Abstract
A small thecate dinoflagellate was encountered during winter and spring in the phytoplankton community of a shallow, brackish and hypertrophic pond in the South of France. The abundance of material permitted its identification, using scanning electron microscopy, as Oblea rotunda Balech ex Sournia. However, morphological features showed some discrepancies with the typical characteristics of O. rotunda. Environmental hydrographic parameters in the pond were very different from marine ecosystems where O. rotunda is usually reported. In this polluted and alkaline pond, salinity was low, ranging from 1.1 to 6.2 p.s.u.; the maximum densities of O. rotunda (greater than 1 × 105 cells l − 1) were observed at temperatures around 13°C, after a Cylindrotheca closterium – Heterocapsa rotundata bloom in early spring. These two species and other abundant taxa of the phytoplankton had seasonal patterns that could explain the presence of O. rotunda, since they could have been a food for this heterotrophic species. As this population showed most of the morphological criteria of O. rotunda, but with some variability and a different ecology, it is better considered as a variant of the marine species, rather than as a new species. This study provides additional data on the ecological and morphological features of this poorly known small peridinioid species, previously only reported from open seas and oceans.
Introduction
In highly eutrophic ecosystems, phytoplankton is frequently studied because of algal blooms dominated by cyanobacteria, which limit the use of recreational waters and cause a nuisance for lakeside human populations. Algal flagellates in hypertrophic environments can reach high abundances and their motility allows them to cope with the poor light climate typical of these environments (Rojo & Alvarez Cobelas, Citation1993). The biology and ecology of flagellates need to be studied in order to understand spatial and temporal phytoplankton community dynamics in hypertrophic ecosystems. However, little data concerning these groups, especially dinoflagellates, is available (Romo & Miracle, Citation1994; Wasmund, Citation1994).
In south-east France, the hypertrophic Étang de Bolmon, a shallow and brackish pond, is characterized by high phytoplankton densities all the year round, promoted by high nutrient enrichment from domestic sewage effluents of the drainage basin. Owing to high phosphorus concentrations in the water, phytoplankton communities are dominated for most of the year by the cyanobacterium Planktothrix agardhii (Gomont) Anagnostidis & Komarek, which occurs at densities of 106 – 109 cells l − 1. Phytoplankton abundance and environmental parameters have been studied because proliferation of this species can lead to health problems (Codd et al., Citation1999; Duy et al., Citation2000). In this algal community, the small marine dinoflagellate Oblea rotunda (Lebour) Balech ex Sournia has been observed and scanning electron microscopy was used to observe its fine structure. O. rotunda was initially described from Plymouth Sound by Lebour (Citation1922) as Peridiniopsis rotunda Lebour, and has previously been reported only from open seas and oceans (Dodge, Citation1982; Sournia, Citation1986; Steidinger & Tangen, Citation1996). However, we have now studied its environmental preferences and its dynamics in a quite different habitat.
Materials and methods
The Étang de Bolmon is located in south-east France, near Marseilles (). It is about 5 km long, up to 1.5 km wide, and its total area is 0.58 km2. Because of accumulation of sediments, the mean depth is only 1 m. Brackish water is exchanged with the Étang de Berre (a brackish coastal lagoon), which explains the salinity of the pond. This exchange takes place through three canals cut in the sand bar called ‘le Jaï’. Four stations were chosen across this pond because of heterogeneous conditions and water inputs. Station 1 was located in the middle canal. Station 2 was located in the middle of the pond. On the south shore, station 3 was located near a channel into the Rove canal, which opens directly into the Étang de Berre. Freshwater enters mainly from the Cadière River, the single tributary whose drainage basin is heavily urbanized. This river receives large amounts of raw domestic sewage, accounting for the high phosphate and nitrate concentrations at station 4, located at the mouth of the Cadière River.
Water samples were collected 0.5 m below the surface, twice a month during winter and spring 2002. Subsamples of 500 ml were preserved in 5% formaldehyde. Temperature and dissolved oxygen concentrations were measured in the field using an oxythermometer (WTW Oxi 197) and salinity with a conductivity meter (WTW LF330); pH was measured in the laboratory (Metrohm-Herisau Titriskop E516). Nutrient analyses were carried out according to Mackereth et al. (Citation1978).
Subsamples for quantitative phytoplankton analysis were settled in 10 ml counting chambers after adequate dilution (Utermöhl, Citation1958). Cells were counted and measured at × 400 magnification under an Olympus IX70 inverted microscope fitted with phase contrast and a calibrated micrometer. For scanning electron microscopy (SEM), formaldehyde-fixed cells were isolated by micro-pipette and rinsed in distilled water. Cells were dehydrated in ethanol and dried to critical point using liquid carbon dioxide. Samples were coated with gold/palladium in a sputter coater (Bourrelly & Couté, Citation1980). The cells were then examined and photographed using a JEOL JSM-840A SEM.
The terminology used for thecal plates in this paper is the generally accepted scheme based on the Kofoid system as modified by Balech (Citation1980).
Results
Morphology
Cells observed in light microscopy and SEM were almost globular in shape and possessed a sulcal list extending below the hypotheca. Their mean width was 23.7 μm and mean apical axis length 21.8 μm (n = 320). The cingulum was equatorial or slightly sub-equatorial, with lists along both margins (, ). Scanning electron microscopy clearly revealed the shape, position and insertion of thecal plates ( – ). These were smooth and had many minute perforations on their surface (). The apical pore complex (APC) consisted of a nearly oval pore plate with an oval opening (Po) and a canal plate X (, ). The observed APC belongs to the D' type in the classification proposed by Toriumi & Dodge (Citation1993). Plate 1′ is clearly of meta type (five-sided; , , ). In most of the specimens, there were three apical plates (, ) but, in two of the cells observed, there were four apical plates (, ). These two specimens had different patterns of apical plate organization: one cell had a plate 2′ divided in two smaller plates, 2′* and 2′** (); the other had four true plates in the series (). In ventral view (, ), plate 6′′ was slightly higher than 1′′. In dorsal and apical views, plates 2′′, 3′′ and 4′′ appeared small (, , ) and plate 5′′ was rather large (, , ). The unique intercalary plate 1a was pentagonal, large, curved, and extended on the left part of the epitheca. It was in contact with five of the six precingular plates (, ). The girdle or cingulum consisted of three cingular plates. Plates c1 and c3 were short and c2 extended along the whole dorsal part of the theca (, ). Prominent lists on the epitheca and hypotheca bordering the cingulum were smooth (, ). Next to these lists, aligned minute pores surrounded the cingulum (, ). The sulcus, which was short and did not reach to the centre of the hypotheca (, ,), was bordered on the left side by a prominent list, well coiled (, ,). Only anterior sulcal plates Sa, Sd and the transitional plate t were visible, the others being hidden by the sulcal list (, ). Plate Sd was entirely located below the cingulum (, ). The hypotheca consisted of seven plates, five of which form the postcingular series. Plates 2′′′, 3′′′ and 4′′′ were almost equal in size, but 1′′′ and 5′′′ were smaller (, ). Of the two antapical plates, 1′′′′ was slightly smaller than 2′′′′ (, ). The general plate formula observed is Po, X, 3′ (or 4′?), 1a, 6′′, 4 (3 + t)c, 5?s, 5′′′, 2′′′′ (, , ), which is typical of the species Oblea rotunda (Lebour) Balech ex Sournia. We have noticed in SEM that many rod-shaped bacteria remain at the surface of the cells (e.g. , ), in spite of the preliminary isolation and treatment.
Figs 2 – 7. Line drawings of Oblea rotunda. Fig. 2. Ventral view of cell. Note that sulcal list extends below body of cell. Fig. 3. Dorsal view. Fig. 4. Antapical view. Figs 5, 6, 7. Apical views of cells with 3 (Fig. 5), and 4 apical plates (Figs 6 – 7). Note the division of plate 2′ (Fig. 6) and the different organization of the apical plates of these two specimens (Figs 6 – 7).
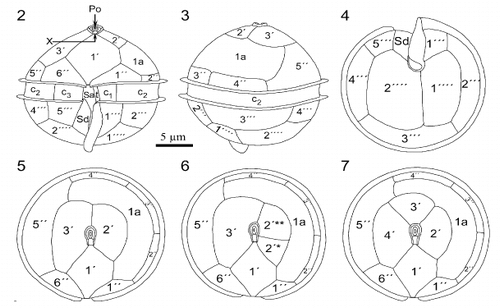
Figs 8 – 13. Oblea rotunda observed under scanning electron microscopy (SEM). Scale bars represent 10 μm. . Cell in ventral view showing the general plate arrangement. . Dorsal view. . Apical view of two cells with 3 () and 4 () apical plates showing epitheca tabulation. Note that girdle lists are totally smooth. . Antapical views showing the prominent left sulcal list coiled.

Figs 14 – 17. Oblea rotunda observed under SEM. Lateral views and detail of the apical pore complex (APC). Scale bars represent 10 μm in , and 1 μm in . . Right lateral view showing the sulcal list. Note the presence of bacteria on the surface of the cell. . Left lateral view. . Detail of the first apical plate (arrow) showing its meta pattern and the APC. . Detail of the apical pore and canal plate on a cell with 4 apical plates. Note the small perforations surrounding the APC.
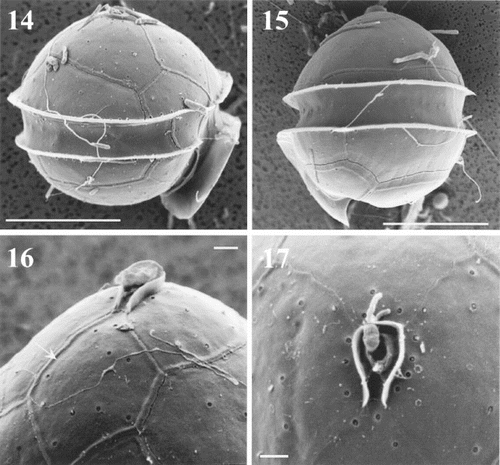
Fig. 18. Cell densities of Oblea rotunda at four stations (S1 to S4) in the Étang de Bolmon, from January 2002 to May 2002. Note the lower density scale for S4.
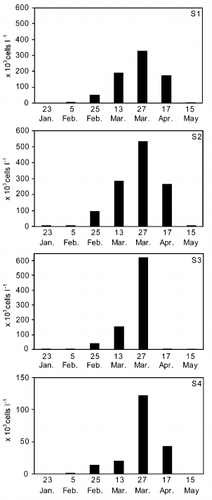
Figs 19 – 20. Hydrographic parameters in sampling stations during the study period (7 dates) when Oblea rotunda was present. Open circles: O. rotunda present at cell densities lower than 1 × 104 cells l − 1; shaded circles: cell densities between 1 × 104 and 1 × 105 cells l − 1; black circles: cell densities greater than 1 × 105 cells l − 1. . Temperature and salinity. . pH and dissolved oxygen concentration.
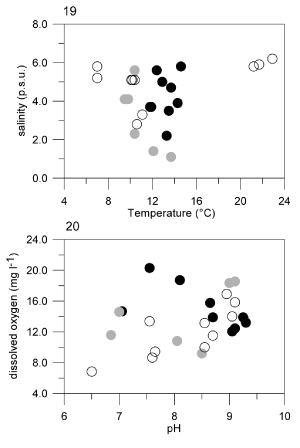
Ecology
During the study, Oblea rotunda was present in 25 samples, from January to May 2002. Since two samples per month were available for most of this period, the variations in temporal abundance of this dinoflagellate could be observed with a good resolution. The pattern was similar in all stations (). O. rotunda appeared in the Étang de Bolmon in January at very low densities (about 500 cells l − 1) in stations 2 and 3, but cell number increased in February and March, until a maximum was reached at the end of March, with 4 × 105 cells l − 1 (average of the four stations). From April to May, densities decreased to values similar to those observed in January. O. rotunda was observed only sparsely during the other months of the year. Cell density was highly heterogeneous among stations. Highest densities (6 × 105 cells l − 1) were found at S3 (). Cell densities were always lower at S4, located in the mouth of the polluted Cadière River. At this station, salinity was significantly lower, but PO4-P and NO3-N concentrations were significantly higher than in other parts of the Étang ().
Table 1. Selected environmental variables at the four sampling stations during the study period (means ± standard deviations, with ranges of variation in brackets). Differences among stations tested by one-way ANOVA – NS: non significant, *p<0.05, **p<0.01, ***p<0.001
O. rotunda reached its maximum densities (over 1 × 105 cells l − 1) at temperatures between 10 and 15°C () but living cells were observed from 7.0 up to 22.9°C. This species appeared in a salinity range from 1.1 to 6.2 p.s.u. () but no significant effect of salinity was observed on the cell concentration (r = − 0.1676, p = 0.445; ). This dinoflagellate was found in a wide range of pH (6.5 – 9.30) and when dissolved oxygen concentrations were relatively high (6.85 – 20.30 mg l − 1, ).
During the study, Oblea rotunda was present in a phytoplankton community dominated by the cyanobacterium Planktothrix agardhii (mean density 1.5 × 109 cells l − 1) and mean densities of other phytoplankton groups were markedly lower (). Among autotrophic phytoplankton, four of the dominant taxa, Cylindrotheca closterium (Ehrenb.) Reimann & Lewin, Heterocapsa rotundata (Lohmann) Hansen, Skeletonema spp. and Pyramimonas sp., showed a seasonal pattern of abundance that could be interpreted in relation to the presence of O. rotunda (). The ratio of the density of O. rotunda to these autotrophic species is about 1 to 100. C. closterium (Bacillariophyceae) and H. rotundata (Dinophyceae) densities increased from January to the middle of March and reached ca. 40 × 106 cells l − 1 during the bloom (). A rapid decline of these populations followed and, 2 weeks later, when O. rotunda reached its maximum, densities were less than 15 × 106 cells l − 1. Then, they decreased until May. Cell numbers of Skeletonema spp. were highest in early February (10 × 106 cells l − 1) and then decreased to the end of March (less than 4 × 105 cells l − 1), while the density of O. rotunda increased. A slight increase in the density of this diatom was observed when the dinoflagellate decreased at the end of March (). Thus, the peak abundance of these three autotrophic taxa occurred at different times from that observed for O. rotunda. In contrast, Pyramimonas sp. (Prasinophyceae) and O. rotunda had a similar seasonal pattern and they both reached a peak abundance at the end of March (). However, when O. rotunda decreased in April, Pyramimonas sp. population increased to its highest level (8.5 × 106 cells l − 1).
Table 2. Mean densities (106 cells l − 1) of Oblea rotund a and main phytoplanktonic groups recorded in the sampling stations during the study (7 dates)
Discussion
Specific features of Oblea rotunda in the Étang de Bolmon
Observations using light microscopy and SEM revealed that the dinoflagellate belongs to the order Peridiniales. Considering only the tabulation of both epitheca and hypotheca, it could belong to either of the genera Oblea Balech or Peridiniopsis Lemmermann. They have the same number of plates on the epitheca (Po, X, 3′, 1a, 6′′) and hypotheca (5′′′, 2′′′′). The major difference between these genera is the number of cingular plates. The genus Oblea has been distinguished from Peridiniopsis to include organisms that have a five-sided first apical plate, a large curved anterior intercalary and three cingular plates (Balech, Citation1964). Distinguishing between these genera using this criterion alone was discussed by Bourrelly (Citation1968) who proposed to group all species in the genus Peridiniopsis, but it is now obvious that these two genera are morphologically and ecologically different (Sournia, Citation1973, Citation1986). The prominent left sulcal list observed in our specimens is one of the distinctive characters of the species belonging to the Diplopsalis group (Abé, Citation1941; Sournia, Citation1986; Dodge & Toriumi, Citation1993; Steidinger & Tangen, Citation1996), now grouped in the family Diplopsalidaceae (Matsuoka, Citation1988). As the first apical plate is of the meta type, the relations of the 1a plate with the other plates of the epitheca and shape of 1′and 6′′ clearly identify the observed dinoflagellate as Oblea rotunda, initially described as Peridiniopsis rotunda Lebour (Citation1922).
Despite this identification based on morphology and plate formula, there are obvious morphological and ecological differences from previous descriptions of O. rotunda. A variable number of apical plates (3 or 4) in an Oblea population was reported for O. baculifera Balech, where the occasional presence of a fourth apical plate was described (Balech, Citation1971), but this has not previously been reported for O. rotunda. The specimen observed with two small plates, 2′* and 2′**, was probably the result of a division of the 2′ plate, and can be considered as O. rotunda. Consequently, only one specimen with four true apical plates has been encountered in our SEM investigations. Since it was not possible to see antapical and lateral views of this cell, it cannot be established whether it belongs to O. rotunda. However, other features of the epitheca and the size were similar to O rotunda and the cell was found in the same population. We suggest, therefore, that this was a morphological variation. Taylor (Citation1987) reported an important thecal plate variability in a clonal culture of Alexandrium tamarense (Lebour) Balech (formerly Protogonyaulax tamarensis) and of 63 epithecae examined by this author, 60 were normal with four apical plates, one had fewer apical and precingular plates and two had an extra apical plate, with different patterns. In our case, only a clonal culture of O. rotunda could confirm the presence of a new species or a (probably rare) morphological variant within the population. Another morphological difference appeared in our samples. Girdle lists were always completely smooth and not interrupted by spines, as is generally described or illustrated for O. rotunda (Wood, Citation1954; Dodge & Hermes, Citation1981; Dodge, Citation1982). Balech (Citation1964) mentioned the presence of girdle lists strengthened by radial spicules in his diagnosis of the genus Oblea, but he was uncertain of the persistence of this criterion. Lewis (Citation1990) described and illustrated precisely the hollow ribs supporting the girdle lists, also visible in light microscopy, but we have never observed these structures in our specimens. As phenotypic variability is frequent in dinoflagellates, we believe that it could be a morphological variant of the species, due to the unusual habitat for this species (Taylor, Citation1987).
Ecological data
The Étang de Bolmon, where we have encountered Oblea rotunda, is a brackish ecosystem with low salinity (< 10 p.s.u.), always well below the marine salt concentration of the Mediterranean Sea (ca. 35 p.s.u.). O. rotunda was first described from Plymouth Sound (Lebour, Citation1922), and the other two species of the genus have been reported only from marine environments. O. baculifera was reported from the South Atlantic Ocean (Balech, Citation1964; Citation1971), O. torta (Abé) Balech ex Sournia from the Pacific Ocean and O. rotunda from Australia, the North Sea, the Pacific Ocean and the British Isles (Lebour, Citation1922; Wood, Citation1954; Holligan et al., Citation1980; Dodge & Toriumi, Citation1993). Dodge (Citation1982) also mentioned records from the Antarctic, the Straits of Florida and the Gulf of Mexico. In the Atlantic Ocean, this species has recently been found in the lower part of a shallow estuary, in salinities ranging from 34.0 to 35.4 p.s.u. (Trigueros & Orive, Citation2001). Using a multivariate analysis, Holligan et al. (Citation1980) included O. rotunda in the Prorocentrum micans Ehrenberg group, which was associated with lower salinities around the British Isles, but salinity values (ca. 25 p.s.u.) were greater than those measured in the Étang de Bolmon. Salinity conditions here appear to be more favourable to species of Peridiniopsis, since some live in freshwater (Bourrelly, Citation1985) and others are adapted to brackish water (Ricard & Bourrelly, Citation1982; Bourrelly, Citation1985; Trigueros, Citation2000). Peridiniopsis species have never been reported from fully saline water, in contrast to most species of the family Diplopsalidaceae, which are generally marine (Sournia, Citation1986; Matsuoka, Citation1988). However, Diplopsalis acuta (Apstein) Entz fil., described as a typically freshwater species (Bourrelly & Couté, Citation1980), was recorded in the brackish water of the Caspian sea (Lindemann, Citation1927) indicating the possibility of ecological adaptation in this group. In our case, the marine species O. rotunda can live in a brackish ecosystem, the Étang de Bolmon. Consequently, this peridinioid appears to be euryhaline; it can survive in salinities ranging from 1.1 (Étang de Bolmon) to 35.4 p.s.u. (Trigueros & Orive, Citation2001).
O. rotunda did not occur only sporadically in our study, but during five consecutive months. Vegetative cells of O. rotunda were not senescent, but appeared to be actively dividing. Cell concentrations were never high compared with those observed for the dominant cyanobacterium Planktothrix agardhii. Cell densities found in the Étang de Bolmon were very high (up to 500,000 cells l − 1) compared to a marine environment, where the maximum density of O. rotunda was ca. 700 cells l − 1, during a Ceratium bloom (Olseng et al., Citation2002). It has been reported as a minor component of the phytoplankton in an estuary where its density was lower than 1000 cells l − 1 (Trigueros & Orive, Citation2001). Nevertheless, Lassus (Citation1988) reported O. baculifera as forming red tides in the Oslo fjord where densities reached 7.8 × 106 cells l − 1. In our study, cell density reached its maximum in a restricted temperature range, around 13°C, and decreased rapidly at lower or higher values. O. rotunda seemed to be quite stenothermic in our investigation since it was present only during winter and spring. It is described as a regular member of the coastal plankton around the British Isles from March to November (Dodge, Citation1982), unlike O. baculifera which is described as preferring cold waters from the South Atlantic and the North Pacific Oceans (Balech, Citation1964; Steidinger & Tangen, Citation1996).
Rather than selective requirements for temperature, salinity or nutrients, the dynamics of O. rotunda in the winter-spring season may be explained by the proliferation of particular autotrophic species. Only a few species of dinoflagellates can be considered as autotrophic (Gaines & Elbrächter, Citation1987) and those of the Diplopsalis group are regarded as strictly heterotrophic (Naustvoll, Citation2000). Heterotrophic dinoflagellates are often associated with blooms of autotrophic species, mainly diatoms and dinoflagellates (Hansen, Citation1991; Jeong, Citation1999; Olson & Strom, Citation2002; Stelfox-Widdicombe et al., Citation2004). The Cylindrotheca-Heterocapsa bloom observed in the middle of March, corresponding to the diatom spring bloom, could have been an opportunity for the development of various grazers, including O. rotunda. The delay observed between the peak abundances of these species can be attributed to the commonly called ‘hide and seek’ relationship in predator-prey interactions (Begon et al., Citation1996). Olseng et al. (Citation2002) observed the growth of O. rotunda after an autumnal Ceratium bloom but no grazing incidents involving these species. Tiselius & Kuylenstierna (Citation1996) observed that the development of heterotrophic dinoflagellates (Gymnodinium cf. spirale and cf. Gymnodinium sp.) occurred at the expense of a diatom spring bloom, dominated by Skeletonema costatum. Similarly, O. rotunda in our samples responded to the Cylindrotheca-Heterocapsa bloom by an accelerated growth from 13 March. These results suggest that C. closterium and H. rotundata could have been prey for the dinoflagellate, and this is in agreement with Hansen (Citation1991), who reports observations of O. rotunda being abundant in association with Heterocapsa triquetra and diatoms. However, the dynamics of Skeletonema spp. in the Étang de Bolmon were different. From a maximum of 10 × 106 cells l − 1 (cf. Tiselius & Kuylenstierna, Citation1996) in early February, the density of these diatoms decreased while the O. rotunda population increased. Predation by O. rotunda on the Skeletonema spp. population could explain this decline, but this cannot be verified as it coincided with the increase in the C. closterium and H. rotundata populations, and competition for light or nutrients among these species could have limited the growth of Skeletonema spp.
As no feeding or prey captures were observed in our samples, experimental data may help us to understand the food preferences of O. rotunda. Several studies of heterotrophic nutrition of marine dinoflagellate species have been conducted with cultures, including O. rotunda (Gaines & Elbrächter, Citation1987; Strom & Buskey, Citation1993; Naustvoll, Citation2000). Species in the Diplopsalis group (Diplopsalis, Oblea, Zygabikodinium) are regarded as feeding generalists (Naustvoll, Citation2000). In culture experiments, various members of the cryptomonad, dinoflagellate and diatom classes were able to support growth of O. rotunda (Strom & Buskey, Citation1993). No prey size selectivity was observed and Oblea could feed phagotrophically on species much smaller and much larger than itself (Hansen, Citation1991; Strom & Buskey, Citation1993). This supports our hypothesis of predation on C. closterium, H. rotundata or Skeletonema spp. On the other hand, Strom & Buskey (Citation1993) observed that O. rotunda could not grow when fed with a small prasinophyte (Mantonellia squamata) and this could explain why the dynamics of Pyramimonas sp. and O. rotunda were quite similar in our study, with no apparent interaction between them. However, the increase in the population of Pyramimonas sp. in April, when O. rotunda density fell below 20 × 104 cells l − 1, could have been due to the weaker predation pressure, but grazing of O. rotunda on this prasinophyte cannot be proved without experiment. Grazing on the dominant species, Planktothrix agardhii, probably occurred since dinoflagellates capture a wide range of food items from bacteria to copepods (Jeong, Citation1999; Naustvoll, Citation2000) but, because of the different scales of density (ratio of 1 to 104), it is not possible to determine whether O. rotunda had an impact on this cyanobacterium.
Oblea rotunda may have entered the Étang de Bolmon with seawater inflow from the Étang de Berre. However, this species has not been reported in earlier records of the phytoplankton in this system (Kim, Citation1981; Kim & Travers, Citation1984). By contrast, low densities of O. rotunda were observed in the Étang de Berre during our study, but it reached higher abundances in the Étang de Bolmon (Chomérat, unpublished). Therefore, the appearance of the species in the brackish Étang de Bolmon may be due to the germination of resting cysts present on the sediment surface when conditions become favourable. Cysts with a thick membrane, which permit survival during unfavourable periods (West & Fritsch, Citation1927; Taylor, Citation1987), are produced by O. rotunda, as shown by Lewis (Citation1990). However, the development of viable cells of O. rotunda from cysts has yet to be demonstrated; no cysts were found in the water and we did not sample bottom-sediments.
Using only morphological and ecological criteria, it is difficult to decide whether this population of Oblea rotunda from the Étang de Bolmon should be regarded as a new variety or a new species. Morphological variations (phenotypic) are frequently observed in the peridinioid group (Taylor, Citation1987). This dinoflagellate population, adapted to this particular environment, should, in our view, be considered as a morphological and ecological variant of the species. Before proposing a new species, further investigations (e.g. clonal cultures) are needed. Moreover, confirmation of a new species would require molecular comparisons with different populations of O. rotunda from various marine and brackish environments, and with the other Diplopsalidaceae and Peridiniopsis species from similar habitats to our specimens.
Acknowledgements
The authors thank Mr R. Garnier for carrying out much of the routine sampling and nutrient analyses, and Mr P. Webber for his suggestions on the manuscript. We are grateful to Prof. J. Lewis, Prof. M. J. Dring and an anonymous referee for valuable comments on this article. The study was funded by the Provence-Alpes-Côte-d'Azur Regional Council and the Groupement d'Intérêt Public pour la Réhabilitation de l'Étang de Berre.
References
References
- Abé , TH . 1941 . Studies on protozoan fauna of Shimoda Bay. The Diplopsalis group . Records Oceanography, Japan , 12 : 121 – 144 .
- Balech , E . 1964 . El plankton de mar del plata durante el periodo 1961 – 62 . Bol. Inst. Biol. Mar. Mar del Plata , 4 : 1 – 49 .
- Balech , E . 1971 . Microplancton de la campaña oceanogràfica Productividad III . Rev. Mus. Argent. Cienc. Nat. ‘Bernardino Rivadavia’, Hidrobiol , 3 : 1 – 202 .
- Balech , E . 1980 . On thecal morphology of Dinoflagellates with special emphasis on circular and sulcal plates . An. Centro Cienc. del Mar y Limnol. Univ. Nal. Auton. Mexico , 7 : 57 – 68 .
- Begon M Harper JL Townsend CR 1996 Ecology. Individuals, Populations and Communities Blackwell Science, Oxford
- Bourrelly , P . 1968 . Notes sur les Péridiniens d'eau douce . Protistologica , 4 ( 1 ) : 5 – 14 .
- Bourrelly P 1985 Les Algues d'Eau Douce. Initiation à La Systématique. Tome 3. Les Algues Bleues et Rouges. Les Eugléniens, Péridiniens et Cryptomonadines Boubée, Paris
- Bourrelly , P and Coute , A . 1980 . Trois Dinophycées d'eau douce observées en microscopie électronique à balayage . Cryptogamie : Algologie , 1 : 161 – 169 .
- Codd , GA , Bell , SG , Kaya , K , Ward , CJ , Beattie , KA and Metcalf , JS . 1999 . Cyanobacterial toxins, exposure routes and human health . Eur. J. Phycol , 34 : 405 – 415 .
- Dodge JD 1982 The Marine Dinoflagellates of the British Isles Her Majesty's Stationery Office, London
- Dodge , JD and Hermes , H . 1981 . A revision of the Diplopsalis group of dinoflagellates (Dinophyceae) based on material from British Isles . Bot. J. Linnean Soc , 83 : 15 – 26 .
- Dodge , JD and Toriumi , S . 1993 . A taxonomic revision of the Diplopsalis group (Dinophyceae) . Bot. Mar , 36 : 137 – 147 .
- Duy , TN , Lam , PKS , Shaw , GR and Connell , DW . 2000 . Toxicology and risk assessment of freshwater cyanobacterial (blue-green algal) toxins in water . Rev. Environ. Contam. Toxicol , 163 : 113 – 186 .
- Gaines G Elbrächter M 1987 Heterotrophic nutrition In: Biology of Dinoflagellates (Taylor, F.J.R., editor) 224 268 Blackwell Scientific Publications, Oxford
- Hansen , PJ . 1991 . Quantitative importance and trophic role of heterotrophic dinoflagellates in a coastal pelagial food web . Mar. Ecol. Prog. Ser , 73 : 253 – 261 .
- Holligan , PM , Maddock , L and Dodge , JD . 1980 . The distribution of Dinoflagellates around the British isles in July 1977: a multivariate analysis . J. Mar. Biol. Ass. U.K , 60 : 851 – 867 .
- Jeong , HJ . 1999 . The ecological roles of heterotrophic dinoflagellates in marine planktonic community . J. Eukaryot. Microbiol , 46 : 390 – 396 .
- Kim KT 1981 Le Phytoplancton de l'Étang de Berre : Composition Spécifique, Biomasse et Production; Relations Avec les Facteurs Hydrologiques, les Cours d'Eau Afférents et le Milieu Marin Voisin (Méditerrannée Nord-occidentale) Thèse Doct. Univ. Aix-Marseille II, Marseille
- Kim , KT and Travers , M . 1984 . Le phytoplancton des étangs de Berre et Vaïne (Méditerranée Nord-occidentale) . Int. Revue Ges. Hydrobiol , 69 : 361 – 388 .
- Lassus P 1988 Plancton Toxique et Plancton d'Eaux Rouges Sur les Côtes Européennes IFREMER, Plouzané
- Lebour , MV . 1922 . Plymouth Peridinians: I. Diplopsalis lenticulata and its relative . J. Mar. Biol. Ass. U.K , 12 : 795 – 818 .
- Lewis , J . 1990 . The cyst-theca relationship of Oblea rotunda (Diplopsalidaceae, Dinophyceae) . Br. Phycol. J , 25 : 339 – 351 .
- Lindemann , E . 1927 . Über einige Dinoflagellaten des Kapischen Meeres . Arch. Protistenkd , 59 : 417 – 422 .
- Mackereth FJH Heron J Talling JF 1978 Water analysis: some revised methods for limnologists Freshwater Biological Association Scientific Publication no. 36
- Matsuoka , K . 1988 . Cyst-theca relationships in the Diplopsalid group (Peridiniales, Dinophyceae) . Rev. Paleobot. Palyn , 56 : 95 – 122 .
- Naustvoll , L-J . 2000 . Prey size spectra and food preferences in thecate heterotrophic dinoflagellates . Phycologia , 39 : 187 – 198 .
- Olseng , CD , Naustvoll , L-J and Paasche , E . 2002 . Grazing by the heterotrophic dinoflagellate Protoperidinium steinii on a Ceratium bloom . Mar. Ecol. Prog. Ser , 225 : 161 – 167 .
- Olson , MB and Strom , SL . 2002 . Phytoplankton growth, microzooplankton herbivory and community structure in the southeast Bering Sea: insight into the formation and temporal persistence of an Emiliania huxleyi bloom . Deep-Sea Res. II , 49 : 5969 – 5990 .
- Ricard , M and Bourrelly , P . 1982 . Quelques algues microscopiques du lagon de l'atoll de Clipperton (Pacifique tropical nord) . Cryptogamie: Algologie , 3 : 25 – 31 .
- Rojo , C and Alvarez Cobelas , M . 1993 . Taxonomy and ecology of phytoplankton in a hypertrophic, gravel-pit lake. II. Cryptophyceae, Euglenophyceae, Dinophyceae . Nova Hedwigia , 57 : 47 – 63 .
- Romo , S and Miracle , MR . 1994 . Population dynamics and ecology of subdominant phytoplankton species in a shallow hypertrophic lake (Albufera of Valencia, Spain) . Hydrobiologia , 273 : 37 – 56 .
- Sournia , A . 1973 . Catalogue des espèces et taxons infraspécifiques de dinoflagellés marins actuels publiés depuis la révision de J. Schiller I. Dinoflagellés libres . Nova Hedwigia , 48 : 1 – 92 .
- Sournia A 1986 Atlas du Phytoplancton Marin. 1 : Introduction, Cyanophycées, Dictyochophycées, Dinophycées et Raphidophycées Éditions du Centre National de la Recherche Scientifique, Paris
- Steidinger KA Tangen K 1996 Dinoflagellates In: Identifying Marine Diatoms and Dinoflagellates (Tomas, C.R., editor) 387 598 Academic Press, San Diego
- Stelfox-Widdicombe , CE , Archer , SD , Burkill , PH and Stefels , J . 2004 . Microzooplankton grazing in Phaeocystis and diatom-dominated waters in the southern North Sea in spring . J. Sea Res , 51 : 37 – 51 .
- Strom , SL and Buskey , EJ . 1993 . Feeding, growth, and behavior of the thecate heterotrophic dinoflagellate Oblea rotunda . Limnol. Oceanogr , 38 : 965 – 977 .
- Taylor FJR 1987 Dinoflagellate morphology In: Biology of Dinoflagellates (Taylor, F.J.R., editor) 24 91 Blackwell Scientific Publications, Oxford
- Tiselius , P and Kuylenstierna , M . 1996 . Growth and decline of a diatom spring bloom: phytoplankton species composition, formation of marine snow and the role of heterotrophic dinoflagellates . J. Plankt. Res , 18 : 133 – 155 .
- Toriumi , S and Dodge , JD . 1993 . Thecal apex structure in the Peridiniaceae (Dinophyceae) . Eur. J. Phycol , 28 : 39 – 45 .
- Trigueros , JM . 2000 . Peridiniopsis salina (Peridiniales, Dinophyceae), a new species of brackish dinoflagellate from Urdaibai estuary, North Spain . Phycologia , 39 : 126 – 133 .
- Trigueros , JM and Orive , E . 2001 . Seasonal variations of diatoms and dinoflagellates in a shallow, temperate estuary, with emphasis on neritic assemblages . Hydrobiologia , 444 : 119 – 133 .
- Utermöhl , H . 1958 . Zur Vervollkommnung der quantitativen Phytoplankton - Methodik . Mitt. Internat. Verein. Limnol , 9 : 1 – 39 .
- Wasmund , N . 1994 . Phytoplankton periodicity in a eutrophic coastal water of the Baltic Sea . Int. Revue Ges. Hydrobiol , 79 : 259 – 285 .
- West GS Fritsch FE 1927 A treatise on the British freshwater algae University Press, Cambridge
- Wood , EJF . 1954 . Dinoflagellates in the Australian region . Aust. J. Mar. Freshw. Res , 5 : 171 – 351 .