Abstract
Littoral diatom assemblages from different natural substrates (stones, mud, reeds) were used for estimating trophic status in seven perialpine lakes near Salzburg (Austria). Four trophic status indices were compared with the actual chemical data and the annual averages of total phosphorus concentrations. Diatom assemblages on different substrates indicated significantly different trophic status for the site. Indicators based on epiphytic diatoms from young reed stalks correlated strongly with the concentrations of total phosphorus, so that reeds were found to be the most suitable substrate for bioindication. Bioindication based on three of the four indices studied (Rott's TDIA, Schönfelder's TS, van Dam's TDW) was positively correlated with actual phosphorus concentration at each site. While other indices indicated higher trophic status than expected according to annual averages of total phosphorus, Rott's TDIA was found to be the most applicable to perialpine lakes.
Introduction
Eutrophication is the most widespread form of lake pollution on a global scale, and has many deleterious impacts on aquatic systems (Harper, Citation1992). In addition to increasing overall primary production, eutrophication causes considerable changes in biochemical cycles and biological communities. For example, decrease in the ratio of N : P often results in primary production shifting from primarily diatoms, and other small edible algae, towards larger cyanobacteria that are stronger competitors for nitrogen (Tilman et al., Citation1996) and more resistant to grazing (Reynolds, Citation1984). A major concern is that lake ecosystems can become unstable and biodiversity may be lost during eutrophication (Margalef, Citation1968).
Two main approaches can be used for monitoring lake trophic status; direct chemical analyses or bioindicators. The use of bioindicator species is considered to have advantages over intermittent chemical analyses, because organisms integrate environmental influences (Cox, Citation1991). For this reason, the choice of suitable indicator organisms or groups of organisms is crucial.
Diatoms are an abundant, diverse and important component of algal assemblages in freshwater lakes. They comprise a large portion of the total algal biomass over a broad spectrum of lake trophic status. Moreover, they are well suited for studies of eutrophication because individual species are sensitive to changes in nutrient concentrations and ratios (Tilman et al., Citation1982).
Periphytic diatoms have been used for monitoring streams and two basic approaches are used today. Based on the work of Kolkwitz & Marsson (Citation1908), autecological indices were developed to indicate levels of pollution from the ecological preferences and tolerances of the species assemblages (e.g. Zelinka & Marvan, Citation1961, Lange-Bertalot, Citation1979). In contrast, Patrick's early monitoring studies (Patrick & Strawbridge, Citation1963) relied primarily on diatom diversity as a general indicator of river ‘health’. Many advances in the use of diatoms and other algae for monitoring stream and river quality have been reviewed recently by Whitton et al. (Citation1991).
It is a different situation in the case of monitoring lake water quality. Estimation of trophic status of lakes is based on (i) annual averages of total phosphorus or chlorophyll-a concentration, (ii) long-term observation of phytoplankton or (iii) diatom remains in profundal sediment samples from the deepest locations in lakes (Stoermer & Smol, Citation1999). On the other hand, use of littoral diatoms for lake water quality monitoring is rare because of the great microhabitat variability found in littoral diatom communities (Schönfelder et al., Citation2002).
The present study focuses on microhabitat variability in the littoral zone of perialpine lakes and its influence on the assessment of trophic status. In contrast to previous studies, our sampling design allows us to control for effects of different microhabitats (substrates). We tried to answer the following questions:
1. | Do diatom assemblages on different substrates sampled at the same sites result in different trophic status assessments? | ||||
2. | Which of the substrates studied is the most suitable for bioindication of site trophic status? | ||||
3. | Which of the trophic status indices studied provides the best indicator of the trophic status of the sites? |
Methods and localities
We studied 18 sites in seven perialpine lakes near Salzburg, Austria () covering the spectrum from (ultra)-oligotrophic to meso-eutrophic. Sites within each lake represent either natural habitats or those with human influence (beaches, recreational areas). The investigations were carried out in July 2000 (in Mondsee, Attersee and Wallersee) and July 2001 (all seven lakes).
List of localities (including sampled substrates: r – reeds, st – stones, s – sediment / mud):
1. | Mo1 – Mo6: Mondsee (Dachsbrücke, r, st, s; Kreuzstein r, st; Stony beach, st; Loibichl beach, r, st, s; Baggersee, stream inflow, r, st, s; Schwarzindien, r, st, s) | ||||
2. | At7 – At10: Attersee (Weyregg, st; Steinbach, st; Weissenbach, st; Burgau beach, st) | ||||
3. | Wa11 – Wa14: Wallersee (Seebrunn, r, st, s; Marieninsel, r, st, s; Wallerbach, steam inflow, r, st, s; railway station, r, st, s) | ||||
4. | Gr: Grabensee, r, st, s | ||||
5. | Mat: Mattsee, r, st, s | ||||
6. | Ob: Obertrumer See, r, st, s | ||||
7. | Hol: Holzöster See, r, st, s |
Limnological parameters, and the population structures and quantity of pelagic organisms in these lakes have been monitored for several decades. Their trophic development in the past and their present trophic status is therefore well documented (Dokulil & Jagsch, Citation1992; Dokulil, Citation1993). Littoral algae, however, have been studied only occasionally (Kann, Citation1958), focusing on the taxonomy or the floristics of blue-green algae, without any reference to environmental characteristics.
The geographical positions, basic morphometric and hydrological data of the lakes are summarized in . The limnological conditions and trophic status are indicated as annual averages of selected physical and chemical variables. Annual averages are representative for each lake, but not for the individual sampling points, whose chemical features are summarised in .
Table 1. Geographic and morphometric data for the seven Austrian lakes studied, and annual averages of environmental variables (Dokulil, unpubl.)
Table 2. Actual values of environmental variables at investigated sites (single sampling July 2000 and July 2001) Mo – Mondsee, At – Attersee, Wa – Wallersee, Gra – Grabensee, Ob – Obertrumer See, Ma – Mattsee, Hol – Holzöster See)
Water samples were collected in 500 ml glass bottles, 10 cm beneath the lake surface, using standard methods (Hindák, Citation1978). Conductivity and pH were measured in situ using mobile instruments (WTW Company, Germany) and nutrients (N, P, Si) were analysed by standard methods (DEV, Citation1981). At each site, one water sample was taken for chemical analysis () and three samples from each of the three types of substrate were taken if available. The substrates investigated were: stones (epilithon), submerged parts of young reed stalks (Phragmites australis, epiphyton), and fine bottom mud (epipelon). Epilithon was brushed from the stones with a toothbrush and the upper layer of epipelon was pipetted off with a vacuum suction system (Sládečková, Citation1962). Epiphyton was sampled with the substrate (section of young reed stalks) and placed in plastic bags. All reed stalks (15 cm sections) were collected from 50 cm below the water surface in the inner part of the littoral zone.
Diatom frustules were mounted in Naphrax and identified according to Krammer & Lange-Bertalot (Citation1986, Citation1988, Citation1991 a,Citation b ). Relative abundances of individual diatom species were estimated by counting 400 individuals from each Naphrax preparation. Pielou's evenness index (Legendre & Legendre, Citation1998) was computed for each sample.
Four trophic indices were calculated for each sample: (i) TDIA according to Rott (Citation1999), representing the arithmetic average of indicator values weighted by relative species abundance and fidelity; (ii) two modifications of a trophic index based on indicator values according to van Dam et al., (Citation1994), representing the arithmetic average of species indicator values, and either unweighted (TDU) or weighted (TDW) by the relative abundance of these species; (iii) TS according to Schönfelder et al. (Citation2002), representing the arithmetic average of ln TP opt (assemblage optimum for total phosphorus in μg l − 1) weighted by the relative abundance of species.
Differences in species richness and evenness among substrates and lakes (full data set) were analysed using two-way main effect ANOVA. Tukey–Kramer multiple comparison tests (at p = 0.05) were used to examine all pairs of treatment means. In the analysis of differences in bioindication among substrates, we used two-way ANOVA with randomized block design on a reduced data matrix containing only sampling points ( = blocks) with all three substrates sampled. Tukey-Kramer multiple comparison tests (at p = 0.05) were again used to examine all pairs of treatment means.
We used multivariate statistical methods for the exploratory analyses using the software package CANOCO for Windows, version 4 (ter Braak & Šmilauer, Citation1998). We analysed the complete diatom data set with a detrended correspondence analysis (DCA) using Hill's scaling. The results indicated that a linear approach is appropriate for the elucidation of a relationship between diatom variability and environmental factors (data not shown). We therefore used principal components analyses (PCA) for further analysis. The species data were arcsine transformed while environmental data were log-transformed (y = log [ x + 1]). These exploratory analyses can be summarized as follows: (i) Species variation among substrates was analysed with partial PCA. Prior to analysis, 6 sampling points, which contained only one or two of the three substrates, were excluded from the data matrix (= reduced data matrix). Sampling points (in the form of dummy variables, cf. ter Braak & Šmilauer, Citation1998) were used as covariables. The relationships between the species ordination and trophic indices (TDIA, TS, TDU, TDW; i.e. supplementary environmental data) were evaluated by non-parametric Spearman correlation (rs) after PCA. To confirm the exploratory analysis, we used redundancy analysis (RDA). We tested the hypothesis H0: there is no difference in species composition among the different substrates. Types of substrate were used as ‘dummy’ environmental variables; sampling points were used as covariables. The Monte Carlo permutation test (999 permutations, split-plot design) was used to assess the significance of the RDA analysis. (ii) Variation in species composition among sampling points was analysed using partial PCA on the reduced data matrix. Substrates (in the form of dummy variables) were used as covariables. The relationships between the species ordination and the set of environmental variables (pH, conductivity, concentrations of Si, N, P) and trophic indices (TDIA, TS, TDU, TDW) were evaluated by Spearman's rank correlation (rs) after PCA.
Analysis of covariance (ANCOVA) was used to assess the relationship between total phosphorus (TP) and the trophic indices. At first, the homogeneity-of-slopes model was used to test whether the continuous predictor variable (TP) had a different effect at different levels of categorical independent variables (substrates). Since none of these analyses revealed non-homogeneity-of-slopes (data not shown), only classical ANCOVA was used in further analyses. All analyses were performed with the statistical package STATISTICA 6.0 (StatSoft, Inc.).
Results
Influence of substrate on species richness, variation and bioindication
In total, 148 diatom taxa were found during our investigation. Achnanthes minutissima, Cymbella microcephala, Amphora pediculus, Fragilaria delicatissima, Fragilaria pinnata and Denticula tenuis were the most frequent species.
Species richness per sample ranged from 5 to 32 species in 2001. The lowest average species richness per sample was found in Holzöstersee (mean ± standard deviation, 18 ± 1 species, n = 6) and the highest in Grabensee (25 ± 4, n = 6). The average species richness of samples from different substrates was: stones, 20 ± 5 (n = 36); mud samples, 21 ± 8 (n = 28); reeds, 23 ± 5 (n = 28). Neither lakes nor substrates had a significant effect on species richness (two-way main effect ANOVA; p > 0.05). On the other hand, evenness of samples from reeds was significantly lower than from other substrates (Pielou's evenness: stones 0.78a ± 0.09; mud samples 0.78a ± 0.10; reeds 0.50b ± 0.10; results of Tukey-Kramer tests shown by superscripts). Diatom assemblages on reeds were dominated by 1 – 2 species while communities on stones and on mud showed more balanced species representation. Similar patterns were also found in three of the lakes (Mondsee, Attersee, Wallersee) in 2000 (data not shown).
The PCA ordination of samples for 2001, with sampling points as covariables, is shown in . The covariables explained 45.7% of the total variance. The first and second axes explained 18.2% and 6.0% of the variance of species data, respectively, after fitting covariables. The analysis clearly separated samples from different substrates in the ordination diagram (). Samples from reed stalks were dominated by a few species – Achnanthes minutissima, Fragilaria delicatissima, Cymbella aspera and C. tumidula (). The occurrence of Diatoma tenuis, Cymbella naviculiformis, Navicula cryptotenella and Cocconeis pediculus characterised the assemblages on stones, and Achnanthes lanceolata and species of the genera Navicula and Fragilaria characterised the mud samples ().
Fig. 2. Partial PCA ordination diagram of samples (reduced data matrix). Sampling points were used as covariables. (Mo – Mondsee, Wa – Wallersee, Gr – Grabensee, Ob – Obertrumer See, Mat – Mattsee, Hol – Holzöster See; TDW , TDU – indices according to van Dam et al. (Citation1994), TDIA – index according to Rott (Citation1999); TS – ln TP opt according to Schönfelder et al. (Citation2002); open square, r = reeds, open diamond, s = sediment/mud, open circle, st = stones). Arrows indicate correlations of indices with the first two ordination axes.
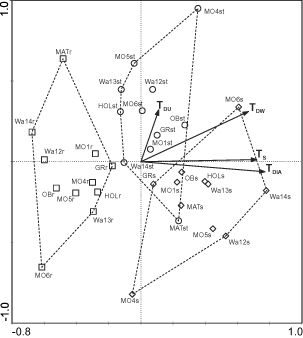
Fig. 3. Partial PCA ordination diagram of species (reduced data matrix). Sampling points were used as covariables. Only species with a higher fit (cf. ter Braak & Šmilauer, Citation1998) in the analysis are shown. Species names are abbreviated. Arrows indicate correlations of indices with the first two ordination axes (see Fig. 2).(Achhol – Achnanthes holsatica, Achlan – Achnanthes lanceolata , Achmin – Achnanthes minutissima, Achsp – Achnanthes sp., Ampped – Amphora pediculus, Ampthu – Amphora thumensis, Cocped – Cocconeis pediculus, Cymaff – Cymbella affinis, Cymasp – Cymbella aspera, Cymgra – Cymbella gracilis, Cymlep – Cymbella leptoceros, Cymnav – Cymbella naviculiformis, Cymsp – Cymbella sp., Cymtum – Cymbella tumidula, Diaten – Diatoma tenuis, Episp – Epithemia sp., Eunexi – Eunotia exiqua, Fracon – Fragilaria construens, Fradel – Fragilaria delicatissima, Frapin – Fragilaria pinnata, Gomang – Gomphonema angustum, Gomexi – Gomphonema exiguum, Gomgra – Gomphonema gracilis, Gomoli – Gomphonema olivaceum, Gompar – Gomphonema parvulum , Gomtru – Gomphonema truncatum, Navctl – Navicula cryptotenella, Navpup – Navicula pupula, Navrei – Navicula reinhardtii, Navrhy – Navicula rhynchocephala, Nitsp – Nitzschia sp., Opeols – Opephora olsenii, Tabflo – Tabellaria flocculosa).
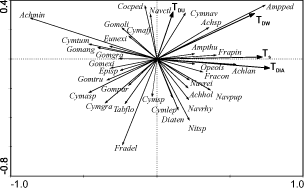
The position of samples on the first PCA axis correlated with three of the four indices (TDIA: rs = 0.74; TDW: rs = 0.67; TS: rs = 0.73; p < 0.001; ). In contrast, TDU was not significantly correlated with the first axis (rs = 0.06, N.S) but was with the second axis (rs = 0.38, p = 0.02). Thus, three of the four trophic indices indicated lower values of trophy for samples from reeds than for samples from stones and mud.
The RDA revealed a similar pattern (data not shown). The difference in diatom composition among substrates was found to be significant in the Monte Carlo permutation test (p = 0.002).
This is supported by the significant differences in mean TDIA, TS and TDW among substrates (Tukey–Kramer test results indicated by superscripts: TDIA – reeds, 1.60a, stones, 1.94ab, mud, 2.26b; TS – reeds, 3.24a, stones, 3.68b, mud, 3.81b; TDW – reeds, 3.75a, stones, 4.26b, mud, 4.28b). In contrast, no significant differences in mean TDU were found among substrates (reeds, 3.97; stones, 4.11; mud, 4.00).
Variation among sampling points
shows the PCA ordination of samples from 2001 with substrates as covariables which explained 17.7% of the total variance. The first and second axes explained 18.8% and 15.3% of the variance of species data, respectively, after fitting the covariables. The first axis can be interpreted as the trophic gradient from the eutrophic and mesotrophic lakes in the left part of the diagram to the oligotrophic ones on the right. This trend was confirmed by the correlation of sample scores for the first axis vs environmental factors. Total phosphorus (TP) and nitrogen (N) showed significant negative correlations with the first axis (TP: rs = − 0.38; N: rs = − 0.60; p < 0.05; ). Three of the four trophic indices also showed significant negative correlations with the first axis (TDIA: rs = − 0.48; TDW: rs = − 0.55; TS: rs = − 0.37; p < 0.05; TDU: rs = − 0.15, p > 0.05). In contrast to the clumped patterns of samples in most of the lakes studied, the samples from Mondsee are scattered throughout the diagram. This is due to the greater number of sampling points and larger variation in the character of the littoral zone of this lake.
Fig. 4. Partial PCA ordination diagram of samples, environmental factors and trophic indices. Substrates were used as covariables. Arrows indicate correlations of environmental factors and indices with the first two ordination axes; actual environmental variables: pH, conductivity, Si = silicon, N = nitrogen, TP = total phosphorus; indices: TDW , TDU, TDIA and TS, as in Fig. 2; substrates: r = reeds, s = sediment/mud, st = stones; localities: open circle, Mo = Mondsee, open diamond, Ob = Obertrumer See, filled square, Wa = Wallersee, open square, Mat = Mattsee, filled circle, Hol = Holzöster See, open rectangle, Gr = Grabensee.
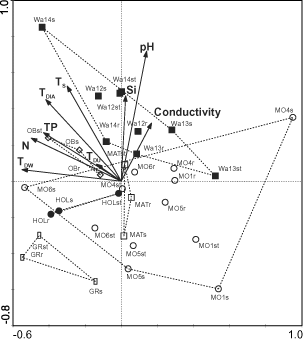
Mondsee was characterized by the occurrence of the following species: Cymbella laevis, C. affinis, Fragilaria delicatissima, Fragilaria pinnata (). Wallersee was distinguished by the dominance of Achnanthes lanceolata and by the occurrence of Navicula decussis and N. subadnata. Grabensee could be characterized by the occurrence of Fragilaria pinnata, Navicula cryptotenella and Cyclotella sp.
Fig. 5. Partial PCA ordination diagram of species. Substrates were used as covariables. Only species with a higher fit (cf. ter Braak & Šmilauer, Citation1998) in the analysis are shown. Species names are abbreviated.(Achbio – Achnanthes bioretii, Achlae – A. laevis, Ampmon – Amphora montana, Astfor – Asterionella formosa, Aulgra – Aulacoseira granulata, Aulsp – Aulacoseira sp., Cycato – Cyclotella atomus, Cycpse – C. pseudostelligera, Cymhel – Cymbella helvetica, Cymlae – C. laevis, Cymsil – C. silesiaca, Denten – Denticula tenuis, Diaehr – Diatoma ehrenbergii, Diasp – Diatoma sp., Fracap – Fragilaria capucina, Franan – Fragilaria nanana, Frasp – Fragilaria sp., Navcapr – Navicula capitatoradiata, Navdec – N. decussis, Navexi – N. exigua, Navlan – N. lanceolata, Navscu – N. scutelloides, Navsp – Navicula sp., Navsubad – N. subadnata, Pinmic – Pinnularia microstauron, other abbreviations see Fig. 3).
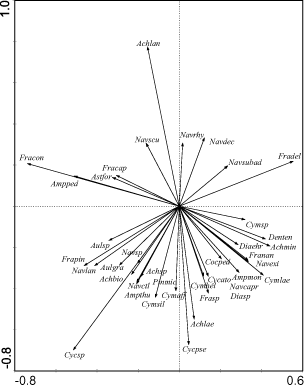
The results from 2000, when only three lakes were sampled, revealed similar patterns (data not shown).
Relationships among total phosphorus and trophic indices
Except for TDW, the trophic indices increased significantly with increasing TP concentration (, ). Regression lines of TDIA and TS significantly differed in their intercepts among different substrates (ANCOVA; , ). For TDIA, the index based on samples from the mud displayed systematically higher values than those from other substrates. For TS, the index based on samples from reeds displayed systematically lower values than those from stones and mud. In general, indices based on samples from mud-sediments and stones exhibited a greater variation with many outliers that strongly affect the analyses, especially at higher levels of TP ().
Table 3. Results of analysis of covariance (ANCOVA), testing relationships among total phosphorus (TP) concentrations and trophic indices at different levels of categorical independent variables (substrates). Analysis tests whether the continuous predictor variable (TP) has an effect on trophic indices and if the regression lines differ in their intercepts
Fig. 6. Relationships between concentration of total phosphorus in μg l − 1 (TP) and each trophic index for the sampling points studied. A: TDIA – index according to Rott (Citation1999); B: TS – ln TP opt according to Schönfelder et al. (Citation2002); C: TDU – unweighted index according to van Dam et al. (Citation1994), D: TDW – weighted index according to van Dam et al. (Citation1994). In each panel, regression lines of each index on TP are fitted separately for each type of substrate: full line, open triangle = reeds, dashed line, filled circle = stones, dotted line, open square = sediment. Stars in the panels A, B and D denote significantly different (at p < 0.05) intercepts of regression line from the other lines (see Table 3).
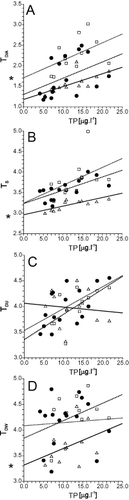
There was no significant correlation between the mean TP from all sites in each lake () and the annual averages of the same parameter for 2001 (TPA, ; rs = 0.45, p = 0.31). Only the mean TDIA, eTs and TDW based on samples from reed stalks showed a significant correlation with TPA (, ).
Table 4. Spearman rank correlation between TPA and trophic indices for different substrates (average of samples per lake). Degrees of freedom differ for each pair of variables. *, ** – Significant correlation at p = 0.05, 0.01
Fig. 7. Relationships between annual averages of total phosphorus in μg l − 1 (TPA) and mean values of each trophic index for each lake (mean TDIA, TDW, TDU) and transformed optimal TP concentrations according to Schönfelder et al. (Citation2002) (eTs); A – sediments, B – stones, C – reeds. Ranges of each trophic index were not standardized before analyses and thus do not allow direct comparison. Vertical broken line divides each diagram into an oligotrophic segment on the left and mesotrophic on the right according to the OECD categories based on TPA. Only stones were available in Attersee (oligotrophic lake).
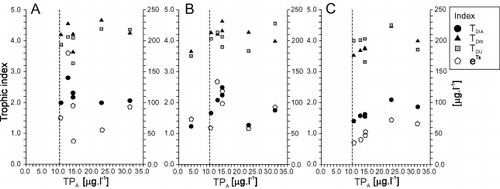
With annual TP averages of 10 – 35 μg l – 1, all lakes can be classified, according to the OECD (1982), as mesotrophic, except for the ultra-oligotrophic Attersee. Neither TDW nor TDU reflected the trophic status (TPA) of the lakes, irrespective of the type of substrate (). According to their indicators (cf. van Dam et al., Citation1994), Attersee should be mesotrophic, and all the others meso-eutrophic. Similarly, based on samples from mud surfaces, TDIA did not reflect the trophic status of the lakes over the full range of TPA. Based on samples from stones and reeds, however, TDIA did reflect the trophic status of the lakes (TPA) in 55.5% and 66.6% of the total lake numbers (). After recalculation of Schönfelder's TS (ln TP opt) to real optimum TP values (eTs), we found that diatoms in general indicate higher TP concentrations than expected from annual averages (TPA).
Discussion
Influence of substrate type on species variation and bioindication
Comparison of the three substrates studied indicated that (i) species composition differs greatly among substrates and that (ii) the trophic status assessment is influenced by these differences. Diatom assemblages occurring on reed stalks tend to indicate low trophic status, epilithic assemblages indicate medium or higher values, while epipelon overestimates the trophic status of the site when compared to the trophic situation deduced from open water samples.
Epilithon has previously been reported (Round, Citation1991) as the most suitable substrate for monitoring streams but Round also referred to the importance of representative sampling and the need to eliminate contamination of epilithon by epipelon (in slowly moving parts of the streams) or epiphyton (on macroscopic red and green algae). Although stones were available in the perialpine lakes studied, they could be missing in other still-water systems, such as floodplain lakes or fishponds. Their use for monitoring fresh-water systems is therefore limited, although stony ‘artificial’ substrates can substitute for stones in some cases (Sládečková, Citation1962). Washing can eliminate contamination of epilithon by epipelon. Nevertheless, washing stones does not correct for the shift in species composition of epilithon caused by competition with epipelon (Round, Citation1991). Therefore, the contamination of stones by epipelon probably caused great variability in species composition of assemblages on stones and assemblage trophic indices calculated from species composition ( and ).
While deep-water sediments have been used for bioindication (Stoermer & Smol, Citation1999), our results indicate that littoral mud samples are less useful. The contradictions arise from the different origins of these two sediment types. Deep-water sediments contain mainly dead sedimented planktonic diatoms, whereas sediments of the littoral zone contain a mixture of living epipelic (epipsammic) cells, diatoms drifted from other substrates (plants) due to wind disturbance, metaphyton (free living organisms associated with littoral higher plants) and dead frustules from the plankton. The proportion of planktonic species reached a maximum of 60% (centric diatoms 10 – 20%, different planktonic species of Fragilaria 20 – 30% and Nitzschia 5 – 10%). Asterionella formosa and Cyclostephanos dubius were the most frequent planktonic diatoms in sediment samples.
Although it is possible to use macroscopic plants for sampling diatom assemblages, there are some limitations for using them in running waters: no single species of macroscopic plant occurs throughout the length of most rivers, so that different species must be used (Round, Citation1991). Moreover, aquatic macrophytes, unlike stones or sand grains, are not inert surfaces for the microorganisms that grow on them, and they can potentially influence the species composition of diatoms. Eminson & Moss (Citation1980) studied the influence of macrophytes on periphytic algal communities and showed host specificity of the periphyton communities only in lakes of low trophic status. In contrast, periphyton communities on different plants showed great similarity in the eutrophic lakes that they studied. Cattaneo (Citation1978) found that the species composition of epiphyton on artificial plants did not differ from that on natural plants.
In contrast to running waters, some macroscopic plants are commonly found in most standing waters and thus provide unbiased results. Young reed stalks (Phragmites australis) were used for sampling during our investigations because they are common in the littoral zone of the lakes. However, slightly different diatom assemblages may be found on old and dead stems (Grimes et al., Citation1980). Young reed stalks were preferred since they represent the earliest stages of the periphyton succession, while the stones and mud largely accumulate dead diatom frustules from previous seasons. Since new reed stalks grow each spring, it was expected that epiphytic diatom assemblages would be the best indicator of the actual chemical parameters. Moreover, this substrate is less contaminated by epipelon and phytoplankton, which results in lower variation in species composition. The analyses confirmed this assumption.
Besides the potential influence of the host species, several other environmental factors influence the epiphyton communities, e.g. time and vertical distribution. Seasonal changes were found by Müller (Citation1994) in the composition of epiphytic algae on Phragmites australis, and variations in the vertical distribution of epiphytic algae were revealed by Hoagland & Peterson (Citation1990). This is why standardization of sampling methods is necessary in biomonitoring.
In summary, the use of various substrates for sampling diatoms results in different indications of lake trophic status. Comparison of trophic indices based on different sampling procedures and substrates can give erroneous results.
Comparison of trophic indices
In using attached littoral algae for biomonitoring, the selection of a suitable index is of prime importance. The majority of published concepts were developed for streams (Lange-Bertalot, Citation1979; Rott, Citation1999). The checklist of indicator species published by van Dam et al. (Citation1994) is recommended for fresh waters. The list of indicator species constructed for lakes by Hofmann (Citation1994) contains only a limited number of species. Recently, Schönfelder et al. (Citation2002) published ecological optima and tolerances of littoral diatoms in the Brandenburg region, Germany. Altogether 113 diatom species were used for trophic status assessment of seven lakes in 2001, a high proportion of which was covered by Rott's list of indicator species (TDIA75 species, TDU, TDW 65 species, TS 67 species).
Our indirect gradient analysis, based on periphytic diatoms, ordered the lakes according to their trophic status (). This gradient was significantly correlated with the concentrations of nitrogen and phosphorus, and with TDIA, TS and TDW but, when the trophic indices were compared directly with the independent measures of trophic conditions (TP and TPA), according to the delineation from OECD (1982), the diatom indices indicated a higher trophic status than given by the OECD scheme, especially at higher values of TP, TPA (, 7).
In many cases, the trophic status of the littoral zone is higher than that deduced from water samples in the centre of the lake (Komárková & Marvan, Citation1987) but the increased development of littoral algae is considered to be a symptom of the eutrophication process in lakes (Eiseltová, Citation1994). In spite of this, Rott's TDIA was found to be the most applicable to perialpine lakes in Austria. Nevertheless, the results are strongly substrate-specific (see above). According to these results, only the combination of TDIA and reed stalks (i) provided meaningful bioindication of actual and average total phosphorus concentrations, and (ii) more or less reflected the variation in the trophic conditions of the lakes studied.
The same sampling methods were applied to shallow fishponds in the Czech Republic (Kitner & Poulíčková, Citation2003) and the best indicator system for their evaluation was found to be the index given by van Dam et al. (Citation1994). These results were influenced by the strong dominance of centric diatoms and other planktonic species, which are not included in Rott's index (Rott, Citation1999). Our experience thus led us to the conclusion that each region or freshwater biotope type needs its own calibration. The occurrence of species is influenced not only by nutrients, but a complex of environmental variables and biotic interactions. From this point of view, perialpine lakes are closer to alpine streams (Rott, Citation1999), than to German lakes (Schönfelder et al., Citation2002).
The advantage of using littoral diatoms in lakes is most evident in the results obtained for specific sites rather than in the more general indication for the whole lake, which can more easily be assessed by sampling the phytoplankton.
Conclusions
We found that:
1. | Diatom assemblages on different substrates, sampled at the same sites, result in different assessments of trophic status. | ||||
2. | Diatom assemblages sampled on young reeds are the most suitable for bioindication of the trophic status of sites. | ||||
3. | Bioindication based on three of the four indices studied (TDIA, TS, TDW) was positively correlated with phosphorus concentration at each site. | ||||
4. | Except for TDIA based on samples from reeds and stones, the indices studied failed to indicate lake trophic status – they indicated a higher trophic status than expected according to the TP annual averages. |
In summary, assessments based on littoral diatoms were found to be useful for evaluation of specific trophic situations and variations along the shores of lakes (point-pollution sources). We recommend careful standardization of sampling methods, because the results are substrate-biased. Our results lead us to the conclusion that, for the most accurate estimation of trophic status, it is necessary to use a bioindicator system developed for a related region and/or ecosystem.
Acknowledgements
This study was supported by grants from the Ministry of Education of the Czech Republic ‘Kontakt’ Czech Republic – Austria (No. 2000/3) and the Ministry of Education of the Czech Republic (No. 153100014). The comments of the reviewers are gratefully acknowledged. Special thanks to Mary Morris-Burgis for linguistic corrections.
References
References
- Cattaneo , A . 1978 . The microdistribution of epiphytes on the leaves of natural and artificial macrophytes . Br. Phycol. J , 13 : 183 – 188 .
- Cox EJ 1991 What is the basis for using diatoms as monitors of river quality? In Use of Algae for Monitoring Rivers (Whitton, B.A., Rott, E. & Friedrich, G., editors) 33 40 Universität Innsbruck
- Dev 1981 Deutsche Einheitsverfahren zur Wasser-, Abwasser- und Schlamm-Untersuchung Physikalische, chemische, biologische und bakteriologische Verfahren 1 Aufl., Loseblatt-Sammlung, Wiley-VCH, Weinheim
- Dokulil , M . 1993 . Long-term response of phytoplankton population dynamics to oligotrophication in Mondsee, Austria . Verh. Internat. Verein. Limnol , 25 : 657 – 661 .
- Dokulil , MT and Jagsch , A . 1992 . Dynamics of phosphorus and nitrogen loading and its effects on phytoplankton in Mondsee, Austria . Hydrobiologia , 243/244 : 389 – 394 .
- Eiseltová M (ed.) 1994 Restoration of lake ecosystems – a holistic approach IWRB Publ. 32, Oxford
- Eminson , D and Moss , B . 1980 . The composition and ecology of periphyton communities in freshwaters. 1. The influence of host type and external environment on community composition . Br. Phycol. J , 15 : 429 – 446 .
- Grimes , JA , Clair , LL and Rushforth , SR . 1980 . A comparison of epiphytic diatom assemblages on living and dead stems of the common grass Phragmites australis . Great Basin Naturalist , 40 : 223 – 228 .
- Harper D 1992 Eutrophication of Freshwaters Chapman Hall, London
- Hindák F (ed.) 1978 Sladkovodné riasy [Freshwater algae] National Pedagogical Publisher, Bratislava
- Hoagland , D and Peterson , ChG . 1990 . Effects of light and wave disturbance on vertical zonation of attached microalgae in a large reservoir . J. Phycol , 26 : 450 – 457 .
- Hofmann , G . 1994 . Aufwuchs-Diatomeen in Seen und ihre Eignung als Indikatoren der Trophie . Bibliotheca Diatomologica , 30 : 1 – 241 .
- Kann , E . 1958 . Der Algenaufwuchs in der eulitoralen Zone alpiner und norddeutscher Seen . Verh. Internat. Ver. Limnol , 13 : 311 – 319 .
- Kitner , M and Poulíčková , A . 2003 . Littoral diatoms as indicators for the eutrophication of shallow lakes . Hydrobiologia , 506/509 : 519 – 524 .
- Kolkwitz , R and Marsson , M . 1908 . Ökologie der pflanzliche Saprobien . Berichte der Deutsche Botanische Gesellschaften , 26 : 505 – 519 .
- Komárková , J and Marvan , P . 1987 . The role of algae in the littoral zone of carp ponds . Arch. Hydrobiol. Beih , 27 : 239 – 249 .
- Krammer K Lange-Bertalot H 1986 Bacillariophyceae. 1. Teil In Süsswasserflora von Mitteleuropa 2/1 (Ettl, H., Gerloff, J., Heynig, H. & Mollenhauer, D., editors) 876 pp G. Fischer Verlag, Stuttgart
- Krammer K Lange-Bertalot H 1988 Bacillariophyceae. 2. Teil In Süsswasserflora von Mitteleuropa 2/2 (Ettl, H., Gerloff, J., Heynig, H. & Mollenhauer, D., editors) 596 pp G. Fischer Verlag, Stuttgart
- Krammer K Lange-Bertalot H 1991a Bacillariophyceae. 3. Teil In Süsswasserflora von Mitteleuropa 2/3 (Ettl, H., Gerloff, J., Heynig, H. & Mollenhauer, D., editors) 576 pp G. Fischer Verlag, Stuttgart
- Krammer K Lange-Bertalot H 1991b Bacillariophyceae. 4. Teil In Süsswasserflora von Mitteleuropa 2/4 (Ettl, H., Gerloff, J., Heynig, H. & Mollenhauer, D., editors) 437 pp G. Fischer Verlag, Stuttgart
- Lange-Bertalot , H . 1979 . Pollution tolerance of diatoms as a criterion for water quality estimation . Nova Hedwigia , 64 : 285 – 304 .
- Legendre P Legendre L 1998 Numerical Ecology. Second English Edition Elsevier, Amsterdam
- Margalef R 1968 Perspectives in Ecological Theory University of Chicago Press, Chicago
- Müller , U . 1994 . Seasonal development of epiphytic algae on Phragmites australis in a eutrophic lake . Arch. Hydrobiol , 129 : 273 – 292 .
- Patrick , R and Strawbridge , D . 1963 . Variation in the structure of natural diatom communities . American Naturalist , 97 : 51 – 57 .
- Reynolds CS 1984 The Ecology of Freshwater Phytoplankton Cambridge University Press, Cambridge
- Rott E (ed.) 1999 Indikationslisten für Aufwuchsalgen in Österreichischen fliessgewässern. Teil 2: Trophieindikation sowie geochemische Präferenz; taxonomische und toxikologische Anmerkungen WWK, Bundesministerium für Land-und Forstwirtschaft, Wien
- Round FE 1991 Use of diatoms for monitoring rivers In Use of algae for monitoring rivers (Whitton, B.A., Rott, E. & Friedrich, G., editors) pp. 25 – 32 Universität Innsbruck
- Sládečková , A . 1962 . Limnological investigation methods for the periphyton (‘Aufwuchs’) community . Botanical Review , 28 : 286 – 350 .
- Schönfelder , I , Gelbrecht , J , Schönfelder , J and Steinberg , ChEW . 2002 . Relationships between littoral diatoms and their chemical environment in northeastern German lakes and rivers . J. Phycol , 38 : 66 – 82 .
- Stoermer F Smol J (eds) 1999 The diatoms: applications for the environmental and earth sciences Cambridge Univ. Press, Cambridge
- Tilman , D , Kiesling , R , Sterner , R , Kilham , SS and Johnson , FA . 1996 . Green, blue-green and diatom algae: Taxonomic differences in competitive ability for phosphorus, silicon and nitrogen . Archiv für Hydrobiologie Beiheft Ergebnisse der Limnologie , 106 : 473 – 485 .
- Tilman , D , Kilham , SS and Kilham , P . 1982 . Phytoplankton community ecology: the role of limiting nutrients . Annual Review of Ecology and Systematics , 13 : 349 – 372 .
- Ter Braak CJF Šmilauer P 1998 CANOCO reference manual and Userś guide to CANOCO for Windows: Software for Canonical Community Ordination (version 4) Microcomputer Power, Ithaca, NY
- Van Dam , H , Mertens , A and Sinkeldam , J . 1994 . A coded checklist and ecological indicator values of freshwater diatoms from the Netherlands . Neth. J. Aquatic Ecol , 28 : 117 – 133 .
- Whitton BA Rott E Friedrich G (eds) 1991 Use of algae for monitoring rivers Inst. Botany, Universität Innsbruck
- Zelinka , M and Marvan , P . 1961 . Zur Präzisierung der biologischen Klassifikation des Reinheit fliessender Gewässer . Archiv für Hydrobiologie , 57 : 389 – 407 .