Abstract
Ditylum brightwellii (T. West) Grunow in Van Heurck is now a model organism for population genetic studies of marine phytoplankton, but observations of sexual reproduction in this species are sparse and there are controversial aspects to the identification of its gametes. Culture studies and field observations of D. brightwellii from Wadsworth Cove (Castine), Maine (USA) showed that it was homothallic, producing and releasing two naked, spherical eggs from each oogonium and 64 uniflagellate sperm per spermatogonangium. Eggs and sperm were produced by larger and smaller cells, respectively, and were formed in nutrient-replete medium, without special procedures for induction. Size regeneration was achieved from a putatively fertilized egg via a true auxospore. Vegetative cell enlargement occurred in senescent cultures and resulted in intermediate-sized cells. The size structure of natural populations of D. brightwellii was monitored weekly in autumn 2004 and was unimodal throughout, with slight shifts in size accompanying low levels of gametogenesis and auxosporulation. Gametogenesis and size reduction ceased with the onset of winter. Previous reports concerning gametogenesis and auxosporulation in Ditylum are reinterpreted on the basis of our studies.
Introduction
Recent studies of the population genetics of diatoms show striking differences in population structure between the centric diatom Ditylum brightwellii (T. West) Grunow in van Heurck (Rynearson & Armbrust, Citation2000, Citation2004, Citation2005) and the pennate diatom Pseudo-nitzschia pungens (Grunow ex Cleve) Hasle (Evans et al., Citation2005). Ditylum brightwellii shows a high degree of differentiation within relatively small geographical areas, whereas P. pungens behaves as a single panmictic population across the North Sea. Knowledge of the mating systems of these species may help to explain these differences. The life history of Pseudo-nitzschia H. Peragallo in Peragallo et Peragallo is now well documented and usually involves heterothally (Davidovich & Bates, Citation1998; Hiltz et al ., Citation2000; Amato et al ., Citation2005; Chepurnov et al ., Citation2005; homothally has been reported only in P. subcurvata (Hasle) G. Fryxell: Fryxell et al ., Citation1991), but information on sexual reproduction in D. brightwellii is mostly indirect or anecdotal.
Ditylum brightwellii is commonly found in coastal waters and is frequently used in studies of diatom physiology and cytology (e.g. Eppley et al ., Citation1967; Falkowski, Citation1975; Li & Volcani Citation1984, Citation1985; Li et al ., Citation1989; Pickett-Heaps et al ., Citation1988; Lee & Li, Citation1992; Pollock & Pickett-Heaps, Citation2005, Citation2006). The high levels of genetic diversity found in populations of D. brightwellii from Puget Sound, Washington (USA), suggest that sexual reproduction is an important component of the life history of this species (Rynearson & Armbrust, Citation2000, Citation2004, Citation2005). Male gametangia (spermatogonangia) were observed in natural populations of D. brightwellii during late summer and early autumn (Steele, Citation1967; Drebes, Citation1974; Waite & Harrison, Citation1992), but were not found in an extensively sampled spring bloom (Rynearson & Armbrust, Citation2005). Female gametangia have also been reported in natural populations of D. brightwellii (Waite & Harrison, Citation1992, ) but were possibly misidentified vegetative cells with contracted cytoplasm (Waite, personal communication to L.K.B.). Auxospores of D. brightwellii have apparently never been observed in natural populations. On a few occasions, gametes and auxospores have been reported in laboratory cultures, but published descriptions of the morphology of eggs and auxospores are inconsistent and misleading. This is partly because two quite different and entirely vegetative processes have been confused with egg production. These are the extrusion of the protoplast into the medium (Karsten, Citation1899, ; Gross, Citation1937, p. 20, pl. 3, , ; Steele, Citation1967, pl. 2, figs ) and vegetative cell enlargement (Gross, Citation1937, pl. 3, ).
Our goals were (i) to verify the life history of Ditylum brightwellii in the laboratory, using clones recently isolated from natural populations; (ii) to determine the mating system of D. brightwellii and examine the relationship between cell size and gametogenesis; and (iii) to monitor natural populations of D. brightwellii for the presence of sexual stages and changes in cell size distributions.
Materials and methods
Cell isolation and culturing
Single cells of Ditylum brightwellii were isolated from Wadsworth Cove, Castine, Maine, USA (44°23′75″N, 68°48′75″W), during late summer and early autumn, 2004. Clonal, non-axenic cell lines were obtained by micropipetting individual cells through aliquots of sterilized seawater into 0.5 ml of f/20 or f/10 medium (Guillard & Ryther, Citation1962) in a 48-well plate (Costar, Corning, NY). After a week, each clone was transferred to 10 ml of more concentrated medium (f/10 or f/2), depending on the starting medium (after Rynearson & Armbrust, Citation2000). It was later determined that cells could be isolated directly into f/10 with no ill effects. Stock cultures of each clone were maintained in f/2 (osmolality ∼899 mmol kg−1, pH 8.0) at 17°C, with irradiance of approximately 50 µmol photons m−2 s−1 provided from Sylvania Cool White, 60 watt tubes (Sylvania, Danvers, MA), and a 13:11 h light–dark photoperiod.
Mating system experiments
Two separate mating system experiments were conducted 4 weeks apart. To ensure minimal variation in cell size within experimental clones, three to five individual cells were re-isolated from each of 10 stock cultures, 14–18 days prior to each experiment. A rapidly dividing cell line of each original clone was maintained in exponential growth by transferring it daily to fresh f/2 medium. Four clones were selected for each experiment () based on cell density, minimal variation in cell size, and preliminary data for two of the clones demonstrating the potential for gametogenesis.
Table 1. Clones of Ditylum brightwellii used in two mating system experiments. The mean diameter of each clone at the beginning of each experiment (n = 100 cells per clone) is given. Two clones, N3 and N4, were used in both experiments, and denoted as N3′ and N4′ in the second experiment.
Each clone was paired with itself and with every other clone, giving six interclonal and four intraclonal pairs, by mixing 75 ml of each clone at a final concentration of approximately 200 cells ml−1 in 250-ml Erlenmeyer flasks. Each of the 10 treatments was duplicated within an experiment. Treatments were incubated for 7 days at 17°C, with a 13:11 h light–dark photoperiod, and an irradiance of 50 µmol photons m−2 s−1. The flasks were repositioned randomly each day after sampling to reduce possible effects of light attenuation within the incubator. Daily, at approximately 0800 h, aliquots of 5 ml were collected from each treatment (without volume replacement) and fixed immediately in a mixture containing 1% glutaraldehyde (Biological Grade, Electron Microscopy Sciences, Hatfield, PA) and 1% formaldehyde (Sigma, St Louis, MO) in Gulf of Maine seawater (approximately 32 psu) adjusted to 60% osmolality (Wescor 5500 Vapor Pressure Osmometer, Logan, UT) with deionized water.
Gametes, auxospores, and vegetative cells from fixed preparations were enumerated with a Nikon Optiphot-2 microscope (100 × magnification), using a glass Sedgewick–Rafter chamber (Wildco, Buffalo, NY). Intact spermatogonangia were counted separately from spermatogonangia whose thecae had separated but still had sperm remaining inside one lone theca. The number of separated spermatogonangia was halved and the resultant value was added to the number of intact spermatogonangial cells. Because immature oogonia could not be distinguished from vegetative cells and because each oogonium produced two eggs, the total number of mature oogonia in each sample was calculated as half the number of eggs that were present. Cells were counted as auxospores only when a (silicified) properizonium (von Stosch, Citation1982) could be detected.
To evaluate uncertainty in our counts, we randomly chose 18 samples (from three different treatments) for triplicate counts of cell numbers; uncertainty in counts ranged from 2.1 to 6.1% for the total number of cells counted. However, for cell types such as auxospores that were relatively rare when compared with the number of vegetative cells, the coefficient of variation was over 100% in multiple samples. For these 18 samples, the triplicate counts were pooled, and the average was used in statistical analyses.
Size distributions of cells in each clone were determined at the beginning of the experiment and then again on day 5 for a subset of the clones and interclonal crosses. Cells were measured in girdle view, perpendicular to the pervalvar axis at the widest point, using a Zeiss IM-35 inverted microscope (400× magnification) equipped with an ocular micrometer.
Natural populations
Weekly samples of surface water (1 litre) were collected with a Niskin bottle from Wadsworth Cove, Maine () from 31 August to 7 December 2004. Samples were fixed immediately with a final concentration of 1% glutaraldehyde. Samples were concentrated by allowing cells to settle for at least 48 h before carefully drawing off 650–800 ml of the supernatant by suction. The remaining sample was then thoroughly (but gently) mixed by inversion and subsamples of 5–20 ml (depending on the concentrations of cells in the samples) were allowed to settle for 24–48 h (Utermöhl, Citation1931). A minimum of three subsamples was counted for each sampling date. Cell sizes of D. brightwellii were measured as previously described (n = 491–1,000 cells per sample) and sexual stages were identified. Cell sizes were binned in 4- µm increments to smooth artificial steps created by ocular scaling. Statistical analysis was performed using SYSTAT software (v. 11.00.01; Point Richmond, CA); this analysis is provided with the caveat that the samples were taken as a collection within a Niskin bottle, and individual cells were not sampled randomly one at a time from the water column (Venrick, Citation1986).
Microscopy
Preparations of live cells at various stages in the life cycle of D. brightwellii were viewed in 48-well plates with a Zeiss IM-35 inverted microscope, and fixed preparations of cells from the mating experiments were observed in a Sedgewick–Rafter chamber with a Nikon Optiphot-2 microscope. All photomicrographs were taken with a SPOT digital camera (Diagnostics Instruments, Sterling Heights, MI).
Nuclei in fixed cells were stained for 5–7 min with the DNA stain 4,6 diamidino-2-phenylindole (DAPI: Sigma, St Louis, MO) at a concentration of 1.25 µg l−1 DAPI in sterile seawater. The cells were retained in a beam capsule fitted with Nitex mesh (1-µm mesh size) during staining and subsequent washes in sterile seawater. DAPI-stained cells were viewed with fluorescence microscopy (Nikon Optiphot-2 microscope equipped with UV excitation and a red suppression filter, >620 µm, Chroma Technology Corp., Rockingham, VT).
Results
Gametogenesis and auxosporulation
Clonal isolates of Ditylum brightwellii from Maine undergoing sexual reproduction were homothallic and oogamous, each clone produced both eggs and sperm. Frequently, two eggs were observed at the open ends of closely associated thecae (), indicating that two eggs are produced by the same mother cell. Subsequently, eggs often separated from the thecae and many eggs were observed free in the culture medium. Eggs were neutrally buoyant and floated (occasionally with attached thecae) off the bottom of the culture vessel, but did not rise to the surface of the medium in any of the observed cultures. Eggs were uninucleate, as determined by DAPI-staining (, ). Oogonial mother cells were not positively identified prior to egg release despite careful examination of the cultures and photomicrographs for changes in pigmentation and length of cells that otherwise appeared to be vegetative.
Figs. 2–10. Life-cycle stages in Ditylum brightwellii. . Live egg (arrow) and sperm (arrowhead) and auxospore (double arrow). . Fixed egg viewed with bright-field microscopy. . The same egg stained with DAPI. . Mass spermatogenesis in a live culture. Note swelling of spermatogonia (arrow). . Spermatogonangium with rudimentary thecae (arrow) in 1% glutaraldehyde and formaldehyde fixative. . Early stage auxospores in living preparation. Note properizonium (arrows). . Auxospores developed fully; formation of both valves of the initial cells is nearly complete (living preparation). . Resting spore of Ditylum brightwellii in a clonal culture from a natural population at Wadsworth Cove (fixed sample). . Vegetative cell enlargement in living Ditylum brightwellii. Scale bars: 10 µm.
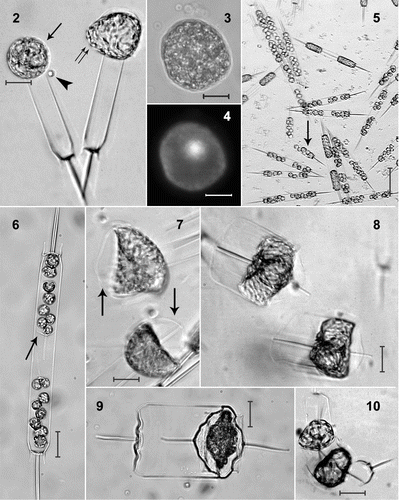
Fig. 11. Life history of Ditylum brightwellii. Size reduction occurs over time via vegetative cell division. Resting spores develop from, and germinate into vegetative cells (Hargraves, Citation1984). Sexual reproduction takes place between haploid eggs and sperm; the auxospore (diploid) is a specialized zygotic cell.
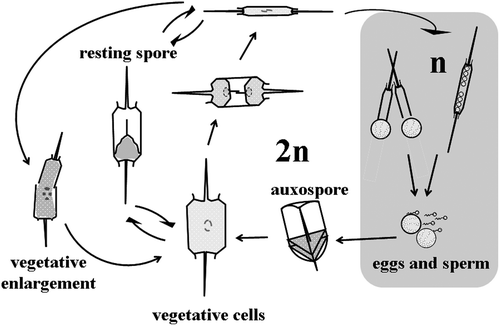
Fig. 12. Natural logarithm of the ratio of production of oogonia to spermatogonangia (O:S) in Ditylum brightwellii. Each clone is represented by its diameter measured on day 0. The ratio is the average of the duplicated treatments over the entire experiment ± SD (n = 16). The clones with the open symbol (○) did not produce gametes.
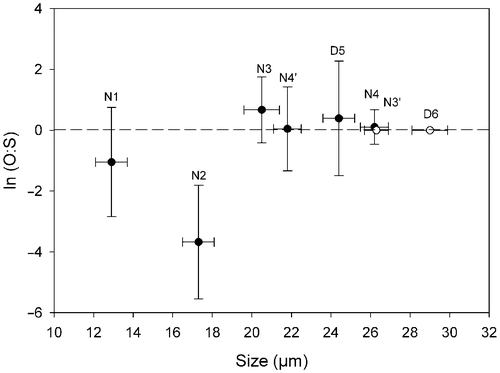
In the spermatogonangia, the spermatogonia tended to cluster towards the valves and away from the centre of the girdle (). Occasionally, rudimentary thecae were observed separating the clusters of spermatogonia (). Spermatogenesis was hologenous, the chloroplasts were retained and divided among the sperm instead of being expelled into a residual body (‘merogenous’ spermatogenesis). Sixty-four sperm were generally produced and released per spermatogonangium. Some spermatogonia swelled at the 16-cell stage, prior to meiosis (). This swelling may aid in dehiscence of the spermatogonangia (cf. Pickett-Heaps & Pickett-Heaps, Citation2003). Each spherical sperm had a single anterior flagellum that moved in a sinusoidal pattern while the sperm were swimming.
Fig. 13. The frequency (%) of gamete production in three mating crosses of Ditylum brightwellii over 7 days. Shaded areas represent the area under the curve of the mean of duplicate measurements (filled circles). Trends in gamete production are highlighted with the upper solid line representing the mean multiplied by five.
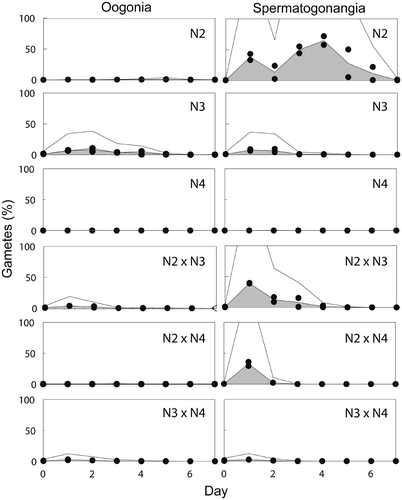
Fig. 14. Frequency (%) of auxospores produced in three mating crosses of Ditylum brightwellii over 7 days. Shaded areas represent the area under the curve of the mean of duplicate measurements (filled circles). Trends in gamete production are highlighted with the upper solid line representing the mean multiplied by five.
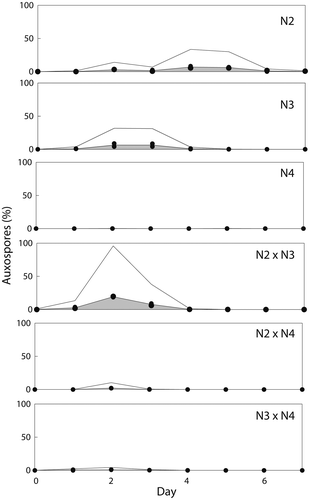
The resolution of sampling was 24 h. During this interval, cultures that were initially purely vegetative produced both eggs and sperm, so that the whole time required for gametogenesis must be <24 h. Sperm were observed close to eggs (), but fertilization was not observed. Auxospores were observed in various stages of development (, , ). If eggs were still attached to the oogonial thecae upon presumed fertilization, auxospore development continued without detachment of the thecae (, ); otherwise, the auxospores developed freely in the medium (). Silicification and the moulding of the characteristic prism-shape of D. brightwellii became apparent shortly after initiation of auxospore development (). Late-stage auxospores and initial cells were 47.5–71 µm in diameter (mean: 59 ± 5 µm SD; n = 43) and had become triradiate (). The appearance of large, prism-shaped cells in cultures in which auxospores were absent on the previous day indicates that initial cells developed from zygotes within 24 h. The whole process of auxosporulation, from the beginning of gametogenesis to the formation of new initial cells, thus took approximately 2 days.
Fig. 15. Abundance of Ditylum brightwellii at Wadsworth Cove, autumn 2004. Mean number of cells l−1 ± SD (○ indicates days on which spermatogonangia were absent).
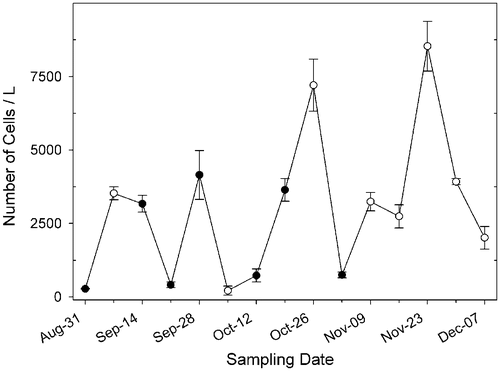
Fig. 16. Size frequency distributions of populations of Ditylum brightwellii from Wadsworth Cove by sampling date. The stars denote presence of spermatogonangia and the dotted line is the upper size limit for gametogenesis observed in lab experiments.
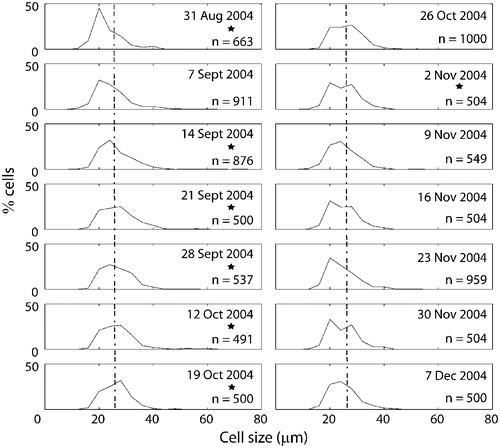
Resting spores (, agreeing with the description given by Hargraves, Citation1984) and vegetative cell enlargement (, cf. von Stosch, Citation1965) were observed in some clones; neither process was associated with sexual reproduction and both were observed in cultures entering or in stationary phase. Cells resulting from vegetative cell enlargement were typically smaller than initial cells (range: 27–53 µm; mean: 40 µm ± 7 µm; n = 19) and had irregular or rounded valve morphologies contrasting with the triradiate, sexually-derived cells ().
Fig. 17. Changes in mean, median and principal mode of Ditylum brightwellii from the Wadsworth Cove population during autumn 2004.
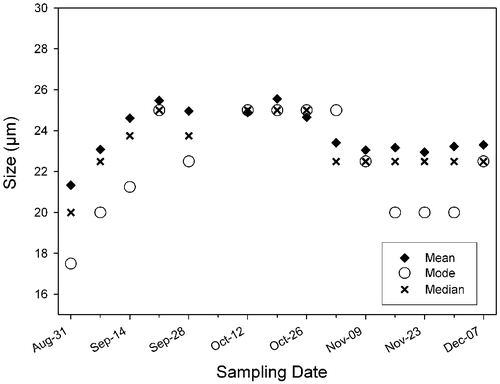
Fig. 18. Size distributions in Ditylum brightwellii N2 × N4 cross over time. Straight line represents day 0, dashed line represents day 5. Day 0, n = 50 (intraclonal crosses), n = 200 (interclonal crosses); day 5, n = 200 (all treatments). The dotted line is the upper size limit for gametogenesis observed in lab experiments.
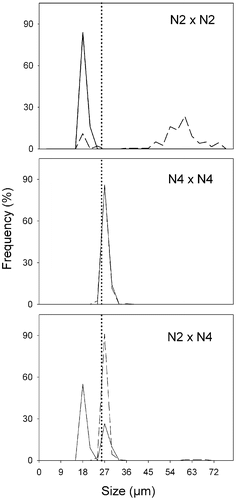
A summary of the life history of D. brightwellii is provided in .
Mating system experiments
The potential of each clone to produce gametangia was evaluated from the intraclonal ‘crosses’ of the mating system experiments. The differentiation of vegetative cells of D. brightwellii into spermatogonangia and oogonia was related to size (). Clones of different sizes produced both spermatogonangia and oogonia but in different proportions. Clones of the smallest mean cell size (<20 µm) produced predominantly spermatogonangia (N1 and N2). Clones of intermediate mean cell sizes (20–26 µm) produced either equal numbers of oogonia and spermatogonangia (N4 and N4′, although very few gametes were produced by N4) or predominantly oogonia (N3 and D5). Clones with the largest cell sizes (mean ≥26 µm; N3′ and D6) did not produce any gametes ().
A broad range in gametogenesis and fertilization occurred among the crosses of the mating experiments (). To highlight this variation, we focused on N2 (predominantly a sperm producer), N3 (producing both types of gametes, but with a slight predominance of oogenesis) and N4 (predominantly vegetative). The highest levels of spermatogenesis and oogenesis occurred in N2 and N3, respectively (, ). By the fourth day of the experiment, spermatogonangia accounted for up to 70% of all cells in N2 (). N3 produced both oogonia and spermatogonangia in equal numbers for the first 2 days of the experiment, after which more oogonia than spermatogonangia were produced (). It was expected that the absolute number of gametangia produced at the beginning of the experiment in the N2 × N3 cross would be approximately equal to the sum of half of the gametes produced by N2 and N3 when present alone, due to dilution when the clones were mixed together. In fact, early in the experiment, spermatogenesis was greater than expected in the interclonal N2 × N3 cross, and the number of spermatogonangial cells was equal to that in N2 alone (); oogenesis was also greater than expected. However, by day 3 in the N2 × N3 cross, gametogenesis, especially spermatogenesis, had decreased considerably and less than half the number of spermatogonangia was observed as in the intraclonal treatments ().
Table 2. Gamete and auxospore production by Ditylum brightwellii in two, 7-day, mating experiments.
Auxospores were formed in N2 and N3, and auxospores represented up to 10% of all cell types in these treatments (). Fertilization appeared to occur earlier in the N2 × N3 cross than in N2 or N3 alone, and up to 20% of the cells in the cross were auxospores ().
In contrast to N2 and N3, gamete production by N4 (larger mean cell size) was rare and there was virtually no auxosporulation (Figs , ). When N4 was paired with the sperm-producing N2, spermatogenesis in the N2 × N4 cross was roughly equal to that in N2 alone for the first two days, and then ceased altogether (). Very few auxospores were produced in the N2 × N4 cross () and only on the second day of the experiment. By contrast, when N2 was grown on its own, spermatogenesis peaked on days 3 and 4 and continued until day 6 (). The formation of auxospores in pure N2 showed a similar pattern to spermatogenesis but with a delay of one day, peaking on days 4 and 5 ().
When N4 was crossed with the egg-producing N3 (N3 × N4), gametogenesis and auxosporulation were less than in the intraclonal N3 × N3 (Figs , , , . This result suggests that the reduction of sexual reproduction in N3 × N4 was due to the lack of any effect in N4 cells plus the dilution of N3 by N4 cells.
Natural populations
The abundance of D. brightwellii at Wadsworth Cove varied throughout autumn 2004, ranging from 215 ± 154 cells l−1 to 8535 ± 848 cells l−1 (). Spermatogonangia were observed in most samples collected from late summer to mid-autumn (August to early November), which corresponded to a decrease in daylength (from 13 to 10 h) and sea surface temperature (from 17 to 10°C). Spermatogonangia were absent in samples collected from mid-November to early December. Eggs and auxospores were not detected at any time. Concentrations of spermatogonangia were always low, representing 0.2–2.4% of the D. brightwellii population (). There was no correlation between the concentrations of spermatogonangia and vegetative cells (; ). The proportion of spermatogonangia was greatest (2.4%) on 21 September, when the concentration of D. brightwellii was low (413 cells l−1), but was low (0.2%) on 19 October, when D. brightwellii was relatively abundant (3644 ± 389 cells l−1). There was no correlation (p > 0.05; R 2 = 0.13) between the concentration of spermatogonangia and the concentration of cells smaller than 26 µm, which laboratory experiments indicated was the upper limit of the sexually inducible size range (see above). Spermatogonangia varied in size from 12.5 to 26.5 µm (mean: 18.6 ± 3.5 µm; n = 42), agreeing well with the range seen in the laboratory.
Table 3. Relative abundance and mean size of spermatogonangia of Ditylum brightwellii at Wadsworth Cove, autumn 2004.
Only one of many thousands of D. brightwellii cells observed in field samples had an aberrant morphology suggestive of vegetative enlargement. The cell had three valves, two of which were fused together at one end of an otherwise normally shaped frustule.
Size frequency distributions of D. brightwellii cells from Wadsworth Cove were essentially unimodal (). Mean size of D. brightwellii increased gradually between late summer (31 August) and mid-autumn (12 October), and then began to decrease (). The smallest size class disappeared on 21 September and a greater number of large cells appeared, creating a significantly different size distribution than the one observed on 31 August (Kolmogorov–Smirnoff test, n = 663 and 500, respectively; p<0.01).
Discussion
The sexual cycle
Conventional wisdom holds that most diatoms are obligately sexual organisms. However, sexual stages are known from only a tiny minority of diatom species (Chepurnov et al ., Citation2004). It is easy to demonstrate that populations possess the kind of morphological variation that would be expected from the presence of a size reduction–restitution cycle, but this does not necessarily mean that gametogenesis and sexual reproduction occur. Some diatoms are known to be apomictic (see below, ‘Mating system’). In D. brightwellii, the morphological plasticity associated with vegetative cells, especially when under physiological stress, and the presence of alternative life-history strategies (the formation of resting spores and vegetative cell enlargement) have confused several previous researchers, leading to erroneous descriptions of auxosporulation.
In our experiments, eggs, sperm and auxospores were produced in healthy, nutrient-replete (exponentially growing) cultures, without intentionally supplying extra cues for sexual induction. However, the low level of light under which cultures were maintained may have contributed to the induction of sexuality (Mizuno & Okuda, Citation1985). The early stages in oogenesis in D. brightwellii were not studied because young oogonia could not be distinguished from vegetative cells. Later, differentiation became obvious through the release of two naked eggs from each oogonium; each egg contained one nucleus. Release of two eggs was mentioned briefly by von Stosch (Citation1987) and illustrated in video sequences by Pickett-Heaps & Pickett-Heaps (Citation2003). The related genus Lithodesmium Ehrenberg seems to be the only other diatom known to produce and release two naked eggs per oogonium (von Stosch, Citation1954). Recent studies in molecular genetics corroborate the close relationship between Ditylum and Lithodesmium (Kooistra et al ., Citation2003; Medlin & Kaczmarska, Citation2004) that is evident from valve structure (von Stosch, Citation1977; Kaczmarska et al ., Citation2005). Other genera belonging in the same monophyletic clade are Bellerochea van Heurck and Helicotheca (Shrubsole) Ricard (18S rDNA evidence: Kooistra et al ., Citation2003; Medlin & Kaczmarska, Citation2004; morphology: Kaczmarska et al ., Citation2005) and Lithodesmioides von Stosch and Neostreptotheca von Stosch (morphological data: von Stosch, Citation1987). However, the oogonia of Bellerochea and Helicotheca produce only one egg (von Stosch, Citation1977). Two eggs are produced by some other multipolar diatoms, such as Odontella Agardh spp. (von Stosch, Citation1954, Citation1956; Drebes, Citation1974) and Attheya decora T. West (Drebes, Citation1977 a), but these eggs are retained within the separated halves of the parent frustule during fertilization. The patterns of oogenesis in centric diatoms are classified into three types (von Stosch & Drebes, Citation1964; Mizuno, Citation2006). Those producing two eggs per oogonium correspond to Type 1. Other centric diatoms produce only one egg per oogonium, which may (Type 2) or may not (Type 3) be accompanied by formation of a polar body at meiosis I.
The eggs we observed differed in their morphology from the ‘eggs’ reported in previous studies (Steele, Citation1967; Waite & Harrison, Citation1992). In those studies, cultures were subjected to various forms of nutrient limitation, which tend to cause changes in cell morphology and, in some species, can lead to vegetative cell enlargement (von Stosch, Citation1965). When marine diatoms are physiologically stressed by subjecting cultures to prolonged darkness (Gross, Citation1940 a) or nutrient limitation (Boleyn, Citation1972), the cytoplasm tends to contract, detach itself from the frustule and form a sphere within the cell. Plasmolysis produces similar effects (Gross, Citation1940 a, Citation b ; Pollock & Pickett-Heaps, Citation2005, Citation2006). Often, such cells are still viable and recover a normal morphology when returned to favourable growth conditions (Gross, Citation1940 a; Boleyn Citation1972; Pollock & Pickett-Heaps, Citation2005, Citation2006). Physiologically stressed cells may also extrude their protoplasts and these cells may be easily mistaken for sexual stages (e.g. those observed by Gross, Citation1937). Extruded protoplasts sometimes secrete new valves and enlarge vegetatively (von Stosch, Citation1965), providing an alternative size restitution mechanism to auxosporulation, though the increase in size seems always to be much less than during expansion of a sexually or asexually derived auxospore (e.g. Chepurnov & Mann, Citation1997). Thus, the multinucleate ‘eggs’ described by Steele (Citation1967, Figs ) may be reinterpreted as extruded protoplasts of stressed cells or stages in vegetative enlargement, because they (1) were found in senescent cultures, (2) formed ‘auxospores’ in the absence of sperm, and (3) developed into relatively small vegetative cells, approximately 40–70 µm in diameter versus 110 µm for the largest cells observed (Steele, Citation1967). The ‘egg cells’ reported from field samples by Waite & Harrison (Citation1992, ) resemble plasmolysed cells and were likely a result of physiological stress, not gametogenesis.
Vegetative enlargement was observed in our cultures, as in the clones studied by von Stosch (Citation1965, Citation1987). The main difference between the morphology of vegetatively enlarged cells and sexually derived cells was that the vegetatively enlarged cells no longer had the triradiate symmetry of cells produced by auxosporulation. The valve face and outline of the marginal fringe were oval or circular in vegetatively enlarged cells, as opposed to the triangular outline of initial cells. However, this distinction applies only to large cells: as cells become smaller they also become more rounded, whatever their original derivation. Similar contrasts between vegetatively enlarged cells and auxospores are seen in the related genera Lithodesmium, Bellerochea and Helicotheca: the diploid cells that undergo vegetative cell enlargement secrete numerous valves apparently haphazardly around a partially or fully extruded protoplasm, producing rounded cells, contrasting with the prismatic shape of post-auxospore cells (von Stosch, Citation1965). Size diminution was not inevitable in our Ditylum: sometimes, the new hypotheca was visibly larger than the epitheca (discussed also by Crawford, Citation1981). In late-stage cultures, cells with three valves were also observed following internal valve formation (cf. Pollock & Pickett-Heaps, Citation2006).
Spermatogenesis in our clones of D. brightwellii, and in the natural samples from Wadsworth Cove, was similar to that described for Lithodesmium undulatum Ehrenberg, where the 16 spermatogonia produced by mitosis undergo meiosis to produce 64 sperm (Manton & von Stosch, Citation1966; Manton et al ., Citation1969). By contrast, in the few other observations of spermatogenesis in Ditylum, spermatogonia produced 32 or 64 spermatogonia (Drebes, Citation1974, fig. 44b and p. 59; von Stosch, Citation1987, p. 62). This difference is probably genotypic rather than phenotypic (driven by size) because among the clones we studied several were close to the maximum size for gametogenesis. A few Gulf of Maine spermatogonangia were observed to have rudimentary valves separating the spermatogonia; similar structures occur in the spermatogonangia of some other multipolar diatom genera, such as Odontella (Drebes, Citation1974; Schmid, Citation1995) and Chaetoceros Ehrenberg (von Stosch et al ., Citation1973). However, rudimentary valves have not been reported previously in Ditylum (Drebes, Citation1974; von Stosch, Citation1987; although Drebes shows the spermatogonia in two groups, as in our material) or in the related genera Lithodesmium, Bellerochea or Helicotheca. The formation of these rudimentary valves appears to be a relic of vegetative mitosis and occurs after the first mitotic division differentiating the spermatogonia.
Mating system
True auxosporulation, identified as such by developmental and structural features, can occur without prior sexual reproduction in some centric diatoms (reviewed by Chepurnov et al ., Citation2004). Examples are known from Melosira Agardh (Drebes, Citation1977 b), Skeletonema Greville (Gallagher, Citation1983) and Coscinodiscus (Nagai et al ., Citation1995). In this connection, we must note that we did not actually observe fertilization in Ditylum. However, the correlation between simultaneous availability of male and female gametes and auxosporulation, and our observations of sperm close to egg cells, do suggest that auxosporulation was preceded by plasmogamy in the Gulf of Maine clones.
Ditylum brightwellii from the Gulf of Maine has strong gametogenic potential in culture (, clone N2). Clones are homothallic and self-compatible, but oogonia and spermatogonangia are not always produced in the same proportions. With rare exceptions (Drebes, Citation1968; Roshchin & Chepurnov, Citation1999), other centric diatoms are also homothallic and self-compatible (von Stosch, Citation1951, Citation1954, Citation1956, Citation1982; von Stosch & Drebes, Citation1964; Stosch et al ., 1973; Drebes, Citation1977 a, Citation b ; Idei & Chihara, Citation1992; Schmid, Citation1995; Roshchin & Chepurnov, Citation1999).
Cell size is the first order of control on gametogenesis in two ways. First, as in many other diatoms, cells that are larger than a critical cell size do not undergo sexual reproduction (reviewed by Drebes, Citation1977 b; Edlund & Stoermer, Citation1997; Chepurnov et al ., Citation2004). In our clones, this threshold for sexual reproduction was at c. 26 µm. Gametogenesis was rare or absent in clones N4, N3′ and D5, which were larger than this threshold size (). Second, among D. brightwellii cells that were gametogenic, the small cells tended to produce sperm, whereas larger cells produced mostly eggs; intermediate-sized cells produced both types of gamete. This pattern was first documented in the chain-forming centric diatom Melosira varians C. Agardh and in the multipolar genus Odontella (von Stosch, Citation1951, Citation1956). Size-selective gametogenesis may provide a mechanism of control for homothallic and self-compatible diatoms to limit the amount of self-fertilization that occurs. It is also important that eggs are large enough to accommodate the energetic requirements of the cell during auxospore expansion (Davidovich, Citation1994), and for the silicification of the two initial cell thecae and their associated two mitotic divisions; however, the exact energetic pathways remain unknown (Zurzolo & Bowler, Citation2001). Consecutive formation of eggs, then sperm, requires explanation from a population biology perspective, because vigorous oogenesis could pre-empt the possibility of smaller, ‘male’ cells being formed through size reduction (cf. Chepurnov et al ., Citation2004, p. 114). There are at least three ways in which populations could assure the simultaneous availability of sperm and eggs. The first would be for there to be some intrinsic mechanism preventing mass egg production (e.g. if there was a negative feedback on oogenesis mediated by inhibitory chemicals secreted by differentiating oogonia). In this connection, it is interesting to note that, in our cultures, maximum rates of oogenesis were rarely as great as the maximum rate of spermatogenesis. The second would be for oogenesis to be stimulated by spermatogenesis, via some kind of chemical messenger, so that small cells would have to be present before larger cells began to differentiate. The third would involve restriction of the time window for sexual reproduction, relative to the rate of cell division (and hence size reduction), so that a significant proportion of the population would pass through the ‘female’ phase of the life cycle during times of the year when sexual induction is absent.
Aside from control of the male–female ratio, cell-to-cell signalling could be beneficial in a variable environment where the time frame for sex may be short and dependent on environmental cues. Our data hint at the existence of a system of chemical cues that co-ordinates synchrony of gametogenesis and gametangium dehiscence between potential mates. In culture, mixing the two gametogenic clones N2 and N3 (which were predominantly sperm- and egg-producing, respectively) advanced gametogenesis and increased the frequency of auxosporulation over that occurring in either of the intraclonal treatments (Figs , , , ). This suggests signalling occurs, possibly involving pheromones in clones actively producing gametes. Large clones that did not produce gametes were not induced to do so in the presence of smaller gamete-producing clones (Figs , ). Because D. brightwellii has external fertilization (both eggs and sperm are released from the thecae), a pheromone that induces chemotaxis, such as ectocarpene (effective for this in some brown algae: Maier & Müller, Citation1986), could optimize encounter rates between eggs and sperm (Dusenbery, Citation2000). Ectocarpene has been isolated from egg-producing cultures of the related diatom Lithodesmium undulatum Ehrenberg and also in the more distantly related diatom Skeletonema costatum (Greville) Cleve (Derenbach & Pesando, Citation1986; clade nomenclature from Medlin & Kaczmarska, Citation2004), suggesting that pheromone production could be widespread in centric diatoms (see also Chepurnov et al ., Citation2004, p. 123).
Sexual reproduction and size changes in natural populations
Information on the frequency of sexual events in natural populations of diatoms is practically non-existent. However, some inferences can be made from the size distributions of cells (e.g. Nipkow, Citation1927; Mann, Citation1988; Jewson, Citation1992 a) and the presence of gametangia in natural populations.
The abundance of D. brightwellii was extremely variable from week to week in Wadsworth Cove during late summer and autumn 2004 (), perhaps indicating that we were sampling different water masses passing through the same geographical location. The presence of spermatogonangia was independent of population abundance and their frequency varied from 0 to 2.4%. Similar percentages were observed by Waite & Harrison (Citation1992) in much denser natural populations, ∼20,000 cells l−1. Steele (Citation1967) reported 5–15% of the cells within a population to be sexual, but this may be an over-estimate because it is unclear whether misidentified “eggs” were included in the tally of “fertile cells” (Steele Citation1967, pp. 14 and 77, ). Although clones isolated from the Gulf of Maine are homothallic in culture, they may not self-fertilize in nature. Self-fertilization may simply be a ‘bet hedging’ strategy, ensuring that maximum numbers of progeny result once gametogenesis is induced (Stebbins, Citation1957). Alternatively, the population may be so diverse and well mixed that most egg-sperm encounters are between distantly related genotypes. Recent studies on the population genetics of D. brightwellii and P. pungens have shown large numbers of unique genotypes, indicating that recombination must occur in nature, even though sexual stages have not been detected directly in natural populations (Rynearson & Armbrust, Citation2000, Citation2004, Citation2005; Evans et al ., Citation2005). Heterozygote deficiency in Puget Sound populations of D. brightwellii (Rynearson & Armbrust, Citation2000, Citation2004, Citation2005) may be explained by self-fertilization producing an excess of homozygous genotypes and this would be consistent with our observations of intraclonal auxosporulation; however, null alleles detected in the microsatellite analysis may produce the same result (Rynearson et al ., Citation2006). On the other hand, the North Sea population of the pennate diatom P. pungens is in Hardy–Weinberg equilibrium (Evans et al ., Citation2005), indicating random mating, and thus outcrossing. In contrast to D. brightwellii, selfing is impossible in P. pungens because it is heterothallic (Chepurnov et al ., Citation2005), like its close relatives P. multiseries (Hasle) Hasle and P. delicatissima Hasle (Davidovich & Bates, Citation1998; Hiltz et al ., Citation2000; Amato et al ., Citation2005).
The presence of spermatogonangia alone in a natural population does not mean that sexual reproduction is occurring and there is evidence that some populations of D. brightwellii are essentially asexual, despite the formation of sperm. von Stosch (Citation1987) concluded that most or all Ditylum in the North Sea and Narragansett Bay did not undergo auxosporulation, because he did not observe any large, multipolar cells. Thus these populations are asexual, size restitution taking place only through vegetative cell enlargement. It is possible that D. brightwellii is not a single species but a complex of cryptic or semi-cryptic species, like the Skeletonema taxa recently studied by Sarno et al ., (Citation2005) and other species reviewed by Mann (Citation1999). Our data on cell dimensions support this idea. Because our study included clones that were close to the size threshold for sexual reproduction (c. 26 µm), it is likely that the 71 µm diameter auxospores we observed are close to the maximum size for the local population of D. brightwellii. By contrast, Drebes (Citation1974) gave a maximum of 100 µm for North Sea populations, and von Stosch (Citation1987) recorded 88 µm diameter cells from Australia. Maximum cell sizes in Puget Sound, WA appear to between 95 and 100 µm (Rynearson et al ., Citation2006).
The frequency of sampling in our study of natural populations was greater than the longevity of gametes and auxospores (about 24 h for each); therefore, the size structure of populations may be used to infer the occurrence of auxosporulation. Interpretation is complicated by the differential effects of other factors, including the possibility that the rate of size reduction may vary during the life cycle (through changes in the decrement per division, changes in growth rate, or both); parasitism (Mann, Citation1988; Jewson, Citation1992 a); selective grazing (Naustvoll, Citation2000); the length of time before a single valve, having experienced multiple rounds of cell division, succumbs to damage and dissolution (Jewson, Citation1992 b); apoptosis; changes in buoyancy and sedimentation (Boyd & Gradmann, Citation2002); and resuspension of cells from the sediments (Jewson, Citation1992 a). If all these factors remain constant, a population undergoing sexual reproduction continuously (Chepurnov et al ., Citation2004) will have a size distribution that is skewed to the right, with a long tail extending up to the sizes of the initial cells (Mann, Citation1988). This is because of the short-term cost of sexual reproduction (Mann Citation1988, p. 404) due to (1) superfluous and aborted gametes and zygotes, (2) the formation of deleterious new genotypes, and (3) the interruption to vegetative growth caused by gametogenesis and auxospore development (Lewis, Citation1983). This cost can be demonstrated visually using results from clones N2 and N4 and the interclonal cross N2 × N4 (). Spermatogenesis and auxosporulation was extensive in N2, and few small vegetative cells remained after five days (, N2 × N2), the culture being dominated by large post-auxospore cells. N4, on the other hand, produced few gametes and the size spectrum did not change after 5 days (, N4 × N4). In mixtures of the two (, N2 × N4), N4 continued to divide vegetatively at a high rate (twice a day, incurring an eight-fold penalty in lost mitotic divisions for any N2 cells undergoing the 2-day process of auxosporulation) while N2 cells were engaged in gametogenesis and auxosporulation, leading to overwhelming dominance (>95%) of N4 after 5 days.
There is evidence that sexual reproduction is episodic in some diatoms (reviewed by Edlund & Stoermer Citation1997); large cells enter the population at discrete intervals and create separate modes in the size distribution. Again, however, because of the short-term costs of sexual reproduction, the magnitude of a mode of large cells is not necessarily indicative of the magnitude of the preceding gametogenic events within a population. For example, Corethron pennatum (Grunow) Ostenfeld is capable of high levels of gametogenesis (as C. criophilum, Crawford, Citation1995), but relatively few large cells subsequently entered the population (Crawford et al ., Citation1997). Episodic sexual reproduction was inferred for the pennate diatoms Nitzschia sigmoidea (Nitzsch) W. Smith, Tabellaria fenestrata (Lyngbye) Kützing, Asterionella gracillima (Hantzsch) Heiberg, and Fragilaria crotonensis Kitton (Schröter & Vögler, Citation1901; Nipkow, Citation1927; Mann, Citation1988). The size spectra of some N. sigmoidea populations exhibit several peaks that can be interpreted as having arisen through successive, annual bouts of (sexual) auxosporulation (Mann, Citation1988).
There was no convincing evidence of multimodality in the Wadsworth Cove Ditylum population in 2004 and so, even if sexual reproduction is episodic, it left no obvious trace in the size distribution. A few large cells of D. brightwellii, similar in valve width to the initial cells obtained in laboratory experiments, appeared in mid-autumn. At the same time, gametogenesis may have contributed to the loss of cells in the smaller size classes. The consequence was a significant (Kolmogorov–Smirnoff Test; p < 0.01) shift to the right in the size distribution (), but without the formation of a noticeable peak of post-auxospore cells. Large cells disappeared by late autumn. A similar trend was observed for the benthic pennate Diatoma moniliformis Kützing, in the Baltic Sea, which reproduces sexually in the spring without subsequent development of a major mode of large cells (Potapova & Snoeijs, Citation1997). Few centric diatoms have been studied in the same way, a rare exception being the freshwater planktonic diatom Stephanodiscus Ehrenberg (Nipkow, Citation1927; Round, Citation1982; Jewson, Citation1992 b). In one study, large cells became the dominant size class of cells within seven weeks of their first appearance in early October (Round, Citation1982). By contrast, smaller cells dominated D. brightwellii populations throughout our 15-week study period, reflecting different sexual dynamics. In both species, however, the rate of decline in mean cell-size and changes in the size structure of the population slowed or stopped as winter approached. This implies a reduction in the true growth rate (responsible for the lack of size reduction) and also the cessation of auxosporulation during winter and the absence of size-selective loss processes, such as grazing.
Temperate diatoms, such as D. brightwellii, encounter large seasonal variations in environmental conditions that will directly affect their growth rates. The rate of size reduction will also vary seasonally (Margalef, Citation1969; Mizuno & Okuda, Citation1985; Jewson, Citation1992 b), with an extra complication, the rate of reduction per division is sometimes proportional to cell size (e.g. Jewson, Citation1992 a; Amato et al ., Citation2005). Therefore, cell size and environmental conditions interact to trigger sexual reproduction in these diatoms. Further studies will be required to determine which external cues induce sexual reproduction in D. brightwellii. However, spermatogenesis seems primarily to be a function of cell size because it was observed in cultures subjected to simultaneous high light and nutrient limitation (Waite & Harrison, Citation1992), as well as moderate light and nutrient-replete conditions (Steele, Citation1967). Changes in temperature may still be important in inducing spermatogenesis. On the other hand, although spermatogonangia occur in natural populations of D. brightwellii during the late summer and early autumn in the North Atlantic and Pacific Northwest (Steele, Citation1967; Drebes, Citation1974; von Stosch, Citation1987; Waite & Harrison, Citation1992; this study), they were not found during an intensively studied spring bloom in Puget Sound (Rynearson & Armbrust, Citation2005). Spermatogonangia also seemed to disappear from the Gulf of Maine population in November and early December, even though large proportions of the populations were within the inducible size range. The consistency with which sexual reproduction occurs in the autumn in D. brightwellii is striking, given the differences in seasonality and cell abundance between the sites sampled in the Atlantic and Pacific. Suitable conditions for sexual reproduction in D. brightwellii seem therefore to be any or all of the following: increased nutrients due to mixing, medium to short photoperiod (between 13 and 10 h of daylight), and moderate temperature (between 10 and 17°C). Further characterization of spring blooms could help determine whether these cues are absolute, or if the diatoms are responding to directional changes, viz. decreasing light and/or temperature.
Acknowledgements
Financial support for this research was provided by NSF OCE-99043 to Susan Brawley, and by a Phycological Society of America grant-in-aid of research to Julie Koester. We thank Dr Robert Vadas for discussion throughout the research, Molly Harris for laboratory assistance and Dr Tatiana Rynearson for thoughtful insights. Special thanks to Jessica Muhlin and Nicolas Blouin for laboratory support.
References
- Amato , A , Orsini , L , D’Alelio , D and Montresor , M . 2005 . Life cycle, size reduction patterns, and ultrastructure of the pennate planktonic diatom Pseudo-nitzschia delicatissima (Bacillariophyceae) . J. Phycol. , 41 : 542 – 556 .
- Boleyn , BJ . 1972 . Silica availability and the formation of dysplastic cells in cultures of Ditylum brightwellii . Arch. Mikrobiol. , 81 : 169 – 177 .
- Boyd , CM and Gradmann , D . 2002 . Impact of osmolytes on buoyancy of marine phytoplankton . Mar. Biol. , 141 : 605 – 618 .
- Chepurnov , VA and Mann , DG . 1997 . Variation in the sexual behavior of Achnanthes longipes (Bacillariophyta) . Eur. J. Phycol. , 32 : 147 – 154 .
- Chepurnov , VA , Mann , DG , Sabbe , K and Vyverman , W . 2004 . Experimental studies on sexual reproduction in diatoms . Int. Rev. Cytol. , 237 : 91 – 154 .
- Chepurnov , VA , Mann , DG , Sabbe , K , Vannerum , K , Casteleyn , G , Verleyen , E , Peperzak , L and Vyverman , W . 2005 . Sexual reproduction, mating system, chloroplast dynamics and abrupt cell size reduction in Pseudo-nitzschia pungens from the North Sea (Bacillariophyta) . Eur. J. Phycol. , 40 : 379 – 395 .
- Crawford , RM . 1981 . “ Some considerations of size reduction in diatom cell walls ” . In Proceedings of the 6th Symposium on Recent and Fossil Diatoms , Edited by: Ross , R . 253 – 265 . Koenigstein, , Germany : O. Koeltz .
- Crawford , RM . 1995 . The role of sex in the sedimentation of a marine diatom bloom . Limnol. Oceanogr. , 40 : 200 – 204 .
- Crawford , RM , Hinz , F and Rynearson , T . 1997 . Spatial and temporal distribution of assemblages of the diatom Corethron criophilum in the Polar Frontal region of the South Atlantic . Deep-Sea Res. II , 44 : 479 – 496 .
- Davidovich , NA . 1994 . Factors controlling the size of initial cells in diatoms . Russ. J. Plant Physiol. , 41 : 220 – 224 .
- Davidovich , NA and Bates , SS . 1998 . Sexual reproduction in the pennate diatoms Pseudo-nitzschia multiseries and P. pseudodelicatissima (Bacillariophyceae) . J. Phycol. , 34 : 126 – 137 .
- Derenbach , JB and Pesando , D . 1986 . Investigations into a small fraction of volatile hydrocarbons . Mar. Chem. , 19 : 337 – 341 .
- Drebes , G . 1968 . Subdiözie bei der zentrischen Diatomee Coscinodiscus granii . Naturwissenschaften , 55 : 236
- Drebes , G . 1974 . Marines Phytoplankton. Eine Auswahl der Helgoländer Planktonalgen (Diatomeen, Peridineen) , Stuttgart, , Germany : Thieme .
- Drebes , G . 1977a . Cell structure, cell division, and sexual reproduction of Attheya decora West (Bacillariophyceae, Biddulphiineae) . Nova Hedwig. Beih. , 54 : 167 – 178 .
- Drebes , G . 1977b . “ Sexuality ” . In The Biology of Diatoms , Edited by: Werner , D . 250 – 283 . Oxford, UK : Blackwell Scientific Publications .
- Dusenbery , DB . 2000 . Selection for high gamete encounter rates explains the success of male and female mating types . J. Theor. Biol. , 202 : 1 – 10 .
- Edlund , MB and Stoermer , EF . 1997 . Ecological, evolutionary, and systematic significance of diatom life histories . J. Phycol. , 33 : 897 – 918 .
- Eppley , RW , Holmes , RW and Paasche , E . 1967 . Periodicity in cell division and physiological behavior of Ditylum brightwellii, a marine planktonic diatom, during growth in light-dark cycles . Arch. Mikrobiol. , 56 : 305 – 323 .
- Evans , KM , Kühn , SF and Hayes , PK . 2005 . High levels of genetic diversity and low levels of genetic differentiation in North Sea Pseudo-nitzschia pungens (Bacillariophyceae) populations . J. Phycol. , 41 : 506 – 514 .
- Falkowski , PG . 1975 . Nitrate uptake in marine pytoplankton: Comparison of half-saturation constants from seven species . Limnol. Oceanogr. , 20 : 412 – 417 .
- Fryxell , GA , Garza , SA and Roelke , DL . 1991 . Auxospore formation in an Antarctic clone of Nitzschia subcurvata Hasle . Diatom Research , 6 : 235 – 245 .
- Gallagher , JC . 1983 . Cell enlargement in Skeletonema costatum (Bacillariophyceae) . J. Phycol. , 19 : 539 – 542 .
- Gross , F . 1937 . The life history of some marine plankton organisms . Phil. Trans. R. Soc. Lond. B , 228 : 1 – 47 .
- Gross , F . 1940a . The osmotic relations of the plankton diatom Ditylum brightwellii (West) . J. Mar. Biol. Ass. UK , 24 : 381 – 415 .
- Gross , F . 1940b . The development of isolated resting spores into auxospores in Ditylum brightwellii (West.) Gran . Mar. Biol. Ass. UK , 24 : 375 – 380 .
- Guillard , RRL and Ryther , JH . 1962 . Studies of marine plankton diatoms. I. Cyclotella nana Hustedt, and Detonula confervacea (Cleve) Gran . Can. J. Microbiol. , 8 : 229 – 239 .
- Hargraves , PE . 1984 . “ Resting spore formation in the marine diatom Ditylum brightwellii(West) Grun. ex V. H. ” . In Proceedings of the 7th International Diatom Symposium , Edited by: Mann , DG . 33 – 46 . Koenigstein, Germany : Otto Koeltz .
- Hiltz , M , Bates , SS and Kaczmarska , I . 2000 . Effect of light:dark cycles and cell apical length on the sexual reproduction of a pennate diatom Pseudo-nitzschia multiseries (Bacillariophyceae) in culture . Phycologia , 39 : 59 – 66 .
- Idei , M and Chihara , M . 1992 . Successive observations on the fertilization of a centric diatom Melosira moniliformis var octagona . Bot. Mag. Tokyo , 105 : 649 – 658 .
- Jewson , DH . 1992a . Size reduction, reproductive strategy and the life cycle of a centric diatom . Phil. Trans. R. Soc. Lond. B , 336 : 191 – 213 .
- Jewson , DH . 1992b . Life cycle of a Stephanodiscus sp. (Bacillariophyta) . J. Phycol. , 28 : 856 – 866 .
- Kaczmarska , I , Beaton , M , Benoit , AC and Medlin , LK . 2005 . Molecular phylogeny of selected members of the order Thalassiosirales (Bacillariophyta) and evolution of the fultoportula . J. Phycol. , 42 : 121 – 138 .
- Karsten , G . 1899 . Die Diatomeen der Kieler Bucht . Helgol. wiss. Meeresunters. , 4 : 17 – 205 .
- Kooistra , WHCF , De Stefano , M , Mann , DG and Medlin , LK . 2003 . The phylogeny of diatoms . Progr. Mol. Subcell. Biol. , 33 : 59 – 97 .
- Lee , M and Li , C-W . 1992 . The origin of the silica deposition vesicle of diatoms . Bot. Bull. Acad. Sin. , 33 : 317 – 325 .
- Lewis , WM . 1983 . Interruption of synthesis as a cost of sex in small organisms . Am. Nat. , 121 : 825 – 834 .
- Li , C-W and Volcani , BE . 1984 . Aspects of silicification in wall morphogenesis of diatoms . Phil. Trans. R. Soc. Lond. B , 304 : 519 – 528 .
- Li , C-W and Volcani , BE . 1985 . Studies on the biochemistry and fine structure of silica shell formation in diatoms VIII. Morphogenesis of the cell wall in a centric diatom . Ditylum brightwellii. Protoplasma , 124 : 10 – 29 .
- Li , C-W , Chu , S and Lee , M . 1989 . Characterizing the silica deposition vesicle of diatoms . Protoplasma , 151 : 158 – 163 .
- Maier , I and Müller , DG . 1986 . Sexual pheromones in algae . Biol. Bull. , 170 : 145 – 175 .
- Mann , DG . 1988 . “ Why didn't Lund see sex in Asterionella? A discussion of the diatom life cycle in nature ” . In Algae and the aquatic environment , Edited by: Round , FE . 383 – 412 . Bristol, UK : Biopress .
- Mann , DG . 1999 . The species concept in diatoms . Phycologia , 38 : 437 – 495 .
- Manton , I and von Stosch , HA . 1966 . Observations on the fine structure of the male gamete of the marine centric diatom Lithodesmium undulatum . J. Roy. Microsc. Soc. , 85 : 119 – 134 .
- Manton , I , Kowallik , K and von Stosch , HA . 1969 . Observations on the fine structure and development of the spindle at mitosis and meiosis in a marine centric diatom (Lithodesmium undulatum). II. The early meiotic stages in male gametogenesis . J. Cell Sci. , 5 : 271 – 298 .
- Margalef , R . 1969 . Size of centric diatoms as an ecological indicator . Mitt. Int. Ver. Theor. Angew. Limnol. , 17 : 202 – 210 .
- Medlin , LK and Kaczmarska , I . 2004 . Evolution of the diatoms: V. Morphological and cytological support for the major clades and taxonomic revision . Phycologia , 43 : 245 – 270 .
- Mizuno , M . 2006 . Evolution of meiotic patterns of oogenesis and spermatogenesis in centric diatoms . Phycol. Res. , 54 : 57 – 64 .
- Mizuno , M and Okuda , K . 1985 . Seasonal changes in the distribution of cell size of Cocconeis scutellum var. ornata (Bacillariophyceae) in relation to growth and sexual reproduction . J. Phycol. , 21 : 547 – 553 .
- Nagai , S , Hori , Y , Manabe , T and Imai , I . 1995 . Restoration of cell size by vegetative cell enlargement in Coscinodiscus wailesii (Bacillariophyceae) . Phycologia , 34 : 533 – 535 .
- Naustvoll , L-J . 2000 . Prey size spectra and food preferences in thecate heterotrophic dinoflagellates . Phycologia , 39 : 187 – 198 .
- Nipkow , F . 1927 . Über das Verhalten der Skelette planktischer Kieselalgen im geschichteten Tiefschlamm des Zürich-und Baldeggersee . Z. Hydrol. Hydrogr. Hydrobiol. , 4 : 71 – 120 .
- Pickett-Heaps , JD and Pickett-Heaps , J . 2003 . Diatoms: Life in Glass Houses (videotape and commentary) , Sunderland, , USA : Sinauer Associates .
- Pickett-Heaps , JD , Wetherbee , R and Hill , DRA . 1988 . Cell division and morphogenesis of the labiate process in the centric diatom . Ditylum brightwellii. Protoplasma , 143 : 139 – 149 .
- Pollock , FM and Pickett-Heaps , JD . 2005 . Spatial determinants in morphogenesis: Recovery from plasmolysis in the diatom Ditylum . Cell Motil. Cytoskelet. , 60 : 71 – 82 .
- Pollock , FM and Pickett-Heaps , JD . 2006 . Valve formation without mitosis in the diatom Ditylum recovering from plasmolysis . Nova Hedwig. Beih. , 130 : 119 – 126 .
- Potapova , M and Snoeijs , P . 1997 . The natural life cycle in wild populations of Diatoma moniliformis (Bacillariophyceae) and its disruption in an aberrant environment . J. Phycol. , 33 : 924 – 937 .
- Roshchin , AM and Chepurnov , VA . 1999 . “ Dioecy and monoecy in the pennate diatoms (with reference to the centric taxa) ” . In Proceedings of the 14th International Diatom Symposium , Edited by: Mayama , M , Idei , M and Koizumi , I . 241 – 261 . Koenigstein, , Germany : Koeltz Scientific Books .
- Round , FE . 1982 . Auxospore structure, initial valves and the development of populations of Stephanopyxis in Farmoor Reservoir . Ann. Bot. , 49 : 447 – 459 .
- Rynearson , TA and Armbrust , EV . 2000 . DNA fingerprinting reveals extensive genetic diversity in a field population of the centric diatom Ditylum brightwellii . Limnol. Oceanogr. , 45 : 1329 – 1340 .
- Rynearson , TA and Armbrust , EV . 2004 . Genetic differentiation among populations of the planktonic marine diatom Ditylum brightwellii (Bacillariophyceae) . J. Phycol. , 40 : 34 – 43 .
- Rynearson , TA and Armbrust , EV . 2005 . Maintenance of clonal diversity during a spring bloom of the centric diatom Ditylum brightwellii . Mol. Ecol. , 14 : 1631 – 1640 .
- Rynearson , TA , Newton , JA and Armbrust , EV . 2006 . Spring bloom development, genetic variation, and population succession in the planktonic diatom Ditylum brightwellii . Limnol. Oceanogr. , 51 : 1249 – 1261 .
- Sarno , D , Kooistra , WHCF , Medlin , LK , Percopo , I and Zingone , A . 2005 . Diversity in the genus Skeletonema (Bacillariophyceae). II. An assessment of the taxonomy of S. costatum-like species with the description of four new species . J. Phycol. , 41 : 151 – 176 .
- Schmid , A.-MM . 1995 . “ Sexual reproduction in Coscinodiscus granii Gough in culture: A preliminary report ” . In Proceedings of the 13th International Diatom Symposium , Edited by: Marino , D and Montresor , M . 139 – 159 . Bristol, , UK : Biopress .
- Schröter , C and Vögler , P . 1901 . Variationsstatistische Untersuchung über Fragilaria crotonensis (Edw.) Kitton im Plankton des Zürichsees in den Jahren 1896–1901 . Vierteljahrsschr. Naturforsch. Ges. Zürich , 46 : 185 – 206 .
- Stebbins , GL . 1957 . Self-fertilization and population variability in the higher plants . Am. Nat. , 91 : 337 – 354 .
- Steele , RL . 1967 . Induction of sexuality in two marine centric diatoms: Ditylum brightwellii (West) Grunow and Stephanopyxis palmeriana (Grev.) Grunow , Seattle, , USA : Ph. D. Dissertation. University of Washington .
- Utermöhl , H . 1931 . Neue Wege in der quantitativen Erfassung des Planktons. (Mit besonderer Berücksichtigung des Ultraplanktons.) . Verh. Int. Ges. theor. angew. Limnol. , 5 : 567 – 596 .
- Venrick , EL . 1986 . The Smirnov statistic: an incorrect test for vertical distribution patterns . Deep Sea Res. , 33 : 1275 – 1277 .
- von Stosch , HA . 1951 . Entwicklungsgeschichtliche Untersuchungen an zentrischen Diatomeen I. Die Auxosporenbildung von Melosira varians . Arch. Mikrobiol. , 16 : 101 – 135 .
- von Stosch , HA . 1954 . Die Oogamie von Biddulphia mobiliensis und die bisher bekannten Auxosporenbildungen bei den Centrales . Rapp. Comm. 8 Congr. Int Bot. , 17 : 58 – 68 .
- von Stosch , HA . 1956 . Entwicklungsgeschichtliche Untersuchungen an zentrischen Diatomeen II. Geschlechtszellenreifung, Befruchtung und Auxosporenbildung einiger grundbewohnender Biddulphiaceen der Nordsee . Arch. Mikrobiol. , 23 : 327 – 365 .
- von Stosch , HA . 1965 . Manipulierung der Zellgrösse von Diatomeen im Experiment . Phycologia , 5 : 21 – 44 .
- von Stosch , HA . 1977 . Observations on Bellerochea and Streptotheca, including descriptions of three new planktonic diatom species . Nova Hedwig. Beih. , 54 : 113 – 166 .
- von Stosch , HA . 1982 . On auxospore envelopes in diatoms . Bacillaria , 5 : 127 – 156 .
- von Stosch , HA . 1987 . Some marine diatoms from the Australian region, especially from Port Phillip Bay and tropical north-eastern Australia. II. Survey of the genus Palmeria and of the family Lithodesmiaceae including the new genus Lithodesmioides . Brunonia , 9 : 29 – 87 .
- von Stosch , HA and Drebes , G . 1964 . Entwicklungsgeschichtliche Untersuchungen an zentrischen Diatomeen IV. Die Planktondiatomee Stephanopyxis turris – ihre Behandlung und Entwicklungsgeschichte . Helgol. wiss. Meeresunters. , 11 : 209 – 257 .
- von Stosch , HA , Theil , G and Kowallik , K . 1973 . Entwicklungsgeschichtliche Untersuchungen an zentrischen Diatomeen V. Bau und Lebenszyklus von Chaetoceros didymum, mit Beobachtungen übereinige anderen Arten der Gattung . Helgol. wiss. Meeresunters. , 25 : 384 – 445 .
- Waite , A and Harrison , PJ . 1992 . Role of sinking and ascent during sexual reproduction in the marine diatom Ditylum brightwellii . Mar. Ecol. Prog. Ser. , 87 : 113 – 122 .
- Zurzolo , C and Bowler , C . 2001 . Exploring bioinorganic pattern formation in diatoms. A story of polarized trafficking . Plant Physiol. , 127 : 1339 – 1345 .