Abstract
Protoperidinium vorax sp. nov. is a small heterotrophic dinoflagellate with a plate formula of Po, X, 4′, 3a, 7′′, 3C, 5S, 5′′′, 0p, 2′′′′. P. vorax has an ortho-hexa thecal plate arrangement. Its peculiar features are the presence of only three cingular plates and the marked extension of the second intercalary plate (2a), which abuts the second precingular plate (2′′). TEM sections revealed the presence of a large sac pusule, several convoluted vesicular structures and electron-dense accumulation bodies scattered in the cell periphery. These accumulation bodies probably represent the Periodic Acid-Schiff (PAS) bodies, which fluoresce yellow–green under blue light excitation. P. vorax is a pallium feeder and was observed feeding on diatoms of different sizes. When fed with the chain-forming diatom Skeletonema pseudocostatum under non-limiting food concentrations, doubling rates up to 1.28 divisions d−1 were recorded. Ingestion rates of 35.95 cells dinoflagellate−1 h−1 and clearance rates of 18.34 µl dinoflagellate−1 h−1 were reached, the highest ever recorded for a small-sized dinoflagellate. These data support the hypothesis that small heterotrophic species might contribute to diatom bloom decay.
Introduction
The dinoflagellates are an extraordinarily diversified group of protists in terms of size, shape, life strategies and modes of nutrition. In addition to strictly autotrophic species, mixotrophic, kleptoplastidic, symbiotic, parasitic and heterotrophic taxa are included in this lineage. It is now estimated that at least half of the 2000 extant dinoflagellate species are either heterotrophic or mixotrophic (Stoecker, Citation1999), and their abundance, grazing pressure and role in structuring marine food webs has only recently been acknowledged (Lessard, Citation1991; Hansen, Citation1991, Citation1992; Verity et al., Citation1993 a; Jeong, Citation1999; Kjaert et al., Citation2000; Levinsen & Nielsen, Citation2002).
The abundance of heterotrophic dinoflagellates in the natural environment is regulated by food availability, and their seasonal biomass patterns reflect those of autotrophs (Levinsen & Nielsen, Citation2002). Heterotrophic dinoflagellates quickly increase their numbers following diatom and, in some cases, phototrophic dinoflagellate blooms; they can thus play a significant role in bloom decay (Hansen, Citation1991; Verity et al., Citation1993 b; Jeong, Citation1999; Levinsen et al., Citation1999; Levinsen & Nielsen, Citation2002; Olseng et al., Citation2002). The abundance of heterotrophic dinoflagellates in marine coastal environments may be equivalent to or greater than that of ciliates (Lessard, Citation1991; Levinsen et al., Citation1999; Levinsen & Nielsen, Citation2002), but the two groups have distinct food niches. Ciliates generally feed on prey <20 µm in size (Hansen, Citation1992; Jacobsen & Hansen, Citation1997), while heterotrophic dinoflagellates are able to feed on larger cells (Naustvoll, Citation1998; Kjaert et al., Citation2000), overlapping with the size of food items grazed by herbivorous copepods (Berggreen et al., Citation1988; Kiorboe & Nielsen, Citation1994).
Heterotrophic dinoflagellates can predate upon a wide range of prey, such as phytoplankton cells, ciliates, injured metazoa, copepod eggs and naupliar stages (Jacobson, Citation1999; Jeong, Citation1999). Three kinds of feeding mechanisms have been described: (i) direct engulfment of the whole prey (widespread among non-thecate species); (ii) peduncle feeding (reported for both thecate and naked species); (iii) pallium feeding (typical of thecate dinoflagellates) (Jacobson & Anderson, Citation1986).
The genus Protoperidinium includes more than 250 thecate heterotrophic species of different sizes and morphology. Some species have characteristic shapes, with a pointed epitheca and more or less developed antapical horns or spines, while others have a roughly pentagonal or round outline. Protoperidinium species share a plate pattern of Po, 4′, 2-3a, 7′′, 4C, 6S, 5′′′, 2′′′′ and are separated on differences in gross cell morphology and number and shape of particular plates. Balech (Citation1974) subdivided the genus into three subgenera, characterized by different numbers of precingular and intercalary plates: Minusculum Lebour (6 precingular, 3 intercalary plates), Archaeperidinium Jörgensen (7 precingular, 2 intercalary plates) and Protoperidinium Gran sensu stricto (7 precingular, 3 intercalary plates). Species belonging to the subgenus Protoperidinium are further grouped on the shape of the first apical (1′) and second intercalary (2a) plates.
The identification of Protoperidinium species can be difficult, especially in the case of small species lacking distinctive morphological features. Even the recognition of small dinoflagellates as heterotrophic is only possible when samples are examined with epifluorescence microscopy. Information on taxonomic diversity, life cycles and feeding preferences of this group of dinoflagellates is notably limited. We describe a new species of Protoperidinium, P. vorax sp. nov., a small heterotrophic dinoflagellate, whose plate pattern does not correspond to any previously described species within the genus. We also provide information on ultrastructure features and feeding behaviour of this species.
Materials and methods
Cultures
Surface sediment samples were collected with a gravity corer offshore in the Gulf of Naples (40°18′ N, 15°48′ E) at a depth of 75 m on 16 May 2002. The first two centimetres of the core sample were placed in plastic tubes wrapped with aluminium foil and stored in the dark at 5–6°C. A small amount of sediment was gently sonicated for 45 s and filtered on 130, 36 and 20 µm-mesh metallic sieves. The 20 and 36 µm fractions were used for germination experiments. Sediments were placed in tissue culture plates filled with 6 ml of K medium without silicate (Keller et al., Citation1987), diluted to 1/10 strength with sterile oligotrophic seawater and adjusted to a salinity of 32 psu by the addition of sterile, double-distilled water. Plates were incubated at 20°C, at about 100 µmol photons m−2 s−1, under 12 h:12 h light:dark (L:D) cycle and checked daily under an inverted microscope for the presence of motile stages. Protoperidium-like cells were isolated with a sterile micropipette and placed into single wells of tissue culture plates with K/10 medium. They were fed with Skeletonema pseudocostatum Medlin and incubated under the above conditions. Protoperidium-like cultures were inspected every 2–3 days and, when necessary, transferred into new Petri dishes. Cultures were diluted with K/10 medium and a small quantity of food was added.
Observations on the feeding behaviour of P. vorax were carried out in cultures fed with S. pseudocostatum, as well as in cultures fed with the natural phytoplankton assemblage present in the culture plates in which germination experiments were carried out.
Three different clonal cultures identified as P. vorax sp. nov. were obtained. To stimulate the production of resting cysts, starvation was induced in each of the three cultures and in an additional culture, formed by mixing the three. Diatom prey (S. pseudocostatum) was not added to the Protoperidinium cultures for over 2 weeks. Observations were carried out regularly under the inverted microscope. The mixed culture was also maintained with normal food concentrations (see above) and checked for cyst production for two months.
Light and electron microscopy
Live cells were observed under bright field, phase contrast and Nomarski differential interference contrast, using a Zeiss Axiophot light microscope equipped with an ultraviolet mercury lamp for epifluorescence illumination and a Zeiss AxioCam digital system for photographic documentation (Carl Zeiss, Oberkochen, Germany). Subsamples were fixed with formaldehyde (1.6 % final concentration). To identify the plate pattern, cells were stained with calcofluor white (Fritz & Triemer, Citation1985) and observed under the same microscope using the Zeiss epifluorescence filter set 487901. Plate designation follows that proposed by Balech (Citation1974).
For scanning electron microscopy (SEM), fixed samples were placed on a Nucleopore (Nucleopore, Pleasanton, CA) polycarbonate filter, dehydrated in an ethanol series (25%, 50%, 75%, 95%, 100%) and critical point dried. The filter was mounted on a stub, sputter coated with gold and examined with a Philips 505 scanning electron microscope (Philips, Eindhoven, The Netherlands). For transmission electron microscopy (TEM) cultures of starved Protoperidinium were pelleted by centrifugation and fixed with 1% glutaraldehyde (final concentration) on ice for 1 h. The pellet was centrifuged, rinsed with filtered seawater (FSW) and post-fixed with 1% osmium tetroxide for 1 h. After two rinses with FSW, the sample was dehydrated in an ethanol series, transferred to propylene oxide and embedded in Epon. After polymerization at 70°C for 24 h, sections were cut on a Reichert Ultracut ultramicrotome (Depew, NY, USA), stained with uranyl acetate and lead citrate, and examined with a Philips EM 400 microscope (Philips, Eindhoven, The Netherlands).
Feeding experiments
Experiments were carried out using three replicate Petri dishes containing 30 ml K/10 culture medium, P. vorax (∼100 cells · ml−1) and S. pseudocostatum (∼3000 cells ml−1). The P. vorax culture used for the experiments was not starved but contained an average food concentration of about 1200 cells ml−1. Additional S. pseudocostatum cells, in exponential growth, were added to obtain the required concentration. The average cellular volume of P. vorax was 20.34 µm3 and that of S. pseudocostatum was 6.59 µm3, providing a predator/prey ratio of about 1:10 in terms of cell biovolume in the culture. Two control Petri dishes were inoculated with S. pseudocostatum only, at the same concentration as the feeding experiments, and exposed at the same temperature, irradiance and day length. The concentration of P. vorax and S. pseudocostatum cells in the experimental and control dishes was estimated at the beginning of the experiment (t 0), after ∼20 h (t 1), and at the end of the experiment, after ∼28 h (t 2). 2.5 ml of culture was withdrawn, fixed with formaldehyde (1.6% final concentration) and cell concentration was estimated using a 1-ml Sedgwick–Rafter counting chamber. Only intact diatom cells were counted due to difficulties in seeing empty frustules. The specific growth rates (µ) for P. vorax were calculated using the formula: μ = ln (N 2/N 0)·t −1, where N 2 and N 0 are the number of cells (cells ml−1) at the end (t 2) and at the beginning (t 0) of the experiment, respectively, and t is the incubation time (h). Ingestion and clearance rates were calculated using the equation by Frost (Citation1972), modified by Heinbokel (Citation1978) to account for the increased number of predators (P. vorax) during the incubation period.
Results
Species description
Protoperidinium vorax Siano & Montresor sp. nov.
(Class: Dinophyceae Pascher, Order: Peridiniales Haeckel).
DIAGNOSIS:
Cellulae 16–26 longae et 16–25 µm latae forma quasi globosa, sine spinis. Epitheca tholi forma, hypotheca rotunda est. Cingulum excavatum est et in cellulae equatoriali parte situm. Cellulae chloroplasti non sunt et varia corpora globosa insunt quae luce caerulea collustrata viridia apparent. Formula laminarum: Po, X, 4′, 3a, 7′′, 3C, 5S, 5′′′, 0p, 2′′′′. Prima apicalis lamina (1′) ortho; tres intercalares laminae ad epithecae sinistram partem positae sunt. Prima intercalaris lamina (1a) quinque latera habet, secunda (2a) sex latera et admodum non aequalis, tertia (3a) sex latera habet et aequalis est. Secunda intercalaris lamina (2a) cum secunda praecingularis lamina cuniuncta est (2′′). Tres cingulares laminae varia longitudine sunt. Sulci dextera lamina parvam alam in suo latere sinistro habet. Laminae politae sunt, cum poris dispersis iuxta margines.
Cells are round, without spines, 16–26 µm long and 16–25 µm wide. Epitheca is dome-shaped, hypotheca rounded. The cingulum is excavated and located in the equatorial part of the cell. The cell lacks chloroplasts but several globular bodies are present in the cytoplasm. The globules appear yellow–green in colour when observed with epifluorescence microscopy under blue light. The plate formula is: Po, X, 4′, 3a, 7′′, 3C, 5S, 5′′′, 0p, 2′′′′. The first apical plate (1′) is ortho; the positions of the 3 intercalary plates are shifted to the left-hand side of the epitheca. The first intercalary plate (1a) is pentagonal, the second (2a) is hexagonal and markedly asymmetrical, the third (3a) is hexagonal and symmetrical. Plate 2a touches the second precingular plate (2′′). The cingulum consists of three plates of different lengths. The right sulcal plate (S.d.) bears a small wing on its left side. The thecal plates are smooth with pores near their borders.
ETYMOLOGY: vorax (=voracious) refers to the high grazing rates detected for the species.
HOLOTYPE:
ICONOTYPE: –
ISOTYPES: –
TYPE LOCALITY: Gulf of Naples, Mediterranean Sea (40°18′ N, 15°48′ E)
The species was isolated by Raffaele Siano. A sample of the cultured strain (PV 19), fixed with formaldehyde, is stored at the Museum of the Stazione Zoologica ‘A. Dohrn’ in Naples (Italy) as n° PV 19.
Figs 1–4. Light micrographs of Protoperidinium vorax sp. nov. . Cell in bright field, sac pusule arrowed. . Same specimen as in with Nomarski interference contrast, sac pusule arrowed. . Broken cell, note the globular bodies randomly distributed in the cytoplasm. . Same specimen as with epifluorescence microscopy under blue light. The globular bodies appear yellow–green. Scale bars represent 10 µm.
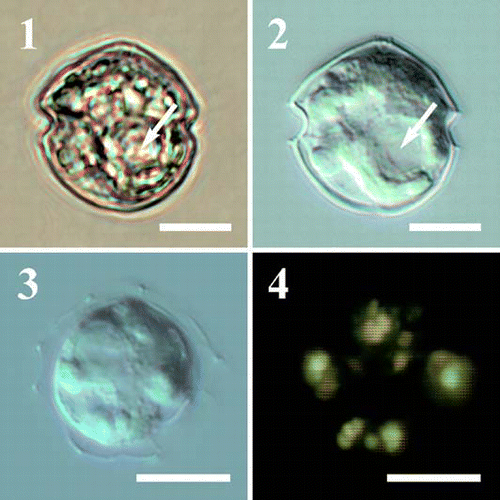
Figs 5–8. Schematic drawings of the thecal plate pattern of Protoperidinium vorax sp. nov. . Ventral view. . Dorsal view. . Epitheca, apical view. . Hypotheca, antapical view. Abbreviations: Po: pore plate; X: X platelet; 1′ to 4′: apical plates; 1′′ to 7′′: precingular plates; 1a to 3a: intercalary plates; 1C to 3C: cingular plates; S.a.: sulcal anterior plate; S.d.: sulcal right plate; S.m.: sulcal median plate; S.s.: sulcal left plate; S.p.: sulcal posterior plate; 1′′′ to 5′′′: postcingular plates; 1′′′′ and 2′′′′: antapical plates.
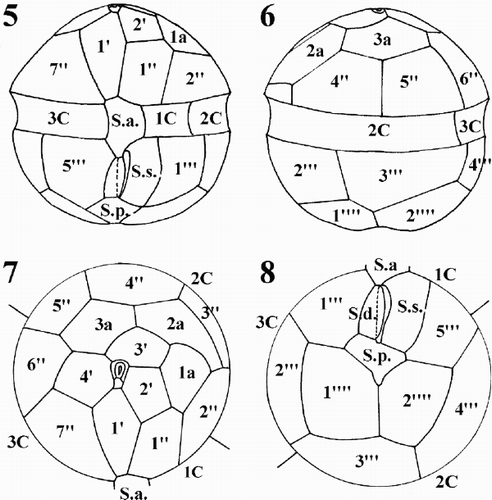
Figs 9–15. Scanning electron micrographs of Protoperidinium vorax sp. nov. . Ventral view. . Dorsal view. . Epitheca, apical view. . Hypotheca, antapical view. . Apical pore complex. . Sulcal area. . Sulcal posterior plate (S.p.), detail of . Abbreviations: see –. Scale bars: – : µm; , : 2 µm; : 1 µm.
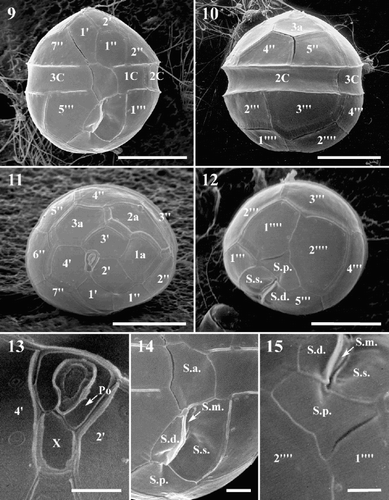
Protoperidinium vorax is almost spherical in shape, – µm high (mean = 20.96 µm, n = 50) by 16–25 µm wide (mean = 20 µm, n = 50). The epitheca is dome-shaped, the hypotheca is rounded and, at times, a faint depression is visible corresponding to the antapical end of the sulcus (, ). The cingulum is excavated and located in the equatorial portion of the cell. When observed with light microscopy (LM), a vesicle is visible in the equatorial part of the cell (, ). Several small globular bodies are randomly distributed in the cytoplasm ( –). When observed in epifluorescence microscopy under blue light, the globular bodies appear yellow–green (). The plate formula of P. vorax is: Po, X, 4′, 3a, 7′′, 3C, 5S, 5′′′, 0p, 2′′′′ ( –). The thecal plates appear smooth in LM. With SEM, relatively large intercalary bands are visible beside the epithecal and hypothecal plates, as well as large pores near the borders of the plates ( –). No variability in the plate pattern and/or shape and size of the thecal plates was observed within the culture.
The apical pore complex lies at the centre of the epitheca, pointing slightly to the right-hand side of the epitheca (). It comprises an irregularly quadrangular pore plate (Po) and a small, rectangular X platelet. The pore is located in the middle of the Po plate and is surrounded by a marked rim (). The first apical plate (1′) has 4 major sides (ortho-plate) and the shape of a truncated, asymmetrical lozenge (, ). Plate 1′ is in contact with the X platelet and the sulcal anterior (S.a.) plate. The apical margins are shorter than the antapical ones; the anterior right margin is shorter than the left one and the posterior left margin is shorter than the right one. Plate 2′ is longer than wide and pentagonal. Plates 3′ and 4′ are hexagonal, with plate 3′ being displaced toward the left-hand side of the epitheca (, ). The three intercalary plates differ markedly in size and shape and their position is asymmetrical, to the left-hand side of the epitheca. Plate 1a is pentagonal with a convex margin bordering plate 2a. Plate 2a is hexagonal, wider than high and markedly asymmetrical. It extends to the left-hand portion of the epitheca below plate 1a, abutting plate 2′′. Plate 3a is hexagonal and symmetrical (, ). The first precingular plate (1′′) is hexagonal and has the shape of an irregular polygon with almost parallel left and right sides (, ). Plate 2′′ is pentagonal and has the upper right side much shorter than the others. Plate 3′′ is quadrangular, very narrow and lies below the long 2a plate (, , , ).
The cingulum consists of three plates. The first cingular plate (1C) is the smallest of the three and its suture with plate 2C almost corresponds with the left-hand margin of the first post-cingular plate. Plate 1C is in contact with sulcal left (S.s.) plate (, , ). Plate 2C is the widest cingular plate and the suture with plate 3C is located slightly before the suture, between plates 5′′ and 6′′ in the epitheca, and between plates 3′′′ and 4′′′ in the hypotheca (, ).
The sulcal area consists of five plates (, ). Plate S.a. is rather big and borders plates 1′ and 1′′ of the epitheca, plate 5′′′ in the hypotheca, plates C1 and C3 in the cingulum, S.s., S.m. (median sulcal), and S.d. (right sulcal) of the sulcal series. Plate S.d. is almost rectangular in shape and bears a small wing on its left side. Plate S.m. is very small, elongated, narrow and almost completely hidden by the wing of S.d. plate. Plate S.s. is the largest plate of the sulcal series and is in contact with plate 1C (). Plate S.p. is irregularly triangular in shape and in contact with S.d., S.s., 1′′′, 5′′′, and the two antapical plates (, , ). Plate 1′′′ is almost rectangular in shape, longer than wide. Plates 2′′′ and 4′′′ are quadrangular. Plates 3′′′ is pentagonal and plate 5′′′ is hexagonal and slightly wider than 1′′′ plate. The two antapical plates are wide and pentagonal; plate 1′′′′ is slightly smaller than plate 2′′′′ (, ).
Cyst production was not obtained, in the clonal strains or in the mixed cultures. Starved strains observed for two weeks never showed the formation of resting stages.
The samples from which P. vorax cultures were established were collected in the eastern part of the Gulf of Naples. The coastal area of the Gulf is characterized by relatively high chlorophyll and phytoplankton cell concentrations. Phytoplankton populations generally attain their maximum concentrations and productivity in late spring–early summer and are dominated by small-sized diatoms, although dinoflagellate blooms sometimes occur along the eastern part of the Gulf (Zingone et al., Citation1990, Citation1995; Ribera d’Alcalà et al., Citation2004).
Ultrastructure
Protoperidinium vorax has a typical dinoflagellate ultrastructure. The cell is surrounded by thecal plates (tp) and sutures (s) are clearly visible. The apical pore complex (apc) is detectable in longitudinal sections through the cell centre (). A large nucleus (n) is located in the equatorial part of the cell. It has permanently condensed chromosomes and a nucleolus (). The Golgi apparatus, comprising many dictyosomes, is located in the vicinity of the nucleus (, ). Numerous mitochondria of different sizes (mt) are scattered throughout the cytoplasm (, ). A large sac pusule (pus) is evident (, ) in the equatorial part of the cell or in the epicone. Several convoluted vesicular structures (ves) are located in the peripheral portion of the cell (). These structures are formed of a series of more or less packed and convoluted membranes. The dimensions of the vesicular structures vary between cells and according to the orientation of the TEM sections. In some specimens vesicles are numerous and large, and the membranes are more widely separated from each other () ; in others, vesicles are less numerous and the membranes are compacted (). Electron-dense dark accumulation bodies (ab) and bodies containing homogeneously stained material, probably lipids, (lb) are located toward the periphery of the cell (). Empty vesicles are often present beneath the cell wall, and might represent accumulation bodies and/or lipid bodies, emptied due to sub-optimal fixation procedures. Vesicles containing fibrous material are also visible (fv) (). Several trichocysts (tr) are scattered in the cytoplasm (). The trichocyst body is surrounded by a membrane-bound vesicle. This vesicle is connected to the thecal surface by a tubular structure that opens through the cell wall by a pore, through which the trichocyst body is extruded. These pores are visible on the thecal plates next to the plate sutures ( –, , , ). When cut transversally, trichocysts appear as irregular quadrangular polygonal bodies (), whereas they appear as long, electron-dense dark bodies when cut longitudinally (). In this view, the end of the trichocyst directed toward the cell surface often appears covered by many long spines, possibly representing an early stage of trichocyst body extrusion.
Figs 16–25. TEM micrographs of Protoperidinium vorax sp. nov. . Longitudinal section of the whole cell. . Longitudinal section of the whole cell. . Detail of the nucleus with the nucleolus. . Golgi apparatus, detail of . . Large vesicular structure with many convoluted membranes. . Small vesicular structure with compressed membranes. . Accumulation and lipid bodies, detail of . . Fibrous vesicles. . Longitudinal section of the terminal portion of a trichocyst, detail of . . Transverse section of a trichocyst, detail of . Abbreviations: (tp) thecal plate; (apc) apical pore complex; (s) thecal suture; (n) nucleus; (pus) sac pusule; (ves) vesicular structure; (ab) accumulation body; (lb) lipid body; (fv) fibrous vesicle; (tr) trichocyst; (mt) mitochondrion. Scale bars: , : 5 µm; , : 2 µm; , , , : 1 µm; , : 0.5 µm.
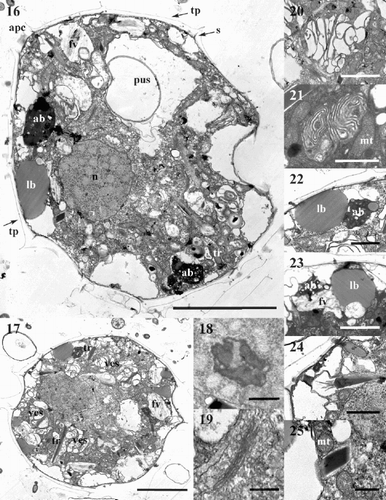
Feeding behaviour
Protoperidium vorax is a pallium-feeder and was observed feeding on various species, both small, unicellular (Thalassiosira sp.) and chain-forming diatoms (S. pseudocostatum, Chaetoceros spp. and Leptocylindrus danicus Cleve). It has been observed feeding on single cells, colonies of different size, and also on a single, or a few cells within a colony. Each time P. vorax hit potential prey, it started to rotate around its transapical axis and was observed moving to different points along the diatom chain. When it attempted to capture a cell, it stopped moving with its sulcal region facing the selected prey. After a few seconds, a membranous body, the pallium, was visible at the point of contact between the dinoflagellate and the diatom (, ). The pallium developed quickly and surrounded the cell or the colony by amoeboid movement. During the feeding process, the diatom cytoplasm gradually changed consistency and was progressively sucked into the dinoflagellate. When the digestion process was over, the pallium was reabsorbed in the sulcal area () and the diatom frustule(s) was left empty or almost so (). The whole feeding process was relatively quick, lasting 5–15 min, depending on the size and number of diatom cells.
Figs. 26–29. Feeding behaviour of Protoperidinium vorax sp. nov. . P. vorax starts to surround a colony of Skeletonema pseudocostatum with the pallium (arrowed). . Final phase of the feeding process, the empty diatom is still surrounded by the pallium. . P. vorax retrieves the empty pallium (same cells as in ). . Empty diatom frustules left behind after feeding is completed (same cells as in and ). Scale bars: 10 µm.
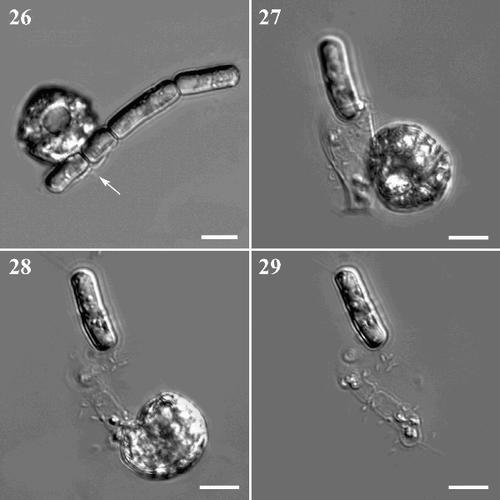
In the feeding experiments carried out with S. pseudocostatum under non-limiting food concentrations, P. vorax division rates were between 0.67 and 1.09 d−1 (mean = 0.89d−1), corresponding to doubling rates from 0.97 to 1.57 doublings d−1 (mean = 1.28 doublings d−1). Prey ingestion rates varied from 33.02 to 37.63 cells · dinoflagellate−1 h−1 (mean = 35.95 cells dinoflagellate−1 h−1) and clearance rates from 17.07 to 19.93 µl dinoflagellate−1 h−1 (mean = 18.34 µl dinoflagellate −1h−1). Cannibalism was never observed.
Discussion
Taxonomy
Species of the subgenus Protoperidinium have been subdivided into three groups based on the shape of plate 1′: ortho (quadrangular), meta (pentagonal) and para (hexagonal) species. For each of these three groups, an additional subdivision has been suggested, depending on the shape of the second intercalary plate (2a): 4 sides (quadra species), 5 sides (penta species) and six sides (hexa species) (Balech, Citation1974). A somewhat different classification system was suggested for Peridinium Ehrenberg (including freshwater Peridinium and marine Protoperidinium species) by Abé (Citation1981). Different subgenera, groups and sections were established based on the combination of characters such as the shape of plates in the ventral area (both first apical plate and plates in the sulcal region, with major emphasis on the sulcal posterior plate) and the form of the antapical appendages. Other characters, such as the shape of the cell, the presence of horns and/or spines and the shape and arrangement of individual plates are also considered for species identification.
Protoperidinium vorax is a small, spherical dinoflagellate with an ortho-hexa arrangement (sensu Balech, Citation1974) of the first apical and second intercalary plates, respectively. To our knowledge, there are no other Protoperidinium species with this plate pattern and morphology. The presence of only three cingular plates and the unusual size and shape of the second intercalary plate (2a) that extends behind the first intercalary plate to abut the second precingular plate are peculiar characters of this species. The genus Protoperidinium is characterized by having four cingular plates (Steidinger & Tangen, Citation1997). In the majority of species, the first cingular plate is a small transitional plate (t-plate), located at the cingulum–sulcus juncture. However, some species (e.g. Protoperidinium americanum (Gran & Braarud) Balech, Protoperidinium parthenopes Zingone & Montresor) have a rather wide first cingular plate, which cannot be considered a ‘t-plate’. This is also the case for P. vorax. In the vast majority of Protoperidinium, the third cingular plate is the widest cingular plate and runs along the dorsal part of the cell, joining the fourth cingular plate on the right ventral part of the cingulum. There are, however, exceptions to this pattern. In P. americanum and P. parthenopes, for example, the second, third and fourth cingular plates are similar in size and the suture between plates 2C and 3C is located on the dorsal part of the cell. Notwithstanding its peculiarity in having only three cingular plates, we decided to retain P. vorax within Protoperidinium, pending further investigations to define the phylogenetic relationships within the genus.
In Protoperidinium the dorsal epithecal plate arrangement generally has plates 3′, 2a and 4′′ mid-dorsally aligned (bipepsioid arrangement), but, less commonly, these plates are not so aligned (cinctioid arrangement) (Fensome et al., Citation1993). However, of those species characterized by a cinctioid epithecal arrangement, none have been described in which plate 2a extends as far to the ventral side of the cell and abuts plate 2′′, as in P. vorax. An elongated 2a plate bordering plate 2′′ is only reported for species of the subgenus Archaeperidinium, which are characterized by having only 2 intercalary plates.
A number of Protoperidinium species share the cellular outline of P. vorax (). Protoperidinium americanum and P. parthenopes have the same ortho-hexa plate arrangement as P. vorax, although P. americanum can be distinguished from P. vorax by its larger size and the presence of four intercalary plates (Lewis & Dodge, Citation1987). Protoperidinium parthenopes differs from P. vorax in being slightly larger and having differently shaped intercalary plates and, consequently, apical and precingular ones (Zingone & Montresor, Citation1988). Protoperidinium asymmetricum (Abé) Balech, incorrectly placed in Archaeperidinium by Balech (Citation1974), possesses an ortho-penta arrangement and differs from P. vorax in having a straight suture ridge on the epitheca, diagonally crossing the apical pore. Some Archaeperidinium species (Protoperidinium minutum (Kofoid) Loeblich, Protoperidinium monospinum (Paulsen) Zonneveld & Dale, Protoperidinium mutsuense (Abé) Balech) also have rounded cells similar to P. vorax (). However, these species possess only two intercalary plates. P. vorax shares the morphology of plate 1′, which has the shape of a truncated asymmetrical lozenge, with P. mutsuense and P. parthenopes. The form of the sulcal posterior plate also varies among the apparently closely related species (), as well as the pattern of thecal plate ornamentation and the morphology of the cysts. It is therefore extremely difficult to deduce relationships among these species based on the morphological characters of their cells and/or cysts.
Table 1. Wall features of Protoperidinium and Archaeperidinium spp. of similar size and shape as P. vorax sp. nov. Superscript numbers indicate source of data as listed in the bottom row
Motile cells of P. vorax were obtained by incubating sediment samples and we therefore expected to obtain resting cysts in our cultures. The sediment samples from which P. vorax was isolated contained small sized (∼20 µm diameter) cysts attributable to heterotrophic dinoflagellates (Siano, unpublished data). These cysts had finely granular, green–brown contents, without a stigma. Both smooth cysts and cysts ornamented by thin spines were present in the samples, and both morphotypes were characterized by a brown cyst wall. Unfortunately, we were not able to obtain cyst production in cultures of P. vorax, in clonal strains or in mixed cultures. We present three hypotheses to explain the lack of cyst formation: (i) P. vorax does not produce resting stages but only temporary cysts; (ii) P. vorax is heterothallic and produces cysts only after conjugation of strains of opposite mating type (we only obtained a few strains and they could have belonged to the same mating type); (iii) the culture conditions under which our strains were maintained were not appropriate for inducing cyst production. Starvation has been reported to induce encystment in the heterotrophic dinoflagellates Polykrikos schwartzii Bütschli and Polykrikos kofoidii Chatton (Nagai et al., Citation2002), but there is extremely limited information on the factors that induce encystment in heterotrophic species or on their life cycles in general.
Ultrastructure
The TEM sections of P. vorax showed the presence of a large sac pusule, which is also visible in LM and occupies a relatively wide portion of the cell. Different kinds of pusule have been described for freshwater and marine dinoflagellates, both thecate and naked. Two main types of pusules have been described: (i) pusules comprising a more or less large chamber located close to the flagellar area; (ii) pusules with a large chamber and a rather complex tubular system (Dodge, Citation1972). In pallium feeders, such as P. spinulosum Schiller, the pusule appears as a large sac connected to a complex system of organelles that constitute the feeding apparatus (Jacobson & Anderson, Citation1992).
Several vesicular structures with convoluted membranes were present in the cytoplasm of P. vorax, but their function is not clear. They might be a storage system for the pallium membranes. The pallium is a membranous sac extruded from the sulcal area to envelop the prey and subsequently retracted within the cell, when digestion is complete (Jacobson & Anderson, Citation1992). It seems reasonable that the dinoflagellate does not synthesize the membrane system de novo every time it feeds, but might store it within the cell. Jacobson & Anderson (Citation1992) described a system of fingerprint-like membranous whorls in the sulcal region of cells that were extruding the pallium. They interpreted these ultrastructural features as condensed storage-bodies. The membranous whorls described for P. spinulosum are smaller than the membranous vesicles of P. vorax, but very similar in shape (Jacobson & Anderson, Citation1992).
When excited with blue light, P. vorax shows several green-fluorescing globular bodies scattered in the cytoplasm. These might correspond to the accumulation bodies seen in TEM sections. They are similar to the PAS (Periodic Acid-Schiff) bodies described for Gonyaulax polyedra Stein (=Lingulodinium polyedrum (Stein) Dodge), Alexandrium tamarense (Lebour) Balech (= Gonyaulax tamarensis Lebour) (Schmitter, Citation1971), Prorocentrum lima (Ehrenberg) Dodge, P. maculosum Faust (Zhou & Fritz, Citation1994), Pyrocystis lunula (Schütt) Schütt and P. noctiluca Murray ex Schütt (Seo & Fritz, Citation2002). PAS bodies are described as containing electron-dense, fibrillar and membranous material, often enclosed by smooth endoplasmic reticulum and considered equivalent to lysosomes (Zhou & Fritz, Citation1994). PAS bodies in the above species also fluoresce green under blue light excitation.
Feeding behaviour
Protoperidinium vorax was observed feeding on different unicellular (Thalassiosira sp.) and chain-forming diatom species (S. pseudocostatum, Chaetoceros spp. and L. danicus). Feeding experiments were carried out with S. pseudocostatum, one of the most abundant diatoms in the Gulf of Naples (Ribera d’Alcalà et al., Citation2004). The feeding behaviour of P. vorax is similar to that described for other Protoperidinium species (Jacobson & Anderson, Citation1986) and for other heterotrophic dinoflagellates of comparable size, such as the freshwater peduncle-feeder Peridiniopsis berolinensis (Lemmermann) Bourelly (Calado & Moestrup, Citation1997), the heterotrophic Oblea rotunda (Lebour) Balech (Strom & Buskey, Citation1993) and the ectoparasitic Paulsenella sp. (Schnepf & Drebes, Citation1986). In general, heterotrophic dinoflagellates spent rather a long time feeding. Values reported for other pallium feeders (Protoperidinium spp., O. rotunda, Zygabikodinium lenticulatum Loeblich Jr. & Loeblich III) show that the feeding process can last from 7 to 30 min, exceptionally up to 2 h in O. rotunda (Jacobson & Anderson, Citation1986, Citation1993). In P. vorax the time spent in exploring for prey and for prey capture and ingestion are shorter (5–15 min).
P. vorax exhibited high growth, ingestion and clearance rates. Its growth rate was higher than that reported for other similar-sized heterotrophic dinoflagellates such as O. rotunda (Strom & Buskey, Citation1993) and Diplopsalis lenticula Bergh (Naustvoll, Citation1998), although slightly higher growth rates, up to 1.23 d−1 have been reported for Protoperidinium hirobis Abé (Jacobson & Anderson, Citation1993). Ingestion and clearance rates for P. vorax are the highest recorded for a thecate heterotrophic dinoflagellate (see Naustvoll, Citation1998, table 2). Experiments with small sized species such as O. rotunda, D. lenticula and P. hirobis were carried out at the same temperature conditions as those used for P. vorax (19–20°C). However, the small (∼20 µm in diameter) dinoflagellates were fed with larger diatom prey (Ditylum brightwellii (West) Grunow in Van Heurck and L. danicus) and should have required more time to digest the food items. Differences in feeding rates would also appear less marked if data were expressed in terms of biomass instead of cell numbers.
P. vorax is very difficult to identify in fixed natural samples, like many other small and relatively featureless heterotrophic dinoflagellates. To assign such small dinoflagellates to trophic categories, as heterotrophs, autotrophs or mixotrophs, live samples should be examined under epifluorescence microscopy, but this is rarely done during routine monitoring programmes. Experienced workers may be able to recognize autotrophic species, on the presence of chloroplasts and distinct pigmentation, or species of the ‘Diplopsalis group’ (sensu Abé, Citation1981) on the structure of cingular and sulcal lists (membranous extensions, see Steidinger & Tangen Citation1997). However, this requires considerable time, and small dinoflagellates are often lumped in size categories. Small thecate heterotrophs are thus grouped with small phototrophic dinoflagellates such as round Scrippsiella species (e.g. S. rotunda Lewis or S. operosa (Deflandre) Montresor) or Heterocapsa spp. It follows that the abundance of such small heterotrophs is poorly assessed. The relatively high growth and feeding rates for P. vorax and other small heterotrophic dinoflagellates would suggest a potential role in controlling diatom blooms. However, small thecate heterotrophic species seldom reach high concentrations in coastal environments, and this suggests marked top-down pressure from grazers, such as small crustaceans and/or ciliates.
Acknowledgments
We wish to thank Prof. Marta Maria Giannone for translating the species diagnosis into Latin, Gennaro Iamunno for technical assistance with electron microscopy, Ugo Sacchi for assistance with the Axiocam, Ciro and Gianluca Zazo for help during sediment sampling.
References
References
- Abé , TH . 1927 . Reports of the biological survey of Mutsu Bay 29. Notes on the protozoan fauna of Mutsu bay. II. Peridiniales . Sci. Rep. Tohoku Imp. Univ. Ser. 4 , 2 : 383 – 438 .
- Abé , TH . 1936 . Reports of the biological survey of Mutsu Bay 29. Notes on the protozoan fauna of Mutsu bay. II. Genus Peridinium; subgenus Archaeperidinium . Sci. Rep. Tohoku Imp. Univ. Ser. 4 , 10 : 639 – 486 .
- Abé , TH . 1981 . Studies on the family Peridinidae, an unfinished monograph of the armoured Dinoflagellata . Pub. Seto Mar. Biol. Lab. Special Pub. Ser. , 6 : 1 – 409 .
- Balech , E . 1964 . Tercera contribucion al conocimiento del genero Peridinium . Rev. Mus. Arg. C. Nat. B. Rivadavia Hidrobiologia , 1 : 180 – 195 .
- Balech , E . 1974 . El genero Protoperidinium Bergh, 1881 (Peridinium Bergh, 1831, partim) . Rev. Mus. Arg. C. Nat. B. Rivadavia Hidrobiologia , 4 : 1 – 79 .
- Berggreen , U , Hansen , B and Kiorboe , T . 1988 . Food size spectra, ingestion and growth of the copepod Acartia tonsa during development: implications for determinations of copepod production . Mar. Biol. , 99 : 341 – 352 .
- Calado , AJ and Moestrup , Ø . 1997 . Feeding in Peridiniopsis berolinensis (Dinophyceae): new observations on tube feeding by an omnivorous, heterotrophic dinoflagellate . Phycologia , 36 : 47 – 59 .
- Dodge , JD . 1972 . The ultrastructure of the dinoflagellate pusule: a unique osmo-regulatory organelle . Protoplasma , 75 : 285 – 302 .
- Fensome RA Taylor FJR Norris G Sarjeant WAS Wharton DJ Williams GL 1993 A classification of living and fossil dinoflagellates Micropaleontology, Special Publication Number 7. Sheridan Press, Hanover, Pennsylvania
- Fritz , L and Triemer , RE . 1985 . A rapid simple technique utilizing Calcofluor White M2R for the visualization of dinoflagellate thecal plates . J. Phycol. , 21 : 662 – 664 .
- Frost , BW . 1972 . Effects of size and concentration of food particles on the feeding behavior of the marine planktonic copepod Calanus pacificus Limnol . Oceanogr , 17 : 805 – 815 .
- Fukuyo , Y , Kittaka , J and Hirano , R . 1977 . Studies on the cysts of marine dinoflagellates. I. Protoperidinium minutum (Kofoid) Loeblich . Bull. Plankton Soc. Jap. , 24 : 11 – 19 .
- Hansen , PJ . 1991 . Quantitative importance and trophic role of heterotrophic dinoflagellates in a coastal pelagic food web . Mar. Ecol. Prog. Ser. , 73 : 253 – 261 .
- Hansen , PJ . 1992 . Prey size selection, feeding rates and growth dynamics of heterotrophic dinoflagellates with special emphasis on Gyrodinium spirale . Mar. Biol. , 114 : 327 – 334 .
- Heinbokel , JF . 1978 . Studies on the functional role of tintinnids in the southern California Bight. I. Grazing and growth rates in laboratory cultures . Mar. Biol. , 47 : 177 – 189 .
- Jacobson , DM . 1999 . A brief history of dinoflagellate feeding research . J. Eukaryot. Microbiol. , 46 : 376 – 381 .
- Jacobson , DM and Anderson , DM . 1986 . Thecate heterotrophic dinoflagellates: feeding behavior and mechanisms . J. Phycol. , 22 : 249 – 258 .
- Jacobson , DM and Anderson , DM . 1992 . Ultrastructure of the feeding apparatus and myonemal system of the heterotrophic dinoflagellate Protoperidinium spinulosum . J. Phycol. , 28 : 69 – 82 .
- Jacobson , DM and Anderson , DM . 1993 . Growth and grazing rates of Protoperidinium hirobis Abé a thecate heterotrophic dinoflagellate . J. Plankton Res. , 15 : 723 – 736 .
- Jacobsen , HH and Hansen , PH . 1997 . Prey selection, grazing and growth response of the small heterotrophic dinoflagellate Gymnodinium sp. and the ciliate Balanion comatum: a comparative study . Mar. Ecol. Prog. Ser. , 158 : 75 – 86 .
- Jeong , HJ . 1999 . The ecological roles of heterotrophic dinoflagellates in marine planktonic communities . J. Eukaryot. Microbiol. , 46 : 390 – 396 .
- Keller , MD , Selvin , RC , Claus , W and Guillard , RRL . 1987 . Media for the culture of oceanic ultraphytoplankton . J. Phycol. , 23 : 633 – 638 .
- Kiorboe , T and Nielsen , TG . 1994 . Regulation of zooplankton biomass and production in a temperate, coastal ecosystem. 1. Copepods . Limnol. Oceanogr. , 39 : 493 – 507 .
- Kjaert , AH , Naustvoll , L-J and Paasche , E . 2000 . Ecology of the heterotrophic dinoflagellate genus Protoperidinium in the inner Oslofjord (Norway) . Sarsia , 85 : 453 – 460 .
- Lessard , EJ . 1991 . The trophic role of heterotrophic dinoflagellates in diverse marine environments . Mar. Microb. Food Webs , 5 : 49 – 59 .
- Levinsen , H , Nielsen , TG and Hansen , BW . 1999 . Plankton community structure and carbon cycling on the western coast of Greenland during the summer situation. II. Heterotrophic dinoflagellates and ciliates . Aquat. Microb. Ecol. , 16 : 217 – 232 .
- Levinsen , H and Nielsen , TG . 2002 . The trophic role of marine pelagic ciliates and heterotrophic dinoflagellates in arctic and temperate coastal ecosystem: a cross-latitude comparison . Limnol. Oceanogr. , 47 : 427 – 439 .
- Lewis , J and Dodge , JD . 1987 . The cyst-theca relationship of Protoperidinium americanum (Gran and Braarud) Balech . J. Micropaleontol. , 6 : 113 – 121 .
- Nagai , S , Matsuyama , Y , Takayama , H and Kotani , Y . 2002 . Morphology for Polykrikos kofoidii and P. schwartzii (Dinophyceae, Polykrikaceae) cysts obtained in culture . Phycologia , 41 : 319 – 327 .
- Naustvoll , LJ . 1998 . Growth and grazing by the thecate heterotrophic dinoflagellate Diplopsalis lenticula (Diplopsalidaceae, Dinophyceae) . Phycologia , 37 : 1 – 9 .
- Olseng , CD , Naustvoll , L-J and Paasche , E . 2002 . Grazing by the heterotrophic dinoflagellate Protoperidinium steinii on a Ceratium bloom . Mar. Ecol. Prog. Ser. , 225 : 161 – 167 .
- Ribera d’Alcalà , M , Conversano , F , Corato , F , Licandro , P , Mangoni , O , Marino , D , Mazzocchi , MG , Modigh , M , Montresor , M , Nardella , M , Saggiomo , V , Sarno , D and Zingone , A . 2004 . Seasonal patterns in plankton communities in a pluriannual time series at a coastal Mediterranean site (Gulf of Naples): an attempt to discern recurrences and trends . Sci. Mar. , 68 ( Suppl. 1 ) : 65 – 83 .
- Schiller J 1937 Dinoflagellatae (Peridineae) in monographischer Behandlung 2. Teil, Akademische Verlagsgesellschaft Leipzig
- Schmitter , RE . 1971 . The fine structure of Gonyaulax polyedra a bioluminescent marine dinoflagellate . J. Cell Sci. , 9 : 147 – 173 .
- Schnepf , E and Drebes , G . 1986 . Chemotaxis and appetence of Paulsenella sp. (Dinophyta), an ectoparasite of the marine diatom Streptotheca thamesis Shrubsole . Planta , 167 : 337 – 343 .
- Seo , KS and Fritz , L . 2002 . Ultrastructure of vegetative cysts of Pyrocystis (Dinophyta), with special reference to PAS bodies and trichocysts . Phycologia , 41 : 10 – 14 .
- Steidinger KA Tangen K 1997 Dinoflagellates Identifying Marine Phytoplankton (Tomas, C.R., editor) 387 584 Academic Press San Diego CA
- Stoecker , DK . 1999 . Mixotrophy among dinoflagellates . J. Eukaryot. Microbiol. , 46 : 397 – 401 .
- Strom , SL and Buskey , EJ . 1993 . Feeding, growth and behavior of the thecate heterotrophic dinoflagellate . Oblea rotunda Limnol. Oceanogr. , 38 : 965 – 977 .
- Verity , PG , Stoecker , DK , Sieraki , ME , Burkill , PH , Edwards , ES and Tronzo , CR . 1993a . Abundance, biomass and distribution of heterotrophic dinoflagellates during the North Atlantic spring bloom . Deep-Sea Res. , 40 : 227 – 244 .
- Verity , PG , Stoecker , DK , Sieraki , ME and Nelson , JR . 1993b . Grazing, growth and mortality of microzooplankton during the 1989 North Atlantic spring bloom at 47°N, 18°W. . Deep-Sea Res. , 40 : 1793 – 1814 .
- Wall , D and Dale , B . 1968 . Modern dinoflagellate cysts and evolution of the Peridiniales . Micropaleontology , 14 : 265 – 304 .
- Zhou , J and Fritz , L . 1994 . The PAS accumulation bodies in Prorocentrum lima and Prorocentrum maculosum (Dinophyceae) are dinoflagellate lysosomes . J. Phycol. , 30 : 39 – 44 .
- Zingone , A and Montresor , M . 1988 . Protoperidinium parthenopes sp. nov. (Dinophyceae), an intriguing dinoflagellate from the Gulf of Naples . Cryptogam. Algol. , 9 : 117 – 125 .
- Zingone , A , Montresor , M and Marino , D . 1990 . Summer phytoplankton physiognomy in coastal waters of the Gulf of Naples . P.S.Z.N.I: Mar. Ecol. , 11 : 157 – 172 .
- Zingone , A , Casotti , R , Ribera d’Alcalà , M , Scardi , M and Marino , D . 1995 . ‘St Martin's Summer’: the case of an autumn phytoplankton bloom in the Gulf of Naples (Mediterranean Sea) . J. Plankton Res. , 17 : 575 – 593 .
- Zonneveld , KA and Dale , B . 1994 . The cyst-motile stage relationships of Protoperidinium monospinum (Paulsen) Zonneveld et Dale comb. nov. and Gonyaulax verior (Dinophyta, Dinophyceae) from the Oslo Fjord (Norway) . Phycologia , 33 : 359 – 368 .