Abstract
In order to obtain new insights on the origin and physiology of the marine chlorophyte Koliella antarctica, the response of the microalga was studied at a salinity of 0.2 in the light and during a 60-day dark period. In light conditions, the alga grows and maintains a functional cell organization. In darkness, the chloroplast-to-chromoplast transition, previously described during dark-acclimation in K. antarctica under a salinity of 34 was only partially triggered; thylakoid lamellae became re-organized into short bundles, but storage substructures were almost completely missing. Microspectrofluorometry, pigment analyses, and morphological observations revealed dark-induced degradation of photosystem II (PSII) with relative stability in the light-harvesting complex (LHCII). Chromatin condensation, mitochondrion fragmentation and material digestion in vacuoles were similar to morphological hallmarks of programmed cell death (PCD), but only 30% of cells underwent cell death and, at the end of the experiment, only 1–2% of cells were TUNEL-positive. Rapid recovery in culture growth after exposure to light showed that the changes apparent in the rest of cells were reversible. Taking into account the response of the plastid and assuming an adaptive benefit of PCD, our results are consistent with the hypothesis that K. antarctica evolved from an Antarctic freshwater ancestor.
Introduction
The green marine microalga Koliella antarctica was isolated during the austral summer 1989–1990 from samples of ice-free seawater collected at a depth of 3 m in Terra Nova Bay, Ross Sea, Antarctica (Andreoli et al ., Citation1991, Citation1998). The alga, recently shown to belong to the Trebouxiophyceae (Andreoli et al ., Citation2000), has been cultured in a liquid seawater medium under controlled conditions at Padua University (Andreoli et al ., Citation1991, Citation1998). The strong environmental pressures in the Antarctic sea have selected interesting adaptive abilities in this organism. For instance, the peculiar composition of the cell wall, formed by a loose network of cellulose micro-fibrils in an extended matrix (Piro et al ., Citation1997, Citation2000), ensures a singular plasticity, which allows K. antarctica to change cell shape under different salinities, from 5 to 70, reflecting acclimation to different situations linked to melting or formation of ice in seawater (Zanetti et al ., Citation2001). Moreover, overall cell structures are well adapted to operate efficiently at low temperatures. The activity of NADH-nitrate reductase (NR) was assayed in crude extracts and also with the purified enzyme from K. antarctica and compared with the mesophilic Chlorella sorokiniana (Vona et al ., Citation2004; Di Martino Rigano et al ., Citation2006). The maximal NADH-dependent activity of NR was found at 15°C in K. antarctica, while it peaked at 25°C in C. sorokiniana. It was also shown that photosynthetic and respiratory rates in K. antarctica are high at temperatures as low as 5°C (Vona et al ., Citation2004). Interestingly, K. antarctica shows elevated respiratory oxygen consumption rates, which suggests that the alga obtains through respiration a large quantity of metabolic energy to power cellular reactions at low temperatures (Vona et al ., Citation2004). An enhanced dissipation of excess light energy as heat in the thylakoid membranes has also been proposed to favour metabolic activities (Giacometti et al ., Citation2001; Baldisserotto et al ., Citation2005a ). Thus, growth under cold conditions appears to be linked to an intrinsically high capacity of cell structures to operate at the low temperatures that characterize the Antarctic sea (Vona et al ., Citation2004; Baldisserotto et al ., Citation2005a ; Lenucci et al ., Citation2006).
Polar marine algae are not only exposed to low temperatures, but also to extremely variable light conditions, including complete darkness for long periods in winter, especially if the ice is covered with snow (Lüder et al ., Citation2002). Survival of marine and freshwater phytoplankton species from various environments to month-long darkness is well documented (Antia & Cheng, Citation1970; Smayda & Mitchell-Innes, Citation1974; Antia, Citation1976; Dehning & Tilzer, Citation1989). In the Southern Ocean, the capacity of microalgae to survive the polar winter determines the level of production after the annual break-up of sea ice (Bunt & Lee, Citation1972; Palmisano & Sullivan, Citation1982, Citation1983). Like other polar algae (Lüder et al ., Citation2002; Bell & Laybourn-Parry, Citation2003; Zhang et al ., Citation2003), K. antarctica exhibits interesting acclimation features to the stress of complete darkness, as has been shown in a laboratory experiment in which an austral night condition (90 days) was simulated (Baldisserotto et al ., Citation2005a ). What deserves particular attention is the dark-triggered transition of the chloroplast to a plastid type that combines the characteristics of chloroplast and chromoplast in a structure that is surely distinctive among algae. A combination of degradative processes and assembly modulations of photosystem II (PSII) chlorophyll-protein complexes occurs through a precise sequence of events, which have been reconstructed based on fluorescence emission spectra (Baldisserotto et al ., Citation2005a ). The molecular reorganization of the photosynthetic membranes is accompanied by a modification of the thylakoid architecture, from lamellae to short bundles (Baldisserotto et al ., Citation2005a ).
On the whole, information available on morphological, functional, and biochemical aspects of K. antarctica indicates that this organism is well-adapted to living in the Antarctic marine environment. This is even more interesting because Koliella is typically a genus of freshwater microalgae (Katana et al ., Citation2001). The existence of a member of the genus in the Antarctic sea has opened a debate about the origin of this organism. Andreoli et al . (Citation2000) proposed that K. antarctica could have evolved in freshwater habitats and subsequently been introduced into the Antarctic sea ecosystem. In fact, the most typical spindle-shaped cell morphology of the genus is observed in K. antarctica at a salinity of 5; curled cells with rounded extremities are observed at typical seawater salinities (Andreoli et al ., Citation1998, Citation2000; Zanetti et al ., Citation2001). Thus, the adaptive traits to the marine environment may have developed over the ancestral freshwater traits, whose traces have not completely disappeared, but are somehow ‘hidden’ in the organism K. antarctica. While the freshwater origin of K. antarctica can be convincingly supported, there is still doubt about whether its ancestor came from temperate regions, according to a hypothesis suggested by Andreoli et al . (Citation2000), or from freshwater bodies in Antarctica.
In this research, we aimed to test the hypothesis that K. antarctica originated from freshwater in the Antarctic. Specific objectives of this study were:
-
To test the ability of the alga to survive a simulated polar night in freshwater.
-
To verify specific responses of the chloroplast, in particular the transition to chloro-chromoplast, which was previously described as a characteristic phenomenon during dark-acclimation in K. antarctica at a salinity of 34 (Baldisserotto et al ., Citation2005a ).
Therefore, the alga has been cultured at extremely low salinity (0.2) in the light and then in long-lasting darkness (60 days). This combination of parameters is clearly artificial by comparison with the natural environment of K. antarctica. However, it could be representative of an Antarctic freshwater body, where microalgae are exposed to prolonged darkness along with low salinity (Bell & Laybourn-Parry, Citation2003).
Materials and methods
Culture conditions
Koliella antarctica SAG 2030 (Chlorophyta, Trebouxiophyceae) was kindly provided by Prof Carlo Andreoli, Department of Biology, University of Padova, Italy (Andreoli et al ., Citation1998). The organism was maintained in axenic culture in seawater enriched with F/2 medium (Guillard, Citation1975) under conditions similar to those of its origin: continuous light (10 µmol photons m−2 s−1), 5 ± 1°C temperature, salinity 34, no shaking (Andreoli et al ., Citation1998, Citation2000; Baldisserotto et al ., Citation2005a ).
The alga was then inoculated into six 200-ml Erlenmeyer flasks containing a medium with a salinity of 0.2 and the following composition: 2 mM NaNO3, 0.1 mM NaH2PO4, 1 µM ZnCl2, 1 µM MnCl2, 1 µM Na2MoO4, 0.1 µM CoCl3, 0.1 µM CuSO4, 20 µM Fe(III) citrate, 26.4 µM Na2EDTA, 35 µg l−1 thiamine, 50 µg l−1 biotin (Fabregas et al ., Citation1984). The initial cell density was about 8 × 106 cells ml−1. The other culture conditions were unchanged. After 10 days in the new medium, three cultures were placed in complete darkness for 60 days by wrapping the flasks with multiple layers of aluminium foil, while the other three continued in light. Cell number was counted with a Thoma's haemocytometer periodically throughout the experiment for light- and dark-maintained cultures. Aliquots of cell suspensions were sampled daily from 0 (light conditions) to 4 days of darkness, and then after 7, 15, 21, 30, 35, 40, 50 and 60 days. Parallel analyses were performed on the light-grown cultures. At the end of the experiment, the cultures maintained in darkness were again exposed to the light and the growth was monitored.
All manipulations of samples from darkened cultures were performed under a dim green safe-light (15 W green Philips globe, <0.1 µmol m−2 s−1; Stürzl & Schiff, Citation1986; Baldisserotto et al ., Citation2005a ).
Light and fluorescence microscopy
For in vivo microscopic observations, a Zeiss model Axiophot microscope equipped with conventional or fluorescent attachments was employed. The light source for fluorescence examinations was a HBO 100 W pressure mercury vapour lamp. Chloroplasts were visualized by chlorophyll (Chl) autofluorescence with excitation at 436 nm (filter set, BP436/10, LP470).
Mitochondria were visualized using rhodamine 123 (Rh123; Sigma Chemicals, St Louis, Mo), a rapid and selective fluorochrome for vital staining of mitochondria (Vannini et al ., Citation1988). Rh123 was prepared as an aqueous 1 mg ml−1 solution and diluted in the culture medium to a final concentration of 10 µg ml−1. After incubation for 20 min at 4°C in darkness, cell samples were rinsed in dye-free culture medium. The cells, collected by centrifugation, were observed with excitation at 436 nm (filter set, BP436/10, LP470).
Staining of DNA in nuclei was performed with 4′,6-diamino-2-phenilindole (DAPI; SERVA, Heidelberg, Germany), a fluorochrome that binds to the minor groove of double stranded DNA. DAPI was prepared and used as described for Rh123. After rinsing, the cells were collected by centrifugation and observed with excitation at 365 nm (filter set, BP365/12, LP397).
Transmission electron microscopy
Cells were fixed with glutaraldehyde and postfixed with OsO4 in cacodylate buffer as described in Baldisserotto et al . (Citation2005a ). Embedding and staining procedures for transmission electron microscopy (TEM) were routinely performed as described in previous reports (Fasulo et al ., Citation1983; Pancaldi et al ., Citation2002). Samples were observed with a Hitachi H800 electron microscope (Electron Microscopy Centre, Ferrara University, Italy). Ultrastructural details were observed in at least 30 cells from different samples and grids. Representative aspects were photographed.
Photosynthetic pigment analyses
Algal cell suspensions were pelletted by centrifugation at 500g for 5 min and extracted for 2 h at −20°C with aqueous 85% methanol containing 1.5 mM sodium hydrosulphite (Carlo Erba, Milano, Italy). The latter compound is thought to cleave disulphide bridges to relax cell-wall proteins and render the cells permeable to methanol (Porra Citation1990, Citation2002). Absorption of methanol extracts was measured at 665 nm (Chl a), 652 nm (Chl b) and 470 nm (carotenoids) with a Pharmacia Ultrospec spectrophotometer. The concentrations of Chl a and Chl b were determined using the equations of Porra (Citation2002). Total carotenoids were evaluated according to Wellburn (Citation1994).
Microspectrofluorometry
Room temperature microspectrofluorometric analyses were performed to study the assembly state of the Chl-protein complexes of PSII in darkened K. antarctica by comparison with the light-grown cells (Pancaldi et al ., Citation2002; Baldisserotto et al ., Citation2005a ). Data acquisition and processing was substantially as in Pancaldi et al . (Citation2002), with some modifications. Fluorescence emission spectra were recorded using a microspectrofluorometer (RCS, Firenze, Italy), combined with a Zeiss Axiophot photomicroscope. For analyses, cells were mounted on pre-cooled (4°C) poly-lysine microslides (Menzel-Gläzer, Braunschweig, Germany) and all spectra were recorded on single living cells viewed under fluorescent light. The excitation light (436 nm) was focussed on a single cell at a time. Only viable cells, which were easily recognized due to their red fluorescence, were analysed. Autolab software (RCS, Firenze, Italy) set the recording range (620–750 nm) and optimized the photomultiplier response. At least three spectra were recorded for each culture.
Spectra were processed with Microcal Origin 6.0 software as previously reported with some modifications (Pancaldi et al ., Citation2002; Baldisserotto et al ., Citation2005a ). Average spectra, smoothed with a Fast Fourier Transform filter function, were deconvoluted into Gaussian components after fourth derivative analysis, taking into account the limitations of the method (Böddi & Franck, Citation1997).
Fluorescence emission bands were interpreted according to previous work on K. antarctica (Baldisserotto et al ., Citation2005a ) and other organisms (Pancaldi et al ., Citation2002; Baldisserotto et al ., Citation2004, Citation2005b ; Ferroni et al ., Citation2004). The attribution of bands is reported in . The contributions of the emission components of interest (PSII reaction centre, RCII; inner antennae of PSII, CP43-47; light harvesting complex II, LHCII) were evaluated as the areas subtended under the corresponding Gaussian curves. CP43–47 is usually the main emission source at room temperature, when proper energy transfer processes occur. Since individual emissions from CP43 (around 685 nm) and CP47 (around 695 nm) are not always resolved, they are discussed jointly in this paper as CP43–47 (Baldisserotto et al ., Citation2005a ). Events preventing energy transfer to RCII increase the relative emission from RCII and/or LHCII (Vassiliev et al ., Citation1995; Pancaldi et al ., Citation2002). Thus, the degree of assembly of the main Chl-protein complexes of PSII can be evaluated from the RCII/CP43–47 and LHCII/PSII fluorescence emission ratios (where PSII is the sum of RCII and CP43–47). RCII/CP43–47 is an index of assembly of the PSII core complex; LHCII/PSII is an index of the assembly state of the peripheral antenna with the PSII core. Even subtle changes can be highlighted by variations in these fluorescence emission ratios (Pancaldi et al ., Citation2002; Baldisserotto et al ., Citation2004, Citation2005a , Citation2005b ; Ferroni et al ., Citation2004).
Table 1. Attribution of fluorescence emission bands
TUNEL staining
Fragmentation of nDNA was detected in situ by TUNEL labelling of K. antarctica cells cultured in darkness for 60 days (Gavrieli et al ., Citation1992). Cell samples were collected by centrifugation (7 min at 500g) and fixed with 10% formaldehyde in 0.1 M Na-K-phosphate buffer (pH 7.2) at 4°C overnight. The pellets were then rinsed with phosphate buffer and treated with a graded series of methanol prepared in phosphate buffer: 50%, 85% with addition of 1.5 mM Na-hydrosulphite, 50%, 30%. Finally cells were rinsed with phosphate buffer and harvested by centrifugation. Na-hydrosulphite was used to loosen cell wall structure for the labelling reactions.
Pelletted cells were mounted onto poly-lysine-coated microslides, which had just been covered with a thin film of 1% agar. Cells, immobilized by being embedded in the agar matrix, were then made more permeable with 0.1% Triton X-100 in phosphate-buffered saline (PBS; 10 min), washed with PBS, and labelled with the TUNEL mixture according to the manufacturer's instructions (Boehringer Mannheim, Germany). After overnight incubation in a humid chamber at 37°C and rinsing with PBS, cells were counter-stained with Tripan blue and Hoechst 33258 (0.1 µg ml−1 in PBS for 10 min). The samples were then mounted upside-down on a non-fluorescent glass slide in a drop of Mowiol (Calbiochem, Inalco S.p.A., Italy). Fluorescence due to fluorescein-isothiocyanate (emission at 525 nm) was observed with excitation at 488 nm (Soldani et al ., Citation2001, Citation2005).
Results
Growth
Koliella antarctica cultured in the light at a salinity of 0.2 grew exponentially with a doubling time of about 25 days. When the cultures were transferred to darkness, cell density slowly increased up to 30 days with an estimated doubling time of about 75 days (linear regression, p < 0.001; ). Subsequently, cell density decreased from about 15 to 10 × 106 cells ml−1 after 50–60 days. The dark-maintained cultures were then exposed to the light again: after a lag-phase of 3 days, cell density rapidly increased (doubling time, 11 days). After 21 days in light, the culture density reached a plateau at approx. 30 × 106 cells ml−1 ().
Fig. 1. Growth kinetics of Koliella antarctica in a salinity of 0.2. Open circles: light-grown cells; closed circles: cells grown for 10 days in light, transferred to complete darkness for 60 days, and re-exposed to light for 30 days. Error bars indicate standard deviations (n = 3 cultures).
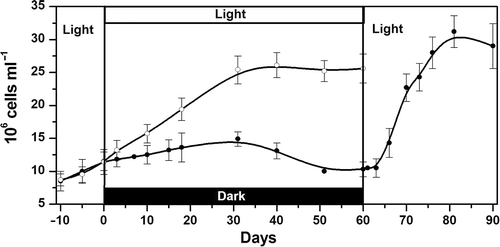
We have focussed our attention on the changes occurring in the cells during the 60 days of dark cultivation. In parallel, the maintenance of the morphological and analytical aspects of the light-cultured cells was monitored (data not shown).
Cell morphology
Koliella antarctica cultured in the light at low salinity had spindle-shaped cells with rounded extremities (). The cells were generally 10 µm in length and 3–4 µm in diameter and were mostly organized in isolated pairs or short filaments. The chloroplast occupied most of the cell volume and emitted bright red fluorescence under 436 nm excitation. Close to the chloroplast, the cells generally contained only one elongated mitochondrion, which emitted a green fluorescence after uptake of Rh123 (). Sub-microscopic observation of cell structures showed the endoplasmic reticulum, the Golgi apparatus, several ribosomes and one or two mitochondria with elongated, thin cristae protruding into the matrix (). The nucleus contained dispersed chromatin and an easily distinguishable nucleolus (). The reaction of DNA with DAPI yielded a diffuse light-blue staining of the nucleus (). The chloroplast of K. antarctica lacked a pyrenoid, and the inner membranous system was organized into 2–3 thylakoid lamellae, regularly arranged along the longitudinal axis of the organelle, without grana stacking (, ). Some starch grains were found in the stroma ().
Figs. 2–9. Light and fluorescence micrographs of Koliella antarctica cells cultured in continuous light or in complete darkness for 60 days. . Spindle-shaped light grown cells, mostly organized in pairs. . Green-fluorescent elongated mitochondrion after Rh123 staining and red-fluorescent chloroplast in light-grown cells (excitation = 436 nm). . Uniform light-blue DAPI staining of the nucleus in light-grown cells (excitation = 365 nm). . Cells cultured in darkness for 60 days. . After 60 days darkness, Rh123-stained mitochondria show three different typologies. . After 60 days darkness, DAPI shows chromatin condensed in a ring. . TUNEL-test indicates DNA-fragmentation only in 1–2% of cell population: only one positive reaction (picture on the right), visualized by green fluorescence of FITC, is detected in the field (arrow). . Hoechst DNA counterstaining performed on the same cells as in shows the chromatin condensed in a ring.
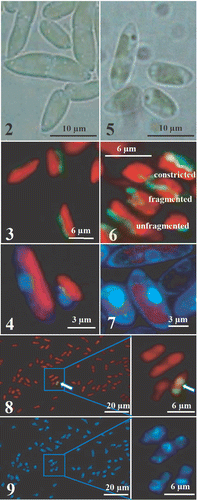
Figs. 10–13. TEM images of light-grown cells of Koliella antarctica. . Section of a cell showing the parietal chloroplast (C), two mitochondria (M) with thin cristae, a large Golgi apparatus (G), tubules of the endoplasmic reticulum (arrow), and several ribosomes spread in the cytoplasm. . Detail of the nucleus, characterized by nucleolus (Nu) and dispersed chromatin. . Detail of the chloroplast showing lamellae formed by three thylakoids. . Detail showing some starch grains (S) in the chloroplast.
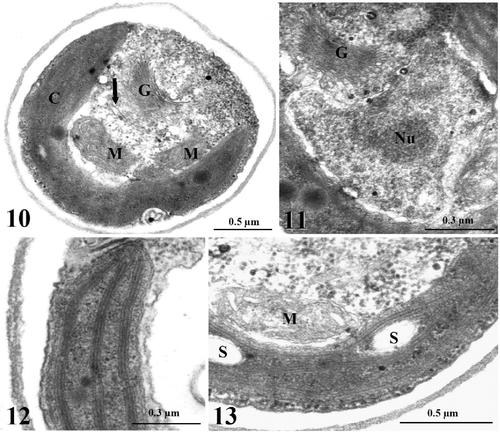
Cultivation of cells in darkness caused a wide range of ultrastructural alterations. After 4 days in darkness, the thylakoid system, although still organized into lamellae, was looser () and some stroma regions were free from membranes, or occupied by osmiophilic plastoglobules (). In some cells, an increased number of mitochondria was observed (up to 4), and mitochondrial cristae were often swollen (). Autophagic phenomena were shown by the increased vacuolization in the cytoplasm (). The plasma membrane tended to form convoluted invaginations () and, concomitantly, membranous inclusions appeared in some vacuoles (). The ultrastructure of the nucleus was unaffected (). After 15 days of darkness, the thylakoid lamellae were re-organized into short bundles of tightly stacked thylakoids without regular orientation (). Some concentrically arranged single thylakoids were observed in the central portion of the plastid (). In the extra-plastid compartments, the modifications described above were more pronounced, with dark inclusions in autophagic vacuoles (). In the nucleus of some cells (c. 30%), a portion of the chromatin was condensed in a semi-continuous layer close to the envelope, while the nucleolus was unaffected (). After 21 days, up to five mitochondria and profound invaginations of the cell membrane were observed (, ). In the chloroplast, the thylakoid bundles frequently showed a tendency to curl, leaving large stroma regions free from membranes (). Chromatin condensation was evident in many cells (). After 30 days in darkness, single straight thylakoids often originated from the bundles, while the girdle thylakoids had disappeared (). Outside the chloroplast, autophagic phenomena were more and more evident (). Apart from a few cells with a regularly formed nucleus, many cells had alterations in the distribution of chromatin. Condensation of chromatin was found not only as a thin layer, but also in the shape of voluminous patches (, ). Where condensation was more pronounced, the nucleolus had lost its rounded shape, showing incipient dissolution of the structure (). The structure of thylakoid bundles was still maintained after 40 days in darkness, in spite of the visibly reduced thylakoid system and the presence of single straight thylakoids (). Some stroma regions had a pale granular appearance, especially in the central portion of the plastid (). Finally, in several cells, patches of condensed chromatin accumulated close to the nuclear envelope and the nucleolus was clearly dissolving (). While the ultrastructure of the chloroplast did not substantially change up to the end of the experiment (60 days), the alterations in the extra-plastid compartments were further emphasized. Voluminous dark globules of digested materials were present inside large vacuoles (). The highest degree of chromatin condensation was reached and the nucleolus had often disappeared (). DAPI staining of cells showed the chromatin condensed in a ring close to the envelope (). Rh123 revealed several different conformations of the mitochondrial apparatus inside the population of cells, based on the fragmentation of the organelle: (a) one elongated mitochondrion; (b) mitochondrion affected by several constrictions; (c) mitochondrion fragmented into individual rounded entities (). Inside the organelle, swelling of cristae was frequent (). A degenerating cell is shown in .
Figs. 14–19. TEM images of Koliella antarctica cells maintained in complete darkness for 4 days. . Section of a cell with a chloroplast (C) characterized by a loose thylakoid system. Detail of the thylakoid lamellae in the insert. M, mitochondrion. . Stroma region free from membranes and occupied by osmiophilic plastoglobules (asterisks) in a plastid. . Detail of a cell with four mitochondria (arrows). Detail of convoluted invaginations from the plasma membrane. . Membranous inclusion (arrow) inside a vacuole. . Normally formed nucleus, with a large nucleolus (Nu) and dispersed chromatin.
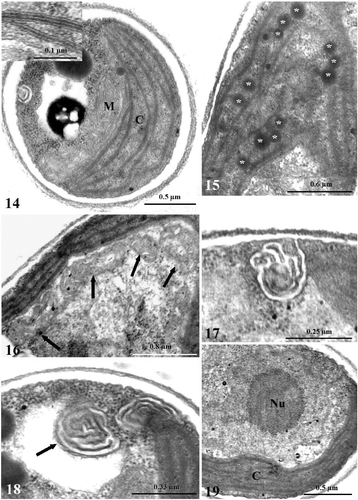
Figs. 20–26. TEM images of Koliella antarctica cells maintained in complete darkness for 15 or 21 days. . Cells cultured in dark for 15 days. . Chloroplast (C) with the thylakoid membranes organized in irregularly oriented short bundles. M, mitochondrion. . Detail of central portion of a plastid with two concentrically arranged thylakoids (arrow). . Cell with an autophagic vacuole (V), convoluted plasma membrane invaginations (arrow), and altered chloroplast. . Nucleus with semi-continuous layer of condensed chromatin close to the envelope, and normally formed nucleolus (Nu). . Cells cultured in darkness for 21 days. . Convoluted plasma membrane invaginations (arrow); in the chloroplast, curled thylakoid bundles and stroma regions free from membranes are visible. . Portion of cell with five mitochondria (arrows). . Nucleus showing evident chromatin condensation close to the envelope, and a large nucleolus.
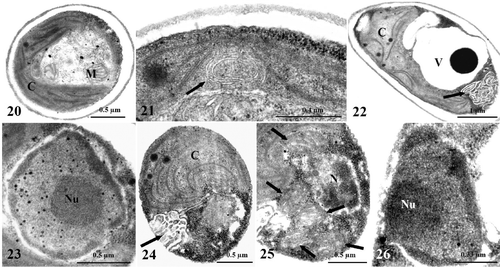
Figs. 27–32. TEM images of Koliella antarctica cells maintained in complete darkness for 30 or 40 days. . Cells cultured in dark for 30 days. . Detail of plastid showing single straight thylakoids, originating from bundles of membranes. . Cell showing autophagic phenomena at the cytoplasmic level, altered plastid (C), and strong chromatin condensation in the nucleus (N). . Cell with condensed chromatin in the shape of voluminous patches in the nucleus. Nu, nucleolus. . Dissolving nucleolus in a cell. , . Cells cultured in dark for 40 days. . Plastid showing reduced membrane system with appressed (dark arrows) and single straight (white arrow) thylakoids; degeneration of stroma has occurred in central portion of organelle. . Nucleus with large patches of condensed chromatin close to the envelope, and dissolving nucleolus.
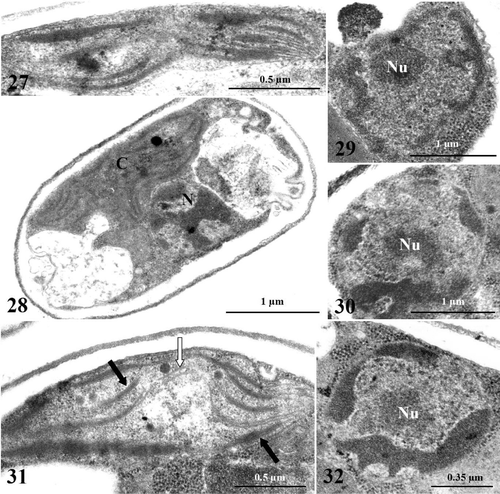
Figs. 33–36. TEM images of Koliella antarctica cells maintained in complete darkness for 60 days. . Overall aspect of cells showing general alteration; large dark globules are deposited inside vacuoles (arrows). . Nucleus lacks the nucleolus; chromatin is condensed in a ring close to the envelope. . Mitochondrion with swollen cristae (arrows). . Overall appearance of degenerating cell. C, chloroplast; M, mitochondrion; N, nucleus; V, vacuole.
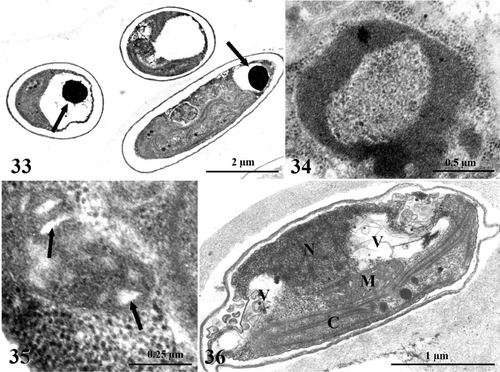
Since the morphological alterations in the nucleus, cytoplasm and mitochondria recalled some markers of programmed cell death (PCD), we investigated the occurrence of DNA-fragmentation in K. antarctica after 60 days of dark-cultivation. A TUNEL-test positive reaction was found in only 1–2% of the cells (), although counter-staining with Hoechst showed that most of the cells exhibited chromatin condensation ().
Photosynthetic pigments
The molar ratio of Chl a:b in K. antarctica grown in the light was 3.9 (). At the end of the first day of darkness, the pigment content, mainly Chl a, had decreased markedly, and the Chl a:b ratio was about 3.5. No significant change occurred during the next 3 days of dark-cultivation, but the Chl a:b ratio was restored to the initial value after 7 days. A 44% decrease in Chl a occurred between 21 and 30 days in darkness, which, since it was not accompanied by changes in Chl b, resulted in a strong decrease in the Chl a:b ratio. Little further change in Chl content was observed between 30 and 60 days in darkness.
Fig. 37. Photosynthetic pigment concentrations (above) and their ratios (below) in Koliella antarctica cells during 60 days in darkness, determined by spectrophotometry of methanol extracts. Values are means with standard deviations (n = 3).
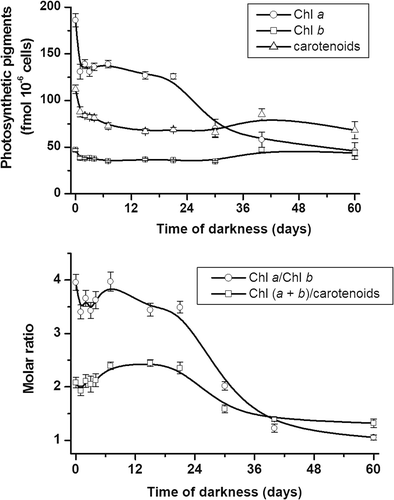
The carotenoid content was also affected by cultivation in darkness (). During the first 4 days, carotenoids decreased in parallel with Chl. From 4 to 7 days, they continued to decrease and this resulted in an increase in the ratio of Chl a + b to carotenoids. The subsequent alterations in the ratio were mainly dependent on the changes in Chl content.
Microspectrofluorometric responses
Because of the rapid decay of Chl fluorescence, it was difficult to record the microspectrofluorometric responses in K. antarctica cultured in the light at low salinity. Consequently, the spectra were obtained as a collage of six short spectra of 20 nm, recorded from six cells and joined together to cover the 640–750 nm range (). Gaussian deconvolution of the reconstructed spectra highlighted the main fluorescence emissions; attribution of bands was based on the literature (). On day 0, the fluorescence emission ratios were 0.47 for RCII/CP43–47 and 0.17 for LHCII/PSII (). These ratios did not change significantly in light-grown cells over the period of 60 days (not shown).
Fig. 38. Microspectrofluorometric responses of Koliella antarctica cells grown in light (upper panel) or in complete darkness for 60 days (lower panel). Fluorescence emission spectra were recorded in vivo on single living cells (excitation light, 436 nm). Reported spectra are means of nine recordings, made on three cells for each of three separate experiments (standard deviations at fixed wavelengths are shown). Gaussian components of special interest are marked: RCII (black); CP43–47 (hatched); LHCII (arrows); difference between Gaussian sum and original data (not reported) was always negligible (r 2 ≥ 0.999). Middle panel: changes in relative contribution of RCII, CP43–47, and LHCII to total emission during experiment in dark. Values are means of three replicate cultures (for each culture, at least three spectra were recorded and averaged); error bars indicate standard deviations.
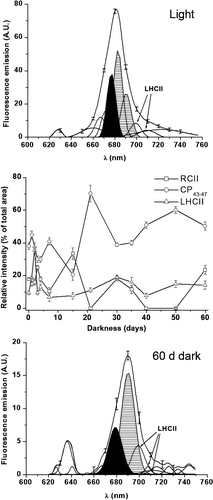
Fig. 39. Change of RCII/CP43–47 (above) and LHCII/PSII (below) fluorescence emission ratios (calculated from microspectrofluorometric analyses, ) with length of culture in darkness. Both ratios have been calculated from areas under Gaussian curves with maxima at 677–680 nm (RCII), 683–696 nm (CP43–47), 700–715 nm (LHCII). PSII is sum of RCII and CP43–47. Error bars indicate standard deviations (n = 3).
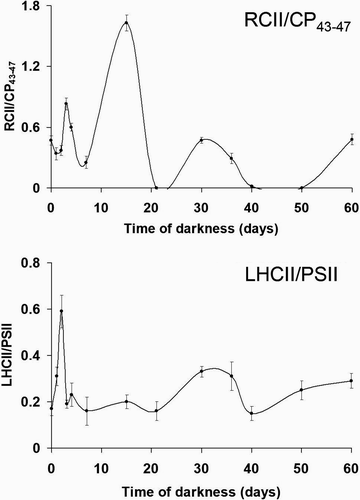
Acquisition of spectra of dark-cultured samples became progressively easier, and continuous spectra were recorded from the third day. Darkness strongly affected the relative intensity of the fluorescence bands (). After a slight decrease during the first day, the RCII/CP43–47 ratio showed three peaks after 3, 15 and 30 days of darkness (). The peaks after 3 and 30 days corresponded with the two main phases in pigment degradation (), and were also matched by peaks in the LHCII/PSII ratio (). After 40 days, both ratios showed an increasing trend up to the end of the experiment.
Discussion
In recent years, extensive studies have been performed to gain information on the morphology, physiology and biochemistry of the green microalga K. antarctica (Andreoli et al ., Citation1991, Citation1998, Citation2000; Piro et al ., Citation1997, Citation2000; Giacometti et al ., Citation2001; Zanetti et al ., Citation2001; Vona et al ., Citation2004; Baldisserotto et al ., Citation2005a ; Lenucci et al ., Citation2006; Di Martino Rigano et al ., Citation2006). The constancy of the strain is ensured by parallel surveys performed in different laboratories in Italy, including those of the Department of Biology at Padua University, where the organism had been isolated and classified and has been maintained in stable liquid culture (Andreoli et al ., Citation1998). Andreoli et al . (Citation2000) suggested that the ability of K. antarctica to grow in low-salinity media (5) points to the freshwater origin of this marine microalga. This is confirmed in the present study, where the alga has been cultured at a salinity of 0.2, which is outside the salinity range in the Antarctic sea (Zanetti et al ., Citation2001). However, the doubling time of 25 days is considerably higher than in seawater media (c. 10 days; Baldisserotto et al ., Citation2005a ) and indicates that low salinity is not fully congenial to the alga, as is also indicated by the absence of the large lipid droplets that fill most of the cytoplasm when the alga is cultured in seawater (Andreoli et al ., Citation2000; Baldisserotto et al ., Citation2005a ). Nevertheless, the morphology of the cell organelles appears to be unaffected (Baldisserotto et al ., Citation2005a ). Rh123 retention in the mitochondrion indicates that the voltage gradient across the inner membrane is preserved (Vannini et al ., Citation1988). In the chloroplast, despite the modified stoichiometry in the photosynthetic pigments (Chl a:b ratio of 3.9 v. 1.4; Baldisserotto et al ., Citation2005a ), the arrangement of the thylakoid membranes still allows dissipation of excess light energy as heat (with rapid decay of Chl fluorescence), as in high salinity (Giacometti et al ., Citation2001; Baldisserotto et al ., Citation2005a ). This could depend on the adjustment of the Chl-protein complexes, which in turn affect the fluorescence emission ratios. In particular, the LHCII/PSII ratio (0.17 v. 0.45 in seawater; Baldisserotto et al ., Citation2005a ) indicates a different organization of the peripheral antennae in the two salinities. In the light of these considerations, the hypothesis of the freshwater origin of K. antarctica appears to be well-grounded and we exposed the alga to prolonged darkness to test whether it derived from temperate or polar regions. Month-long darkness is distinctive of polar regions and polar algae, K. antartica included, have developed special adaptive traits of the plastid to survive it (Lüder et al ., Citation2002; Baldisserotto et al ., Citation2005a ).
In K. antarctica, low salinity negatively affects the response of the chloroplast to darkness compared with our observations on the same alga grown in darkness in a seawater medium (Baldisserotto et al ., Citation2005a ). Accumulation of materials within storage sub-structures, chiefly carotenoid-bearing membranes and globules, is almost completely missing, so that the chloroplast-to-chromoplast transition described by Baldisserotto et al . (Citation2005a ) does not occur. The plastoglobules transiently occurring during the first days in darkness cannot be involved in storage, but are merely linked to membrane degradation (Bonora et al ., Citation2000; Baldisserotto et al ., Citation2005a ). Nevertheless, the reorganization of the thylakoid architecture from lamellae to short bundles is largely comparable with that observed in a salinity of 34 (Baldisserotto et al ., Citation2005a ). High values of RCII/CP43–47 during periods of pigment decline (, ) indicate that the energy transfer within the PSII core is impaired due to degradation of PSII Chl-protein complexes (Pancaldi et al ., Citation2002; Baldisserotto et al ., Citation2005b ). Conversely, the peak recorded after 15 days is not linked to major degradation of complexes, since none is shown in the pigment analyses, but may reflect the reorganization of PSII leading to the changes in thylakoid architecture. Compared with our observations in a seawater medium, the RCII/CP43–47 kinetics is considerably delayed, with peaks at days 3, 15 and 30, instead of 1, 3 and 21 (Baldisserotto et al ., Citation2005a ). Consequently, the formation of the thylakoid bundles is completed in 15 days instead of only 3 (Baldisserotto et al ., Citation2005a ).
The variations in the organization of the PSII core are accompanied by altered relations between PSII and LHCII. During the first 2 days, the increase in LHCII/PSII is due to the intense fluorescence emitted from LHCII, which is unable to transfer excitation to PSII. This results from an imbalance between PSII and LHCII and is linked to PSII degradation. A similar effect was described by Vassiliev et al . (Citation1995) in iron-starved Dunaliella tertiolecta and by Ferroni et al . (Citation2004) in manganese-treated Euglena gracilis. The subsequent decrease in the ratio indicates the recovery of the association between PSII and LHCII (Pancaldi et al ., Citation2002). A similar trend is observed around 30 days of dark-cultivation. On the whole, fluorometric and pigment analyses show that LHCII is more stable than the PSII core. The peripheral antenna of PSII probably plays a structural role in promoting, or stabilizing, thylakoid appression (McDonnel & Staehelin, Citation1980; Simidjiev et al ., Citation1998; Prakash et al ., Citation2001). The high stability of LHCII in dark-cultured K. antarctica could be beneficial since it will enhance light harvesting after the polar night (Baldisserotto et al ., Citation2005a ; Morgan-Kiss et al ., Citation2006). Thus, alteration in thylakoid architecture does not result from an unregulated degeneration, but presumably reflects an imperfect activation of the ‘acclimation to darkness’ programme we had previously described in K. antarctica (Baldisserotto et al ., Citation2005a ). The occurrence of single thylakoids, which are absent at high salinity in light-grown (Andreoli et al ., Citation1998) and in dark-acclimated K. antarctica (Baldisserotto et al ., Citation2005a ), could be symptomatic of an unstable condition. This is shown also by the increasing fluorescence emission ratios after 50 days in darkness (Baldisserotto et al ., Citation2005b ). The decrease of the RCII/CP43–47 ratio to zero does not necessarily imply that RCII is completely absent, because the association of complexes may allow very efficient back-transfer of excitation energy from RCII to the inner antennae (Pancaldi et al ., Citation2002). Recovery from apparent zero-values is possible when this efficiency is reduced.
In low salinity, the response to darkness is not restricted to the chloroplast, but involves strong alterations in other compartments, which closely recall morphological hallmarks of PCD: chromatin condensation around the margins of the nucleus, mitochondrion fragmentation, swelling of mitochondrial cristae, autophagy (Arnoult et al ., Citation2002; Bossy-Wetzel et al ., Citation2003; Ludovico et al ., Citation2003; Segovia et al ., Citation2003; Deponte & Becker, Citation2004; Franklin & Berges, Citation2004; Geitmann et al ., Citation2004). There is rapidly growing evidence that PCD may occur in unicellular organisms, for instance in protozoan parasites and dinoflagellates (Deponte & Becker, Citation2004; Franklin & Berges, Citation2004). Up to now, PCD in unicellular chlorophytes has been shown only in Dunaliella tertiolecta and Chlamydomonas reinhardtii (Segovia et al ., Citation2003; Moharikar et al ., Citation2006). Koliella antarctica cannot be considered truly unicellular because of its tendency to form short filaments, which however do not have physiological connections and often fall into solitary cells shortly after division (Hindák, Citation1996; Stibal & Elster, Citation2005). Nonetheless, the colonial existence of K. antarctica, albeit temporary, is more reason for the induction of PCD under environmental stress as the cells may have some ‘awareness’ of their conspecifics.
In K. antarctica, nuclear and mitochondrial morphology strongly recall apoptosis (Segovia et al ., Citation2003), but only a few cells were TUNEL-positive at the end of the experiment. A fundamental role seems to be played by autophagy, but digestion of cell materials occurs gradually inside vacuoles, which is different to the autolytic PCD pathway found in higher plant cells, where the collapse of the vacuole is an early event leading to protoplast (and even cell wall) hydrolysis (Jones, Citation2001; Gunawardena et al ., Citation2004). Thus, the cells are slowly dying in a starvation situation, but DNA fragmentation and vacuole collapse, rapidly leading to cell dissolution, occur only in some cells. The rapid growth recovery after light exposure can be attributed to the recovery of metabolic activity in cells in which DNA-fragmentation has not yet occurred. Whereas chloroplast destabilization in higher plants can be an early and causal event in PCD, in all probability linked to the light-induced generation of reactive oxygen species (Gray et al ., Citation2002; Samuilov et al ., Citation2002; Chen & Dickman, Citation2004), a PCD-promoting role of the chloroplast is clearly excluded in our dark-acclimation experiment. Conversely, the non-degenerated, dark-acclimated plastid allows prompt reactivation of photosynthesis in the light.
Our results allow us to propose that the ancestor of K. antarctica could have been an organism already adapted to Antarctic freshwater. In fact, the scenario triggered by darkness in low salinity could be the result of the selective pressure in an Antarctic freshwater sub-environment, where the ancestor of K. antarctica would have survived the austral night by means of slowed metabolism, accompanied by acclimation of the plastid system. Could dark-induced PCD have had an adaptive benefit? It has been proposed that, in unicellular organisms, some individuals could ‘sacrifice’ themselves for the survival of the population, thus ensuring the continuity of the species with an altruistic behaviour (Fröhlich & Madeo, Citation2000; Franklin et al ., Citation2006). In the Antarctic sea, the altruistic PCD may have become unnecessary, perhaps due to higher richness in nutrients. According to this interpretation, it appears that some ancestral processes, which are usually silent in K. antarctica, but can be re-activated, represent effective strategies for species maintenance. In particular, its ability to survive stressful conditions is above all related to the plasticity of the plastid, which has evolved as a result of the strong environmental pressures in Antarctica.
Acknowledgements
This research work was supported by grants from Italian MIUR (Ministero per l'Istruzione, l'Università e la Ricerca) PRIN2003 and FIRB2001 (Fondo per gli Investimenti della Ricerca di Base). We are grateful to Prof C. Andreoli (University of Padova) for the kind gift of the organisms used in this research. We also thank two anonymous referees and the Associate Editor Prof J. Raven for their highly appreciated suggestions.
References
- Alfonso , M , Montoya , G , Cases , R , Rodriguez , R and Picorel , R . 1994 . Core antenna complex, CP43 and CP47, of higher plant photosystem II. Spectral properties, pigment stoichiometry, and amino acid composition . Biochemistry , 33 : 10494 – 10500 .
- Andreoli , C , Spini , S , Grassi , C and Scarabel , L . 1991 . A preliminary survey on a green microalga of the Antarctic Sea . Giorn. Bot. Ital. , 126 : 959 – 960 .
- Andreoli , C , Lokhorst , GM , Mani , AM , Scarabel , L , Moro , I , La Rocca , N and Tognetto , L . 1998 . Koliella antarctica sp. nov. (Klebsormidiales) a new marine green microalga from the Ross Sea (Antarctica) . Algolog. Stud. , 90 : 1 – 8 .
- Andreoli , C , Moro , I , La Rocca , N , Dalla Valle , L , Masiero , L , Rascio , N and Dalla Vecchia , F . 2000 . Ecological, physiological, and biomolecular surveys on microalgae from Ross Sea (Antarctica) . Ital. J. Zool. , 67 ( suppl ) : 147 – 156 .
- Antia , NJ . 1976 . Effects of temperature on the darkness survival of marine microplanktonic algae . Microb. Ecol. , 3 : 41 – 54 .
- Antia , NJ and Cheng , JY . 1970 . The survival of axenic cultures of marine planktonic algae from prolonged exposure to darkness at 20°C . Phycologia , 9 : 179 – 183 .
- Arnoult , D , Akarid , K , Grodet , A , Petit , PX , Estaquier , J and Ameisen , JC . 2002 . On the evolution of programmed cell death: apoptosis of the unicellular eukaryote Leishmania major involves cysteine proteinase activation and mitochondrion permeabilization . Cell Death Diff. , 9 : 65 – 81 .
- Baldisserotto , C , Ferroni , L , Medici , V , Pagnoni , A , Pellizzari , M , Fasulo , MP , Fagioli , F , Bonora , A and Pancaldi , S . 2004 . Specific intra-tissue responses to manganese in the floating lamina of Trapa natans L . Plant Biol. , 6 : 578 – 589 .
- Baldisserotto , C , Ferroni , L , Andreoli , C , Fasulo , MP , Bonora , A and Pancaldi , S . 2005a . Dark-acclimation of the chloroplast in Koliella antarctica exposed to a simulated austral night condition . Arct. Antarct. Alp. Res. , 37 : 146 – 156 .
- Baldisserotto , C , Ferroni , L , Moro , I , Fasulo , MP and Pancaldi , S . 2005b . Modulations of the thylakoid system in snow xanthophycean alga darkened for two months: comparison between microspectrofluorimetric responses and morphological aspects . Protoplasma , 226 : 125 – 135 .
- Bell , EM and Laybourn-Parry , J . 2003 . Mixotrophy in the Antarctic phytoflagellate, Pyramimonas gelidicola (Chlorophyta: Prasinophyceae) . J. Phycol. , 39 : 644 – 649 .
- Böddi , B and Franck , F . 1997 . Room temperature fluorescence spectra of protochlorophyllide and chlorophyllide forms in etiolated bean leaves . J. Photochem. Photobiol. B , 41 : 73 – 82 .
- Bonora , A , Pancaldi , S , Gualandri , R and Fasulo , MP . 2000 . Carotenoid and ultrastructure variations in plastids of Arum italicum Miller fruit during maturation and ripening . J. Exp. Bot. , 51 : 873 – 884 .
- Bossy-Wetzel , E , Barsoum , MJ , Godzik , A , Schwarzenbacher , R and Lipton , SA . 2003 . Mitochondrial fission in apoptosis, neurodegeneration and aging . Curr. Opin. Cell Biol. , 15 : 706 – 716 .
- Bunt , JS and Lee , CC . 1972 . Data on the composition and dark survival of four sea-ice microalgae . Limnol. Oceanogr. , 17 : 458 – 461 .
- Chen , S and Dickman , MB . 2004 . Bcl-2 family members localize to tobacco chloroplasts and inhibit programmed cell death induced by chloroplast-targeted herbicides . J. Exp. Bot. , 55 : 2617 – 2623 .
- Dehning , J and Tilzer , MM . 1989 . Survival of Scenedesmus acuminatus (Chlorophyceae) in darkness . J. Phycol. , 25 : 509 – 515 .
- Deponte , M and Becker , K . 2004 . Plasmodium falciparum – do killers commit suicide? . Trends Parasitol. , 20 : 165 – 169 .
- Di Martino Rigano , V , Vona , V , Lobosco , O , Carillo , P , Lunn , JE , Carfagna , S , Esposito , S , Caiazzo , M and Rigano , C . 2006 . Temperature dependence of nitrate reductase in the psychrophilic unicellular alga Koliella antarctica and the mesophilic alga Chlorella sorokiniana . Plant Cell Environ. , 29 : 1400 – 1409 .
- Fabregas , J , Herrero , C , Cabezas , B and Abalde , J . 1984 . Growth of the marine microalga Tetraselmis suecica in batch cultures with different salinities . Aquaculture , 42 : 207 – 215 .
- Fasulo , MP , Bassi , M and Donini , A . 1983 . Cytotoxic effects of hexavalent chromium in Euglena gracilis II: physiological and ultrastructural studies . Protoplasma , 114 : 35 – 43 .
- Ferroni , L , Baldisserotto , C , Pagnoni , A , Fasulo , MP and Pancaldi , S . 2004 . Adaptive modifications of the photosynthetic apparatus in Euglena gracilis Klebs exposed to manganese excess . Protoplasma , 224 : 167 – 177 .
- Franklin , DJ and Berges , JA . 2004 . Mortality in cultures of the dinoflagellate Amphidinium carterae during culture senescence and darkness . Proc. Royal Soc. Lond. Ser. B. Biol. Sci. , 271 : 2099 – 2107 .
- Franklin , DJ , Brussard , CPD and Berges , JA . 2006 . What is the role and nature of programmed cell death in phytoplankton ecology? . Eur. J. Phycol. , 41 : 1 – 14 .
- Fröhlich , K-U and Madeo , F . 2000 . Apoptosis in yeast – a monocellular organism exhibits altruistic behaviour . FEBS Lett. , 473 : 6 – 9 .
- Gavrieli , Y , Sherman , Y and Ben-Sasson , SA . 1992 . Identification of programmed cell-death in situ via specific labelling of nuclear DNA fragmentation . J. Cell Biol. , 119 : 493 – 501 .
- Geitmann , A , Franklin-Tong , VE and Emons , AC . 2004 . The self-incompatibility response in Papaver rhoeas pollen causes early and striking alterations to organelles . Cell Death Diff. , 11 : 812 – 822 .
- Giacometti , GM , La Rocca , N , Zanetti , S and Andreoli , C . 2001 . “ Non-photochemical quenching in Koliella antarctica, a new antarctic green algae isolated in the Ross Sea ” . In Proceedings of the XL Congresso Annuale della Società Italiana di Fisiologia Vegetale , 52 – 53 . Padova, , Italy : Abano Terme .
- Gray , J , Buckner-Janick , D , Buckner , B , Close , PS and Johal , GS . 2002 . Light-dependent death of maize lls1 cells is mediated by mature chloroplast . Plant Physiol. , 130 : 1894 – 1907 .
- Groot , M-L , Frese , RN , De Weerd , FL , Bromek , K , Petterson , Å. , Peterman , EJG , Van Stokkum , IHM , Van Grondelle , R and Dekker , JP . 1999 . Spectroscopic properties of the CP43 core antenna protein of photosystem II . Biophys. J. , 77 : 3328 – 3340 .
- Guillard , RRL . 1975 . “ Culture of phytoplankton for feeding marine invertebrates ” . In Culture of Marine Invertebrate Animals , Edited by: Smith , WL and Chanley , MH . 29 – 60 . New York, , USA : Plenum Press .
- Gunawardena , AHLAN , Greenwood , JS and Dengler , NG . 2004 . Programmed cell death remodels lace plant leaf shape during development . Plant Cell , 16 : 60 – 73 .
- Hindák , F . 1996 . New taxa and nomenclatural changes in the Ulotrichineae (Ulotrichales, Chlorophyta) . Biologia (Bratislavia) , 51 : 357 – 364 .
- Ignatov , NV and Litvin , FF . 1993a . Light-induced synthesis of pheophytin a in plant-leaves . Biochemistry (Moscow) , 58 : 1074 – 1083 .
- Ignatov , NV and Litvin , FF . 1993b . Regulation of biosynthesis of pheophytin a in plant-leaves by light . Biochemistry (Moscow) , 58 : 1210 – 1219 .
- Ignatov , NV and Litvin , FF . 1994 . Photoinduced formation of pheophytin/chlorophyll-containing complexes during the greening of plant-leaves . Photosynth. Res. , 42 : 27 – 35 .
- Ignatov , NV and Litvin , FF . 1998 . A comparative study of the terminal stages of chlorophyll biosynthesis before and after water (D2O) introduction into greening plant leaves . Photosynth. Res. , 56 : 83 – 93 .
- Jones , AM . 2001 . Programmed cell death in development and defence . Plant Physiol. , 125 : 94 – 97 .
- Katana , A , Kwiatowski , J , Spalik , K , Kakryś , B , Szalacha , E and Szymańska , H . 2001 . Phylogenetic position of Koliella (Chlorophyta) as inferred from nuclear and chloroplast small subunit rDNA . J. Phycol. , 37 : 443 – 451 .
- Krause , GH and Weis , DN . 1991 . Chlorophyll fluorescence and photosynthesis: the basics . Ann. Rev. Plant Physiol. Plant Mol. Biol. , 42 : 313 – 349 .
- Le Lay , P , Böddi , B , Kovacevic , D , Juneau , P , Dewez , D and Popovic , R . 2001 . Spectroscopic analysis of desiccation-induced alterations of the chlorophyllide transformation pathway in etiolated barley leaves . Plant Physiol. , 127 : 202 – 211 .
- Lenucci , M , Leucci , MR , Andreoli , C , Dalessandro , G and Piro , G . 2006 . Biosynthesis and characterization of glycoproteins in Koliella antarctica (Klebsormidiales, Chlorophyta) . Eur. J. Phycol. , 41 : 213 – 222 .
- Lüder , UH , Wiencke , C and Knoetzel , J . 2002 . Acclimation of photosynthesis and pigments during and after six months of darkness in Palmaria decipiens (Rhodophyta): a study to simulate antarctic winter sea ice cover . J. Phycol. , 38 : 904 – 913 .
- Ludovico , P , Sansonetty , F , Silva , MT and Corte-Real , M . 2003 . Acetic acid induces a programmed cell death process in the food spoilage yeast Zygosaccharomyces bailii . Fems Yeast Res. , 3 : 91 – 96 .
- McDonnel , A and Staehelin , LA . 1980 . Adhesion between liposomes mediated by the chlorophyll a/b light-harvesting complex isolated from chloroplast membrane . J. Cell Biol. , 84 : 40 – 56 .
- Moharikar , S , D'souza , JS , Kulkarni , AB and Rao , BJ . 2006 . Apoptotic-like cell death pathway is induced in unicellular chlorophyte Chlamydomonas reihardtii (Chlorophyceae) cells following UV irradiation: detection and functional analyses . J. Phycol. , 42 : 423 – 433 .
- Morgan-Kiss , RM , Priscu , CJ , Pocock , T , Gudynaite-Savitch , L and Huner , NPA . 2006 . Adaptation and acclimation of photosynthetic microorganisms to permanently cold environments . Microbiol. Mol. Biol. Rev. , 70 : 222 – 252 .
- Palmisano , AC and Sullivan , CW . 1982 . Physiology of sea ice diatoms. 1. Response of three polar diatoms to a simulated summer-winter transition . J. Phycol. , 18 : 489 – 498 .
- Palmisano , AC and Sullivan , CW . 1983 . Physiology of sea ice diatoms. 2. Dark survival of three polar diatoms . Can. J. Microbiol. , 29 : 157 – 160 .
- Pancaldi , S , Baldisserotto , C , Ferroni , L , Bonora , A and Fasulo , MP . 2002 . Room temperature microspectrofluorimetry as a useful tool for studying the assembly of the PSII chlorophyll-protein complexes in single living cells of etiolated Euglena gracilis Klebs during the greening process . J. Exp. Bot. , 53 : 1753 – 1763 .
- Piro , G , La Rocca , N , Moro , I , Rascio , N and Andreoli , C . 1997 . Biosynthesis of glycoproteins in a green microalga of the Southern Oceans . J. Biol. Res. , 73 : 59 – 60 .
- Piro , G , Lenucci , M , Dalessandro , G , La Rocca , N , Rascio , N , Moro , I and Andreoli , C . 2000 . Ultrastructure, chemical composition and biosynthesis of the cell wall in Koliella antarctica (Klebsormidiales, Chlorophyta) . Eur. J. Phycol. , 35 : 331 – 337 .
- Porra , RJ . 1990 . A simple method for extracting chlorophylls from the recalcitrant alga, Nannochloris atomus, without formation of spectroscopically-different magnesium-rhodochlorin derivatives . Biochim. Biophys. Acta , 1019 : 137 – 141 .
- Porra , RJ . 2002 . The chequered history of the development and use of simultaneous equations for the accurate determination of chlorophylls a and b . Photosynth. Res. , 73 : 149 – 156 .
- Prakash , JSS , Baig , MA and Mohanty , P . 2001 . Senescence induced structural reorganization of thylakoid membranes in Cucumis sativus cotyledons; LHCII involvement in reorganization of thylakoid membranes . Photosynth. Res. , 68 : 153 – 161 .
- Samuilov , VD , Lagunova , EM , Dzyubinskaya , EV , Izyumov , DS , Kiselevsky , DB and Makarova , YV . 2002 . Involvement of chloroplasts in the programmed death of plant cells . Biochemistry (Moscow) , 67 : 627 – 634 .
- Santabarbara , S , Neverov , KV , Garlaschi , FF , Zucchelli , G and Jennings , RC . 2001 . Involvement of uncoupled antenna chlorophylls in photoinhibition in thylakoids . FEBS Lett. , 491 : 109 – 113 .
- Segovia , M , Haramaty , L , Berges , JA and Falkowski , PG . 2003 . Cell death in the unicellular chlorophyte Dunaliella tertiolecta. A hypothesis on the evolution of apoptosis in higher plants and metazoans . Plant Physiol. , 132 : 99 – 105 .
- Simidjiev , I , Barzda , V , Mustardy , L and Garab , G . 1998 . Role of thylakoid lipids in the structural flexibility of lamellar aggregates of the isolated light-harvesting chlorophyll a/b complex of photosystem II . Biochemistry , 37 : 4169 – 4173 .
- Smayda , TJ and Mitchell-Innes , B . 1974 . Dark survival of autotrophic, planktonic marine diatoms . Mar. Biol. , 25 : 195 – 202 .
- Soldani , C , Bottone , MG , Pellicciari , C and Scovassi , AI . 2001 . Two-color fluorescence detection of Poly(ADP-ribose) Polymerase-1 (PARP-1) cleavage and DNA strand breaks in etoposide-induced apoptotic cells . Eur. J. Histochem. , 45 : 389 – 392 .
- Soldani , C , Scovassi , AI , Canosi , U , Bramucci , E , Ardissino , D and Arbustini , E . 2005 . Multicolor fluorescence technique to detect apoptotic cells in advanced coronary atherosclerotic plaques . Eur. J. Histochem. , 49 : 47 – 52 .
- Stibal , M and Elster , J . 2005 . Growth and morphology variation as a response to changing environmental factors in two Antarctic species of Raphidonema (Trebouxiophyceae) from snow and soil . Polar Biol. , 28 : 558 – 567 .
- Stürzl , E and Schiff , JA . 1986 . Phototransformation of protochlorophyll(ide) by a proplastid fraction from Euglena . Plant Physiol. , 66 : 309 – 318 .
- Vannini , GL , Pancaldi , S , Poli , F and Fasulo , MP . 1988 . Rhodamine 123 as a vital stain for mitochondria of plant cells . Plant Cell Environ. , 11 : 123 – 127 .
- Vasil'ev , S , Irrgang , K-D , Schrötter , T , Bergmann , A , Eichler , H-J and Renger , G . 1997 . Quenching of chlorophyll a fluorescence in the aggregates of LHCII: steady state fluorescence and picosecond relaxation kinetics . Biochemistry , 36 : 7503 – 7512 .
- Vassiliev , IR , Kolber , Z , Wyman , KD , Mauzerall , D , Shukla , VK and Falkowsky , PG . 1995 . Effects of iron limitation on photosystem II composition and light utilization in Dunaliella tertiolecta . Plant Physiol. , 109 : 963 – 972 .
- Vona , V , Di Martino Rigano , V , Lobosco , O , Carfagna , S , Esposito , S and Rigano , C . 2004 . Temperature responses of growth, photosynthesis, respiration and NADH:nitrate reductase in cryophilic and mesophilic algae . New Phytol. , 163 : 325 – 331 .
- Wellburn , AR . 1994 . The spectral determination of chlorophylls a and b, as well as total carotenoids, using various solvents with spectrophotometers of different resolution . J. Plant Physiol. , 144 : 307 – 313 .
- Zanetti , S , La Rocca , N , Moro , I , Salvato , B , Di Mauro , P , Rascio , N and Andreoli , C . 2001 . Effects of environmental factors on morphology, ultrastructure and growth of the green alga Koliella antarctica . Nova Hedwig. , 123 : 585 – 592 .
- Zhang , Q , Gradinger , R and Zhou , QS . 2003 . Competition within the marine microalgae over the polar dark period in the Greenland Sea of high Arctic . Acta Oceanolog. Sinica , 22 : 233 – 242 .