye , I and Pienaar , RN . 1984 . New observations on the coccolithophorid Umbilicosphaera sibogae var. foliosa (Prymnesiophyceae) with reference to cell covering, cell structure and flagellar apparatus . Br. Phycol. J. , 19 : 357 – 369 .
- Inouye , I and Pienaar , RN . 1985 . Ultrastructure of the flagellar apparatus in Pleurochrysis (Class Prymnesiophyceae) . Protoplasma , 125 : 24 – 35 .
- Inouye , I and Pienaar , RN . 1988 . Light and electron microscope observations of the type species of Syracosphaera, S. pulchra (Prymnesiophyceae) . Br. Phycol. J. , 23 : 205 – 217 .
- Jordan , RW , Kleijne , A , Heimdal , BR and Green , JC . 1995 . A glossary of the extant Haptophyta of the world . J. Mar. Biol. Assoc. UK , 75 : 769 – 814 .
- Kawachi , M and Inouye , I . 1994 . Observations on the flagellar apparatus of a coccolithophorid, Cruciplacolithus neohelis (Prymnesiophyceae) . J. Plant. Res. , 107 : 53 – 62 .
- Klaveness , D . 1972a . Coccolithus huxleyi (Lohmann) Kamptner. I. – Morphological investigations on the vegetative cell and the process of coccolith formation . Protistologica , 8 : 335 – 346 .
- Klaveness , D . 1972b . Coccolithus huxleyi (Lohmann) Kamptner. II. – The flagellate cell, aberrant cell types, vegetative propagation and life cycles . Br. Phycol. J. , 7 : 309 – 318 .
- Klaveness , D and Paasche , E . 1971 . Two different Coccolithus huxleyi cell types incapable of coccolith formation . Arch. Mikrobiol. , 75 : 382 – 385 .
- Kleijne , A . 1991 . Holococcolithophorids from the Indian Ocean, Red Sea, Mediterranean Sea and North Atlantic Ocean . Mar. Micropaleontol. , 17 : 1 – 76 .
- Leadbeater , BSC . 1970 . Preliminary observations on differences of scale morphology at various stages in the life cycle of Apistonema-Syracosphaera sensu Von Stosch . Br. Phycol. J. , 5 : 57 – 69 .
- Leadbeater , BSC . 1971 . Observations on the life-history of the haptophycean algae Pleurochrysis scherffelii with special reference to the micro-anatomy of the different types of motile cells . Annals Bot. , 335 : 429 – 439 .
- Lefort , F . 1971 . Sur l'appartenance à une seule et même espèce de deux Coccolithophoracées, Cricosphaera carterae et Ochrosphaera verrucosa . C. R. Acad. Sci. Paris , 272 : 2540 – 2543 .
- Lefort , F . 1975 . Étude de quelques Coccolithophoracées marines rapportées aux genres Hymenomonas et Ochrosphaera . Cahiers de Biologie Marine , 16 : 213 – 229 .
- Lepailleur H 1969 Etude écologique, biologique et systematique de quelques Chrysophycées marines Masters Thesis, Université de Caen 132 pp
- Magne , F . 1952 . Sur la cytologie d'une Chrysophycée marine vivant en aquarium, Ochrosphaera neopolitana Schussnig . Rev. Gén. Bot. , 59 : 240–
- Marsh , ME . 1994 . Polyanion-mediated mineralization-assembly and reorganization of acidic polysaccarides in the Golgi system of a coccolithophorid alga during mineral deposition . Protoplasma , 177 : 108 – 122 .
- Marsh , ME and Dickinson , DP . 1997 . Polyanion-mediated mineralization in coccolithophore (Pleurochrysis carterae) variants which do not express PS2, the most abundant and acidic mineral-associated polyanion in wild-type cells . Protoplasma , 199 : 9 – 17 .
- Norris , RE . 1967 . Microalgae in enrichment cultures from Puerto Penasco, Sonora, Mexico . Bull. South Calif. Acad. Sci. , 66 : 250–
- Outka , DE and Williams , DC . 1971 . Sequential coccolith morphogenesis in Hymenomonas carterae . J. Protozool. , 18 : 297–
- Paasche , E . 2002 . A review of the coccolithophorid Emiliania huxleyi (Prymnesiophyceae), with particular reference to growth, coccolith formation, and calcification-photosynthesis interactions . Phycologia , 40 : 503 – 529 .
- Parke M 1971 The production of calcareous elements by benthic algae belonging to the class Haptophyceae (Chrysophyta) In Proc. II Planktonic Conference, Roma, 1970 (Farinacci, G., editor) 929 937 Edizioni Technoscienza Rome
- Parke , M and Adams , I . 1960 . The motile (Crystallolithus hyalinus Gaarder & Markali) and non-motile phases in the life history of Coccolithus pelagicus (Wallich) Schiller . J. Mar. Biol. Assoc. UK , 39 : 263 – 274 .
- Pienaar , RN . 1976 . The micronanatomy of Hymenomonas lacuna sp. nov. (Haptophyceae) . J. Mar. Biol. Assoc. UK , 56 : 1 – 11 .
- Rayns , DG . 1962 . Alternation of generations in a coccolithophorid, Cricosphaera carterae (Braarud et Fagerland.) Braarud . J. Mar. Biol. Ass. UK , 42 : 481 – 484 .
- Roberts , KR and Mills , JT . 1992 . The flagellar apparatus of Hymenomonas coronata (Prymnesiophyta) . J. Phycol. , 28 : 642–
- Schussnig , B . 1930 . Ochrosphaera neapolitana, nov. gen., nov. sp., eine neue Chrysomonade mit kalkhülle . Österr. Bot. Z. , 79 : 170–
- Schussnig , B . 1940 . Über einige neue Protophyten aus der Adria . Arch. Protistenk. , 93 : 330–
- Schwartz , E . 1932 . Beiträge zur Entwicklungsgeschichte der Protophyten . Arch. Protistenk. , 77 : 462–
- Sym , S and Kawachi , M . 2000 . Ultrastructure of Calyptrosphaera radiata, sp. nov. (Prymnesiophyceae, Haptophyta) . Eur. J. Phycol. , 35 : 293–
- Thomsen , HA , Ostergaard , JB and Hansen , LE . 1991 . Heteromorphic life histories in Arctic coccolithophorids (Prymnesiophyceae) . J. Phycol. , 27 : 642–
- van der Wal , P , de Jong , EW , Wesbroeck , P and de Bruijn , WC . 1983a . Calcification in the coccolithophorid alga Hymenomonas carterae . Ecol. Bull. (Stockholm) , 35 : 251 – 258 .
- van der Wal , P , de Jong , EW , Westbroek , P , de Bruijn , WC and Mulder-Stapel , AA . 1983b . Polysaccharide localization, coccolith formation and Golgi dynamics in the coccolithophorid Hymenomonas carterae . J. Ultrastruct. Res. , 85 : 158–
- von Stosch , HA . 1955 . Ein morphologischer Phasenwechel bei einer Coccolithophoride . Die Naturwissenschaften , 4 : 1 – 2 .
- von Stosch HA 1967 Haptophyceae In Vegetative Fortpflanzung, Parthenogenese und Apogamie bei Algen. Encyclopedia of plant physiolgy (Ruhland, W., editor) 646 656 Springer-Verlag Berlin
- West , JA . 1969 . Observations on four rare marine microalgae from Hawaii . Phycologia , 8 : 187 – 192 .
- Westbroek , P , de Jong , EW , van der Wal , P , Borman , AH , de Vrind , JPM , Kok , D , de Bruijn , WC and Parker , SB . 1984 . Mechanism of calcification in the marine alga Emiliania huxleyi . Phil. Trans. R. Soc. Lond. , 304 : 444–