Abstract
The extracellular polysaccharides (EPS) released by a freshwater cryptophyte, Cryptomonas tetrapyrenoidosa were characterized and their degradation by heterotrophic microbial populations from the same habitat, a tropical eutrophic reservoir, was evaluated. The EPS were purified by anion exchange column chromatography, the monosaccharide composition was determined by gas-chromatography, and the linkages of the monosaccharides by GC-MS. The EPS were separated by anion exchange into two different acidic fractions with completely different compositions. Fraction 1 was a complex branched heteropolysaccharide dominated by fucose, N-acetyl glucosamine, mannose and galactose. Fraction 2 showed a less complex pattern, which was composed mainly of 1,3-linked glucuronic acid and 1,3-linked galactose. We monitored the concentrations of the monosaccharides in the EPS during microbial degradation using Sepharose CL6B gel filtration and PAD-HPLC. After seven days, the main EPS were degraded into galactose-rich lower molecular weight carbohydrates, evidencing degradation in two steps. The decay patterns of the monosaccharides varied and, in the higher molecular weight (Fraction A), fucose and galactose were degraded more rapidly than rhamnose, glucose and man/xyl. In Fraction B, although the decay coefficients were lower and more homogenous, fucose and rhamnose were degraded more rapidly than galactose, glucose and man/xyl. In spite of the complexity of the EPS, and the specialized degradation pattern, the natural microbial community from the Barra Bonita reservoir was able to degrade the entire polysaccharide mixture in about 21 days.
Introduction
Extracellular polysaccharide (EPS) production by phytoplanktonic populations has been focused on in several studies because of its significance for aquatic systems (Jensen, Citation1983; Giroldo & Vieira, Citation2002). Metal complexation and transport to sediments (Lombardi & Vieira, Citation1998), formation of aggregates and transparent exopolymer particles (Passow et al., Citation1994), and enhancement of functional biodiversity in planktonic environment (Wood & Van Valen, Citation1990) are processes of recent interest, which include phytoplanktonic EPS participation. In many species, EPS production rates increase under nutrient deficiency as a consequence of an excess of photoassimilated carbon (Fogg, Citation1983), and excretion acts as an overflow mechanism to avoid damage in the photosynthetic apparatus (Smith & Underwood, Citation2000).
Carbohydrate release supplies resources of high quality for the heterotrophic community, especially for planktonic bacterial populations. The utilization of such compounds by natural bacterial communities has been demonstrated (Lovell & Konopka, Citation1985; Freire-Nordi & Vieira, Citation1996, Citation1998; Colombo et al., Citation2004), evidencing them as a carbon sources able to support dense bacterial populations in spite of their molecular complexity. Bacterial activity on phytoplanktonic EPS is believed to accelerate the mineralization of essential nutrients, especially on aggregates, such that phytoplanktonic growth would also be enhanced (Wood & Van Valen, Citation1990; Azam & Smith, Citation1991), possibly leading to co-evolution. However, studies correlating the EPS production by phytoplankton with the bacterial utilization of such compounds are rare (Freire-Nordi & Vieira, Citation1996, Citation1998; Colombo et al., Citation2004), as are the characterization of specific algal/bacterial associations based on the organic compounds released by phytoplankton (Bell & Mitchell, Citation1972; Bell & Sakshaug, Citation1980; Fallowfield & Daft, Citation1988).
Despite the remarkable significance of the Cryptophyta in the most diverse habitats, such as sea, freshwater, soil and ice, the physiology of this group is still little studied compared to other taxonomic groups (Giroldo & Vieira, Citation1999, Citation2002). Apart from taxonomic investigations (Klaveness, Citation1985), most studies deal with growth requirements, photosynthetic features and responses to environmental factors in the genus Cryptomonas (Gervais, Citation1997; Ojala, Citation1993; Giroldo & Vieira, Citation1999; Sciandra et al., Citation2000). EPS production by Cryptophyta has received little attention (Paulsen et al., Citation1992; Giroldo & Vieira, Citation2002) and previous work has not investigated microbial utilization of cryptophyte EPS.
Since cryptophytes produce large amounts of EPS (Paulsen et al., Citation1992; Giroldo & Vieira, Citation2002) and also may dominate tropical (Dias, Citation1990; Calijuri & Dos Santos, Citation2001) and temperate environments (Ojala, Citation1993; Gervais, Citation1997), these compounds may have considerable significance in the dissolved organic matter pool of water bodies. Studies of cryptophyte EPS characteristics, as well as their significance to the heterotrophic community and to carbon cycling in aquatic systems, would improve our knowledge on the functions of this group. The aim of this work was to characterize the EPS produced by a tropical freshwater strain of Cryptomonas tetrapyrenoidosa and to investigate their degradation by natural bacterial populations. PAD-HPLC (Gremm & Kaplan, Citation1997) was used to monitor the degradation pattern of each component of the EPS in order to observe alterations in composition during bacterial activity.
Materials and methods
Organisms and culture conditions
Cryptomonas tetrapyrenoidosa Skuja and the ambient heterotrophic microbial community were collected in the Barra Bonita reservoir, located in the Tiete River, SP, Brazil (22° 29's, 48° 34’W). This is a eutrophic human-made lake built mainly to generate electricity. The surface area is 324.84 km2 and the maximum depth 30 m, giving a maximum volume of 3.1 × 106 m3 (Tundisi & Matsumura-Tundisi, Citation1990). C. tetrapyrenoidosa was isolated directly by microscopy, and axenic cultures were obtained by washing individual cells with fresh medium. The culture medium was WC (Guillard & Lorenzen, Citation1972), and the cultures were kept under 100 µmol m−2 s−1 (Photosynthetically Active Radiation) at 22 ± 1°C and in a dark : light cycle 12 h:12 h. Such conditions do not reflect the large environmental variation found in the reservoir, where irradiance can reach 1500 µmol m−2 s−1 and the photoperiod can vary during the year, but C. tetrapyrenoidosa could frequently be found at 10–15% of the surface irradiance and at temperatures around 22°C. Tests for bacterial contamination in the axenic cultures were performed regularly with WC medium modified by the addition of glucose and peptone (250 mg l−1 per test).
Cryptomonas tetrapyrenoidosa cultures
A carboy containing 7 l of WC medium was inoculated with Cryptomonas tetrapyrenoidosa (300 ml, exponential growth phase, approximately 106 cell ml−1) and cultivated in duplicate under the above conditions. The cultures were bubbled with filtered air humidified in sterilized and acidified distilled water (pH 2.0). Samples were collected at 24-h intervals in the first 7 days and every 48 h during the remainder of the period, until the cultures reach the stationary growth phase. A 5-ml fraction was fixed with Lugol's to determine the cell density by direct counts (duplicate) under a microscope. The duplication time was calculated using the equation described by Fogg (Citation1975).
EPS isolation and characterization
After approximately 40 days of growth, Cryptomonas tetrapyrenoidosa axenic cultures (7 l) were filtered by tangential flow through a hollow fibre cartridge (1.0 mm diameter, 0.65 µm pore size; A/G Technology™, Amersham, UK). This cartridge permitted the filtration at a cartridge pressure near to zero, only with the peristaltic pump movement. Previous tests were performed to check cell viability after tangential flow filtration. We observed filtered cells by light microscopy and no damaged cells were found. In addition, intracellular phycoerythrin released by damaged cells coloured extracellular medium instantly, and no red colour was noted in the medium during this work. The filtrate was concentrated in a rotary evaporator at 40°C and dialysed against distilled water in dialysis tubes with a 12,000–14,000 D molecular weight cut-off. Two drops of toluene were added to the tubes to prevent contamination. The dialyzed material was freeze-dried and stored at −4°C under nitrogen. The freeze-dried material was further purified by anion exchange column chromatography, and analysed by gas chromatography and electron impact mass spectrometry after derivatizations as described below. The anion exchange column chromatography was performed using the batch separation method, without gradient, under the following conditions: gel = Sepharose DEAE fast flow (Pharmacia™, Peapack, New Jersey) in chloride form, bed dimensions = 2.5 × 15 cm, flow rate = 0.33 ml min−1, eluent I = distilled water, eluent II = 0.5 M NaCl, eluent III = 1.0 M NaCl and eluent IV: 2.0 M NaCl, running at the ambient temperature. Sodium azide (0.007 M) was used to avoid bacterial contamination. The column was first regenerated with 2.0 M NaCl and washed with distilled water. The sample was applied and eluted with distilled water to obtain the neutral fraction. Elution with 0.5 M NaCl yielded the weak acid fraction and final elution with 1.0 M NaCl gave the strong acid fraction. Carbohydrates were initially detected qualitatively in the fractions by the phenol–sulphuric method (Dubois et al., Citation1956). The fractions with significant amounts of carbohydrates were pooled, dialyzed against distilled water and freeze-dried. The monosaccharide composition of the EPS was determined by gas chromatography of the trimethylsilylated derivatives of the methyl-glycosides obtained by methanolysis using 4 M HCl in methanol at 80°C for 24 h (Barsett et al., Citation1992; Reinhold, Citation1972). Mannitol was used as an internal standard. Methylation of the polymers was carried out to determine the linkages of the monosaccharides (Kim & Carpita, Citation1992), followed by gas chromatographic analysis coupled with electron impact mass spectrometry (GC-MS) of the derived partially methylated alditol acetates (Barsett et al., Citation1992; Samuelsen et al., Citation1995). The fractions with more than 5% of uronic acids were reduced to the corresponding neutral sugars prior to methylation (Sims & Bacic, Citation1995). Protein content was determined in the fractions by the Lowry method (Lowry et al., Citation1951).
Microbial degradation of the EPS
The microbial inoculum was obtained from the euphotic zone (0–5 m, determined by a Spherical Quantum Sensor LI-193SA and a LI-250 Light Meter LiCor™ Lincoln, Nebraska). The sample was filtered aseptically at low vacuum pressure (10 mm Hg cm−1) through calcinated glass-fibre pre-filters AP-20 (Millipore™ Dublin, California) to remove particles, zoo- and phytoplankton. Fifty ml of this sample was inoculated in 1 l of culture medium prepared as follows: 0.5 l WC medium + 0.5 l water from the Barra Bonita reservoir + EPS (100 mg l−1, dry weight final concentration). The water from Barra Bonita reservoir to include in the culture medium was filtered through 0.45 µm pre-washed acetate membranes (Millipore™ Dublin, California). A control composed of culture medium without EPS was inoculated with the microbial community and served as a reference.
The experiments (two replicates plus control) were carried out in the dark at 25°C for 35 days. In spite of the limitation of such conditions in comparison to the field situation, they were chosen to prevent eventual algal growth and to observe the behaviour of bacterial communities under controlled conditions. EPS degradation was analysed in samples taken at 7-day intervals, which were filtered at low vacuum pressure (10 mm Hg cm−1) through 0.45 µm acetate membranes. The EPS degradation was first evaluated by gel filtration column chromatography, using Sepharose CL6B gel (Pharmacia™ Peapack, New Jersey; exclusion limits 104 to 106 D). Gel filtration column chromatography was used to monitor the transformation of the EPS into lower molecular weight carbohydrates by microbial activity. The gel filtration chromatography was performed under the following conditions: bed dimensions = 1.5 × 50 cm, flow rate = 1 ml 7 min−1, eluent = distilled water + 2% butanol, sample volume = 5% of total volume (6 ml), running at ambient temperature. Standard dextrans 104 and 2 × 106 D were used as references. Carbohydrates were detected qualitatively in the 5-ml fractions by the phenol–sulphuric method. The fractions with significant amounts of carbohydrates were pooled, hydrolysed and analysed by pulse amperometric detection in a high performance liquid chromatography (PAD-HPLC) (Gremm & Kaplan, Citation1997). The PAD-HPLC analyses were performed on a Dionex™ (Sunnyvalle, California) DX500, consisting of a PEEK version GP40 gradient pump module, an ED40 electrochemical detector, and an LC5 manual injector with a Rheodyne 9125 valve and a 25-µl peek sample loop. The ED40 was equipped with an amperometric flow cell, a gold working electrode, and an Ag/AgCl reference electrode. A PA-10 (Dionex™, Sunnyvalle, California) anion-exchange analytical column (4 × 250 mm), fitted with an appropriate guard-column (4 × 50 mm), was used to separate the monosaccharides. The eluent for the separation was 18 mM NaOH, and regeneration of the column used 200 mM NaOH at a flow rate of 1 ml min−1. All samples were passed through Bio Rad™ (Hercules, California) ionic exchange resin (AG2X8 – anion exchange and AG50W – cation exchange) to remove salts from culture media.
A first order decay equation was used to describe the EPS degradation following the reaction presented below:
The equation assumes the reaction rates are proportional to the reagent amounts and define a constant whose unit is day−1. The decay coefficients of the total polysaccharide and each monomer were calculated using the following equation:
The temporal variations of the EPS concentration were used to determine the decay coefficients. For this purpose a non-linear regression method was used (Levenberg-Marquardt algorithm), according to Press et al. (Citation1993). The parameterization shown above was used only to compare the degradation pattern of each EPS component and the first order equation was chosen because of its practicality.
Bacterial growth was evaluated by direct counts on an epifluorescence light microscope after staining the cells using 4′6′-diamino-2-phenylindole (DAPI) (Porter & Feig, Citation1980).
Results
Cryptomonas tetrapyrenoidosa growth
shows the growth of Cryptomonas tetrapyrenoidosa during 46 days and no senescent growth phase was observed, since the apparent cell decrease after 20 days was not statistically significant (ANOVA p = 0.314). The doubling time was 6.8 days and the stationary growth phase was well characterized around 20 days, when cells start to grow at a slower rate. At the end of the experiment, we obtained approximately 100 mg of high molecular weight material (dry weight) from approximately 6 × 104 cells ml−1.
EPS characterization
shows the anion exchange column chromatography of the EPS released by Cryptomonas tetrapyrenoidosa. The EPS was eluted in two fractions: Fraction 1 was eluted with 0.5 M NaCl and Fraction 2, the major one, with 1.0 M NaCl. Fraction 1, which represents only 48% of Fraction 2, is a complex heteropolysaccharide composed mainly of fucose, N-acetyl glucosamine, mannose, galactose and also small amounts of rhamnose, xylose, glucose, glucuronic acid and N-acetyl galactosamine (). Fraction 2 was quantitatively more significant than Fraction 1 and showed an uncommon composition for phytoplanktonic EPS, with 47% of glucuronic acid and 36% of galactose, besides smaller proportions of fucose, rhamnose, xylose, mannose, glucose, galacturonic acid, N acetyl-glucosamine and galactosamine. The low glucose percentage in both fractions demonstrated the low level of damaged cells after tangential flow filtration, since excess intracellular starch was not detected. A small amount (less than 0.01 mg) of a neutral fraction was detected in the polysaccharide, but it was not analysed due to the low amount. No EPS material was obtained during elution with 2.0 M NaCl.
Fig. 2. Anion exchange column chromatography (Pharmacia™ DEAE Sepharose fast flow) of the total EPS released by Cryptomonas tetrapyrenoidosa. Fraction 1: eluted with 0.5 M NaCl. Fraction 2: eluted with 1.0 M NaCl.
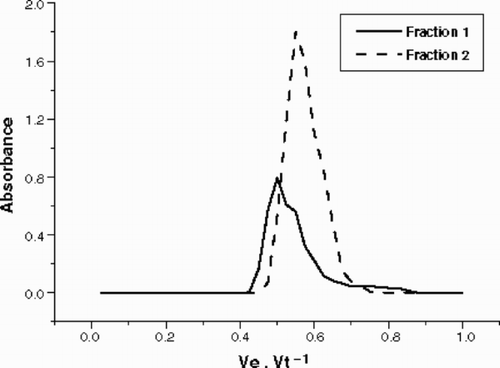
Table 1. Carbohydrate composition (%) determined by gas chromatographic analysis of the EPS released by Cryptomonas tetrapyrenoidosa, after methanolysis and TMS-derivatization of the fractions obtained by anion exchange column chromatography. Fraction 1: fraction eluted with 0.5 M NaCl. Fraction 2: fraction eluted with 1.0 M NaCl
shows the glycosidic linkages of the components of the two fractions. Fraction 1 showed a very complex pattern composed mainly of 1,3,4-linked N-acetyl glucosamine (16.8%), terminal fucose (12%), terminal mannose (11.7%), 1,4 N-acetyl galactosamine (8.6%) and terminal rhamnose (8.1%). This fraction had a central branched portion, composed mainly of N-acetyl glucosamine, fucose and glucuronic acid, which represented 16.8, 8.8 and 4.1%, respectively, of the EPS linkages. In the linear chains, rhamnose, fucose, xylose, mannose and galactose are also present in Fraction 1, as well as their terminal units. Proteins accounted for 9.8% of the EPS dry weight.
Table 2. Glycosidic linkages of the monosaccharides present in EPS from Cryptomonas tetrapyrenoidosa determined by GC-MS of the corresponding partly methylated alditol acetates. The percentages of each linkage in the same monosaccharide (%M) and in the total EPS (%T) are shown for the two fractions of the polysaccharide. F1: fraction eluted in anion exchange chromatography with 0.5 M NaCl. F2: fraction eluted in anion exchange chromatography with 1.0 M NaCl. T linkages represent terminal monosaccharides
Fraction 2 showed a less complex pattern because basically only two types of linked sugars made up 60% of the fraction (1,3-linked glucuronic acid – 36.8%; 1,3-linked galactose – 28%). This fraction was essentially a large linear chain composed of 1,3-linked glucuronic acid and galactose; however, there are a few branched points of glucuronic acid where terminal galactose units are probably located. We also found native methylated monosaccharides in fraction 2. 2-O-methyl-rhamnose, 3-O-methyl-rhamnose, 4-O-methyl-fucose, 3-O-methyl xylose and 4-O-methyl-galactose are present in minor amounts. As no 1,4/1,6 glucose was found, starch was not present in Fraction 1 or Fraction 2. Proteins accounted for 4% of the EPS dry weight.
EPS degradation
shows EPS degradation measured by gel filtration chromatography. The entire EPS (Fraction 1 plus 2) was mostly eluted in one fraction (Fraction A) near the reference Blue Dextran (2 × 106 D). Small amounts, probably fragments of the main polysaccharide, were eluted around 0.5 Ve Vt−1 (Fraction B). After 7 days, Fraction B increased considerably, probably due the transformation of EPS material from Fraction A to Fraction B by microbial degradation, suggesting a two-step degradation. In the rest of the experimental period, the amount of high molecular weight material was reduced considerably, and after 35 days both Fraction A and B were undetectable by the phenol–sulphuric method.
Fig. 3. Bacterial degradation of the total EPS released by Cryptomonas tetrapyrenoidosa measured by gel filtration column chromatography (Pharmacia™ CL6B). Arrows indicate standard dextrans employed to calibrate column: 2 × 106 and 104 D.
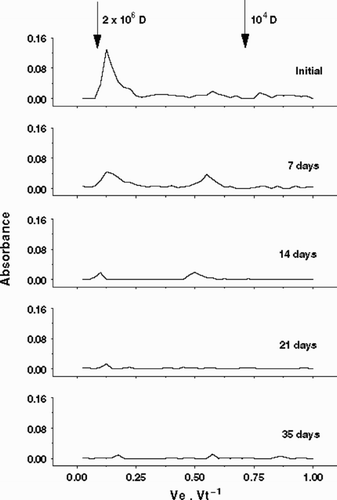
Mannose and xylose were not well separated by the PA-10 column because the PAD-HPLC was performed without a post-column system. The uronic acids and amino sugars were not measured in the PAD-HPLC because the desalting columns retained them. Though only two replicates were performed in this experiment, the coefficients of variation of the carbohydrate concentration averages were below 10%. Although some significant EPS components were not considered due to technical limitations, important information was obtained from this analysis. and show the decay kinetics of the EPS for Fraction A and B, respectively, as well as the decay coefficient of each component of the EPS. The decay coefficient of Fraction B was lower than that of Fraction A, probably because of the increase in the amounts of Fraction B after 7 days. In both Fraction A and B, the decay coefficient of each component of the EPS differed from that of the total EPS. In Fraction A (), fucose and galactose were degraded more rapidly than rhamnose, glucose and man/xyl, while in Fraction B () the decay coefficients were lower and more homogenous, but fucose and rhamnose were degraded more rapidly than galactose, glucose and man/xyl. shows the variation of the composition of the EPS during the degradation experiment, both in relative terms ( and ) and as absolute concentration of each EPS component ( and ). In Fraction A, fucose decreased in both absolute and relative proportion, while glucose increased in relative terms as a consequence of its slow degradation. In Fraction B, the relative proportions of the EPS components did not alter significantly during the experimental period, suggesting homogeneity in the degradation pattern of each EPS component. Only galactose increased its absolute amount and relative proportion significantly after 7 days, probably as a result of the two-step degradation of Fraction A. The high decay coefficient of galactose in Fraction A was correlated with the increase of galactose amounts and proportions in Fraction B. It means that the bacterial community removed the galactose units from EPS in smaller polymers to be degraded afterwards. Absolute data evidenced Fraction A as the main portion of Cryptomonas tetrapyrenoidosa EPS, however after 7 days, Fraction A and B showed almost the same amounts because of the two-step degradation process. These data confirmed the EPS dynamics observed in the gel filtration column chromatography (). Studies will be performed later where the effect on the acidic and amino-sugars will be reported.
Fig. 4. Bacterial degradation of Fraction A of the EPS released by Cryptomonas tetrapyrenoidosa measured by PAD-HPLC. Decay coefficients (k) are shown for each monosaccharide (except for uronic acids and the aminosugars), and also for the total EPS.
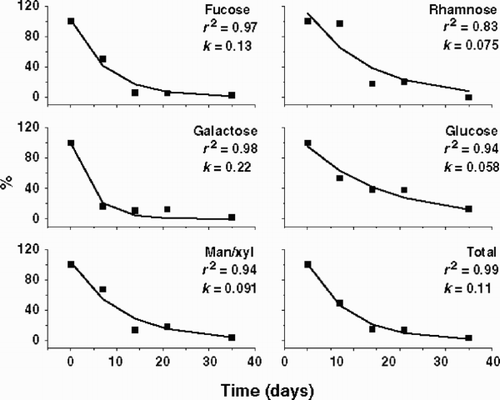
Fig. 5. Bacterial degradation of Fraction B of the EPS released by Cryptomonas tetrapyrenoidosa measured by PAD-HPLC. Decay coefficients (k) are shown for each monosaccharide (except for uronic acids and the aminosugars), and also for the total EPS.
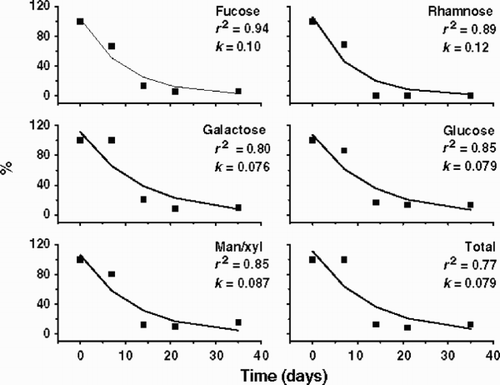
Fig. 6. Relative (A1, B1) and absolute (A2, B2) composition of EPS from Cryptomonas tetrapyrenoidosa during the microbial degradation period. A1: proportion of monomers in Fraction A (2 × 106 D); A2: concentration of monomers in Fraction A; B1: proportion of monomers in Fraction B (104 D); B2: concentration of monomers in Fraction B.
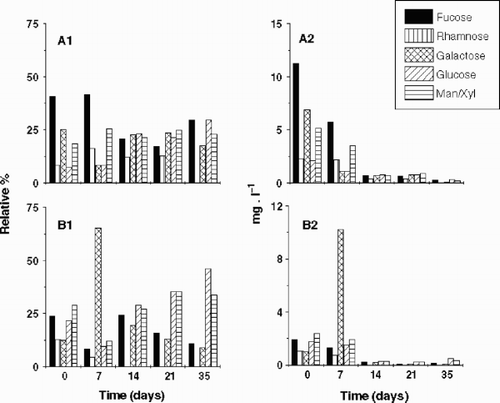
Bacterial growth
Bacterial growth in the cultures was significant (), increasing from 105 to 109 cells ml−1, and reaching the maximum density (2.4 × 109 cells ml−1) after 14 days of incubation. Bacterial growth in the controls was also significant, but two orders of magnitude lower than in the cultures, and reaching a maximum of only 1.45 × 107 cells ml−1 after 35 days of incubation. The growth was related to the degradation of the whole EPS, which decreased from approximately 27 to 0.1 mg l−1. The carbohydrate concentration in the controls was constant during the incubation period and did not reach more than 1 mg l−1. The carboydrate concentration found in the experiment was smaller than the 100 mg l−1 of dry material added in the culture medium, because of the loss caused by hydrolysis (approximately 50%), as well as the exclusion of uronic acids, amino sugars and proteins (approximately 15%). The rest (approximately 8%) was eluted in the gel filtration column chromatography as fragments of lower molecular weight and they were discarded before quantification.
Discussion
The EPS produced by Cryptomonas tetrapyrenoidosa are quite complex with 23 different linkages in the weak acid fraction and 14 in the strong acid fraction. The literature data on cryptophyte EPS production and characterization are rare, however, Paulsen et al. (Citation1992) described the structure of the EPS produced by a temperate soil Cryptomonas sp. and also found two fractions isolated by anion exchange chromatography. In that study, Fraction A had 12 different linkages while Fraction B had 16 linkages, and the major linkages were 1,4-linked galacturonic acid and 1,3-linked galactose in both fractions. Fraction 1 from our tropical phytoplanktonic C. tetrapyrenoidosa had a completely different composition with fucose, N-acetyl glucosamine, galactose and mannose as the major components of this heteropolysaccharide while Fraction 2 was similar with 1,3-linked galactose and 1,3 linked glucuronic acid, instead of 1,4 linked galacturonic acid. Giroldo & Vieira (Citation2002) described the composition of the EPS from a tropical strain of Cryptomonas obovata, which is also phytoplanktonic, but these contained a sulphated fucose-rich polysaccharide, which could have applications in the pharmaceutical industry, and was quite distinct from the EPS of C. tetrapyrenoidosa.
Despite of the complexity of the EPS from C. tetrapyrenoidosa, the microbial community from Barra Bonita reservoir was able to degrade the entire EPS in approximately one month, corroborating previous works with other taxonomic groups (Freire-Nordi & Vieira, Citation1996, Citation1998; Pacobahyba, Citation2002). However, these authors did not investigate the influence of degradation on the composition of the polysaccharide and there have been no studies relating the structure to the degradation of phytoplanktonic polysaccharides (Giroldo et al., Citation2003). Our results show that all components of the EPS are not degraded in the same way, as was also observed with Thalassiosira species by Aluwihare & Repeta (Citation1999) and Giroldo et al. (Citation2003). The variation in the decay coefficients could not be related exclusively to the structure of the EPS. Glucose, which had 100% of the linkages in carbon 1, 2, 4, 6, had the slowest decay coefficient. On the other hand, man/xyl also had a slow decay rate, but 70% of its linkages are terminal and, theoretically, more accessible to microbial communities than fucose, which had only 48.9% of the linkages in a terminal position and decayed rapidly.
The microbial community was able to degrade the lower molecular weight compounds in the EPS, as Freire-Nordi & Vieira (Citation1996) also observed. However, we were able to determine which monosaccharide was removed preferentially. Galactose showed a high decay coefficient in the main fraction (Fraction A) and its proportion increased substantially in the lower molecular weight fraction (Fraction B) after 7 days. This behaviour characterizes a two-step degradation, because the main EPS were transformed into lower molecular weight compounds before the complete degradation to inorganic carbon. Although the microbial community used in this paper had been collected in the same reservoir as that used by Giroldo et al. (Citation2003), the degradation pattern of EPS from C. tetrapyrenoidosa was quite distinct from that for Thalassiosira sp. It is an evidence that the carbon source influences the degradation pattern and suggests that specific compounds may select for specific bacterial population with different degradation patterns, as proposed by Bell & Mitchell (Citation1972), Bell & Sakshaug (Citation1980) and Fallowfield & Daft (Citation1988). This behaviour could increase the functional biodiversity in aquatic systems and also enhance the specific algae/bacteria associations in the planktonic environmental. Studies correlating phytoplanktonic EPS degradation with the bacterial population dynamics and systematics, as well as the role of specific enzymes would supply important information on the roles of such compounds in the algae/bacteria associations, as well as on the aquatic ecology.
Acknowledgements
We thank FAPESP (Processes 99/07766 and 99/01353-5) and PRONEX/CNPq for financing this project. The authors are indebted to Finn Tønnesen, University of Oslo, Norway, for performing the GC-MS analysis, Dr. Irineu Bianchini, Federal University of São Carlos, Brazil, for helping with the first order decay equation. We also thank the two anonymous referees and Professor Matthew Dring for manuscript improvement.
References
References
- Aluwihare , LI and Repeta , DJ . 1999 . A comparison of the chemical characteristics of oceanic DOM and extracellular DOM produced by marine algae . Mar. Ecol. Progr. Ser. , 186 : 105 – 117 .
- Azam F Smith DC 1991 Bacterial influence on the variability in the ocean biochemical state: a mechanicist view Particle Analysis in Oceanography NATO ASI Series G 27, (Demers, S., editor) 213 233 Springer Verlag Berlin
- Barsett , H , Paulsen , BS and Habte , Y . 1992 . Further characterization of polysaccharides in seeds from Ulmus glabra Huds . Carbohyd. Polym. , 18 : 125 – 130 .
- Bell , WH and Mitchell , R . 1972 . Chemotatic and growth responses of marine bacteria to algal extracellular products . Biol. Bull. , 143 : 265 – 277 .
- Bell , WH and Sakshaug , E . 1980 . Bacterial utilization of algae products. 2. A kinetic study of natural populations . Limnol. Oceanogr. , 19 : 833 – 839 .
- Calijuri , MC and Dos Santos , ACA . 2001 . Temporal variations in phytoplankton primary production in a tropical reservoir (Barra Bonita, SP-Brazil) . Hydrobiologia , 445 : 11 – 26 .
- Colombo , V , Vieira , AAH and Moraes , G . 2004 . Activity of glycosidases from freshwater heterotrophic microorganisms on degradation of extracellular polysaccharide produced by Anabaena spiroides (Cyanobacteria) . Braz. J. Microbiol. , 35 : 110 – 116 .
- Dias C 1990 Ciclo Anual do Fitoplâncton e Algumas Variáveis Ambientais an Lagoa do Infernão (SP) MSc Thesis Federal University of São Carlos (Brazil)
- Dubois , M , Guilles , KA , Hamilton , JK , Rebers , PA and Smith , F . 1956 . Colorimetric method for determination of sugars and related substances . Anal. Chem. , 28 : 350 – 356 .
- Fallowfield , HJ and Daft , MJ . 1988 . The extracellular release of dissolved organic carbon by freshwater Cyanobacteria and algae and the interaction with Lysobacter CP-1 . Br. Phycol. J. , 23 : 317 – 326 .
- Fogg GE 1975 Algal Cultures and Phytoplankton Ecology, University of Wisconsin Press Wiscosin
- Fogg , GE . 1983 . The ecological significance of extracellular products of phytoplankton photosynthesis . Bot. Mar. , 26 : 3 – 14 .
- Freire-Nordi , CS and Vieira , AAH . 1996 . Utilization of extracellular polysaccharides from Ankistrodesmus densus Kors. (Chlorophyceae) by heterotrophic bacteria . Rev. Microbiol. , 27 : 27 – 32 .
- Freire-Nordi , CS and Vieira , AAH . 1998 . Degradability of polysaccharide compounds excreted by Ankistrodesmus densus Kors (Chlorophyceae) in cultures of natural bacterial communities . Verh. Internat. Verein. Limnol. , 26 : 1685 – 1688 .
- Gervais , F . 1997 . Light-dependent growth, dark survival, and glucose uptake by Cryptophytes isolated from a freshwater chemocline . J. Phycol. , 33 : 18 – 25 .
- Giroldo , D and Vieira , AAH . 1999 . Assimilation and release of 14C in a tropical strain of Cryptomonas obovata (Cryptophyceae) exposed to several irradiances . J. Plankton Res. , 21 : 1911 – 1921 .
- Giroldo , D and Vieira , AAH . 2002 . An extracellular sulphated fucose-rich polysaccharide produced by a tropical strain of Cryptomonas obovata (Cryptophyceae) . J. Appl. Phycol. , 14 : 185 – 191 .
- Giroldo , D , Vieira , AAH and Paulsen , BS . 2003 . Relative increase of deoxy sugars during microbial degradation of an extracellular polysaccharide released by a tropical freshwater Thalassiosira sp. (Bacillariophyceae) . J. Phycol. , 39 : 1109 – 1115 .
- Gremm , TJ and Kaplan , TJ . 1997 . Dissolved carbohydrates in stream water determined by HPLC-PAD and pulse amperometric detection . Limnol. Oceanogr. , 42 : 385 – 393 .
- Guillard , RRL and Lorenzen , CJ . 1972 . Yellow-green algae with chlorophyllide c . J. Phycol. , 8 : 10 – 14 .
- Jensen , LM . 1983 . Phytoplankton release of extracellular organic carbon, molecular weight and bacterial assimilation . Mar. Ecol. Prog. Ser. , 11 : 39 – 48 .
- Klaveness D 1985 Ecology of Cryptomonadida: A first review Growth and Reproductive Strategies of Freshwater Phytoplankton (Sandgren, C.D., editor) 105 133 Cambridge University Press New York
- Kim , JB and Carpita , NC . 1992 . Changes in esterifications of the uronic acid groups of cell wall polysaccharides during elongations of maize coleoptiles . Plant Physiol. , 98 : 646 – 653 .
- Lombardi , AT and Vieira , AAH . 1998 . Cooper and lead complexation by high molecular weight compounds produced by Synura sp. (Chrysophyceae) . Phycologia , 37 : 34 – 39 .
- Lovell , CR and Konopka , A . 1985 . Excretion of photosynthetically fixed organic carbon by phytoplankton . Microbial Ecol. , 11 : 3 – 8 .
- Lowry , OH , Rosebrough , NH , Farr , AL and Randal , RJ . 1951 . Protein measurements with the Folin phenol reagent . J. Biol. Chem. , 193 : 265 – 275 .
- Ojala , A . 1993 . Effects of temperature and irradiance on the growth of two freshwater photosynthetic cryptophytes . J. Phycol. , 29 : 278 – 284 .
- Pacobahyba LD 2002 Decomposição de Staurastrum c.f. iversenii Nygaard var americanum: efeitos da qualidade do recurso, da disponibilidade de oxigênio e da temperatura. PhD Thesis Federal University of São Carlos Brazil
- Passow , U , Alldredge , AL and Logan , BE . 1994 . The role of particulate carbohydrate exudates in the flocculation of diatom blooms . Deep-Sea Res. , 41 : 335 – 357 .
- Paulsen , BS , Vieira , AAH and Klaveness , D . 1992 . Structure of extracellular polysaccharides produced by a soil Cryptomonas sp. (Cryptophyceae) . J. Phycol. , 28 : 61 – 63 .
- Porter , KG and Feig , Y . 1980 . The use of DAPI for identification and enumeration of bacteria and blue green algae . Limnol. Oceanogr. , 25 : 943 – 948 .
- Press WH Teukolsky SA Vetterling WT Flannery BP 1993 Numerical Recipes in C: The Art of Scientific Computing, Cambridge University Press New York
- Reinhold , VN . 1972 . Gas-liquid chromatographic analysis of constituent carbohydrates in glycoproteins . Meth. Enzymol. , 25 : 244 – 249 .
- Samuelsen , AB , Paulsen , BS , Wold , JK , Otsuka , H , Yamada , H and Espevik , T . 1995 . Isolation and partial characterization of biologically active polysaccharides from Plantago major L . Phytother. Res. , 9 : 211 – 218 .
- Sciandra , A , Lazzara , L , Claustre , H and Babin , M . 2000 . Responses of growth rate, pigment composition and optical properties of Cryptomonas sp. to light and nitrogen stresses . Mar. Ecol. Progr. Ser. , 201 : 107 – 120 .
- Sims , IM and Bacic , A . 1995 . Extracellular polysaccharides from suspension-cultures of Nicotiana plumbaginifolia . Phytochemistry , 38 : 1397 – 1405 .
- Smith , DJ and Underwood , GJC . 2000 . The production of extracellular carbohydrates by estuarine benthic diatoms: The effects of growth phase and light and dark treatment . J. Phycol. , 36 : 321 – 333 .
- Tundisi , JG and Matsumura-Tundisi , T . 1990 . Limnology and eutrophication of Barra Bonita reservoir, S. Paulo State, Southern Brazil . Arch. Hydrobiol. Beih. , 33 : 661 – 676 .
- Wood , AM and Van Valen , LM . 1990 . Paradox Lost? On the release of energy-rich compounds by phytoplankton . Mar. Microb. Food Webs , 4 : 103 – 116 .