Abstract
Frustule structure and symmetry, protoplast morphology, and auxosporulation are described for the raphid diatom genus Petroneis, separated from Navicula in 1990. Several features, such as the constant cis symmetry of frustules, flap-like occlusions of the areolae, the opening of the central external raphe endings into a ‘tear-drop’–like groove, valve-appressed plastids, secretion of mucilage capsules during vegetative cell division, and aspects of sexual reproduction, suggest a close relationship between Petroneis and Lyrella; this is supported by a parsimony analysis. Molecular phylogenetic analysis of selected raphid diatoms, based on rbcL sequences, also indicates that Petroneis and Lyrella are sister genera and supports their separation into the family Lyrellaceae, order Lyrellales. This group is ancient, since Lyrella is known from the Palaeocene.
Introduction
The genus Petroneis was established by Stickle & Mann (Round et al., Citation1990) for 13 species of raphid diatom previously included in Navicula Bory. Five of the 13 have been reported in the British Isles (Hartley, Citation1986) and these form the focus of this paper. Since 1990, a few further species have been transferred to Petroneis, including P. glacialis (Cleve) Witkowski, Lange-Bertalot & Metzeltin and P. xi (Cleve) Witkowski, Lange-Bertalot & Metzeltin (Witkowski et al., Citation2000).
Petroneis species were originally described as Navicula because of their naviculoid shape and central raphe, and Cleve (Citation1895) grouped them (together with some species we regard as unrelated) in Navicula section Punctatae, because of their coarsely punctate striae. However, Petroneis species differ from Navicula sensu stricto (the section Lineolatae of earlier authors, e.g. Cleve (Citation1895), and Navicula subgenus Navicula of Patrick & Reimer (Citation1966)) in many respects, including aspects of valve, raphe, and girdle structure, and chloroplast morphology. The difference in chloroplast type was recognised by Mereschkowsky (Citation1902–3), who removed Petroneis species into a genus called Clevia. However, this genus was not accepted by subsequent authors and in any case it is a later homonym of Cleveia Pantocsek (Fourtanier & Kociolek, Citation1999). Thus, it was not until 1990, when Stickle and Mann (Round et al., Citation1990) created the genus Petroneis, that the differences between members of Petroneis and Navicula sensu stricto were given full recognition.
Round et al. (Citation1990) suggested that Petroneis is closely related to the genus Lyrella Karayeva, another group of marine diatoms that has been removed from Navicula (Karayeva, Citation1978), in this case from Navicula section Lyratae (Cleve, Citation1895). (It should be noted, however, that the Lyratae and Karayeva's concept of Lyrella also included species that are now classified in the genus Fallacia Stickle & D.G. Mann (see Round et al., Citation1990) which we believe to be only distantly related to Lyrella. Mereschkowsky (Citation1902–3) also recognised similarities between species now allocated to Petroneis and Lyrella; his genus Clevia consisted of members of both genera. Round et al. (Citation1990) and Mann & Stickle (Citation1993) noted that several features of frustule and protoplast structure are shared by Petroneis and Lyrella – for example, their frustules always have cis symmetry (i.e. the primary halves of both valves are on the same side of the cell, not diagonally opposite), and they both have chloroplasts that are appressed to the valves and flap-like (volate) pore occlusions – and they proposed that the two genera should be brought together as the only two members of the family Lyrellaceae, order Lyrellales. However, the evidence for this was not given in any detail.
As part of the abortive British Marine Diatom Flora project (1991–4), we searched for all Petroneis species occurring in the British Isles. The aim was to determine the valve and protoplast structure of each species by examining populations from sites around Great Britain and to use this information to provide a detailed description of Petroneis, allowing comparisons between Petroneis and other genera, such as Lyrella and Navicula sensu stricto. There has been a long delay before publication, which has had the sole advantage that we have now been able to undertake a molecular systematic investigation of Petroneis humerosa (Brébisson ex W. Smith) Stickle & D.G. Mann, the type species of Petroneis (Round et al., Citation1990), and selected other raphid diatoms, to probe whether the morphological similarities between Petroneis and Lyrella reflect recent common ancestry or homoplasy.
Material and methods
Sampling and microscopy
Petroneis valves were examined in slides of acid-cleaned material (for methods, see Mann & Stickle (Citation1995)) from samples collected around the coastline of Great Britain (during the British Marine Diatom Flora project), and in slides from existing collections held at the Royal Botanic Garden, Edinburgh (). Wherever possible, a minimum of 25 valves of a given Petroneis species were examined from each sample, and locations were selected to represent a range of sites around the British Isles. Only one valve of P. granulata (Bailey) D.G. Mann was observed – the type specimen, loaned from the Farlow Herbarium, Harvard. Although this species is reported from the British Isles (Hartley, Citation1986), it did not appear in our samples.
Table 1 . Slides and locations studied
Living cells of Petroneis were examined in samples of fresh intertidal sand collected from a number of sites, predominantly in Scotland. Preparation of material suitable for light microscopy was as follows. The sand was allowed to settle in boxes for c. 8–12 h, after which the supernatant water was removed and a layer of lens tissue placed on the sand surface. Large (24 × 50 mm) glass cover-slips were placed on the surface of the tissue, removed after one to two days, and mounted immediately in seawater for microscopical examination. Evaporation of water from the slides was prevented by ringing the cover-slip with petroleum jelly (see also Mann & Stickle, Citation1993).
Light micrographs were taken with a Reichert Polyvar photomicroscope, using Kodak Technical Pan film rated at ISO 50 and developed with Kodak HC110 developer (dilution D). Bright field (BF) optics were generally used for acid cleaned material, and differential interference contrast (DIC) optics for living material. Live cells were photographed using green light (however, we would now recommend yellow or orange filters), to reduce contrast between the chloroplasts and other cell components, and a higher film rating (half a stop) to compensate for the slightly lower sensitivity of the film at green wavelengths (Kodak technical film information: http://www.uk.kodak.com/cluster/global/en/professional/support/techPubs/p255/p255.jhtml#04).
Acid-cleaned material was also examined using scanning electron microscopy (SEM). Approximately 500 µl of material suspended in water was dried at room temperature onto a glass cover-slip (13 mm diameter), which had been glued to a SEM stub and coated with gold–palladium. The loaded cover-slip was coated again with gold–palladium and examined using a Zeiss 952 scanning electron microscope at 20 kV. Photographs were taken using Technical Pan film developed with Technidol developer.
Cultures and DNA extraction
New clonal cultures were established from UK samples (except Encyonema cf. sinicum and Seminavis cf. robusta: ). Single cells were isolated by micropipette, initially into small volumes of medium in the wells of Repli dishes, and grown as monoclonal cultures in Petri dishes in WC (freshwater: Guillard & Lorenzen, Citation1972) or R medium (marine: Roshchin, Citation1994; see also Mann & Chepurnov, Citation2004) under low light (<10 µmol photons m−2 s−1) at 15–18°C under 14:10 or 12:12 h light:dark. Cells were harvested by scraping them from the bottom of the dish using a sterile cell scraper and the resulting slurry of cells was centrifuged at 2500 × g for 10–20 min. The pellet was resuspended in 0.5 ml of preheated 2 × cetyltrimethylammonium bromide (CTAB) and the frustules were broken by shaking at 3800 rpm for 1–1.5 min in the presence of 5–10 glass minibeads (0.5 mm diameter). DNA was extracted according to Doyle & Doyle (Citation1987) and resuspended in 25–50 µl TE buffer.
Table 2 . Culture sources, voucher material and molecular sequence data
PCR, sequencing and aligning
The forward and reverse primers used were modified from Daugbjerg & Andersen (Citation1997) (DPrbcL1: AAGGAGAAATHAATGTCT and DPrbcL7: AARCAACCTTGTGTAAGTCTC) and amplified a region of approximately 1550 bp, covering the rbcL gene and the spacer region between rbcL and rbcS. The reaction volume was 50 µl and contained PCR buffer (67 mM Tris-Cl, pH 8.8; 16 mM (NH4)2SO4; 0.01% Tween-20), 200 µM dNTP, 0.3 µM of each primer, 2.5 units Taq, and 2.5 mM MgCl2 (BIOTAQ™ DNA polymerase; Bioline, UK). PCR conditions were an initial denaturing phase for 3 min (94°C), followed by 30–40 cycles of 94°C for 1 min, 55°C for 1 min and 72°C for 1.5 min, with a final extension of 72°C for 5 min. The resultant DNA fragment was cleaned using QIAquick PCR purification kit (QIAgen, Dorking, Surrey, UK) and the concentration was estimated by electrophoresis on a 1% agarose gel. PCR fragments were sequenced directly using a ThermoSequenase II dye terminator cycle sequencing premix kit (Amersham Pharmacia Biotech) and analysed on an ABI Prism 377 prism automatic DNA Sequencer (PE Biosystems, Inc., Warrington, UK). The sequencing primers used were DPrbcL1 and DPrbcL7 (PCR primers), a number of internal primers (NDrbcL2, NDrbcL3, NDrbcL5, NDrbcL6, NDrbcL11, NDrbcL12 and NDrbcL13: Daugbjerg & Andersen Citation1997), and some designed in-house, specifically for diatom rbcL sequences
(14R: GAATACGCATATCTTCTAAACG;
15R: ACACCAGACATACGCATCCA;
16F: TTAGAAGATATGCGTATT;
17R: TGACCAATTGTACCACC;
18R: AATCAGCTCTATCTGTAG). Because rbcL is a translated protein, the gene sequences across taxa were easily aligned in SeqPup 0.6f (Gilbert, Citation1995) and an area of 1297 bp within the rbcL gene region was used for comparative analyses.
We have been assembling a large data-set of rbcL sequences (Mann et al., Citation2001), designed to complement the 18S rDNA data assembled largely by Medlin and colleagues (e.g. Medlin & Kaczmarska, Citation2004). From the larger rbcL data-set, we abstracted data to examine relationships between a selected small number of taxa. These comprised Petroneis and Lyrella, because these have been suggested to be closely related (Round et al., Citation1990); Placoneis Mereschkowsky, which resembles Petroneis in several aspects of frustule structure (Round et al., Citation1990); Gomphonema C. Agardh and Encyonema Kützing, which were suggested to be allied to Placoneis by Mereschkowsky (Citation1903), Round et al. (Citation1990) and Mann & Stickle (Citation1995); Navicula, because this was the original placing of Petroneis species; Pseudogomphonema Medlin in Medlin & Round and Seminavis D.G. Mann in Round et al., as non-naviculoid diatoms that share many structural characteristics with Navicula; and Sellaphora Mereschkowsky, as a further naviculoid lineage whose cytological, reproductive, and cell wall characteristics are particularly well understood (e.g. Mann, Citation1989a; Round et al., Citation1990). Clearly, it would have been important to include representatives of many other raphid genera if the aim were to establish the exact systematic position of Petroneis within the raphid diatoms. However, the purpose of the current analysis was primarily to test the hypotheses that (i) Round et al. (Citation1990) were correct to separate Petroneis from Navicula; (ii) Lyrella and Petroneis are closely related and might belong to the same family; and (iii) Petroneis and Lyrella are more closely related than either are to Placoneis. Given such aims, a small selective data-set is appropriate. It would have been good to include other Petroneis species in the molecular analysis, but we have been unable to grow any in culture. Fortunately, the one species we were able to isolate and grow, P. humerosa, is the generitype.
GenBank sequences of the centric diatoms Rhizosolenia setigera Brightwell and Thalassiosira nordenskioeldii Cleve were added as outgroups and Eunotia cf. minor was also included, as a possible sister group to all other raphid diatoms.
Maximum parsimony (MP) analyses were performed using branch-and-bound searches on PAUP 4.0b10 (Swofford, Citation2002), ensuring that all most parsimonious trees are found. Branch support was established by heuristic bootstrap analysis using 10,000 replicates of random additions (TBR on, Multrees off, steepest descent off). Maximum likelihood analyses were calculated using constraints determined by Modeltest Version 3.06 (Posada & Crandall, Citation1998), using the GTR + I + G best-fit model suggested by the Akaike model (proportion of invariable sites = 0.5494; substitution rates A ↔ C = 0.8891, A ↔ G = 2.5043, A ↔ T = 2.7557, C ↔ G = 1.0308, C ↔ T = 6.1235; assumed nucleotide frequencies A = 0.2950, C = 0.1584, G = 0.2069, T = 0.3397; gamma shape parameter α = 0.9171). An heuristic ML search was performed in PAUP (100 bootstrap replicates) to find the relative support for internal branches.
Since rbcL is a protein coding gene and a high saturation of third base substitution was observed (data not shown), Bayesian analysis was used to enable separate substitution rates for first, second and third base codon positions. MRBAYES v. 3.0b4 (Huelsenbeck & Ronquist, Citation2001) was set to use the General Time Reversible (GTR) model with gamma distribution (as determined by Modeltest). One million generations were performed with a burn-in of 1000, saving trees every 100 generations. A consensus of 9000 trees were produced.
Morphological cladistics
At the suggestion of a reviewer, rather than simply including morphological data in the form of a simple, comparative table (cf. ), we compiled a matrix of frustule, protoplast and sexual characteristics for cladistic analysis (). Ideally, representatives of all the main lineages of raphid diatoms would have been included. Unfortunately, the choice of taxa is severely restricted by the availability of data for protoplast, girdle and sexual characters and we were not even able to include all the taxa covered by Cox & Williams (Citation2000), who made a cladistic analysis of a similarly incomplete but different set of naviculoid genera, which included Petroneis as an outgroup. For our analysis, we selected species as terminal taxa that probably belong to the same clades as the taxa included in our rbcL analysis and that have been particularly well studied with respect to all aspects of morphology, cell structure and life history characteristics. Further information on some of the genera to which these taxa belong is given in a more readable format in (see Discussion). We also included a few species (Dickieia ulvacea Berkeley ex Kützing, Anomoeoneis sphaerophora Pfitzer, Stauroneis phoenicenteron (Nitzsch) Ehrenberg and Craticula cuspidata (Kützing) D.G. Mann) that were included in Cox & Williams’ study (except that, instead of C. cuspidata, Cox & Williams had the very closely related C. ambigua (Ehrenberg) D.G. Mann & Stickle) but for which we had no molecular data.
Table 3 . Character matrix of frustule, protoplast and reproductive characteristics of selected raphid species, for cladistic analysis.a Due to incomplete data for any particular species that might be used as a terminal taxon, the outgroup is a genus (Eunotia) with alternative states for two characters. The polymorphism of Rhoicosphenia curvata (Kützing) Grunow for auxospore caps may be genuine or may reflect different specimen preparation methods: Cholnoky (Citation1927) observed caps in stained material, but Geitler (Citation1952a) and Mann (Citation1982b) did not see them in live material
Table 4 . Frustule, protoplast and reproductive characteristics of selected genera of raphid diatoms (principally those included in the rbcL analysis) for comparison with Petroneis and Lyrella a
Several of the characters used by Cox & Williams (Citation2000) were inapplicable, and our coding of other characters was simpler than theirs, with fewer multistate characters (only two), which were treated as unordered. Two characters need brief comment, namely dorsiventrality and pore occlusions. We used a very simple characterisation of lateral symmetry, classifying diatoms into those that have a dorsiventral outline and those that are bilaterally symmetrical. In doing so, we have undoubtedly over-simplified the variation pattern, because dorsiventrality can be of two kinds. In one, present in Cymbella C. Agardh and Seminavis, the less convex ‘ventral’ side is formed by the ontogenetically primary side of the valve, whereas in the other, present in Encyonema, the primary side is dorsal (Mann, Citation1981b). We left this distinction out of our analysis because it would have added an uncertainty to the scoring of the outgroup (because the interpretation of the raphe system in Eunotia Ehrenberg is not yet clear) and because the homology of the dorsal and ventral sides was something we wished to try to test via the analysis. We also treated pore occlusions simply. Mann (Citation1981a) distinguished between two principal types of ultimate pore occlusion in raphid diatoms: (1) sieve-like membranes with very fine (5–10 nm diameter) perforations, which were termed hymenate pore occlusions, and (2) flap-like structures – ‘volate pore occlusions’ – which extend out from the pore wall or from the bars of cribra to leave a narrow, curved or straight slit for communication between exterior and interior. There is no doubt that the dichotomy between ‘hymenate’ and ‘volate’ occlusions is crude and does not reflect the diversity of pore occlusions found within the ‘volate’ group, which vary from simple external reniform flaps in some Gomphonema species to the complex internal cribrum-like structures present in Petroneis (Mann, Citation1981a). Nevertheless, the distinction between hymenate and volate occlusions seems to have some evolutionary significance, since genera and families are generally characterised by one or other type of occlusion. Cox (Citation2004) argues that the different types of flap-like pore occlusion may not be homologous and should not be referred to using the same terms (and also that ‘vola’ may not be appropriate anyway, given its original definition by Ross & Sims, Citation1972). However, because homology is unclear and requires testing, we kept the character coding simple and did not try to distinguish different types of occlusion within the hymenate or volate groups, many of which would then have been restricted to only one taxon among those considered (and would therefore have appeared as autapomorphies).
The morphology matrix was analysed using PAUP 4.0b10 (Swofford, Citation2002) and eleven most-parsimonious trees were found using an exhaustive branch-and-bound search. For the bootstrap analysis, the number of replicates was 100. MacClade version 3.07 (Maddison & Maddison, Citation1997) was used to examine character state transformations in an exemplar tree.
Results
Species occurring in the British Isles
According to Hartley (Citation1986; and see also Hartley et al., Citation1996), only five species of Petroneis occur in the British Isles, viz. P. granulata, P. humerosa (Brébisson ex W. Smith) Stickle & D.G. Mann, P. latissima (Gregory) Stickle & D.G. Mann, P. marina (Ralfs in Pritchard) D.G. Mann and P. monilifera (Cleve) Stickle & D.G. Mann (all were listed by Hartley under Navicula). After exhaustive searches of many slides ( and many other samples collected as part of the British Marine Diatom Flora project), in which we were deliberately searching for new records (and in which we succeeded in adding new records in other genera, e.g. Lyrella opuntioides (Simonsen) Witkowski), we cannot add to this total. Williams et al. (Citation1988) also list P. arabica (Grunow ex A. Schmidt) D.G. Mann, but unless a voucher specimen exists, this record must be regarded as non-proven. Our inability to add to Hartley's list of Petroneis species, despite a long search, may be significant in relation to the controversial claim by Finlay (e.g. Citation2002) that all diatom species are ubiquitous.
The circumscriptions of the five species are currently uncontentious and descriptions are given by Hustedt (Citation1927–66). However, our studies suggest that, although each species may be a natural grouping, some, e.g. P. humerosa, are heterogeneous, varying significantly in outline, size and stria pattern, and may consist of several sibling species (cf. Sellaphora pupula (Kützing) Mereschkowsky: Mann, Citation1989b; Behnke et al., Citation2004).
Frustule morphology
Valves are either broadly linear with a slight central constriction and short, blunt to rostrate poles (P. humerosa and P. monilifera), or elliptical (P. latissima), or lanceolate with undifferentiated poles (P. marina and P. granulata) (). As in many pennate diatoms, the large post-auxospore valves are simpler in shape than the valves formed subsequently, being elliptical, without central constrictions or a distinctive pole shape (). The central constrictions and rostrate poles develop as the size reduction cycle progresses and their presence in smaller valves of P. humerosa and P. monilifera, and to a lesser extent in P. latissima, is therefore an ontogenetic trait separating these species from P. marina or P. granulata, where the ‘juvenile’ shape is retained throughout the life cycle. It remains to be determined whether P. marina and P. granulata share the ancestral condition for the genus (the development of rostrate poles and central constrictions would then be apomorphies within Petroneis), or have evolved through neoteny. The valve face is flat and curves gently into the mantles, which are relatively shallow (, , , ), as is the girdle (, ), so that both cells and valves almost always lie in valve view.
Figs 1–14. Petroneis species found in the British Isles. LMs of acid-cleaned material, showing size and shape variation in vegetative populations. –. P. humerosa. –. P. marina. –. P. monilifera. . P. granulata. Only one valve of this species was observed. –14. P. latissima, including a post-auxospore valve (), in which valve outline and pole shape are simpler than smaller valves in the size reduction cycle (–13). Scale bar (in ) represents 50 µm.
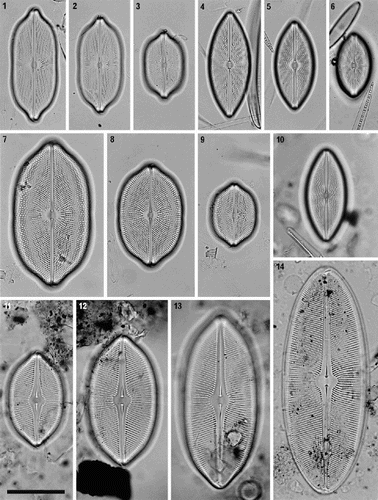
Figs 15–20. External valve structure of Petroneis species, acid cleaned material, SEM. . P. humerosa: exterior of valve. . P. marina: exterior of intact frustule. . P. humerosa: pole and hooked polar raphe ending. . P. marina: pole, showing three girdle bands (1–3) and polar raphe endings in an intact frustule. Note how the terminal raphe fissure descends close to the edge of the valve, interrupting the areola pattern, in contrast to P. humerosa, P. latissima and P. monilifera. . P. monilifera: spathulate groove containing the central raphe endings and central area. Note that the cribra are visible within the large areolae, in contrast to P. humerosa, P. marina and P. latissima. . P. marina: T-shaped central raphe endings and central area. Scale bars represent 10 µm (, ) or 2 µm (–).
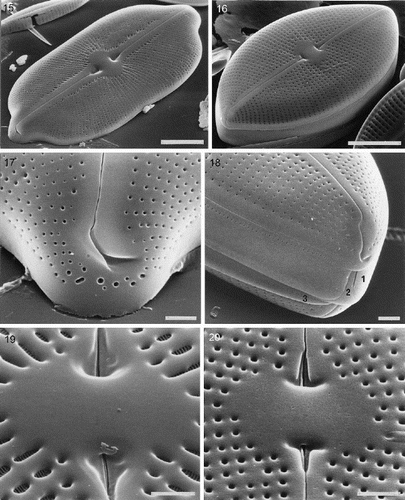
Figs 21–22. External valve structure of Petroneis species, acid cleaned material, SEM. . P. monilifera frustule showing three porous girdle bands (1–3), with open ends arranged alternately. The valvocopula has a single series of poroids, whereas the other two bands have a double row of poroids. . P. latissima: pole and hooked polar raphe ending. Note the similarity to P. humerosa (). Scale bars represent 10 µm () or 5 µm ().
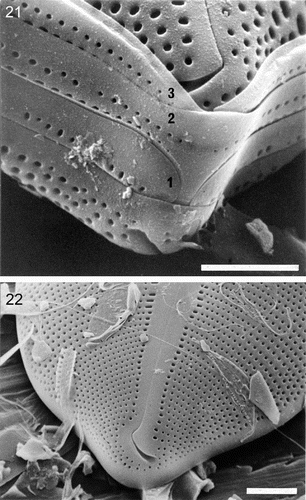
Figs 23–29. Internal valve structure of Petroneis species, acid cleaned material, SEM. . P. humerosa, whole valve interior. . P. marina, whole valve interior. . P. humerosa: internal structure of pole, where the raphe terminates in a helictoglossa. –. Internal views of the central area, showing hooked raphe endings and volate occlusions of the areolae in P. humerosa (), P. latissima (), P. monilifera () and P. marina (). Scale bars represent 10 µm (, ) or 2 µm (–).
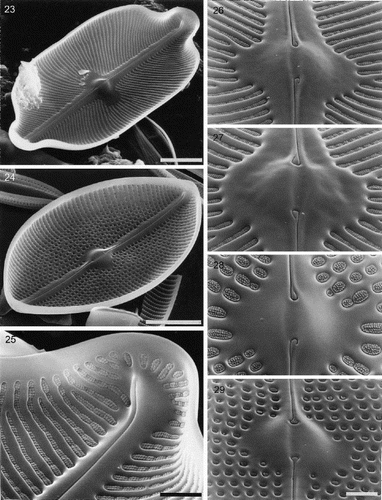
The axial area (raphe-sternum) is linear to linear-lanceolate (P. humerosa and P. monilifera), lanceolate (P. latissima), or bilanceolate (P. marina and P. granulata). The central area is round to transapically elliptical (). Voigt discontinuities are frequently visible, marking the later-formed, ‘secondary’ side of the valve (Mann, Citation1983).
Round or transapically elongate poroids form uniseriate striae, which are not interrupted by accessory sterna (–). The pores are fully open externally (–) but are occluded internally by flaps of silica (volate occlusions sensu Mann, Citation1981a), which are borne on cribra (–) or held by struts from the poroid wall (). The flap-like occlusions may be associated with individual pores (P. monilifera and P. marina: , ), or they may coalesce with the neighbouring occlusions within a stria to form long transapical strips (P. humerosa, P. latissima: , ). In addition, in P. humerosa, P. latissima and P. monilifera, the flaps are ornamented with small bumps (–), whereas those of P. marina are smooth ().
The striae bordering the central area are generally radial, whereas those alongside the axial areas are ± parallel to each other but at an angle to the raphe (). As the striae radiate away from the centre of the valve, shorter striae are intercalated between the main striae and the overall density of the pore arrangement is maintained. In the case of P. marina and P. granulata, the striae radiate less strongly from the central area and there is a correspondingly lower number of shorter striae intercalated at the margin. The striae are slightly convergent near the poles in P. humerosa, P. latissima, P. marina and P. granulata (–, , –), becoming perpendicular to the raphe, but they tend to remain radial in P. monilifera (–).
P. marina and P. granulata are very similar in size, shape and stria orientation (–, ) and brief inspection might suggest that they are conspecific. However, there are several constant differences. In P. marina, the poroids are ± evenly spaced within the striae, becoming gradually (and often only very slightly) denser towards the margin, whereas in P. granulata the valve face is differentiated into a central zone, with widely and rather irregularly spaced poroids, and a marginal zone, with dense, regularly spaced poroids (). Furthermore, the central area, although transversely elliptical in both, is more nearly circular and the central raphe endings are more distant in P. marina than in P. granulata, in valves of the same length (contrast with ; see also Hustedt, Citation1927–66, Vol. 3, Figs 1696, 1697 and pp. 702–707).
The raphe sternum is smooth, externally and internally, and is slightly thicker than the rest of the valve (–, –); the raphe opens along the midline of its internal surface (–). The central raphe endings are complex externally, consisting of an inner slit that opens into a greatly expanded groove, which may be spathulate (P. humerosa, P. monilifera and P. latissima: ), T-shaped (P. marina: ) or bulbous (P. granulata: light microscope observations). Inside the valve, the central endings do not expand, but are strongly reflexed, as in a shepherd's crook, which is turned towards the primary side (–). At the poles, the terminal fissures turn towards the secondary side, as in most raphid diatoms, and they widen towards their ends (, , ). In P. humerosa, P. monilifera and P. latissima, the terminal fissure is a simple hook (, , ), whereas it is more complex, with a double bend, in P. marina (). Internally at the poles, there are narrow helictoglossae, which are slightly bent towards the secondary side (, ).
Figs 30–32. Partially developed valves of Petroneis species, acid cleaned material, SEM. , . P. humerosa: deposition of silica is incomplete and cribra have not yet developed. Although the striae are clearly developed, the areolae have not yet been clearly demarcated in all areas by the formation of cross walls within the striae. The central endings of the interior raphe opening are straight, unlike the hooked structure present in fully silicified valves. . P. monilifera: early stages of cribrum development. The striae and areola walls are fully developed and small peg-like ingrowths have formed around the periphery of individual areolae. Scale bars represent 2 µm.
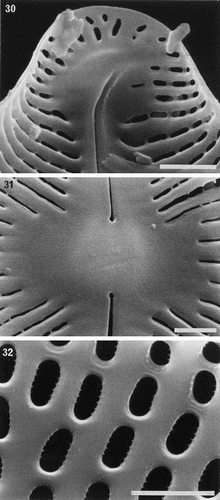
Partially formed valves of P. humerosa show that the transapical ribs are well developed before the addition of frets to delimit the areolae (). The internal central endings are initially straight (), and the final hooked shape () only develops at a later stage of silicification. In P. monilifera, it was clear that cribra develop initially as peg-like ingrowths from the periphery of each areola () and the flaps are produced towards the end of valve formation.
The cingulum consists of three split (open) girdle bands. Each split end is filled by a ligulate structure on the closed end of the adjacent band (, ). The girdle band adjacent to the valve (the valvocopula) is the widest and bears one row of poroids, whereas subsequent girdle bands appear generally to have two rows ().
All frustules are of the cis type (Mann, Citation1983), with the primary (first-formed) sides of both valves lying on the same side of the cell (Mann & Stickle, Citation1988). As a consequence, all four terminal fissures within a frustule point in the same direction and the Voigt discontinuities all lie on the same side of the cell.
Protoplast morphology at interphase
All of the four species examined (no living material of P. granulata was available) have two butterfly-like chloroplasts, one lying against each valve (, , , ). The butterfly shape is created by the formation of four deep indentations, two extending in from the poles along the apical axis (beneath the raphe), and two lying in the median transapical plane. In P. humerosa, P. monilifera and P. latissima these indentations almost meet at the centre of the valve, leaving a narrow, often nearly indiscernible strand linking the two halves of the chloroplast transapically beneath the valve (, , ). In contrast, in P. marina a much broader bridge exists (). All species also exhibit transapical indentations of the chloroplast margin at the centre. Lobes are present at the margins of the chloroplasts, which fold downwards along the valve mantles and girdle bands (seen in section at the cell periphery in, for example, ).
Figs 33–44. Comparison of protoplast structure in interphase cells of Petroneis, Lyrella and Navicula sensu stricto, LM, DIC optics. Adjacent pairs of illustrations show protoplast structure at two focal planes of a single cell, immediately below the upper valve (left), and in the horizontal median plane (right). The lower part of the protoplast is not illustrated, because it resembles that of the upper protoplast in each of these species. , . P. humerosa. Note the thin, transverse bridge (arrowed) that links the four arms of the H-shaped chloroplast; also the pyrenoids (p), nucleus (n) and volutin granules (v). , . P. monilifera. , . P. latissima. Note the thin mucilage sheath that envelopes the cell (arrow), and the numerous small granules, which are frequently observed in this species. , . P. marina. Note the single, square pyrenoid at the centre of the chloroplast, and the rounded nucleus. , . Lyrella species (cf. L. lyra (Ehrenb.) Karayeva). The elaborately lobed chloroplast contains two rounded pyrenoids located beneath the raphe, and a transapically elongate nucleus. , . Navicula reinhardtii Grun. in Van Heurck, with two simple chloroplasts, one lying along each side of the girdle. Scale bars represent 20 µm.
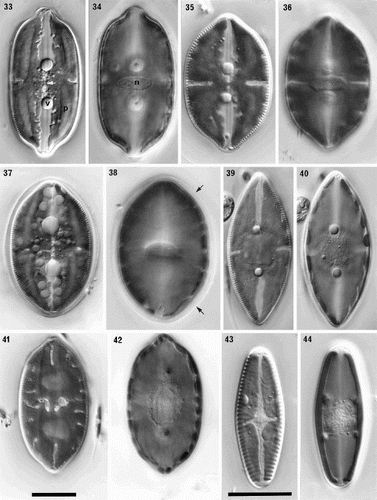
In P. humerosa, P. monilifera and P. latissima, each chloroplast contains four elongate, sometimes wedge-shaped pyrenoids (eight per cell), which lie longitudinally along the four arms of the chloroplast (e.g. ). In P. marina, on the other hand, a single, square pyrenoid is present at the centre (), within the bridge linking the four quarters of the chloroplast. The nucleus is generally transapically elongate in valve view (, , ), and lies in a cytoplasmic bridge that crosses the centre of the cell from valve to valve, surrounded by the large central vacuole; from the bridge, a few thin strands may extend out across the vacuole to the peripheral cytoplasm. Again, P. marina is the anomaly, with nuclei that are ± circular in valve view (). Two very prominent, large, spherical volutin granules are normally present near the bases of the apical indentations of each chloroplast (four per cell; , , , , ) in every species. Additional small granules may be present, especially in P. latissima (), associated with the pyrenoids and chloroplasts.
In some samples, the cells were highly mobile, moving predominantly in straight lines and reversing frequently. In a few cases, however, single, stationary cells of P. latissima, P. monilifera and P. humerosa were observed, surrounded by thin but well-differentiated gelatinous sheaths (, ). Sheaths have also been observed during cell division.
Cell division
Very few observations of cell division were made during studies of Petroneis in natural populations and these were exclusively of P. humerosa. Petroneis humerosa was grown in culture, but here too, dividing cells were very rare, because the clone grew extremely slowly (cells dividing once per week or less). Our data on the cell cycle are therefore incomplete. It was striking that, in natural samples containing many dividing cells of other genera, such as Navicula, Lyrella, Amphora Ehrenberg ex Kützing or Biremis D.G. Mann & E.J. Cox in Round et al., Petroneis cells were almost invariably solitary and vegetative.
Dividing cells are frequently enclosed within a thick mucilage sheath. The transapically elongate nucleus seen in interphase becomes spherical and migrates to the side of the cell, beneath the girdle. We were not able to determine whether there is a correlation between the direction of migration and the polarity of the frustule. In other diatoms with constant cis symmetry of the frustules, however, the nucleus divides on the side of the cell with the primary sides of the valves, there being an apparently obligate relationship between the position of the dividing nucleus and the orientation of the new valves (Mann & Stickle, Citation1988; Round et al., Citation1990).
During cell division, the volutin granules migrate along the raphe towards the poles and disperse. The narrow bridge at the centre of each chloroplast gradually becomes thinner, until longitudinal division is complete. No further observations of chloroplast movement during cytokinesis and hypovalve formation were made. The apical indentations in the chloroplast beneath the raphe are less well developed in recently divided cells than in later interphase (cf. Lyrella atlantica (A. Schmidt) D.G. Mann: Mann & Stickle, Citation1993).
Protoplast morphology during sexual reproduction
Auxospore formation is allogamous. Because our observations were based on mixed natural populations, we do not know whether Petroneis species are homothallic or heterothallic. However, the absence of any trace of sexual reproduction in two monoclonal cultures of P. humerosa that we maintained for several years, during which cells became much smaller than any we have seen in nature (i.e. they must have been well below the critical size threshold for sexual reproduction), suggests (cf. Mann & Chepurnov, Citation2004) that this species is probably heterothallic.
Paired cells usually lie girdle-to-girdle, with primary–primary, primary–secondary, or secondary–secondary alignment. A thick mucilage sheath is secreted (), which appears to consist of a narrow outer layer and a much wider inner layer. This presumably protects the gametangia, auxospores and initial cells, and certainly keeps them in close proximity throughout the whole process of sexual reproduction and size restitution.
Figs 45–52. Sexual reproduction in Petroneis humerosa from Portobello beach, Edinburgh, LM, DIC optics. . Recently formed auxospores and gametangial thecae within a thick mucilage sheath. The junctions between the fused gametes are still visible (arrow) within each auxospore. . Same cells as in , but two days later. Integration of the gametes is complete and the auxospores are retracting towards each of the gametangial frustules. Note the double boundary of the mucilage sheath (arrows). . Spherical, binucleate auxospores after plasmogamy and retraction to within the gametangial thecae. . Same cells as in . Each auxospore has expanded along the longitudinal axis of the parent theca and developed a silicified perizonium around the cell. The protoplast has pulled inwards, away from the perizonium (arrows). . Expanded auxospore showing a lightly silicified transverse perizonium in section at left, and the central longitudinal band (striations right of centre). . Near-surface focus of expanded auxospore, showing transverse perizonium. Note the straight, central band (white arrows) and the other curved bands, which appear to be interrupted at the longitudinal suture (between black arrows). . Different focus of the cell shown in . Two H-shaped chloroplasts lie around the periphery on opposite sides of the auxospore. The nuclei (still unfused) lie beneath the intersection of the longitudinal suture and the central transverse band of the perizonium. . Expanded auxospore of Navicula oblonga. Note the caps of organic material at each pole, and the linear-concave outline of the auxospore. Scale bars represent 20 µm.
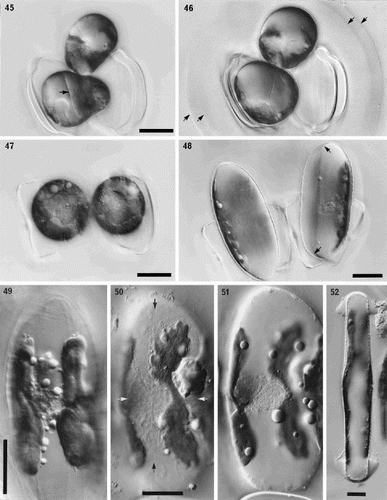
During meiotic prophase, the nuclei become enlarged and migrate towards the side of the cell adjacent to the partner cell, but the chloroplasts do not move appreciably within the cell (not illustrated). Subsequently, cytokinesis occurs and two morphologically similar, uninucleate gametes are formed within each parental cell.
Fusion occurs between a pair of adjacent gametes (plasmogamy), one from each parent cell, to form two auxospores (). Initially, each auxospore can be seen to consist of two halves, because integration of the two protoplasts is not completed for some time after the two gametes have joined (). Later, the boundary between the fused protoplasts disappears () and the gamete nuclei become closely associated and move to one side of the zygotes. However, they do not fuse until much later in auxospore development, so that the young auxospores are binucleate (). After plasmogamy, the zygotes retract in opposite directions (, ) and become spherical (), frequently remaining or becoming closely associated with one of the parent thecae, although this is not always the case. The chloroplasts are arranged around the periphery of the young, spherical auxospores ().
The auxospores normally expand parallel to apical axes of the gametangia to form ellipsoidal auxospores with convex walls (). Caps of broken organic wall are not apparent at the auxospore tips (contrast Navicula: ). Late in expansion (–), the paired nuclei lie against the wall of the auxospore, with two, now recognizably H- or X-shaped chloroplasts (i.e. similar in shape to those in the vegetative cells) lying around the periphery of the cell on either side (–).
The auxospore casing is silicified in the form of a perizonium, which is formed around the periphery of the cell and consists of two parts, transverse and longitudinal (cf. Mann, Citation1982b; Round et al., Citation1990). The transverse perizonium, formed sequentially from the centre outwards as the auxospore expands (cf. Mann, Citation1994b), consists of a series of striated, transverse bands (in section in the right-hand auxospore of and in ). The central transverse band forms a complete girdle around the auxospore () and is slightly broader than the other bands, especially those immediately on either side of it. All the transverse bands except the central one are split, their open ends being aligned to form a suture (), which runs along the longitudinal axis of the auxospore. The peripheral unfused nuclei lie just beneath the suture and central transverse band (, ). Near to the suture, the incomplete transverse bands curve towards the central band, and this curvature becomes more marked towards the poles of the perizonium (e.g. near the top arrow in ). The longitudinal perizonium consists of lightly silicified bands with transverse striations, which are aligned longitudinally beneath and near the suture of the overlying transverse perizonium ().
Prior to development of the initial epivalve, the gametic nuclei fuse. The chloroplasts lie on the side near the longitudinal perizonium. The protoplast contracts slightly away from the perizonium on this side (), but only where the valve faces of the initial valves will form, not where the valve margins and girdle are laid down. Hence the outline of the initial valves conforms fully to the lanceolate outline of the fully expanded auxospore (compare and ).
Habitat and distribution
In total, nearly 800 valves were examined during this study, of which 358 were P. humerosa, 266 P. monilifera, 119 P. marina and 51 P. latissima. Though they are not in any sense a quantitative estimate of abundance, these numbers coincide with our general impression of the overall frequency and extent of each species within the UK, with P. humerosa being by far the most common and widespread, followed by P. monilifera, P. marina, P. latissima, and finally P. granulata, which is apparently rare. All of the species (except P. granulata, for which no habitat information was available) occurred in sandy sediments from the intertidal or uppermost subtidal; interestingly, only P. latissima was observed in deep subtidal samples (e.g. Park, Citation1992). Petroneis humerosa and P. latissima occurred occasionally, and P. marina more frequently, in estuarine and saltmarsh environments. Populations of Petroneis species were detected throughout the year and can be conspicuous among the epipelic diatoms and dinoflagellates forming golden brown discolourations on sand exposed by the receding tide. All five species have a wide geographical distribution (e.g. Hustedt, Citation1927–66; Witkowski et al., Citation2000), though this may in part reflect lack of taxonomic resolution at the species level.
Molecular phylogeny
The rbcL sequence analysis gave a single most-parsimonious tree, which had the same topology as the ML tree shown (), except that Placoneis grouped with Gomphonema, not Encyonema, and Pseudogomphonema with Navicula, not Seminavis. However analysed, the sequence data yielded trees with very high support for a sister relationship between Lyrella and Petroneis (). Other well-supported features were:
1. | a hierarchy of relationships within Lyrella, L. atlantica being sister to L. hennedyi–Lyrella sp. | ||||
2. | a group containing Placoneis, Encyonema and Gomphonema | ||||
3. | two clades representing genera separated from Navicula in the last 20 years, viz. Placoneis and Sellaphora | ||||
4. | the Naviculaceae, containing Navicula, Pseudogomphonema and Seminavis. |
Fig. 53. Phylogram of selected raphid diatoms (with Rhizosolenia, Thalassiosira and Eunotia as outgroups) inferred from maximum likelihood (ML) analysis of rbcL sequences. Numbers on branches are (in order) bootstrap values from maximum parsimony analysis, bootstrap values from ML analysis, and posterior probabilities from Bayesian analysis, all expressed as percentages.
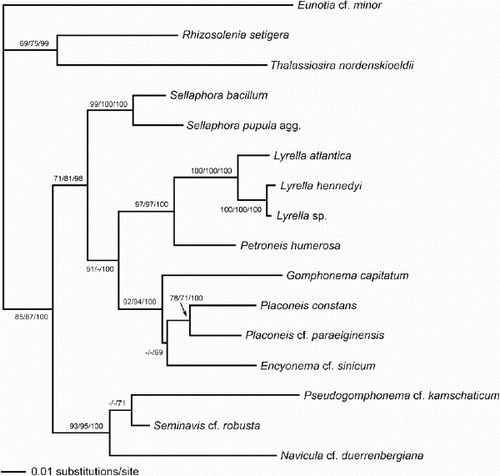
Morphological cladistics
Parsimony analysis of the data matrix in yielded 11 most-parsimonious trees, which showed considerable diversity. shows a majority-rule consensus tree; strict consensus left only those nodes with >50% bootstrap support. Comparisons with the molecular phylogram are difficult because they were inevitably based on different sets of taxa. Common features (using genus names as short-hand for the species included) are (i) strong support for a close relationship between Lyrella and Petroneis; (ii) strong support for a clade containing Navicula, Pseudogomphonema and Seminavis; and (iii) support for an early separation between a lineage containing both the Naviculaceae (Navicula, Pseudogomphonema and Seminavis) and the Stauroneidaceae (Stauroneis Ehrenberg and Craticula Grunow), and a lineage containing genera as apparently diverse as the Sellaphoraceae (Sellaphora and Fallacia), the gomphocymbelloid diatoms (Encyonema, Cymbella, Gomphonema, Placoneis), Lyrella and Petroneis, and miscellaneous raphid genera (Rhoicosphenia Grunow, Anomoeoneis Pfitzer and Dickieia Berkeley ex Kützing).
Fig. 54. Majority rule consensus tree (length 70, consistency index 0.49, retention index 0.64) based on a maximum parsimony analysis of the morphological, cytological and reproductive data matrix in ; 11 most parsimonious trees were found. Numbers above branches indicate the percentage frequency of occurrence of the node among the most parsimonious trees. Italicised numbers below branches are bootstrap values.
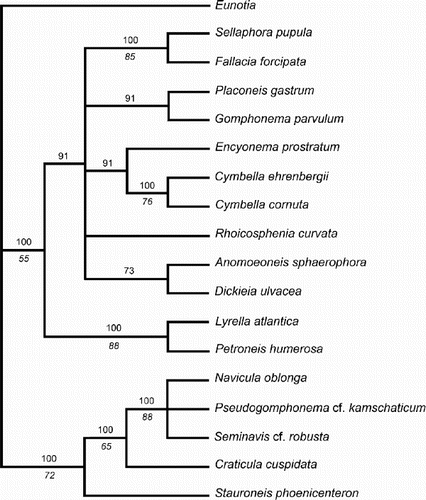
The lack of resolution in the consensus cladogram makes detailed analysis of character state transitions somewhat pointless. One of the 11 most-parsimonious trees () was chosen to display character state changes. Extensive homoplasy is evident, even in the few characters shown in . For example, lateral asymmetry is a synapomorphy of the Cymbella–Encyonema clade and of Seminavis (the outgroup is also asymmetrical); physiological anisogamy characterises both Sellaphora–Fallacia and a clade containing Anomoeoneis, Lyrella and Petroneis, Placoneis and the gomphocymbelloid diatoms; possession of two chloroplasts is a synapomorphy for the Naviculaceae–Craticula–Stauroneis clade and for Lyrella–Petroneis; and heteropolarity (not shown) is triply homoplasious, being autapomorphic for each of Gomphonema, Rhoicosphenia and Pseudogom-phonema. Other most-parsimonious trees show different multiple homoplasies.
Fig. 55. One of the most-parsimonious trees derived from analysis of the matrix. The tree topology is similar to the molecular phylogram () and is used to illustrate some possible character state transitions, following character optimisation using MacClade version 3.07. Queries indicate that the character state transition cannot be placed unequivocally, but cannot be any higher in the tree without incurring a parsimony penalty. c.r.e = central raphe endings.
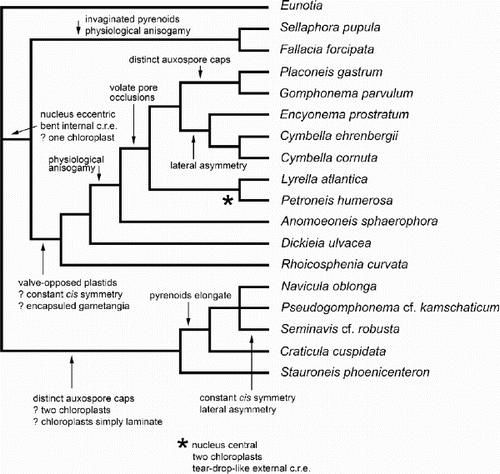
Despite the strong support for a sister-group relationship between Lyrella and Petroneis, the only character that was consistently a synapomorphy for the Lyrella–Petroneis clade was tear-drop-shaped external central raphe endings.
Discussion
The species of Petroneis studied here share many characteristics of valve, raphe and girdle structure, and all have large, butterfly-like, valve-appressed chloroplasts. Petroneis humerosa (the type), P. monilifera and P. latissima are especially similar to each other, while P. marina differs in details of areola structure, valve shape and chloroplast structure (particularly with respect to the shape and position of the pyrenoids). As far as can be judged from the limited data available, P. granulata is closely related to P. marina.
In , we list the principal non-molecular characteristics of genera included in the rbcL analysis and the non-molecular parsimony analysis; this table is more comprehensive than , which refers only to exemplar species. All Petroneis species were previously classified in Navicula. The valve morphology of Navicula sensu stricto is highly characteristic, however, and differs from Petroneis in the following respects (Cox, Citation1979, Citation1987 b; Round et al., Citation1990): the valve pores are apically elongate, slit-like poroids, which are aligned longitudinally, with membranous occlusions (hymenes) perforated by tiny round holes; the raphe sternum bears internal ridges, and internally the raphe generally opens obliquely, to one side of the sternum; the internal central raphe endings are straight; and the external central raphe endings may be expanded, but never open into a groove like that present in Petroneis (). Furthermore, the frustules of Navicula may be of either cis or trans configuration (in a 1 : 2 ratio: Mann & Stickle, Citation1988), the girdle bands are generally not porous (Round et al., Citation1990), and the two chloroplasts (which are simple bands rather than the complex lobed plates in Petroneis), are girdle-appressed (, ), not valve-appressed, during interphase. Lastly, the pyrenoids of Navicula are straight, parallel-sided bars (e.g. Mann, Citation1989 a; Mann & Stickle, Citation1989), rather than the curved strips or square pyrenoids of Petroneis. Navicula sensu stricto shares many characters with the heteropolar Pseudogomphonema and the isopolar but dorsiventral Seminavis (); together, they form a well-supported branch in the molecular and non-molecular trees.
The idea that Navicula sensu stricto and Petroneis are members of distinct taxonomic groups is not new. It was explicitly recognised in 1895, when Cleve divided Navicula Bory into groups, separating the Lineolatae (equivalent to Navicula sensu stricto) from the Punctatae (in which all the current Petroneis species were once grouped). However, until 1990, when the genus Petroneis was created, the separation remained at a sectional or subgeneric level (Cleve-Euler, Citation1953; Hustedt, Citation1927–66; Hendey, Citation1964, Patrick, Citation1959; Patrick & Reimer, Citation1966). Our study confirms that Navicula sensu stricto and Petroneis differ in many fundamental aspects of valve structure and protoplast morphology during both interphase and sexual reproduction, and the rbcL data show that Navicula could not both be made monophyletic and at the same time include Petroneis without it also having to encompass such widely divergent forms as Encyonema, Gomphonema, Pseudogomphonema and Seminavis. The morphological parsimony analysis yields the same conclusion.
Lyrella, on the other hand, has a frustule morphology that is similar in many respects to that of Petroneis, with round or transapically elongate pores that contain flap-like occlusions, a simple raphe sternum, expanded external central raphe endings (the tear-drop-shaped groove is apparently a synapomorphy for the Lyrellaceae), hooked internal central raphe endings (in some species; others have T- or anchor-shaped central internal endings), constant cis frustule symmetry, and porous girdle bands (Mann & Stickle, Citation1993, Citation1997; Mann, Citation1998) (). The protoplasts of living cells of some species of Lyrella are also similar to those in Petroneis (, ), both taxa having highly lobed and indented chloroplasts, which are appressed to the valves; central nuclei in a bridge of cytoplasm between the valve centres; four volutin granules; and the ability to produce mucilage sheaths (Mann & Stickle, Citation1993, Citation1997; Mann, unpublished observations). Admittedly, our limited observations of chloroplast division in P. humerosa indicate differences between it and L. atlantica (Mann & Stickle, Citation1993), in that plastokinesis in P. humerosa is autonomous, whereas in L. atlantica, it is brought about by the cleavage furrow, which disrupts the pervalvar bridges linking the halves of each chloroplast. However, the range of interphase chloroplast morphologies within Lyrella (our unpublished observations and Karsten, Citation1899) shows that the pattern of behaviour in L. atlantica cannot be common to all species. In any case, further evidence of the similarity between Lyrella and Petroneis comes from our observations of a minority of cells in some populations of P. humerosa, in which the normal chloroplast configuration was not present. Instead, the two butterfly-like chloroplasts were linked by a narrow isthmus close to the nucleus, which traversed the cell from valve to valve. Other unusual cells had two chloroplasts, one on either side of the median transapical plane, each consisting of two lobes, one against each valve, joined by a pervalvar bridge (not illustrated). This is the same chloroplast arrangement that occurs in L. atlantica (Mann & Stickle, Citation1993). These unusual P. humerosa cells may have been the result of abnormal cell division, induced by unfavourable conditions in the laboratory, but their resemblance to Lyrella is striking and seems to indicate a similarity in the mechanisms determining the positions and development of the chloroplasts. A mucilage sheath is present during cell division, and sometimes at other stages of the cell cycle, in Petroneis and Lyrella species (Mann & Stickle, Citation1993, and our unpublished observations, e.g. of the Lyrella cultures used for the rbcL analysis).
Lyrella atlantica (see Mann & Stickle, Citation1993) and P. humerosa also share type IA1aα auxosporulation, in Geitler's (Citation1973) classification. Each cell produces two gametes, one apparently active and the other passive. The gametes detach from the thecae and rearrange within the gametangia before fertilisation and plasmogamy occurs without the formation of a copulation tube. During plasmogamy, the fusing pairs of gametes move around within the gametangia so that one zygote lies in one gametangium and the other in the other gametangium. The two auxospores subsequently expand parallel to the long axes of the gametangia, becoming elongate ellipsoids. The asymmetrical progress of plasmogamy, in which the fusing gametes move so that one zygote ends up in each gametangium, was considered by Geitler (Citation1973) to represent physiological anisogamy. However, Mann & Stickle (Citation1993, pp. 59–63) suggested an alternative hypothesis, that gamete behaviour could be determined as much by the spatial constraints imposed by the mucilage sheath and the parent thecae, as by active physiological control and differentiation between the gametes.
Further similarities between Petroneis and L. atlantica auxosporulation are the production of a robust mucilage sheath around the gametangia and auxospores, and the slight contraction of the protoplast away from the perizonium during initial cell formation (). The only obvious difference between the two taxa is that the Petroneis auxospore has two rather than four chloroplasts, which reflects the different arrangement of chloroplasts in the mitotic cells.
Sexual reproduction in Navicula sensu stricto (Mann & Stickle, Citation1989) differs from P. humerosa in several aspects (). In Navicula, plasmogamy is isogamous; no mucilage sheath is formed around the gametangia and the gametangia and auxospores are easily separated; the supernumerary nuclei formed at meiosis II do not degenerate for some time, so that quadrinucleate zygotes are formed in some species (Geitler, Citation1952 b, c; Karsten, Citation1896); the expanded auxospores bear caps of broken zygote wall at each pole ( and Mann & Stickle, Citation1989, , , ); and the auxospores are not ellipsoidal but more or less linear, with a slight taper or concave margins (). Thus, evidence from the sexual phase corroborates the conclusion from wall and plastid data, that Navicula and Petroneis are distinct and unlikely to be closely related. Navicula is instead most similar to, and most closely related to, Pseudogomphonema and Seminavis (, –).
Given all the similarities between Lyrella and Petroneis in cell wall structure, protoplast and sexual reproduction (), it is reassuring to find that the rbcL tree and the parsimony analysis of non-molecular data give robust support for a close relationship between the two genera, and indicate a much more distant relationship between either and Navicula. Extant Lyrella and Petroneis species are marine or brackish, living in the epipelon, and Lyrella is apparently one of the earliest genera of raphid diatoms to appear in the fossil record, being reported from the Palaeocene (Strel’nikova, Citation1992). Lyrella valves are distinctive because of the combination of the tear-drop raphe endings at the centre and the lyrate sternum, but some Mastogloia valves also possess lyre-shaped markings and are sufficiently similar to Lyrella in LM for confusion to be possible. It would therefore be helpful to have SEM data to confirm the identity of the Palaeocene material. Nevertheless, the most likely identification of valves such as those illustrated from Palaeocene material near Arkhangel'sk, northern Russia (for dating, see Ross & Sims, Citation1985) by Witt (Citation1886, pl. 9, , ) is indeed Lyrella. If so, then our rbcL data and the 18S rDNA data of Behnke et al. (Citation2004) and Medlin & Kaczmarska (Citation2004), imply that the fossil record seriously underestimates the diversification of the raphid diatoms, because Lyrella is not a basal lineage in either gene tree. Many Lyrella species are present in the Upper Eocene marine deposits at Oamaru, New Zealand (Schrader, Citation1969; Desikachary & Sreelatha, Citation1989; Edwards, Citation1991), which also contain at least one species that belongs to Petroneis (Edwards, Citation1991, pl. 19, Fig. 262, identified as ‘Navicula japonica Heiden’).
The most obvious difference between Lyrella and Petroneis is the lyre-shaped sternum interrupting the striae in Lyrella. However, there is one species currently classified in Lyrella, L. praetexta Ehrenberg, in which the lyre-shaped areas are not true sterna, i.e. they do not fully interrupt the striae. Instead, they are porous and differ from the remainder of the valve face only in the density and irregularity of the areolae (Hustedt, Citation1927–66, Fig. 1488; Montgomery & Miller, Citation1978, pl. 146G). The L. praetexta species complex is also remarkable for being one of the earliest known raphid diatoms, valves similar to those of living specimens being among those illustrated by Witt (Citation1886) from the Palaeocene. Possibly, then, L. praetexta represents a lineage that diverged from other Lyrella relatively soon after the separation of Lyrella and Petroneis and before the evolution of true lateral sterna. This idea is testable using gene sequence analysis, although obtaining living L. praetexta may be difficult: around northern European coasts, we have found it only in the deep subtidal (where it was found also by Gregory, Citation1857). Another species that may be critical to an understanding of the evolution of the Lyrella–Petroneis group is L. sandriana (Grunow) D.G. Mann. Here, in contrast to L. praetexta, the lyrate lateral areas do contain hyaline zones (though they also contain a strip of pores), but L. sandriana differs from all other Lyrella species that we have examined so far in having a single chloroplast, consisting of two valve-appressed plates, linked by an eccentric bridge containing a pyrenoid. This is the same or similar chloroplast morphology as in Anomoeoneis, Rhoicosphenia, Dickieia, Placoneis and the gomphocymbelloid genera, which are linked to Lyrella and Petroneis by one or both of the molecular and morphological analyses. By contrast, L. praetexta has four valve-appressed chloroplasts, as in several other Lyrella species, including L. abrupta (Gregory) D.G. Mann (our unpublished data).
Cleve (Citation1895) originally grouped many other species in the section Punctatae, together with those now separated into Petroneis. Few of these other species have been studied in sufficient detail to allow useful comparisons with Petroneis. Exceptions are Naricula. carinifera Grunow ex A. Schmidt and N. pusilla W. Smith. Cleve considered that N. carinifera was intermediate between the sections Lyratae and Punctatae, owing to the coarse arrangement of areolae and the lyre-shaped marking on the valve surface. This link is now less certain, because it is clear that the lyrate appearance of N. carinifera is produced by folding of the valve (to produce a raised area near the raphe: Hendey, Citation1964, pl. 31, , pl. 33, ), rather than by non-porous sterna like those characteristic of Lyrella. There is no doubt, however, that N. carinifera possesses many characteristics in common with the Lyrella–Petroneis group, including coarse areolae, uniseriate striae, the internal raphe-sternum structure (simple and lacking ridges), and the interphase protoplast structure (four valve-appressed chloroplasts, indented beneath the raphe, each with one round pyrenoid: H.M. Jones, unpublished observations). On the other hand, N. carinifera has hymenate pore occlusions, rather than the flap-like occlusions of Lyrella and Petroneis, and the internal central raphe endings are simple and straight, rather than hooked or T-shaped (D.G. Mann, unpublished observations), as in Petroneis and Lyrella. Further examination of this species, especially during vegetative and sexual reproduction, is needed before its relationship to Petroneis, Lyrella and Navicula sensu stricto can be determined.
Navicula pusilla differs from Petroneis species in frustule morphology, and has been moved to a separate genus, Cosmioneis D.G. Mann & Stickle (Round et al., Citation1990). During interphase, there are two valve-appressed chloroplasts, as in Petroneis (Round et al., Citation1990). However, Cosmioneis has hymenate pore occlusions, pore-like central external raphe endings (which are much simpler in structure than the tear-drop grooves of Petroneis and Lyrella), stepped mantles at the valve poles, a deeper girdle containing a higher number of bands, and curious pores in the most advalvar girdle bands at the valve poles (Round et al., Citation1990), all of which separate it from Petroneis and Lyrella.
Among the diatoms included in the rbcL analysis, those most closely allied to Lyrella and Petroneis are Placoneis, Encyonema and Gomphonema. The bootstrap support for this link is not high (though bootstrap values can be highly conservative estimators of the correct clades: Hillis & Bull, Citation1993), but the relationship is plausible on other grounds () and is not inconsistent with our non-molecular parsimony analysis (, ). Thus, for example, in the Cymbellales (to which Placoneis, Encyonema and Gomphonema belong, together with several other genera, including Cymbella, Brebissonia Grunow, Didymosphenia M. Schmidt in A. Schmidt and Reimeria Kociolek & Stoermer), the nucleus always divides on the same side of the cell and hence all frustules have cis symmetry (Mann & Stickle, Citation1988). Furthermore, the chloroplasts are appressed principally to the valves, as in the Lyrellales, the central internal raphe endings are hooked towards the primary side (sometimes the endings are obscured by an overgrowth of silica), as in Petroneis, and the girdle bands are porous, with one or two rows of areolae per band (Round et al., Citation1990). Many Cymbellales also produce robust mucilage capsules during sexual reproduction (see Mann & Stickle, Citation1995) and sometimes also during the vegetative phase (our unpublished observations of Cymbella sensu Round et al., Citation1990), and the pattern of sexual reproduction in Cymbella and Gomphonema (summarised by Geitler, Citation1973) is similar to that in Lyrella (Mann & Stickle, Citation1993) and Petroneis. It seems reasonable, therefore, to suggest that the Lyrellales and Cymbellales may form a separate clade within the raphid diatoms, characterised by a fundamental lateral asymmetry of the protoplast (so that the nucleus always divides on the same side, even when the valves are bilaterally symmetrical), valve-appressed chloroplast plates, the formation of robust mucilage capsules around the gametangia and auxospores (and sometimes also around the vegetative cells), and hooked internal central raphe endings. Some of these features may be symplesiomorphies, i.e. synapomorphies for a larger group than simply the Lyrellales and Cymbellales, but without more extensive taxon sampling and better support for tree nodes, it is impossible to be sure. A link between Lyrella and Gomphonema is also suggested by the limited 18S rDNA sequence analysis of Behnke et al. (Citation2004).
It is interesting that in Petroneis (this study) and Gomphonema (Cox, Citation1999), the internal central raphe endings only develop their hooked morphology during the later stages of valve formation; initially, they are straight. Unfortunately, however, there are no comparable data for other members of the Cymbellales and Lyrellales.
The position of genera like Dickieia, Rhoicosphenia and Anomoeoneis, which share many of the characteristics of the Cymbellales and the Lyrellales, except that they have hymenate pore occlusions (e.g. Mann, Citation1982a, Citationb, Citation1994a; Round et al., Citation1990), is currently unclear. Mann (Citation1981a) suggested that it was unlikely that the hymenate type of pore occlusion evolved more than once, and if so, Dickieia, Rhoicosphenia and Anomoeoneis could be basal to the Cymbellales and Lyrellales. However, our non-molecular parsimony analysis is equivocal about their position, and no molecular data-set currently covers all of these taxa. In Cox & Williams’ (Citation2000) non-molecular analysis, Dickieia, Anomoeoneis and Staurophora Mereschkowsky are sister to the Naviculales (including Navicula, Haslea Simonsen, Plagiotropis Pfitzer, Stauroneis and Craticula), and separated from Petroneis by a branch to Mastogloia Thwaites and its allies. However, none of the Cymbellales with flap-like pore occlusions (e.g. Cymbella, Encyonema, Gomphonema, Placoneis) were included in their analysis and the rooting of the tree differs from ours (Petroneis vs. Eunotia), so comparison is difficult. The two trees do have two features in common, however: robust support for a Dickieia–Anomoeoneis clade, and for a clade containing Navicula, Craticula and Stauroneis.
Acknowledgements
The morphological and cytological studies reported here were supported by NERC GR3/7923 and SERC GRC/68484. During the long gestation of this work, we have been supported by many people. We are grateful for overall support to Prof. D.S. Ingram, formerly at the Royal Botanic Garden Edinburgh; Prof. M.M. Yeoman, formerly at the University of Edinburgh; and Prof. F.E. Round, Bristol University. We also thank Dr Eileen Cox (Natural History Museum, London) for confirming the identification of the Placoneis clones; Ms. Debbie White and Mrs. Ingrid Jack for their help with collection of samples; Mr. Bernard Hartley, colleagues at the Natural History Museum (London), and Dr R.K. Edgar (Farlow Herbarium, Harvard), for the loan or provision of material; Dr. Michelle Hollingsworth, Alex Ponge and Dr. Michael Moller for assistance with molecular phylogenetics; and a reviewer for helpful comments. David Mann thanks the Royal Society for an equipment grant enabling purchase of a Reichert Polyvar photomicroscope. Final manuscript preparation was aided by equipment purchased under NERC grant 754/BIO14262.
References
References
- Behnke , A , Friedl , T , Chepurnov , VA and Mann , DG . 2004 . Reproductive compatibility and rDNA sequence analyses in the Sellaphora pupula species complex (Bacillariophyta) . J. Phycol. , 40 : 193 – 208 .
- Chepurnov , VA , Mann , DG , Vyverman , W , Sabbe , K and Danielidis , DB . 2002 . Sexual reproduction, mating system and protoplast dynamics of Seminavis (Bacillariophyta) . J. Phycol. , 38 : 1004 – 1019 .
- Cholnoky , BJ . 1927 . Über die Auxosporenbildung von Rhoicosphenia curvata (Kg) Grun. . Arch. Protistenk. , 60 : 8 – 33 .
- Cholnoky , BJ . 1928 . Über die Auxosporenbildung der Anomoeoneis sculpta E. Cl. . Arch. Protistenk. , 63 : 23 – 57 .
- Cholnoky , BJ . 1929 . Beiträge zur Kenntnis der Auxosporenbildung . Arch. Protistenk. , 68 : 471 – 502 .
- Cleve , PT . 1895 . Synopsis of the naviculoid diatoms . K. Svenska Vetenskaps-Akad. Handl. , 27 ( 3 ) : 1 – 219 .
- Cleve-Euler A 1953 Die Diatomeen von Schweden und Finnland Teil 3. Monoraphideae, Biraphideae 1 K. Svenska Vetenskaps-Akad. Handl. ser. 4 4 5 1 232
- Cox , EJ . 1979 . Taxonomic studies on the diatom genus Navicula Bory: the typification of the genus . Bacillaria , 2 : 137 – 153 .
- Cox , EJ . 1987a . Placoneis Mereschkowsky: the re-evaluation of a diatom genus originally characterised by its chloroplast type . Diatom Res. , 2 : 145 – 157 .
- Cox , EJ . 1987b . Studies on the diatom genus Navicula Bory. VI. The identity, structure and ecology of some freshwater species . Diatom Res. , 2 : 159 – 174 .
- Cox EJ 1996 Identification of freshwater diatoms from live material, Chapman & Hall London
- Cox , EJ . 1999 . Variation in patterns of valve morphogenesis between representatives of six biraphid diatom genera (Bacillariophyceae) . J. Phycol. , 35 : 1297 – 1312 .
- Cox , EJ . 2003 . Placoneis Mereschkowsky (Bacillariophyta) revisited: resolution of several typification and nomenclatural problems, including the generitype . Bot. J. Linn. Soc. , 141 : 53 – 83 .
- Cox , EJ . 2004 . Pore occlusions in raphid diatoms – a reassessment of their structure and terminology, with particular reference to the Cymbellales . Diatom , 20 : 33 – 46 .
- Cox , EJ and Williams , DM . 2000 . Systematics of naviculoid diatoms: the interrelationships of some taxa with a stauros . Eur. J. Phycol. , 35 : 273 – 282 .
- Daugbjerg , N and Andersen , RA . 1997 . A molecular phylogeny of the heterokont algae based on analyses of chloroplast-encoded rbcL sequence data . J. Phycol. , 33 : 1031 – 1041 .
- Desikachary , TV and Sreelatha , PM . 1989 . Oamaru diatoms . Bibliotheca Diatomol. , 19 : 1 – 330 .
- Doyle , JJ and Doyle , JL . 1987 . A rapid DNA isolation procedure for small quantities of fresh leaf tissue . Phytochem. Bull. , 19 : 11 – 15 .
- Edwards AR (editor) 1991 The Oamaru diatomite New Zealand Geol. Surv. Paleontol. Bull. 64 1 260
- Finlay , BJ . 2002 . Global dispersal of free-living microbial eukaryote species . Science , 296 : 1061 – 1063 .
- Fourtanier , E and Kociolek , JP . 1999 . Catalogue of the diatom genera . Diatom Res. , 14 : 1 – 190 .
- Geitler , L . 1932 . Der Formwechsel der pennaten Diatomeen (Kieselalgen) . Arch Protistenk. , 78 : 1 – 226 .
- Geitler , L . 1952a . Die Auxosporenbildung von Rhoicosphenia curvata . Österr. Bot. Z. , 99 : 78 – 88 .
- Geitler , L . 1952b . Untersuchungen über Kopulation und Auxosporenbildung pennater Diatomeen III. Gleichartigkeit der Gonenkerne und Verhalten des Heterochromatins bei Navicula radiosa . Österr. Bot. Z. , 99 : 469 – 482 .
- Geitler , L . 1952c . Untersuchungen über Kopulation und Auxosporenbildung pennater Diatomeen IV, V. IV Vierkernige Zygoten bei Navicula cryptocephala var. veneta fa. V. Allogamie bei Synedra rumpens var. fragilarioides . Österr. Bot. Z. , 99 : 598 – 605 .
- Geitler , L . 1973 . Auxosporenbildung bei einigen pennaten Diatomeen und die Cytologie von Cocconeis-Sippen . Österr. Bot. Z. , 122 : 299 – 321 .
- Gilbert DL 1995 SeqPup (a biosequence editor and analysis application) v 0.6f., http://iubio.bio.indiana.edu/soft/molbio/seqpup/java/seqpup-doc.html
- Gregory , W . 1857 . On new forms of marine Diatomaceae found in the Firth of Clyde and in Loch Fine . Trans. R. Soc. Edinb. , 21 : 473 – 542 .
- Guillard , RRL and Lorenzen , CJ . 1972 . Yellow-green algae with chlorophyllide . c. J. Phycol. , 8 : 10 – 14 .
- Hartley , B . 1986 . A checklist of the freshwater, brackish and marine diatoms of the British Isles and adjoining coastal waters . J. Mar. Biol. Ass. UK , 66 : 531 – 610 .
- Hartley B Barber HG Carter JR Sims PA 1996 An atlas of British diatoms, Biopress Bristol
- Hendey NI 1964 An Introductory Account of the Smaller Algae of British Coastal Waters, V. Bacillariophyeae (Diatoms) : Ministry of Agriculture, Fisheries and Food. Fishery Investigations Series IV. Her Majesty's Stationery Office London
- Hillis , DM and Bull , JJ . 1993 . An empirical test of bootstrapping as a method for assessing confidence in phylogenetic analysis . Syst. Biol. , 42 : 182 – 192 .
- Huelsenbeck JP Ronquist F 2001 MrBayes: Bayesian analysis of phylogeny v. 3.0b4 Biometrics 17 754 755
- Hustedt F 1927–1966 Die Kieselalgen Deutschlands, Österreichs und der Schweiz unter Berücksichtigung der übrigen Länder Europas sowie der angrenzenden Meeresgebiete In Dr L. Rabenhorsts Kryptogamenflora von Deutschland, Österreich und der Schweiz 7 Akademische Verlagsgesellschaft Leipzig
- Karayeva , NI . 1978 . Novye rod iz semejstva Naviculaceae West . Bot. Zh. , 63 : 1593 – 1596 .
- Karsten , G . 1896 . Unterschungen über Diatomeen I . Flora , 82 : 286 – 296 .
- Karsten G 1899 Die Diatomeen der Kieler Bucht. Wiss. Meeresunters Kiel 4 1 205
- Maddison WPR Maddison DR 1997 MacClade, v 3.07 Sinauer Associates Sunderland
- Mann DG 1981a Sieves and flaps: siliceous minutiae in the pores of raphid diatoms In Proceedings of the 6th Symposium on Recent and Fossil Diatoms (Ross, R. editor), 279–300. O. Koeltz, Koenigstein
- Mann , DG . 1981b . A note on valve formation and homology in the diatom genus Cymbella . Ann. Bot. , 47 : 267 – 269 .
- Mann , DG . 1982a . Structure, life history and systematics of Rhoicosphenia (Bacillariophyta) I. The vegetative cell of Rh. curvata . J. Phycol , 18 : 162 – 176 .
- Mann , DG . 1982b . Structure, life history and systematics of Rhoicosphenia (Bacillariophyta) II. Auxospore formation and perizonium structure of R. curvata . J. Phycol. , 18 : 264 – 274 .
- Mann , DG . 1983 . Symmetry and cell division in raphid diatoms . Ann. Bot. , 52 : 573 – 581 .
- Mann , DG . 1984 . Protoplast rotation, cell division and frustule symmetry in the diatom Navicula bacillum . Ann. Bot. , 53 : 295 – 302 .
- Mann , DG . 1989a . The diatom genus Sellaphora: separation from Navicula . Br. Phycol. J. , 24 : 1 – 20 .
- Mann , DG . 1989b . The species concept in diatoms: evidence for morphologically distinct, sympatric gamodemes in four epipelic species . Plant Syst. Evol. , 164 : 215 – 237 .
- Mann , DG . 1994a . Auxospore formation, reproductive plasticity and cell structure in Navicula ulvacea and the resurrection of the genus Dickieia (Bacillariophyta) . Eur. J. Phycol. , 29 : 141 – 157 .
- Mann DG 1994b The origins of shape and form in diatoms: the interplay between morphogenetic studies and systematics IN Shape and Form in Plants and Fungi (Ingram, D.S., Hudson, A.J. editors) 17–38 Academic Press London
- Mann , DG . 1998 . Lyrella cassiteriduma new species of marine epipelic diatom . Nordic J. Bot. , 18 : 503 – 512 .
- Mann , DG and Chepurnov , VA . 2004 . What have the Romans ever done for us? The past and future contribution of culture studies to diatom systematics . Nova Hedwigia , 79 : 237 – 291 .
- Mann DG Stickle AJ 1988 Nuclear movements and frustule symmetry in raphid pennate diatoms In: Proceedings of the 9th International Diatom Symposium (Round, F.E., editor) 281 289 Biopress, Bristol, and Koeltz Scientific Books Koenigstein
- Mann , DG and Stickle , AJ . 1989 . Meiosis, nuclear cyclosis and auxospore formation in Navicula sensu stricto (Bacillariophyta) . Br. Phycol. J. , 24 : 167 – 181 .
- Mann , DG and Stickle , AJ . 1991 . The genus Craticula . Diatom Res , 6 : 79 – 107 .
- Mann , DG and Stickle , AJ . 1993 . Life history and systematics of Lyrella . Nova Hedwigia , 106 : 43 – 70 .
- Mann , DG and Stickle , AJ . 1995 . Sexual reproduction and systematics of Placoneis (Bacillariophyta) . Phycologia , 34 : 74 – 86 .
- Mann , DG and Stickle , AJ . 1996 . The systematics of Stauroneis (Bacillariophyta) II. The life history of S. phoenicenteron and related species . Diatom Res. , 10 : 277 – 297 .
- Mann DG Stickle AJ 1997 Sporadic evolution of dorsiventrality in raphid diatoms, with special reference to Lyrella amphoroides sp nov. Nova Hedwigia 65 59 77
- Mann , DG , Simpson , GE , Sluiman , HJ and Möller , M . 2001 . rbcL gene tree of diatoms: a second large data-set for phylogenetic reconstruction . Phycologia , 40 ( 4, suppl. ) : 1 – 2 .
- Mann , DG , Chepurnov , VA and Idei , M . 2003 . Mating system, sexual reproduction and auxosporulation in the anomalous raphid diatom Eunotia (Bacillariophyta) . J. Phycol. , 39 : 1067 – 1084 .
- Mann , DG , McDonald , SM , Bayer , MM , Droop , SJM , Chepurnov , VA , Loke , RE , Ciobanu , A and du Buf , JMH . 2004 . Morphometric analysis, ultrastructure and mating data provide evidence for five new species of Sellaphora (Bacillariophyceae) . Phycologia , 43 : 459 – 482 .
- Medlin , LK and Kaczmarska , I . 2004 . Evolution of the diatoms: V. Morphological and cytological support for the major clades and a taxonomic revision . Phycologia , 43 : 245 – 270 .
- Medlin , LK and Round , FE . 1986 . Taxonomic studies of marine gomphonemoid diatoms . Diatom Res. , 1 : 205 – 225 .
- Mereschkowsky C 1902–3 Les types de l'endochrome Scripta Botanica Horti Univers. Imp. Petropol 21 1 106 [Russian], 107–193 [French]
- Mereschkowsky , C . 1903 . Über Placoneisein neues Diatomeen-Genus . Beih. Bot. Centralbl. , 15 : 1 – 30 .
- Montgomery RT Miller W 1978 Environmental and ecological studies of the diatom communities associated with the coral reefs of the Florida Keys Vol. II. Ph.D. dissertation, Florida State University College of Arts and Sciences
- Park RA 1992 The Ecology of Epipelic Diatoms in Loch Goil Ph.D. thesis University of Edinburgh Scotland U.K.
- Patrick , R . 1959 . New subgenera and two new species of the genus Navicula (Bacillariophyceae) . Not. Nat. , 324 : 1 – 11 .
- Patrick R Reimer CW 1966 The diatoms of the United States exclusive of Alaska and Hawaii Volume 1. Monographs Acad. Nat. Sci. Philad. 13 1 688
- Posada , D and Crandall , KA . 1998 . Modeltest: testing the model of DNA substitution . Bioinformatics , 14 : 817 – 818 .
- Roshchin AM 1994 Zhiznennye tsikly diatomovykh vodoroslej, Naukova Dumka Kiev
- Ross , R and Sims , PA . 1972 . The fine structure of the frustule in centric diatoms: a suggested terminology . Br. Phycol. J. , 7 : 139 – 163 .
- Ross , R and Sims , PA . 1985 . Some genera of the Biddulphiaceae (diatoms) with interlocking linking spines . Bull. Brit. Mus. (Nat. Hist.) , 13 : 277 – 381 .
- Round FE Crawford RM Mann DG 1990 The Diatoms Biology and Morphology of the Genera, Cambridge University Press Cambridge
- Schmid A-MM 2001 Value of pyrenoids in the systematics of the diatoms: their morphology and ultrastructure In: Proceedings of the 16th International Diatom Symposium (Economou-Amilli, A., editor) 1 31 Faculty of Biology University of Athens
- Schrader , H-J . 1969 . Die pennaten Diatomeen aus dem Obereozän von Oamaru, Neuseeland . Nova Hedwigia, Beih. , 28 : 1 – 124 .
- Strel’nikova NI 1992 Paleogenovye diatomovye vodorosli Izdatel'stvo S.-Peterburgskogo Universiteta, St. Petersburg
- Swofford DL 2002 PAUP*. Phylogenetic analysis using Parsimony. *and other methods Version 4.0b10 Sinauer Sunderland MA
- Williams DM Hartley B Ross R Munro MAS Juggins S Battarbee RW 1988 A coded checklist of British diatoms, ENSIS London
- Witkowski A Lange-Bertalot H Metzeltin D 2000 Diatom flora of marine coasts I In: Iconographia diatomologica 7 Diversity–Taxonomy–Identification (Lange-Bertalot, H., editor) 3 925 A.R.G. Gantner Ruggell Liechtenstein
- Witt ON 1886 Ueber den Polierschiefer von Archangelsk-Kurojedowo im Gouv. Simbirsk Verh. russ-kais. mineral. Ges. St. Petersb. Ser. 2 22 137 177