Abstract
The availability of extensive experimental data and remarkable intra- and interspecific variation in breeding behaviour make Achnanthes Bory sensu stricto an especially good model for studying the reproductive and population biology of pennate diatoms. In most Achnanthes species studied, auxospore formation is accompanied by biparental sexual reproduction, but we found uniparental auxosporulation in Achnanthes cf. subsessilis. Auxosporulation appears to be apomictic and follows contraction of the contents of unpaired cells and then a mitotic division, which is normally acytokinetic: one nucleus aborts before the cell develops into an auxospore. Rarely, both daughter nuclei survive and cytokinesis produces two auxospores (two auxospores per mother cell is highly unusual in pennate diatoms); one may abort. Expansion of auxospores is not accompanied by deposition of a transverse perizonium, but a longitudinal perizonium is produced and consists of a wide central strip (structurally similar to the araphid valve) and at least one narrow lateral strip. This newly discovered asexual lineage in Achnanthes is discussed in relation to other reproductive systems found in the genus, and also in relation to the ‘sex clock’ hypothesis concerning the adaptive significance of the diatom life cycle. Brief information on chloroplast division and nuclear dynamics over the cell cycle is also presented.
Introduction
Understanding the genetic structure of populations and how it varies in time and space is a prerequisite for studying adaptation, divergence, speciation and extinction. In turn, the genetic make-up of populations is influenced primarily by the organization of the breeding system (Holsinger, Citation2000). Each of the three basic modes of reproduction – outbreeding, inbreeding and apomixis – has distinctive consequences for the genetics of higher plant populations (e.g. Richards, Citation1997; Holsinger, Citation2000), but the same principles apply to all sexual eukaryotic organisms, whatever their level of organization, including diatoms.
The diatoms (Bacillariophyceae) are the most species-rich group of algae, with enormous ecological significance and high impact on global primary production (e.g. Field et al., Citation1998; Mann, Citation1999). The evolutionary success of diatoms is still poorly understood, but it has been suggested that ‘their refined control over sexuality’ may play a part (Lewis, Citation1984).
The basic plan of the life cycle in diatoms is the same throughout (e.g. Drebes, Citation1977; Mann, Citation1993; Edlund & Stoermer, Citation1997): diatoms are diplontic and multiply vegetatively by mitotic cell division during most of their life cycle. Vegetative multiplication is accompanied by a gradual reduction in cell size, described by the ‘MacDonald – Pfitzer’ Rule (Round, Citation1972; Crawford, Citation1981). Like many other algae, diatoms (if they have not lost sexuality secondarily) are ‘discontinuously sexual’ (Lewis, Citation1987), i.e. the potential for sexuality is not expressed in all individuals of a species; it is realized only if the cells are sufficiently small and if environmental conditions are suitable. Sexual reproduction is associated, not only with genetic recombination, as in other eukaryotes, but also with cell size restoration: soon after the fusion of gametes, the zygote expands rapidly (in hours to days). This stage of development of the zygote is called the ‘auxospore’ and the process itself ‘auxosporulation’ (Round et al., Citation1990; Edlund & Stoermer, Citation1997). Following a series of morphological transformations, the auxospore then acquires a new silicified cell wall and its mitotic progeny resume the characteristic shape and behaviour of the premeiotic vegetative cells, except that they are much bigger. The maximal size that can be achieved by the auxospore through expansion, as well as the size threshold below which vegetative cells become inducible to sexual activity, are known as the ‘cardinal points’ in the diatom life history and are more or less species-specific (Geitler, Citation1932; Drebes, Citation1977; Mann et al., Citation1999, but see also Davidovich, Citation1994).
The mechanics of sexual reproduction vary immensely among diatoms (e.g. Geitler, Citation1973; Drebes, Citation1977; Round et al., Citation1990; Mann, Citation1993), apparently extensively diversified by natural selection (Lewis, Citation1984). There are often striking correlations with taxonomy. For example, Eunotia and its relatives are characterized by pairing between the gametangia, production of a single gamete per gametangium, isogamy via a copulation tube formed through the fusion of apical papillae, and auxospore expansion through modification and growth of the copulation tube (Geitler, Citation1973; Mann et al., Citation2003). At low taxonomic levels (species and below), however, differences in the visible events of auxosporulation are generally unremarkable, except that in some groups there appears to have been secondary reduction or loss of sexuality (Geitler, Citation1973, Citation1985; Drebes, Citation1977; Round et al., Citation1990). This, however, is rare and auxosporulation is usually associated with allomictic sexual processes (‘biparental’ sexual reproduction); it is only rarely automictic (‘uniparental’ sexual reproduction, in which the sexual events occur within a single cell) or asexual.
In contrast to the morphology and cytology of auxosporulation, biochemical and genetic aspects of mating, such as the existence and nature of heterothallism, have remained largely unexplored in diatoms. Indeed, it has only recently been realized that these more hidden aspects of reproductive systems can exhibit considerable variation, both within a single species and between apparently closely related species (Roshchin, Citation1994 a; Roshchin & Chepurnov, Citation1999; Mann, Citation1999; Mann et al., Citation1999; Chepurnov & Mann, Citation1997, Citation1999, Citation2000).
The significance of this variation needs to be determined, in relation to both the ecology of the organisms and systematics. We believe that, as elsewhere in biology, progress will be facilitated by concentrating on one or a few model organisms and there are strong arguments for choosing Achnanthes Bory sensu stricto (i.e. excluding the small-celled freshwater species now classified in Achnanthidium Kützing and related segregate genera: Round et al., Citation1990; Round & Bukhtiyarova, Citation1996; Round & Basson, Citation1997). This includes the widespread species A. brevipes C. Agardh and A. longipes C. Agardh, which are very common in coastal microphytobenthic communities (McIntire & Moore, Citation1977), together with the marine A. javanica Grunow and A. septata A. Cleve, and several terrestrial species, e.g. A. coarctata (Brébisson in W. Smith) Grunow in Cleve et Grunow and A. inflata (Kützing) Grunow. Achnanthes species are easy to cultivate and extensive experience has accumulated about how to manipulate the life cycle experimentally, through rapid alteration of cell size (von Stosch, Citation1942, Citation1965; Roshchin, Citation1982, Citation1984 a,Citation b , Citation1994 a,Citation b ; Roshchin & Chepurnov, Citation1992, Citation1993, Citation1999; Mizuno, Citation1994; Chepurnov & Roshchin, Citation1995; Mann, Citation1999; Lewis et al., Citation2002). Crucially, remarkable variation in breeding behaviour has been discovered within the genus, at both intra- and interspecific levels (Roshchin, Citation1994 b; Chepurnov & Mann, Citation1997, Citation1999, Citation2000; Mann, Citation1999; Mann & Chepurnov (unpublished observations)). Until recently, however, there was a major disadvantage to the use of Achnanthes as a model system, in that there were no reliable reports of continuously asexual lineages, in contrast to the many reports of allogamous (Idei, Citation1991; Mizuno, Citation1994; Roshchin, Citation1994 b; Chepurnov & Roshchin, Citation1995; Roshchin & Chepurnov, Citation1999; Mann, Citation1999) or apparently automictic, paedogamous reproduction (Lüders, Citation1862; Karsten, Citation1897, Citation1899; see also Geitler, Citation1936, Citation1957, Citation1985), in which two gametes are formed by a single unpaired cell which then fuse to produce an auxospore.
Over 10 years ago, we observed unusual behaviour in clones of an Achnanthes isolated from the Black Sea (Roshchin & Chepurnov, Citation1993). Here, auxospores developed exclusively from single unpaired cells, without any visible sign of gametogenesis. This pattern of auxosporulation seemed to be obligate for the clonal cultures investigated and it did not change during several successive generations (Roshchin, Citation1994 a). Unfortunately, no data were obtained on nuclear behaviour and division during the formation of the auxospores, so that we could not distinguish between autogamy (i.e. a uniparental, sexual pathway of development) and apomixis. We have now isolated new clones from the same location. Detailed investigations of these isolates provide strong evidence for asexual auxosporulation. Hence there is a full range of mating systems in Achnanthes.
Materials and methods
Sampling
Long-term observations (1984 – 1991) of the seasonal dynamics of the marine benthic sublittoral diatoms in Karadag Bay (Black Sea, S.E. Crimea, Ukraine) (Roshchin & Chepurnov, unpublished observations) showed that the Achnanthes studied in this paper is restricted to the mouth of a small brook near Karadag Biostation (Kurortnoe, Feodosia). It could be found regularly in epilithic samples collected in May – September (Chepurnov, Citation1989). On 14 June 2001, a sample of microphytobenthos scraped from a stone taken at an atidal depth of c. 0.2 m was found to contain Achnanthes cells, as in previous years. A few mg of the material collected were placed into a plastic container, with 10 ml of sea water. After storage for a week at 6°C the sample was removed to Ghent University, Belgium, where, on 25 June, a small amount was transferred into polystyrene 90-mm Petri dishes with 40 ml of culture medium. The medium was based on filtered sterilized sea water (c. 32‰) collected from the North Sea, diluted with distilled water to c. 16‰ (the salinity of the Black Sea) and enriched with nutrients as specified by Roshchin (Citation1994 a; see also Chepurnov & Mann, Citation1997). After 3 days, the Achnanthes cells in the crude culture looked very healthy and, in a few cases, had even begun to auxosporulate, producing auxospores from single unpaired cells.
Cultures
Clonal cultures of Achnanthes were established on 29 June 2001 by micropipetting single cells into Repli dish wells of culture medium. Subsequently, monoclonal cultures were maintained in polystyrene 50-mm Petri dishes with 15 – 20 ml of culture medium in an incubator at 18°C, with a 12:12 h light:dark period and 25 – 30 μmol photons m − 2 s − 1 from cool-white fluorescent lights. Reinoculation of cells into fresh medium took place every 2 – 3 weeks. Soon after isolation, three clones began to auxosporulate, exhibiting uniparental auxosporulation. These three clones were selected for detailed observations (see below). Meanwhile, subcultures of the clones were stored in small plastic containers (with 10 ml of culture medium), with less light (a 10:14 light:dark period and c. 5 μmol photons m − 2 s − 1) and at a lower temperature (6 – 7°C). In this way, clones could be maintained without reinoculation for at least 4 – 5 months.
Identity of the diatom
The identity of the Achnanthes cannot be established with certainty without a full revision of the A. brevipes complex. Several species were described from living material by early microscopists (e.g. Kützing, Citation1844) but their descriptions and illustrations are understandably meagre and species boundaries are unclear. We therefore describe the morphology of our clones, so that they can be reclassified once the taxonomy of Achnanthes has improved.
Valves of large cells were linear-elliptical, with obtuse or slightly cuneate apices (). Valves of small cells were more elliptical and their ends were often more acute (). The stalk-producing raphid valve (on the right in ) bore a narrow axial area and a central raphe (, ). The central area was dilated to form a narrow stauros reaching the valve margin (, ). The other valve had a narrow eccentric pseudoraphe (, ), which is presumably derived from a raphe as in the related A. coarctata (Boyle et al., Citation1984). On both types of valve, the striae were coarsely punctate, uniseriate, and slightly radial, 8.5 – 11 in 10 μm (, ; ). The copulae were also coarsely punctate (, ). The poroids were circular to rectangular, 10 – 14 in 10 μm (), and were occluded by complex cribra ().
Figs 1 – 14. Live cells and frustules of Achnanthes cf. subsessilis. Figs 1, 2. Live cells representing F1 progeny formed after auxosporulation in clone C, girdle view (Fig. 1) and valve view (Fig. 2). Figs 3 – 6. Raphid valves. Figs 7 – 10. Frustules in girdle view. Figs 11 – 14. Araphid (pseudoraphe) valves. Valves and frustules are of clone B (Figs 3, 7, 11), clone A (Figs 4, 8, 12) on day 23 after isolation, or clone C (day 23: Figs 5, 9, 13; day 75: Figs 6, 10, 14). Scale bars represent 10 μm (for Figs 3 – 14, see Fig. 2).
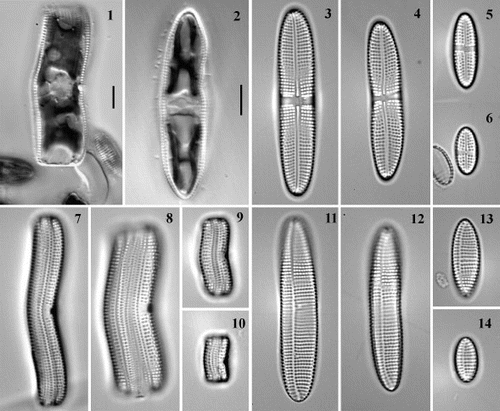
Figs 15 – 20. Scanning electron micrographs of Achnanthes cf. subsessilis, clone C. Fig. 15. Girdle view, showing porous girdle bands. Fig. 16. Raphid valve, internal view. Fig. 17. Araphid valve, internal view, with eccentric pseudoraphe. Figs 18 – 20. Cribrate areolae. Fig. 18. External surface of valve. Fig. 19. Internal surface of valve. Fig. 20. Internal surface of girdle band. Scale bars as labelled (for Figs 19 and 20, see Fig. 18).
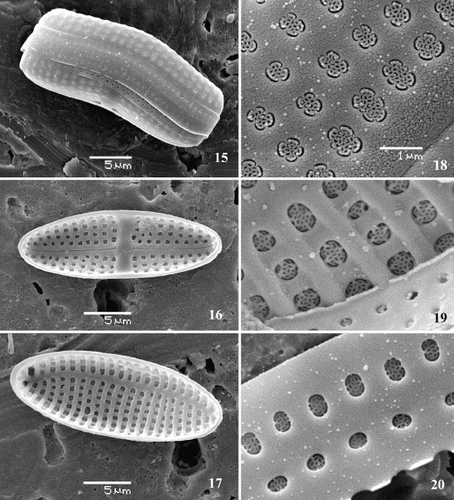
Table 1. Linear dimensions, densities (in 10 μm) of striae in valves and areola in copulae of Achnanthes cf. subsessilis from a natural population at Karadag (Crimea, Ukraine), clones A – C, and the F1 progeny of clone C.
These traits conform to descriptions of a diatom sometimes called Achnanthes subsessilis Kützing (Kützing, Citation1844; Smith, Citation1856; Hendey, Citation1951, Citation1964; Krammer & Lange-Bertalot, Citation1991). However, the taxonomic status of this diatom is unclear (Krammer & Lange-Bertalot Citation1991; Witkowski et al., Citation2000). Some authors recognize it as a separate species, but others make it a synonym of A. intermedia Kützing, which in turn has sometimes been considered a variety of the cosmopolitan, morphologically variable species, A. brevipes C. Agardh, as A. brevipes var. intermedia (Kützing) Cleve (e.g. Cleve, Citation1895; McIntire, Citation1974). This was the policy adopted by Hustedt (Citation1933) in his highly influential monograph (used by most diatomists until c. 1990). In an earlier study of the Black Sea Achnanthes we describe here, Roshchin & Chepurnov (Citation1993) also used the name A. brevipes var. intermedia. However, we consider it best not to assume synonymy between taxa that may well prove to be separate species, and so we refer to the diatom studied in this paper as Achnanthes cf. subsessilis.
Staining and microscopy
For morphological studies and taxonomic identification, frustules were cleaned by oxidation with hydrogen peroxide and glacial acetic acid (for cultures) or nitric and sulphuric acids (for the original natural sample). After repeated rinses with distilled water, frustules were mounted in Naphrax (Northern Biological Supplies Ltd., Ipswich, UK). Light microscopy (LM) was carried out using a Zeiss Axioplan 2 Universal microscope equipped with a digital camera (VIP III, Hamamatsu Photonics Deutschland GmbH, Herrsching) connected to a computer. Scanning electron microscopy (SEM) was performed using a JEOL JSM5600LV. Measurements of stria density and linear dimensions were made on LM digital images with the aid of Scion Image software (Scion Corporation, Frederick).
Staining with DAPI (4′,6-diamidino-2-phenylindole) and microspectrophotometry visualized the nucleus and determined relative DNA content. Before establishing a subculture of a clone to be used for DAPI staining, a cover slip was placed on the bottom of a Petri dish containing culture medium. Then cells were transferred into the dish and the growing culture was examined daily until a large number of auxosporulating cells, at various stages of this process, had appeared in it. The culture was then fixed with Lugol's iodine, formalin and sodium thiosulphate solutions, according to Rassoulzadegan's method (Sherr & Sherr, Citation1993). The following day, the culture was stained with DAPI (0.5 μg ml − 1) for 20 min, after which the cover slip, with cells attached to it via their mucilage stalks, was washed with phosphate-buffered saline water (to avoid excess staining: Lee et al., Citation2000) and mounted in a drop of low fluorescence (halogen-free) immersion oil (Zeiss, Jena). Relative nuclear DNA content was measured with the aid of an Axioskop-1 microscope equipped with a microscope-photometer MPM 100 (Zeiss). Error from extranuclear fluorescence was reduced by use of pinhole diaphragms and by subtracting adjacent cytoplasmic fluorescence for each nuclear value measured (Kapraun et al., Citation1988).
Voucher specimens of cleaned material of the original natural sample and of clones A, B and C [originally designated (st1)1, (st1)5 and (st1)19, respectively] are kept in the Laboratory of Protistology and Aquatic Ecology, Ghent University, Belgium.
Results
Cell structure and division
The living cells observed in natural samples and clonal cultures exhibited features characteristic of Achnanthes sensu stricto. Cells were motile or formed a mucilage stalk attached to the culture dish. The mucilage stalk was short (or just a pad, e.g. ), and was always secreted at one apex of the raphid valve (). Sometimes, the cells formed short ribbon-like filaments supported by a mucilage stalk produced by the basal cell. In clonal cultures, these filaments usually consisted of 4 – 8 cells; after auxosporulation, however, large cells tended to produce longer colonies of up to a few tens of cells. The cells contained two chloroplasts, which were composed of two plates, each appressed to one side of the girdle, linked by an isthmus containing a pyrenoid; in valve view, therefore, the chloroplasts appeared H-shaped ().
The nucleus, containing a single nucleolus, was clearly visible in interphase cells. In valve view, it lay centrally within a cytoplasmic bridge and was transversely elongate (). In girdle view, it was closer to the raphid valve (). Before mitosis, the nucleus moved to the cell centre, where it divided (). Soon after division, the nucleus of the sibling cell inheriting the raphid valve quickly returned to its interphase position, close to the raphe valve (, right cell). The nucleus of the other sibling cell, which received the araphid theca of the parental cells, did not migrate (, left cell).
Figs. 21 – 25. Chloroplast and nucleus dynamics during mitotic cycle illustrated by F1-progeny cells of clone C. Figs 21 – 23. Girdle views. Fig. 21. Two interphase cells, with the nucleus appressed to the raphid valve. Fig. 22. Mitosis. Fig. 23. Sibling cells soon after division. Figs 24, 25. Valve view. Fig. 24. Chloroplast (lower) dividing in a pervalvar direction. Fig. 25. Lower chloroplast has divided. Scale bar (for all) represents 10 μm.
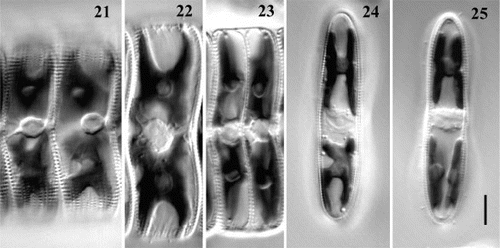
Usually, division of chloroplasts occurred during mitosis. In a few cases, however, chloroplast division was completed before nuclear division started. Normally, the chloroplast split by deepening of the apical constrictions visible in girdle view, along the median valvar plane (). In this case, the chloroplasts just divided in situ and did not undergo any translocations. In a few cases, however, we observed a constriction splitting the chloroplast in a pervalvar direction (, lower plastid), so that after division the daughter chloroplasts had to undertake a 90° translocation to achieve the proper position. The chloroplasts seemed also to be capable of dividing longitudinally by simple splitting of the pyrenoid (). All of these patterns of plastid division led in the end to the restoration of the initial H-shape of the chloroplast and chloroplast inheritance must usually or always be ‘dual’ (Mann, Citation1996), i.e. newly formed vegetative cells contain halves of both the chloroplasts of the parental cell.
Auxosporulation: observations on live cells
Clone C started to auxosporulate shortly after isolation. Twenty-eight days after isolation, its cells were 26 – 30 μm in length (mean = 27.6, SD = 1.43, n = 10) and the auxospore-forming cells fell within the same size range as the vegetative cells. At that time, clones A and B had significantly larger cells than clone C () and neither exhibited auxosporulation. Auxosporulation of clones A and B was first observed in early October, 100 days after isolation. By then, cells of clone A were 33 – 36 μm long (mean = 35.0, SD = 0.95, n = 10) and the size range of auxosporulating cells was the same as that of vegetative cells. In clone B, cells of which were 39 – 41 μm in length (mean = 40.1, SD = 0.99, n = 10), only two reproducing cells were found, each 39 μm long. The upper size threshold for auxosporulation is therefore c. 40 μm.
Once it had begun, auxosporulation became increasingly frequent as the cells became progressively reduced in size. Auxospores developed into large viable initial cells. On day 38 after isolation, measurements of initial cell length were made in clone C, giving a range of 58 – 75 μm (mean = 67.4, SD = 5.0, n = 21). In a series of subsequent re-inoculations, in which we made no attempt to separate the parental cells from the F1 generation, the proportion of large cells increased. At the beginning of September (c. 65 days after isolation), a subculture of clone C was initiated from a few of the remaining small cells but, because of intensive auxosporulation, 3 weeks later most of the cells were of the F1 generation. It was therefore impossible to maintain the original strain and the last measurement of clone C cells was performed on 26 September 2001, 90 days after isolation (); representative small cells in culture at this time are illustrated in .
All three clones exhibited an identical pattern of auxosporulation, which was consistent with the observations of Roshchin & Chepurnov (Citation1993; see also Roshchin & Chepurnov, Citation1999, ). Auxospores were produced by single unpaired cells attached to the bottom of the Petri dish by a mucilage stalk. The contents of auxosporulating cells gradually contracted and rounded off; simultaneously, the frustule dehisced, apparently through production of mucilage (), which formed an ellipsoidal sheath around the contracted cell(s).
Figs 26 – 31. Variation in pattern of auxosporulation, clone C. A single cell produces: one auxospore (Figs 26, 27), one auxospore and small aborted cell (arrows) (Figs 28, 29), or two auxospores (Figs 30, 31). Note a mucilage sheath produced by auxosporulating cells (arrowheads). Scale bars represent 10 μm.
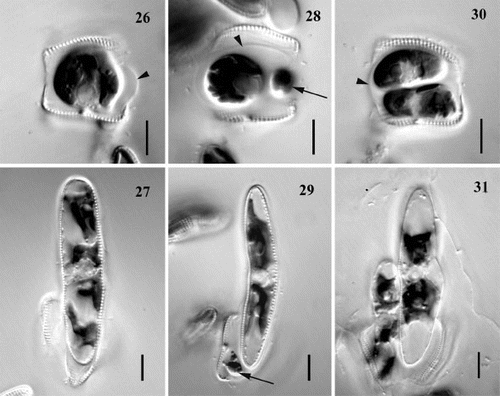
Almost always, the condensed cell contents did not divide and instead began to expand into an auxospore. A single nucleus was visible (). Expansion was bipolar and during this process it became obvious that the auxospore contained four chloroplasts (). Chloroplast division had probably occurred before expansion began, but it was not possible to observe this in the congested protoplast of the young auxospore.
In a few cases – and these are possibly significant in relation to the evolution of apomictic auxosporulation from the gametogenesis of many allogamous Achnanthes species, in which two gametes are produced per gametangium – the contents of the auxospore-forming cell divided. Sometimes, this division was very unequal (), the larger cell developing into an auxospore while the smaller one aborted (). It was impossible to determine how many chloroplasts the small cells contained, but they were never apochlorotic. In the other cases, the cytokinesis produced more-or-less equal cells (), each inheriting two chloroplasts. When the cells were approximately equal, both usually developed into auxospores (), but sometimes one aborted. The fusion of sibling protoplasts within a single auxosporulating cell was never observed.
Cells stained with DAPI
Prior to auxosporulation, there was a single mitotic division in the parent cell (). Fusion of the daughter nuclei did not occur. In the commonest case, when only one auxospore was formed by a single cell, one of the two nuclei quickly aborted in the undivided protoplast (). However, when both nuclei survived, cytokinesis occurred (), leading to the production of two auxospores (but as noted above, one of these would probably often have aborted). We also observed a few cells that had undergone unequal cytokinesis, in which the small cells did not contain a nucleus (); these cases were associated with the formation of one auxospore and a residual body within a single auxosporulating cell (as illustrated in ).
Figs 32 – 40. Auxosporulating cells of clone C stained with DAPI. Figs 32 – 37. Variation at early stages, before expansion into auxospore, associated with a single division of nucleus. Fig. 32. Before division of the nucleus. Figs 33 – 35. Division of the nucleus in an undivided protoplast. There are two possibilities: either one of the two products quickly aborts (Fig. 33) or both the nuclei survive (Fig. 34), in which case cytokinesis follows (Fig. 35). Figs 36, 37. Division of the nucleus after cytokinesis: the cell contents divide first (Fig. 36) and then karyokinesis occurs, accompanied by abortion of one of the two products (Fig. 37). Fig. 38. Developing auxospore containing a single nucleus. Figs 39 – 40. Expanded auxospores, after the first, Fig. 39, and the second, Fig. 40, acytokinetic mitotic divisions that precede formation of the epi- and hypothecae of initial cell. Scale bar (for all) represents 10 μm.
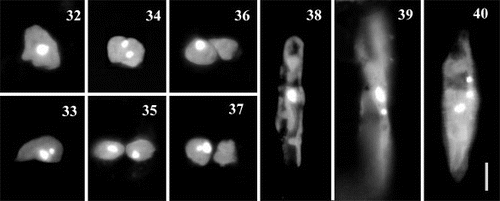
Expanding auxospores always contained a single nucleus (). In a few large auxospores, however, we observed one or two small aborted nuclei in addition to the large functional nucleus (, 40), which is consistent with previous observations that the formation of each initial valve in diatoms is always associated with an acytokinetic mitosis (Geitler, Citation1963; Drebes, Citation1977; Round et al., Citation1990).
The relative DNA content of the nuclei was determined for a single auxosporulating subculture of clone C (). For vegetative cells, we selected specimens that were likely to be at an early stage of the cell cycle, in G1, which corresponds to a 2C level in the nucleus. To do this, we looked for cells that had only just divided and were narrow in girdle view (e.g. ), or where the nucleus was still appressed to the raphid valve (). These cells exhibited a single fluorescence peak, which we assume corresponds to the diploid level (); the tail of higher DNA content indicated the beginning of DNA replication and the presence of a few 4C cells (already in G2). A similar frequency distribution, with a slight shift towards higher DNA content, was present in auxospore mother cells (). In young unexpanded auxospores with two nuclei (corresponding to the stages shown in , 33), the larger functional nuclei were 2C (), while the smaller, degenerating nuclei had a 2C level or less (). Taken together, these results indicate that meiosis is absent, no haploid nuclei are produced and karyogamy is absent: auxosporulation is entirely asexual. They also suggest that the G2 phase is very short in the auxospore mother cells.
Auxospore structure, initial cells and developmental abnormalities
During auxospore expansion, there was no sign of a transverse perizonium and siliceous wall elements were produced apparently only after expansion was complete. Then one side of the auxospore was silicified by formation of a series of porous strips comprising a longitudinal perizonium (). These comprised a robust central band, bearing a system of striae and pores subtended by a sternum, as in the pseudoraphe valve (except that the sternum appears to be closer to the midline in the auxospore band), flanked on either side by at least one more delicate porous bands with an asymmetrical pattern (). The flanking bands appeared to be connected at one end, thus making a single element resembling the ‘open’ girdle bands of the vegetative thecae in many diatoms. Altogether, the longitudinal perizonium resembles a reduced theca, with a valve and at least one girdle band. However, we did not detect a third mitosis in the expanded auxospores that could be associated with the formation of the longitudinal perizonium. In this respect, the longitudinal perizonium differed both from the initial valves (see above) and normal vegetative valves.
Figs 42 – 48. Perizonium, initial cells and abnormalities of auxosporulation in Achnanthes cf. subsessilis, clone C. Fig. 42. Longitudinal perizonium: central (arrow) and lateral (arrowhead) bands. Figs 43 – 45. Initial cell associated with the perizonium (arrow) and the frustule of parental cell: valve view (Fig. 43), girdle view (Fig. 44), and initial cell escaping from the perizonium (Fig. 45). Figs 46, 47. Initial cells of abnormal shape: sigmoid (Fig. 46) and triradiate (Fig. 47). Fig. 48. Multiple deposition of hypothecae during initial cell formation. Scale bars represent 10 μm.
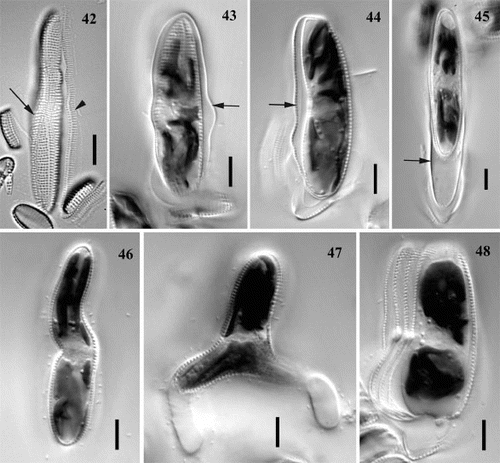
The shape of the expanded auxospore, and hence also of the longitudinal perizonium and initial valves, exhibited some variation. Often the auxospores were straight, but sometimes they were curved, bent or even sigmoid (, which shows the immediate descendant of such an auxospore), and sometimes they were expanded in the middle ().
The initial epivalve was always laid down on the side opposite the perizonium and was a pseudoraphe valve. Then the first raphid valve was formed beneath the perizonium and at some distance from it, as a result of a contraction of the protoplast (), so that the outline of the initial hypovalve did not correspond to that of the perizonium, especially at the centre. Once the formation of the initial valves was completed, the cell either escaped from the perizonium () or divided mitotically while still in association with the perizonium and the frustule of the parental cell.
Occasionally, the expansion of auxospores was not bipolar but tripolar, resulting in the formation of triradiate initial cells. One such cell in A. cf. subsessilis was seen with two mucilage stalks (). A few times, we observed that initial cells produced multiple hypothecae (). The reasons for these abnormal patterns of development (, 48) are not understood.
Discussion
Auxospore formation in A. cf. subsessilis is not accompanied by meiosis and karyogamy. This is clearly demonstrated by the following: DAPI staining gives no evidence of more than one nuclear division in the auxospore mother cell, nor of fusion of the nuclei (); and the DNA content of the nuclei present in the auxospore mother cell, the auxospore and the initial cell is approximately the same in all and indicates that all cells are diploid (assuming that the vegetative cells themselves are diploid, as opposed to haploid). In addition, during observations of many hundreds of auxospores and auxospore mother cells, we never observed fusion of protoplasts, nor saw any evidence of meiotic prophase, which is usually marked by a great expansion of the nucleus (reflecting its 4C DNA content) and often by ‘balling’ of the chromatin during zygotene (e.g. Mann & Stickle, Citation1989, Citation1995).
Sexual events in diatoms always result in auxosporulation, but sexual reproduction is not a necessary precondition for the formation of auxospores: asexual auxosporulation, although ‘the exception rather than the rule’ (Lewis, Citation1984), has been documented in a number of diatom species (e.g. Geitler, Citation1973; Drebes, Citation1977; Nagai et al., Citation1995). In every case where asexual auxosporulation has been found, however, it occurs in what are otherwise predominantly sexual diatom lineages, which suggests that asexual auxosporulation is probably a secondary modification of a basically sexual pathway of development. Hence, asexual auxosporulation is probably best referred to as ‘apomictic’ auxosporulation, by analogy with higher plants, where apomixis generally means ‘asexual reproduction through seeds’, meiosis and fertilization being bypassed. Achnanthes cf. subsessilis exhibits the same basic attributes of auxosporulation that are present in allogamous sexual Achnanthes species, except that pairing, meiosis and fertilization have been excised from the process; it is therefore ‘apomictic’. It is important to emphasize that apomictic auxosporulation is not the same as ‘vegetative enlargement’, although the end result – partial or complete restitution of size – is similar: during vegetative enlargement, the cells lack the special envelopes (properizonia, perizonia, etc) characteristic of auxospores (von Stosch, Citation1965, Citation1982).
The discovery of an apomictic population is important for the exploitation of the Achnanthes species complex as a model system for studies of breeding systems in diatoms. The next step is to determine whether this and other populations of A. cf. subsessilis are obligately apomictic, or whether they are facultatively sexual. These two possibilities would have different consequences for the genetic structure of the population (e.g. Marshall & Weir, Citation1979; Herbert, Citation1987; Berthaud, Citation2001). We saw no evidence for any pattern of reproduction except apomixis. In both natural samples and cultures, both monoclonal and mixed, auxosporulation was always uniparental and asexual. Roshchin (Citation1994 a) reported different behaviour in four clones initially identified as identical to the apomictic population we describe here and which were isolated from the same locality. These four clones did not produce auxospores and exhibited peculiar changes in cell size during a year of observations in culture, fluctuating gradually, up and down, within the range 25 – 56 μm (see Roshchin, Citation1994 a: pp. 122 – 123). We have re-examined voucher preparations of Roshchin's clones and found that they were not A. cf. subsessilis but the similar A. angustata Greville [syn. A. brevipes var. angustata (Greville) Cleve], which we have found to be obligately sexual (heterothallic) (V.A. Chepurnov, unpublished observations). Attempts to cross A. angustata with A. cf. subsessilis isolated from the same locality have revealed no signs of sexual compatibility between them and they probably represent entirely separate species.
Idei (Citation1991) reported allogamous sexual reproduction in a diatom identified as A. brevipes var. intermedia. We collected this diatom from the littoral of the North Sea, in Scotland (1996) and Belgium (2001). In culture, we observed auxosporulation similar to that reported by Idei, and all of the clones exhibited a strong tendency to reproduce intraclonally (monoecious reproduction). We repeatedly prepared mixed cultures of A. cf. subsessilis clones from Karadag, Crimea, with two of the allogamous Belgian clones. There was no sign of interaction between the clones, which continued to auxosporulate independently. Detailed comparative studies of the morphology and 18S rDNA sequences of A. cf. subsessilis and A. brevipes var. intermedia are in progress. However, it is clear that these two taxa are reproductively isolated and there is no evidence for facultative sexuality in A. cf. subsessilis.
The Karadag population of A. cf. subsessilis is apparently not unique. Lüders (Citation1862) and Karsten (Citation1897, Citation1899) both recorded uniparental sexual reproduction in Achnanthes and both identified the species as Achnanthes subsessilis. Their material was taken from the Kieler Bucht (Baltic Sea) and data and measurements (cell size range, cell shape and stria density), made mostly from their drawings, show great similarity to the diatom we studied. However, Lüders' and Karsten's explanation of uniparental auxosporulation differs from ours, because they claimed that formation of the auxospores is associated with division of the protoplast and then fusion of the two daughter cells (Karsten, Citation1899: p. 43). Quite reasonably, this behaviour has been interpreted as automixis, in the form of paedogamy (e.g. Geitler, Citation1932, Citation1936, Citation1985), but close examination of the 19th century accounts suggests that apomixis is just as likely. In Karsten's study, fusion was inferred, not observed (‘Die Verschmelzung selbst habe ich nicht gesehen’: Karsten, Citation1897: p. 49). Lüders reported fusion within an auxosporulating cell, in which the contents of the auxospore mother cell contracted away from the frustule and then divided. After a short time, the two cells were then supposed to fuse and form a spherical zygote (Lüders, Citation1862: p. 60), which Lüders noted was surrounded by a mucilage sheath, as in our specimens. Lüders' Figs 8a and 8b illustrate this idea, but they do not show fusion. Instead, they show a single contracted protoplast, lying within the confines of the dehisced mother cell frustule, as in our . Although the cell contents are polarized in Fig. 8a, with the chloroplasts at opposite ends of the protoplast, the cell is undivided. Lüders' Fig. 8c clearly illustrates a divided protoplast, as in our , containing two rounded cells surrounded by an ellipsoidal mucilage sheath. The same cell was drawn several hours later (Fig. 8d), when the two cells were still present within the frustule of the mother cell but were now visibly unequal in size. Lüders' interpretation was that one had partially absorbed the other. However, such partial fusion is unknown in diatoms and it is much more likely that, as in some of our specimens, only one of the cells became an auxospore, while the other degenerated.
Overall, therefore, we consider that Lüders and Karsten probably observed apomictic A. cf. subsessilis. As a final check, new observations of Baltic specimens would be useful. The variation in reproductive behaviour, both real (between A. brevipes var. intermedia and Crimean A. cf. subsessilis) and possibly imaginary (between the Lüders – Karsten and present interpretations of A. cf. subsessilis), within the A. brevipes complex of species, despite great similarity of frustule morphology, is further illustration of the inadequacy of the current species concept in diatoms (see also Mann, Citation1999). This deficiency in turn undermines attempts to show whether diatom species are ubiquitous (Finlay et al., Citation2002) or show significant endemism (Vyverman, Citation1996; Mann & Droop, Citation1996; Mann, Citation1999).
The development of the auxospores in Crimean A. cf. subsessilis raises interesting issues in relation to the evolution of apomixis. Meiosis and fertilization are entirely absent and yet some other aspects of auxosporulation, such as the dehiscence of the frustule, mucilage sheath formation around the auxospore, auxospore development and perizonium formation, and formation of the initial cell, are essentially identical to those in allogamous or automictic Achnanthes (e.g. Karsten, Citation1897; von Stosch, Citation1982; Idei, Citation1991; Mizuno, Citation1994; Roshchin, Citation1994 a). Thus, apomixis most likely represents a modification of the allogamous processes in other Achnanthes species. There is no doubt of the general direction of evolution – from sexual auxosporulation to apomictic auxosporulation – because of the complexity of meiosis and its plesiomorphic status within diatoms, and more generally in eukaryotes. However, there are at least two possibilities for the evolution of the uniparental auxosporulation found in A. cf. subsessilis.
Pathway A involves direct derivation from allogamy, whereas pathway B leads from allogamy to apomixis via automixis. Pathway B would involve progressive loss of sexuality, (1) by the loss of pairing and modification of the mating system to allow fusion of sister nuclei at meiosis I or II, either within an undivided protoplast (autogamy sensu Geitler, Citation1973) or after plasmogamy between sibling gametes (paedogamy sensu Geitler, Citation1973); and (2) by the subsequent loss of meiosis and karyogamy. Pathway A, on the other hand, would involve a one-step abolition of sexuality and substitution of meiosis by simple mitosis. Intuitively, pathway B seems to us more plausible and Chepurnov & Roshchin (Citation1995) observed paedogamous gamete fusion in otherwise allogamous A. longipes. However, a counter-argument is provided by the Cocconeis placentula Ehrenberg species complex, studied in detail by Geitler over many years (e.g. 1927, 1948, 1973, 1982). Here, two varieties (vars lineata (Ehrenberg) Van Heurck and klinoraphis Geitler) have been found to be capable of parthenogenesis. Var. lineata (at least at the best studied site, at Lunz, Austria) is obligately apomictic. Var. klinoraphis, on the other hand, is only facultatively apomictic: in two instances mentioned by Geitler (Citation1982), the numbers of sexual: apomictic auxospores were 21:15 and 22:121, respectively. Sexual development is allogamous and so involves pairing between gametangia. Apomictic development, on the other hand, does not generally involve pairing and the few cases of this that Geitler observed (Geitler, Citation1982) could perhaps be entirely accidental juxtapositions of cells. It appears, therefore (unless var. klinoraphis is heterogeneous and consists of two or more cryptic species), either that the change from sexual to apomictic auxosporulation is under the control of one or a few genes that are segregating within the Lunz population, or that there is a simple developmental switch in all klinoraphis cells, turning sex and all associated processes (presumably including gametangium signalling and recognition, gametangium pairing, meiosis, gamete signalling and recognition, plasmogamy and karyogamy) on or off. In either case, a major shift in development is apparently a ‘simple’ evolutionary transition and does not need a gradual origin via automixis. Interestingly, whereas apomictic cells of the facultatively apomictic var. klinoraphis pair rarely or not at all, pairing is always seen in the obligately apomictic var. lineata (Geitler, Citation1982). This pseudocopulation would appear to have no function and Geitler suggested that it is relictual, but it may also indicate that there are several different points during auxosporulation at which cells may exit from the normal sexual process and substitute apomictic development.
Testing these ideas, which might have considerable economic spin-off in relation to manipulation of development in crop plants (e.g. Sadivan et al., Citation2001), requires two complementary approaches: molecular phylogenetic studies of the relationships between allogamous, automictic and apomictic taxa, and studies of gene expression and developmental controls during sexual and apomictic development. Cocconeis placentula is unlikely to be a suitable model organism for such studies, despite the wealth of information from Geitler's studies, which included chromosome data (Geitler, Citation1973), because C. placentula grows slowly in culture, its cells are small and attach very strongly to the substratum, and the life cycle apparently cannot be shortened or lengthened by manipulation of cell size (our unpublished observations). Achnanthes is preferable, following our discovery of apomixis in A. cf. subsessilis.
The production of two auxospores by some cells in A. cf. subsessilis has special significance in the context of the ‘sex clock’ hypothesis of Lewis (Citation1984, Citation1987), in which he hypothesized that size reduction is adaptive in allowing the costs of sex to be spread over periods greater than a year. One of the principal costs Lewis identified is interruption of synthesis during meiosis and sexual reproduction (Lewis, Citation1983). Thus, in many allogamous diatoms, including allogamous Achnanthes, the whole process of pairing, meiosis, fertilization and auxospore development takes many hours or days and the numerical outcome is that two small cells give rise to at most two large cells; hence, sexual auxosporulation in pennate diatoms imposes a major short-term penalty that is not experienced by any cells that remain vegetative. In A. cf. subsessilis, however, not only is the process of auxosporulation shorter, because the two meiotic divisions and subsequent plasmogamy are replaced by a single mitotic division, but also each small cell gives rise, on average, to more than one auxospore (see also Roshchin & Chepurnov, Citation1993), thus compensating to some extent for the interruption to normal vegetative growth. A priori, one might expect that many diatoms would have adopted such an apparently advantageous auxosporulation strategy, but there may be hidden costs in the production of up to two auxospores per mother cell (besides the loss of whatever advantages sex brings: see, for example, Maynard Smith, Citation1989), such as slower development of the auxospore. Comparisons between A. cf. subsessilis and its close relatives in the rate of development of the auxospore and the division rate of post-auxospore cells will be instructive.
The longitudinal bands of the auxospore casing are similar to structures reported in A. longipes by von Stosch (Citation1982), who referred to them as a ‘longitudinal perizonium’, by analogy with the longitudinal perizonial bands that underlie the transverse perizonium in Rhabdonema, Rhoicosphenia and other pennate diatoms (von Stosch Citation1962, Citation1982; Mann, Citation1982). Mizuno (Citation1994) and Toyoda et al. (Citation2003) have observed the same kinds of bands in Achnanthes javanica f. subconstricta (Meister) Hustedt and A. yaquinensis McIntire and Reimer, respectively. In A. cf. subsessilis, the lateral band(s) are continuous around one apex, like open girdle bands, which has not been reported before.
The nature and evolutionary origin of the longitudinal perizonium needs to be determined. In Rhoicosphenia and Rhabdonema, the longitudinal perizonium consists of five bands (a wide central strip, flanked on either side by two narrower strips), none of which bear close resemblance to either the valve or the girdle bands of vegetative thecae (von Stosch, Citation1982; Mann, Citation1982); the longitudinal perizonial bands are also unlike vegetative thecae in Amphora arcus (Mann, Citation1994 a). Nevertheless, the longitudinal bands of these genera resemble a rudimentary theca in their positions and in the general property that there is a major central element, the primary longitudinal band (cf. the valve), flanked by lesser subsidiary bands (cf. girdle bands). In A. cf. subsessilis, however, the resemblance between longitudinal perizonium and theca is much greater, because the primary band has a well-developed system of pores and striae subtended by a narrow sternum, because it is flanked by a band (possibly more than one) that is apparently continuous around one pole, as in open girdle bands, and because the secondary band(s) also bear a system of pores and striae, which is oriented with respect to a longitudinal sternum-like axis near their proximal margins, again as in girdle bands. Perhaps these differences between longitudinal perizonia reflect evolutionary transformation of homologous structures. Alternatively, perhaps ‘longitudinal perizonia’ are heterogeneous, some being genuine (i.e. ontogenetically separate elements of the auxospore casing, functioning in the creation of shape: Mann, Citation1994 b) others being modified thecae. If they are modified thecae, however, then their formation should be associated with a prior mitosis or prior DNA replication, because this appears to be a universal feature of diatoms (Geitler, Citation1963; von Stosch & Kowallik, Citation1969); however, even if the pre-auxospore mitosis was the trigger for ‘longitudinal perizonium’ formation in A. cf. subsessilis, this explanation could not be used for meiotic Achnanthes.
The absence of a transverse perizonium in Achnanthes (we consider that the transverse striations in Karsten's (Citation1899) , are not transverse bands but the striae of the longitudinal bands), in contrast to their presence in many other pennate diatoms (e.g. von Stosch, Citation1962, Citation1982; Mann, Citation1982), may explain the high frequency of irregularly shaped auxospores and triradiate auxospores and initial cells in Achnanthes spp., e.g. A. longipes (Hendey, Citation1951; Schmidt in Pickett-Heaps et al., Citation1990; Chepurnov & Roshchin, Citation1995) and A. brevipes (V.A. Chepurnov & D.G. Mann, unpublished observations). Williams (Citation2001) has noted that a similar ‘riot of peculiar shapes’ is present in Fragilariforma and some other araphid pennates, and here again a transverse perizonium is absent.
Acknowledgements
Financial support for this research was provided by FKFO projects nos. G.0292.00 and G.0435.02, and BOF-project GOA 12050398 (Ghent University, Belgium). K. Sabbe is a Senior Research Fellow with the Fund for Scientific Research (FWO, Belgium). We thank our colleagues from Ghent University, Dr. Tom Beeckman (Dep. Plant System Biology) and Peter Chaerle (Dep. Biology, section Pteridology) for their help in performance of spectrophotometric measurements and R. Dasseville for his assistance in SEM studies.
References
References
- Berthaud J 2001 Apomixis and the management of genetic diversity In The Flowering of Apomixis: From Mechanisms to Genetic Engineering (Sadivan, Y., Carman, J.G. & Dresselhaus, T., editors) 9 23 Mexico, D.F.: CIMMYT, IRD, European Commission DG VI (FAIR)
- Boyle , JA , Pickett-Heaps , JD and Czarnecki , DB . 1984 . Valve morphogenesis in the pennate diatom Achnanthes coarctata . J. Phycol , 20 : 563 – 573 .
- Chepurnov VA 1989 Massovye vidy bentosnykh diatomovykh vodoroslej chernomorskogo kamenistogo melkovod'ya rajona Karadaga Deponirovana v VINITI [deposited paper, VINITI library] 13.01.89, No. 328-B89, Kiev 16 pp
- Chepurnov , VA and Mann , DG . 1997 . Variation in the sexual behaviour of natural clones of Achnanthes longipes . Eur. J. Phycol , 32 : 147 – 154 .
- Chepurnov , VA and Mann , DG . 1999 . Variation in the sexual behaviour of Achnanthes longipes (Bacillariophyta). II. Inbred monoecious lineages . Eur. J. Phycol , 34 : 1 – 11 .
- Chepurnov , VA and Mann , DG . 2000 . Variation in the sexual behaviour of Achnanthes longipes (Bacillariophyta). III. Progeny of crosses between monoecious and unisexual clones . Eur. J. Phycol , 35 : 213 – 223 .
- Chepurnov , VA and Roshchin , AM . 1995 . Inbreeding influence on sexual reproduction of Achnanthes longipes Ag. (Bacillariophyta) . Diatom Res , 10 : 21 – 29 .
- Cleve PT 1895 Synopsis of the naviculoid diatoms. Part II Kongliga Svenska Vetenskaps-Akademiens Handlingar N.S 27 1 219
- Crawford RM 1981 Some considerations of size reduction in diatom cell walls In Proceedings of 6th International Symposium on Living and Fossil Diatoms (Ross, R., editor) 253 265 Koeltz, Koenigstein
- Davidovich , NA . 1994 . Factors controlling the size of initial cells in diatoms . Russian Journal of Plant Physiology , 41 : 220 – 224 .
- Drebes G 1977 Sexuality In The Biology of Diatoms (Werner, D., editor), Botanical Monographs 13 250 283 Blackwell, Oxford
- Edlund , MB and Stoermer , EF . 1997 . Ecological, evolutionary, and systematic significance of diatom life histories . J. Phycol , 33 : 897 – 918 .
- Field , CB , Behrenfeld , MJ , Randerson , JT and Falkowski , PG . 1998 . Primary production of the biosphere: integrating terrestrial and oceanic components . Science , 281 : 237 – 240 .
- Finlay , BJ , Monaghan , EB and Maberly , SC . 2002 . Hypothesis: the rate and scale of dispersal of freshwater diatom species is a function of their global abundance . Protist , 153 : 261 – 273 .
- Geitler , L . 1927 . Somatische Teilung, Reduktionsteilung, Copulation und Parthenogenese bei Cocconeis placentula . Arch. Protistenk , 59 : 506 – 549 .
- Geitler , L . 1932 . Der Formwechsel der pennaten Diatomeen (Kieselalgen) . Arch. Protistenk , 78 : 1 – 226 .
- Geitler , L . 1936 . Sexuelle Fortpflanzung der Diatomeen (Auxosporenbildung) . Tabulae Biologicae , 6 : 284 – 290 .
- Geitler , L . 1948 . Zur Kenntnis der Rassenbildung und des Kopulationsverhaltens der Diatomee Cocconeis placentula und ihres Epiphytismus . Bot. Notiser , 1948 : 84 – 92 .
- Geitler , L . 1957 . Die Sexuelle Fortpflanzung der pennaten Diatomeen . Biol. Rev , 32 : 261 – 295 .
- Geitler , L . 1963 . Alle Schalenbildungen der Diatomeen treten als Folge von Zell- oder Kernteilungen auf . Ber. Dtsch. Bot. Ges , 75 : 393 – 396 .
- Geitler , L . 1973 . Auxosporenbildung und Systematik bei pennaten Diatomeen und die Cytologie von Cocconeis-Sippen . Öst. Bot. Z , 122 : 299 – 321 .
- Geitler L 1982 Die infraspezifischen Sippen von Cocconeis placentula des Lunzer Seebachs Arch. Hydrobiol 63(Suppl.) 1 (Algological Studies 30) 1 11
- Geitler , L . 1985 . Automixis bei pennaten Diatomeen . Plant Syst. Evol , 150 : 303 – 306 .
- Hendey , NI . 1951 . Littoral diatoms of Chichester harbour with special reference to fouling . J. Royal Microsc. Soc , 71 : 1 – 86 .
- Hendey NI 1964 An Introductory Account of the Smaller Algae of British Coastal Waters Part V. Bacillariophyceae (Diatoms) London, Her Majesty's Stationery Office
- Herbert PDN 1987 Genotypic characteristics of cyclic parthenogens and their obligately asexual derivatives In The Evolution of Sex and its Consequences (Stearns, S.C., editor) 175 196 Birkhauser Verlag, Basel, Switzerland
- Holsinger , KE . 2000 . Reproductive systems and evolution in vascular plants . Proc. Natl. Acad. Sci. USA , 97 : 7037 – 7042 .
- Hustedt F 1933 Die Kieselalgen Deutschlands, Österreichs und der Schweiz unter Berücksichtigung der übrigen Länder Europas sowie der angrenzenden Meeresgebiete In Dr. L. Rabenhorst's Kryptogamen-Flora von Deutschland, Österreich und der Schweiz 7 2:3 321 432 Akademische Verlagsgesellschaft, m.b.h. Leipzig
- Idei M 1991 Achnanthes brevipes C. Agardh var. intermedia (Kützing) Cleve In An illustrated atlas of the life history of algae. Volume 3. Unicellular and flagellated algae (Hori, T., editor) 261 Uchida Rokakuho Publishing Co., Ltd., Tokyo
- Kapraun , DF , Gargiulo , MG and Tripodi , G . 1988 . Nuclear DNA and karyotype variation in species of Codium (Codiales, Chlorophyta) from the North Atlantic . Phycologia , 27 : 273 – 282 .
- Karsten , G . 1897 . Untersuchungen über Diatomeen. II . Flora , 83 : 33 – 53 .
- Karsten G 1899 Die Diatomeen der Kieler Bucht Wiss. Meeresunters Kiel 4 19 205
- Krammer K Lange-Bertalot H 1991 Bacillariophyceae 4. Teil: Achnanthaceae; Kritische Engänzungen zu Navicula (Lineolatae) und Gomphonema In Süsswasserflora von Mitteleuropa (Ettl, H., Gärtner, G., Gerloff, J., Heynig, H. & Mollenhauer, D., editors) vol. 2/4 1 437 G. Fischer Verlag, Stuttgart & Jena
- Kützing FT 1844 Die kieselschaligen Bacillarien oder Diatomeen W. Köhne Nordhausen
- Lee , S-H , Motomura , T and Ichimura , T . 2000 . Nuclear phase alternation in the life cycle of Derbesia (Chlorophyta) . Phycologia , 39 : 441 – 447 .
- Lewis , RJ , Johnson , LM and Hoagland , KD . 2002 . Effects of cell density, temperature, and light intensity on growth and stalk production in the biofouling diatom Achnanthes longipes (Bacillariophyceae) . J. Phycol , 38 : 1125 – 1131 .
- Lewis, Jr , WM . 1983 . Interruption of synthesis as a cost of sex in small organisms . Am. Nat , 121 : 825 – 832 .
- Lewis, Jr , WM . 1984 . The diatom sex clock and its evolutionary significance . Am. Nat , 123 : 73 – 80 .
- Lewis, Jr WM 1987 The cost of sex In The Evolution of Sex and its Consequences (Stearns, S.C., editor) 33 57 Birkhauser Verlag, Basel, Switzerland
- Lüders , JE . 1862 . Beobachtungen über die Organisation, Theilung und Copulation der Diatomeen . Bot. Zeit , 20 : 41 – 42, 49 – 52, 57 – 61, 65 – 69
- Mann , DG . 1982 . Structure, life history and systematics of Rhoicosphenia (Bacillariophyta) II. Auxospore formation and perizonium structure of Rh. curvata . J. Phycol , 18 : 264 – 274 .
- Mann , DG . 1993 . Patterns of sexual reproduction in diatoms . Hydrobiologia , 269/270 : 11 – 20 .
- Mann , DG . 1994a . The systematics of amphoroid diatoms: the life history of . Amphora arcus. Nova Hedwigia , 58 : 335 – 352 .
- Mann DG 1994b The origins of shape and form in diatoms: the interplay between morphogenetic studies and systematics In Shape and form in plants and fungi (Ingram, D.S. & Hudson, A.J., editors) 17 38 Academic Press, London
- Mann DG 1996 Chloroplast morphology, movements and inheritance in diatoms In Cytology, genetics and molecular biology of algae (Chaudhary, B.R. & Agrawal, S.B., editors) 249 274 SPB Academic Publishing, Amsterdam
- Mann , DG . 1999 . The species concept in diatoms (Phycological Reviews 18) . Phycologia , 38 : 437 – 495 .
- Mann , DG and Droop , SJM . 1996 . Biodiversity, biogeography and conservation of diatoms . Hydrobiologia , 336 : 19 – 32 .
- Mann , DG and Stickle , AJ . 1989 . Meiosis, nuclear cyclosis, and auxospore formation in Navicula sensu stricto (Bacillariophyta) . Br. Phycol. J , 24 : 167 – 181 .
- Mann , DG and Stickle , AJ . 1995 . Sexual reproduction and systematics of Placoneis (Bacillariophyta) . Phycologia , 34 : 74 – 86 .
- Mann , DG , Chepurnov , VA and Droop , SJM . 1999 . Sexuality, incompatibility, size variation, and preferential polyandry in natural populations and clones of Sellaphora pupula (Bacillariophyceae) . J. Phycol , 35 : 152 – 170 .
- Mann , DG , Chepurnov , VA and Idei , M . 2003 . Mating system, sexual reproduction, and auxosporulation in the anomalous raphid diatom Eunotia (Bacillariophyta) . J. Phycol , 39 : 1067 – 1084 .
- Marshall , DR and Weir , BS . 1979 . Maintenance of genetic variation in apomictic plant populations. I. Single locus models . Heredity , 42 : 159 – 172 .
- Maynard Smith J 1989 Evolutionary genetics Oxford University Press, Oxford
- McIntire , CD . 1974 . Some marine and brackish-water Achnanthes from Yaquina Estuary, Oregon (USA) . Bot. Mar , 17 : 164 – 175 .
- McIntire CD Moore WW 1977 Marine littoral diatoms: ecological considerations In The Biology of Diatoms (Werner, D., editor), Botanical Monographs 13 333 371 Blackwell Scientific, Oxford
- Mizuno , M . 1994 . Sexual reproduction and auxospore formation in Achnanthes javanica f. subconstricta . Diatom Res , 9 : 133 – 141 .
- Nagai , S , Hori , Y , Manabe , T and Imai , I . 1995 . Restoration of cell size by vegetative cell enlargement in Coscinodiscus wailesii (Bacillariophyceae) . Phycologia , 34 : 533 – 535 .
- Pickett-Heaps , JD , Schmid , A-MM and Edgar , LA . 1990 . The cell biology of diatom valve formation . Progress in Phycological Research , 7 : 1 – 168 .
- Richards AJ 1997 Plant breeding systems 2nd edition, Chapman & Hall, London
- Roshchin , AM . 1982 . Skorost’ razmnozheniya i umen'sheniya razmerov kletok nekotorykh vidov bentosnykh diatomovykh vodoroslej . Biol. Nauki (Mosk.) , 1982 ( 9 ) : 71 – 75 .
- Roshchin , AM . 1984a . Nekotorye osobennosti rosta i vsplyvanie kletok v kul'turakh bentosnykh diatomovykh vodoroslej . Biol. Nauki (Mosk.) , 1984 ( 6 ) : 49 – 56 .
- Roshchin , AM . 1984b . Zhiznennye tsikly bentosnoj diatomovoj vodorosli Achnanthes longipes Ag . Biol. Nauki (Mosk.) , 1984 ( 11 ) : 71 – 78 .
- Roshchin AM 1994a Zhiznennye tsikly diatomovykh vodoroslej Naukova Dumka, Kiev
- Roshchin , AM . 1994b . Dvudomnoe vospoizvedenie Achnanthes longipes Ag. (Bacillariophyta) . Algologia , 4 ( 1 ) : 22 – 29 .
- Roshchin , AM and Chepurnov , VA . 1992 . Vegetativnoe ukrupnenie kletok v zhiznennykh tsiklakh Achnanthes longipes Ag. (Bacillariophyta) . Algologia , 2 ( 3 ) : 26 – 32 .
- Roshchin , AM and Chepurnov , VA . 1993 . Obrazovanie auksospor v klonovoj kul'ture Achnanthes brevipes Ag. var. intermedia (Kütz.) Cl. (Bacillariophyta) . Algologia , 3 ( 1 ) : 19 – 22 .
- Roshchin AM Chepurnov VA 1999 Dioecy and monoecy in the pennate diatoms (with reference to the centric taxa) In Proceedings of the 14th International Diatom Symposium (Mayama, S., Idei, M. & Koizumi, I., editors) 241 261 Koeltz Scientific Books, Koenigstein
- Round , FE . 1972 . The problem of reduction of cell size during diatom cell division . Nova Hedwigia , 23 : 291 – 303 .
- Round FE Crawford RM Mann DG 1990 The Diatoms. Biology and morphology of the genera Cambridge University Press, Cambridge
- Round , FE and Basson , PW . 1997 . A new monoraphid diatom genus (Pogoneis) from Bahrain and the transfer of previously described species A . hungarica and A. taeniata to new genera. Diatom Res , 12 : 71 – 81 .
- Round , FE and Bukhtiyarova , L . 1996 . Four new genera based on Achnanthes (Achnanthidium) together with a re-definition of Achnanthidium . Diatom Res , 11 : 345 – 361 .
- Sadivan Y Carman JG Dresselhaus T(editors) 2001 The flowering of apomixis: from mechanisms to genetic engineering Mexico, D.F.: CIMMYT, IRD, European Commission DG VI (FAIR)
- Sherr EB Sherr BF 1993 Preservation and storage of samples for enumeration of heterotrophic protists In Handbook of methods in aquatic microbial ecology (Kemp, P.F., Sherr, B.F., Sherr, E.B. & Cole, J.J., editors) 207 212 Lewis Publishers, Boca Raton
- Smith W 1856 A synopsis of the British Diatomaceae; with remarks on their structure, functions and distribution; and instructions for collection and preserving specimens London: J. van Voorst, Paternoster Row
- von Stosch , HA . 1942 . Form and Formwechsel der Diatomee Achnanthes longipes in Abhängigkeit von der Ernährung. Mit besonderer Berücksichtigung der Spurenstoffe . Ber. Dtsch. Bot. Ger , 60 : 2 – 16 .
- von Stosch HA 1962 Über das Perizonium der Diatomeen Vortr. Gesamtg. Bot. Dtsch. Bot. Ges neue Folge 1962 43 52
- von Stosch , HA . 1965 . Manipulierung der Zellgrösse von Diatomeen im Experiment . Phycologia , 5 : 21 – 44 .
- von Stosch , HA . 1982 . On auxospore envelops in diatoms . Bacillaria , 5 : 127 – 156 .
- von Stosch , HA and Kowallik , KV . 1969 . Der von L. Geitler aufgestellte Satz über die Notwendigkeit einer Mitose für jede Schalenbildung von Diatomeen. Beobachtungen über die Reichweite und Überlegungen zu seiner zellmechanischen Bedeutung . Öst. Bot. Z , 116 : 454 – 474 .
- Toyoda K Tanaka J Nagumo T 2003 Morphological features of vegetative and initial cells of Achnanthes yaquinensis McIntire & Reimer (Bacillariophyceae) In Third European Phycological Congress (Queen's University Belfast, 21 – 26 July 2003). Programme & Book of abstracts 94
- Vyverman , W . 1996 . The Indo-Malaysian North-Australian phycogeographical region revised . Hydrobiologia , 336 : 107 – 120 .
- Williams DM 2001 Comments on the structure of ‘post-auxospore’ valves of Fragilariforma virescens In Lange-Bertalot-Festschrift. Studies on diatoms, dedicated to Prof. Dr. Dr. h.c. Horst Lange-Bertalot on the occasion of his 65th birthday (Jahn, R., J.P. Kociolek, J.P., Witkowski, A. & Compère, P., editors) 103 117 A.R.G. Gantner, Ruggell, Liechtenstein
- Witkowski A Lange-Bertalot H Metzeltin D 2000 Diatom flora of marine coasts I A.R.G. Gantner, Ruggell, Liechtenstein