Abstract
Over the winter many phytoplankton in deep lakes encounter prolonged periods of dark and cold. Survival times of the planktonic cyanobacterium Planktothrix rubescens were assessed by isolating single filaments of six strains in separate tubes that were stored at 4–5° C in the dark for 2–16 weeks and then incubating them at 20° C in the light. Viable filaments grew to produce cultures, usually within 3–5 weeks. Filaments survived for longer in isolation than they did in samples of cultures containing many filaments. After 8 weeks in the cold and dark, five of the Planktothrix strains had viable filaments, with 14–67% of filaments sampled surviving. In the most long-lived of these, Planktothrix strain 9736, 50% of the isolated filaments survived for 10 weeks and the maximum survival time exceeded 11 weeks. Combining this information with information on filament floating velocities, it is calculated that filaments would float up 25 m before half of them lost viability. Such calculations can be used in estimating the proportion of the lake population that can be recruited to the spring and summer populations in the metalimnion.
Introduction
In winter, most temperate lakes are so weakly stratified that they become frequently mixed (Mortimer, Citation1974; Goudsmit et al., Citation2002). The deeper the lake, the greater is the probability that a phytoplankton cell will spend time in darkness; many cells may receive insufficient light for maintenance or growth (Walsby et al., Citation2006). The number of cells remaining viable at the end of winter will depend on the number that survived the time spent in darkness and the number that received sufficient light by remaining in or revisiting the surface layers. Colonies of the cyanobacterium Microcystis aeruginosa are known to over-winter in lake sediments (Fallon & Brock, Citation1981; Oliver et al., Citation1985), though survival may be restricted to only a few cells in each colony (Reynolds et al., Citation1981). Information exists on the lower limits of irradiance required for growth of phytoplankton but there is little information on survival times in the dark. In the studies that have been made into the decline of cell viability in the dark, (Fallon & Brock, Citation1979, Berges & Falkowski, Citation1998; Popels & Hutchins, Citation2002; and Popels et al., Citation2007), the investigations were terminated within 1–4 weeks, before all of the cells had died.
We have investigated the survival in darkness at low temperature of several strains of the filamentous cyanobacterium Planktothrix rubescens (Anagnostidis & Komàrek, 1988) isolated from Lake Zürich. The observations are relevant to the over-winter survival of the cyanobacterium and its recruitment from deep layers during the subsequent summer. Lake Zürich, a large subalpine Swiss lake (mean depth 50 m, maximum 136 m), is thermally stratified from spring to autumn (Kutschke, Citation1966; Bossard et al., Citation2001; Livingstone, Citation2003). During the summer the population of P. rubescens stratifies at depths of 8–13 m (Thomas & Märki, Citation1949; Walsby & Schanz, Citation2002) where the irradiance supports phototrophic growth (Walsby et al., Citation1998; Micheletti et al., Citation1998; Walsby & Schanz, 2000). In autumn the population becomes entrained in the deepening epilimnion until it is circulated below the critical depth for growth (Walsby & Schanz, Citation2002). In cold years the water column may cool to 4° C and mix to its maximum depth.
The total Planktothrix biomass gradually declines after November, decreasing to one-third in late April (Walsby et al., Citation1998). Much of the decrease is explained by light limitation of growth (Bright & Walsby, Citation2000; Walsby & Schanz, Citation2002). Of the filaments that remain intact over winter, however, most are viable (Walsby et al., Citation1998; Beard et al., Citation1999). In mid-April the lake restratifies and filaments move towards the newly formed thermocline, establishing a new metalimnetic population, which decreases to a minimum in June. The summer population develops partly from growth of this surviving remnant and partly from filaments floating up from greater depths (Micheletti et al., Citation1998; Bright & Walsby, Citation2000; Walsby & Schanz, Citation2002). Based on theoretical and measured floating velocities of P. rubescens (Walsby & Holland, Citation2006), it is calculated that an average-sized filament must start from depths <45 m to reach the metalimnion by mid-July; those starting from >70 m would not reach the metalimnion before the population became entrained in autumn (Walsby, Citation2005). Whether these filaments make a contribution to the metalimnetic population depends on whether they remain viable for the time they take to float up. Planktothrix spp. do not produce akinetes and survival must depend on the vegetative filaments.
Planktothrix spp. grow at low temperatures and low photosynthetically active irradiances (Foy et al., Citation1976; Davis & Walsby, Citation2002) with a minimum daily photon insolation of <0.1 mol m−2 per day for growth (Bright & Walsby, Citation2000). At lower insolations, filaments eventually die. Filaments will survive for a certain period, however, even in total darkness. Gibson (Citation1975) showed that the respiration rate of Planktothrix filaments gradually decreased over 7 days in the dark, perhaps partly due to depletion of carbohydrate stores and partly due to loss of viability. We have investigated survival of filaments in darkness at 4–5° C, the conditions prevailing at depths exceeding 20 m during winter, when the filaments may be mixed to depths >80 m (Walsby et al., Citation1998), and during the spring, when they are floating up from these depths to join the population in the metalimnion. Our investigation developed from studies by Bright (Citation1999) who showed that 1-ml samples from cultures stored in the cold and dark for up to 4 months grew after incubation in the light at 20° C. This revealed the maximum survival time and not the proportion of filaments that survived: in the extreme case growth might have occurred from a single filament out of thousands in the sample.
Materials and methods
Cultures
The six strains of P. rubescens investigated were isolated from Lake Zürich between 1993 and 1997 (Beard et al., Citation1999) and maintained in the Bristol Cyanobacterial Collection (). The strains included representatives of the three main gas vesicle genotypes in the lake, GV1, GV2 and GV3. The cultures were grown in the medium of Bright & Walsby (Citation2000) using 250 ml Erlenmeyer flasks illuminated from below by Fitzgerald F8W/W white fluorescent tubes with diffusion covers, at an incident photon irradiance within the range of 10 to 55 µmol m−2 s−1, housed in modified Qualicool incubators at 20° C.
Table 1. Percentage viability of 36 individual filaments of each of six strains of Planktothrix after incubation in the cold and dark for 58 days. The calculated maximum survival time (in weeks) is also shown (see text)
Setting up single-filament cultures
From a single drop of culture on a sterile Petri dish lid, observed with a Leica dissecting microscope at 40 × magnification, a small number of filaments were transferred to a drop of fresh culture medium using a 50 µl Terumo or SGM microsyringe. From this diluted suspension an individual filament was transferred to a separate drop of fresh medium. The microsyringe was rinsed twice, part filled with 10 µl of medium and then used to draw up the filament and transfer it to a 15-ml glass culture tube containing 5 ml of medium. The viability of the filament in each tube was determined by incubating it for up to three months at 20° C in incident photon irradiances of 10–55 µmol m−2 s−1, and to provide more even conditions, the positions of the tubes were interchanged twice weekly. At weekly intervals the tubes were inspected for filament growth. Growth was usually first detected after 3 weeks and in most viable cultures by 4 weeks.
It did not prove possible to make viable counts of Planktothrix filaments by the plate count method (Madigan et al., Citation2003) because, when plated on 0.7% agar, the filaments spread over the plate by gliding without forming discrete colonies that could be enumerated.
Survival in dark and cold
The inoculated culture tubes were double wrapped in aluminium foil and placed in the dark in a refrigerator at 4° C. Survival of filaments was monitored by three different methods.
(A) Filament-population cultures
In the first set of experiments with Planktothrix strain 9736, 15 culture tubes, each containing 5 ml of medium, were inoculated with 1 ml of well-grown culture and then incubated in the dark and cold. At the start of the experiment, and at intervals of 2 or 3 weeks thereafter, one or more tubes was removed and the viability was assessed by isolating and culturing 15 to 36 filaments as described above. Unopened tubes were sampled after each interval to ensure that the cultures were kept in complete darkness. The same procedure was followed with Planktothrix strain 97112.
(B) Single-filament cultures
In subsequent experiments, 216 culture tubes with 5 ml of medium were each inoculated with a single filament of P. rubescens, 36 tubes for each of the six different strains shown in . The tubes were incubated in the cold and dark for 58 days, after which the viability of all samples was assessed by growth in the light at 20° C.
(C) Single-filament time course cultures
To investigate the progressive loss of viability, 288 culture tubes with 5 ml of medium were each inoculated with a single filament of Planktothrix strain 9736. The tubes were incubated for up to 113 days in the cold and dark and, at intervals of approximately 1 month, 72 tubes were transferred to light incubators at 20° C.
Results
Survival in filament-population cultures
After 16 days in the cold and dark, all of the single filaments taken from the culture tube of Planktothrix strain 9736 grew when transferred to 20° C in the light (Method A); when transferred after 34 days and 54 days, however, none of the filaments grew. After 88 days the remaining five tubes that had been stored in the cold and dark were placed in the light at 20° C; no growth was observed in any of them.
In the parallel experiment with Planktothrix strain 97112, cultures remained viable for longer, with 39% of filaments viable after 55 days, although the results indicated large variability.
In the first two samplings of both experiments, at time zero and after 16 days, there were ample numbers of filaments in the cultures and picking filaments for incubation was straightforward. In the third sampling (at 34 days) and subsequent samples, however, it became increasingly difficult to find filaments in the drops of suspension taken from the culture tubes. Many of the filaments had lysed or disintegrated while in the dark at 4° C. It became evident that the viability of the single filaments selected represented the viability of only those that remained intact and not of the whole population. This procedure was, therefore, abandoned.
Survival of different strains in single-filament cultures
Incubating single filaments separately in the cold and dark proved a better way of measuring the survival of Planktothrix cultures. A comparison of six different strains of Planktothrix (Method B) indicated wide variation in the percentage of filaments that remained viable after 58 days in the dark at 4° C (). No filaments grew in single filament cultures of Planktothrix strain 9307, whereas in those of Planktothrix strains 9736 and 97112 more than 50% remained viable.
Time course of survival in single-filament cultures
Information on the time course of viability loss was investigated using Planktothrix strain 9736, which had shown the highest proportion of surviving filaments (). After tubes containing single filaments had been incubated in the cold and dark for 34 days (Method C), growth occurred in 93% of those transferred to 20° C in the light (compared with 0% viability using Method A).
The viability decreased to 38% after 79 days and to 0% after 111 days; linear interpolation between 34 and 79 days () indicated 64% viability after 58 days, compared with the value of 66% observed after 58 days in the previous experiment. By a similar procedure it is calculated that 50% of the filaments would have died after 70 days. When a = 93%, b = 34 and c = 111 days, the mean% surviving for filaments sampled daily over the 111 days is given by integration: a[b + (c − b)/2]/c = 61%.
Fig. 1. Changes in percentage viability with time of individual filaments of Planktothrix strain 9736 after incubation in the cold and dark. Each datapoint is the percentage of the sample of 72 filaments, each incubated in a separate tube. The filled symbol is a result from a previous experiment in which the percentage survival of 36 filaments were measured after 58 days.
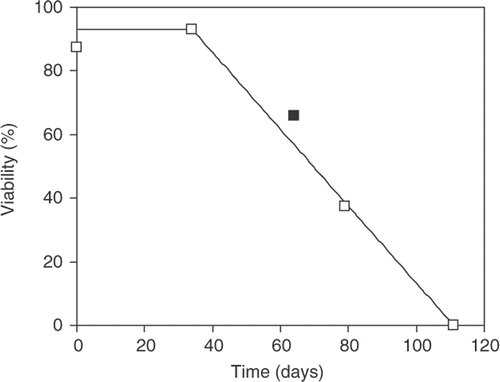
Discussion
There are several reasons why survival in cultures inoculated with many filaments might be worse than in those inoculated with single, isolated filaments: pathogenic viruses, bacteria, chytrids and fungal contaminants (Canter Citation1973; Stewart & Daft, Citation1976; Van Hannen et al., Citation1999) may spread more rapidly; dying cells may release toxins; surviving filaments may compete for light or nutrients. Whatever the cause, cultures are more easily established from single filaments than from clumps of material (Booker & Walsby, Citation1979; Walsby et al., Citation1998) and they also proved here to provide a better means of investigating filament survival. In Lake Zürich, after the Planktothrix population has been mixed throughout the depths, the filament concentration is about 20 ml−1 (Walsby et al., Citation1998), which is 100-fold more than in the initial single filament isolates (one in 5 ml) but 100-fold less than in well-grown cultures. There is no correspondence between the survival times of different strains stored in the cold and dark as single filaments rather than as cultures () and, as already commented, the growth observed by Bright (Citation1999) may be from very few surviving filaments. Similar problems may occur in investigations on other cyanobacteria, such as species of Microcystis investigated by Reynolds et al. (Citation1981) and Anabaena, Aphanizomenon, and Phormidium investigated by Fallon & Brock (Citation1979), who found that 99% of the biomass had died after only 8 days.
The combination of cold and darkness, in which survival of Planktothrix filaments was investigated, are relevant in the deeper layers of Lake Zürich, though in these natural waters survival may be prolonged by uptake of organic substances (Zotina et al., Citation2003; Walsby & Jüttner, Citation2006). During the winter period of deep mixing some of the filaments will, by chance, be circulated through the euphotic zone (Walsby et al., Citation2006) while others may spend months in continuous darkness where the ability to survive such conditions will be of selective value. In spring, part of the population forms the inoculum for the next season's growth. The filaments that are able to contribute are those left within floating distance of the metalimnion when the lake becomes thermally stratified again. Analysis of floating velocities has established the range of time taken for filaments from different depths to reach the metalimnion (Walsby, Citation2005); this information can now be combined with information on survival time for calculating the proportion of filaments that are still viable when they get there.
A result of such an analysis is given in , which shows the depth, z g, where the daily insolation supports zero growth rate, Q g. The value of Q g was shown by Bright & Walsby (Citation2000) to be 0.060 mol m−2. The daily position of z g was calculated from hourly measurements of photon irradiance at the lake surface and measurements of the vertical attenuation coefficient, contained in the data set of Walsby & Schanz (Citation2002); the line shown is actually the 3-day running mean to smooth out some of the irregularity in insolation owing to the variable cloud cover. For comparison the smoothed neutral buoyancy depth, z n, where the daily insolation Q n = 0.28 mol m−2 is shown; z n was usually within 1 m of the depth of the population maximum of P. rubescens in the metalimnion (Walsby et al., Citation2004).
Fig. 2. Plots of the neutral buoyancy depth (z n, upper line) where the daily insolation is 0.28 mol m−2 (data of Walsby et al., Citation2004) and the compensation depth for growth (z g, lower line) in Lake Zürich. The diagonal lines represent the trajectories of filaments floating up from various depths; the trajectories intercept z g after particular times resulting in the percentage survival indicated.
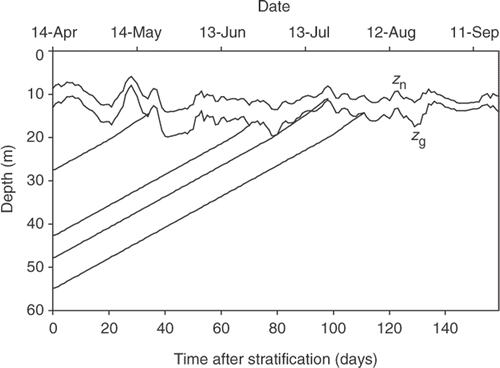
Also shown in are the modelled depth–time trajectories of standard filaments (8 µm width, 0.8 mm length) that started to float up from different depths on 14 April 1999, when the water column of the lake first became thermally stratified (Walsby, Citation2005). A full set of such trajectories of filaments starting at 0.1 m intervals down to 60 m, were calculated by the procedures of Walsby (Citation2005) using the irradiance and temperature data from the lake (Walsby & Schanz, Citation2002). From this set those trajectories that later intercepted z g after the times resulting in different percentage survival have been selected. Thus the model indicates that filaments starting at a depth of 27.4 m on 14 April would intercept z g at 14.5 m on 20 May, 36 days later, after which 90% of the filaments would survive if they had previously been exposed to light prior to stratification. By a similar procedure 50% of the filaments starting at 42.7 m would survive by intercepting z g at 17.2 m after 70 days on 23 June, and the last surviving filaments starting at 54.7 m would intercept z g at 14.3 m after 111 days on 3 August. Note that the analysis cannot predict the precise survival value (even if the survival-time curve were known precisely) because the depth of z g cannot be predicted; it varies with the daily insolation and vertical attenuation of light in the water column. This point is illustrated by the trajectory of filaments starting at about 48 m on 14 April, which, with a small difference in timing, might intercept z g at 19.3 m on 3 July (80 days, 38% survival) or at 11.8 m on 21 July (98 days, 16% survival), because z g decreased rapidly over this period, rising more quickly than the filaments floated up.
Strains that survive longer will make a greater contribution to the inoculum that forms the metalimnetic population but a law of diminishing returns must operate in selection of this character. Following its collapse in early summer, the Planktothrix population, centred in the metalimnion, shows an exponential increase from mid-June to September, up to 100-fold over this period (Micheletti et al., Citation1998; Walsby & Schanz, Citation2002). The inoculum for this population comes from filaments that start to float up when the lake becomes thermally stratified in mid-April and whose arrival in the metalimnion contributes to the biomass increase until mid-July, after which growth accounts for most of it. The contribution made by filaments arriving later than this decreases exponentially with time, approaching zero in September when the Planktothrix population becomes entrained in the surface layer. Consider the population in the 40 m water column between z g at 15 m and 55 m when stratification starts in mid-April: after 111 days, filaments at the bottom will have floated up to z g and during this period 61% (see above) of all the filaments in this water column will have survived on reaching z g. However, the viability will be much higher for those starting in the upper layers, (93% for those in the top 12 m, arriving within 34 days) than in the deeper layers (decreasing to 0% for those at 55 m, arriving after 111 days, in early August). Filaments starting deeper in the water column are, therefore, at a double disadvantage: they have lower viability and, arriving later, leave fewer offspring. While increased survival time will address the first of these problems it does not change the second. This will reduce the selective advantage of increased survival time.
Similar calculations could be made for filaments with different floating velocities and survival characteristics. It should be remembered, however, that even after the lake has stratified, wind action generates vertical movements in the water under the thermocline (Mortimer, Citation1974). Nevertheless, factors that affect the recruitment of filaments to the new metalimnetic population will be strongly selected. It has already been commented that following cold winters with deep mixing, strains with stronger gas vesicles will be selected, because a higher proportion will retain buoyancy after mixing to greater depths (Walsby et al., Citation1998; Beard et al., Citation1999) and it has been demonstrated that the gas vesicle genotype GV3, which conveys this character (Bright & Walsby, Citation1999), occurs only in the deepest lakes (Beard et al., Citation2000). It may be significant that in the small sample of strains investigated here, the longest survival times occurred in strains with this GV3 genotype ().
One of the other genotypes, GV1, deserves comment. Beard et al. (Citation1999) showed that this was the genotype of the only strain of P. rubescens isolated from Lake Zürich forty years previously, by Staub (Citation1961). In 1993, two of the eight isolates analysed, strains 9303 and 9307 (), were GV1, and yet out of 185 Planktothrix clonal isolates established from Lake Zürich between 1993 and 1998 only one more was of this genotype. In subsequent genotype analysis of over 4,000 single filaments isolated (but not cultured) from the lake, only one additional GV1 was encountered (S. J. Beard and B. A. Handley, unpublished): it seems that this genotype, which may once have been abundant, is disappearing. The two GV1 strains isolated from the lake in 1993, not only show lower percentage survival (), they also have narrower filaments (Table 2 in Walsby et al., Citation1998), which would float less quickly. These two characteristics would appear to compromise recruitment of these strains to the metalimnion by processes discussed above. Perhaps changes in the hydrography or other properties of the lake have made recruitment more important in recent years.
Acknowledgements
This work was supported by an Overseas Research Studentship from Universities UK to DPH.
References
- Anagnostidis , K and Komàrek , J . 1988 . Modern approach to the classification system of cyanophytes. 3-Oscillatoriales . Archiv für Hydrobiol. Supp. , 80 : 327 – 472 .
- Anneville , O , Souissi , S , Gammeter , S and Straile , D . 2004 . Seasonal and inter-annual scales of variability in phytoplankton assemblages: comparison of phytoplankton dynamics in three peri-alpine lakes over a period of 28 years . Freshwater Biol. , 49 : 98 – 115 .
- Beard , SJ , Handley , BA , Hayes , PK and Walsby , AE . 1999 . The diversity of gas vesicle genes in Planktothrix rubescens from Lake Zürich . Microbiol. , 145 : 2757 – 2768 .
- Beard , SJ , Davis , PA , Iglesias-Rodríguez , D , Skulberg , OM and Walsby , AE . 2000 . Gas vesicle genes in Planktothrix spp. from Nordic lakes: strains with weak gas vesicles possess a longer variant of gvpC . Microbiol. , 146 : 2009 – 2018 .
- Berges , JA and Falkowski , PG . 1998 . Physiological stress and cell death in marine phytoplankton: Induction of proteases in response to nitrogen or light limitation . Limnol. Oceanogr. , 43 : 129 – 135 .
- Booker , MJ and Walsby , AE . 1979 . The relative form resistance of straight and helical blue-green algal filaments . Br. Phycol. J. , 14 : 141 – 150 .
- Bossard , P , Gammeter , S , Lehmann , C , Schanz , F , Bachofen , R , Bürgi , HR , Steiner , D and Zimmermann , U . 2001 . Limnological description of the Lakes Zürich, Lucerne, and Cadagno . Aquat. Sci. , 63 : 225 – 249 .
- Bright , DI . 1999 . Physiology and gas vesicles of Planktothrix rubescens , UK : Ph.D. Thesis, University of Bristol .
- Bright , DI and Walsby , AE . 1999 . The relationship between critical pressure and width of gas vesicles in isolates of Planktothrix rubescens from Lake Zürich . Microbiol. , 145 : 2769 – 2775 .
- Bright , DI and Walsby , AE . 2000 . The daily integral of growth by Planktothrix rubescens calculated from growth rate in culture and irradiance in Lake Zürich . New Phytol. , 146 : 301 – 316 .
- Canter , HM . 1973 . A guide to fungi occurring on planktonic blue-green algae . Can. J. Microbiol. , 24 : 922 – 931 .
- Davis , PA and Walsby , AE . 2002 . Comparison of measured growth rates with those calculated from rates of photosynthesis in Planktothrix spp. isolated from Blelham Tarn, English Lake District . New Phytol. , 156 : 225 – 239 .
- Fallon , RD and Brock , TD . 1979 . Decomposition of blue-green algal (cyanobacterial) blooms in Lake Mendota, Wisconsin . Appl. Environ. Microbiol. , 37 : 820 – 830 .
- Fallon , RD and Brock , TD . 1981 . Overwintering of Microcystis in Lake Mendota . Freshwater Biol. , 11 : 217 – 226 .
- Foy , RH , Gibson , CE and Smith , RV . 1976 . The influence of daylength, light intensity and temperature on the growth rates of planktonic blue-green algae . Br. Phycol. J. , 11 : 151 – 163 .
- Gibson , CE . 1975 . A field and laboratory study of oxygen uptake by planktonic blue-green algae . J. Ecol. , 63 : 867 – 880 .
- Goudsmit , G-H , Burchard , H , Peeters , F and Wüest , A . 2002 . Application of k-ε turbulence models to enclosed basins: The role of internal seiches . J. Geophys. Res. , 107 : 1 – 13 .
- Kutschke , I . 1966 . Die thermischen Verhältnisse im Zürichsee zwischen 1937 und 1963 und ihre Beeinflussung durch meteorologische Faktoren . Vierteljahrsschr. Naturforsch. Ges. Zürich. , 111 : 47 – 124 .
- Livingstone , DM . 2003 . Impact of secular climate change on the thermal structure of a large temperate central European lake . Clim. Change , 57 : 205 – 225 .
- Madigan , MT , Martinko , JM and Parker , J . 2003 . Brock–Biology of Microorganisms , New Jersey, , USA : Prentice-Hall .
- Micheletti , S , Schanz , F and Walsby , AE . 1998 . The daily integral of photosynthesis by Planktothrix rubescens during summer stratification and autumnal mixing in Lake Zürich . New Phytol. , 138 : 233 – 249 .
- Mortimer , CH . 1974 . Lake hydrodynamics . Mitt. Int. Ver. Theor. Angew. Limnol. , 20 : 124 – 197 .
- Oliver , RL , Thomas , RH , Reynolds , CS and Walsby , AE . 1985 . The sedimentation of buoyant Microcystis colonies caused by precipitation with an iron-containing colloid . Proc. R. Soc. Lond. B , 223 : 511 – 528 .
- Popels , LC and Hutchinson , DA . 2002 . Factors affecting dark survival of the brown tide alga Aureococcus anaphogefferens (Pelagophyceae) . J. Phycol. , 38 : 738 – 744 .
- Popels , LC , Macintyre , HL , Warner , ME , Zhang , Y and Hutchins , DA . 2007 . Physiological responses during dark survival and recovery in Aureococcus anaphogefferens (Pelagophyceae) . J. Phycol. , 43 : 32 – 42 .
- Reynolds , CS , Jaworski , GHM , Cmeich , HA and Leedale , GF . 1981 . On the annual cycle of the blue-green alga Microcystis aeruginosa Kütz. emend. Elenkin . Phil. Trans. R. Soc., B , 293 : 419 – 477 .
- Staub , R . 1961 . Ernährungsphysiologisch-autökologische Untersuchungen an der planktischen Blaualge Oscillatoria rubescens D. C. Schweiz . Z. Hydrol. , 23 : 84 – 198 .
- Stewart , WDP and Daft , MJ . 1976 . Algal lysing agents of freshwater habitats . Soc. Appl. Bacteriol. Symp. Ser. , 4 : 63 – 90 .
- Thomas , EA and Märki , E . 1949 . Der heutige Zustand des Zürichsees . Mitt. Internat. Verein. Limnol. , 10 : 476 – 488 .
- Van Hannen , EJ , Zwart , G , Van Agterveld , MP , Gons , HJ , Ebert , J and Laanbroek , HJ . 1999 . Changes in bacterial and eukaryotic community structure after mass lysis of filamentous cyanobacteria associated with viruses . Appl. Environ. Microbiol. , 65 : 795 – 801 .
- Walsby , AE . 2005 . Stratification by cyanobacteria in lakes: a dynamic buoyancy model indicates size limitations met by Planktothrix rubescens filaments . New Phytol. , 168 : 365 – 376 .
- Walsby , AE and Holland , DP . 2006 . Sinking velocities of phytoplankton measured on a stable density gradient by laser scanning . J. R. Soc. Interface , 3 : 429 – 439 .
- Walsby , AE and Jüttner , F . 2006 . The uptake of amino acids by the cyanobacterium Planktothrix rubescens is stimulated by light at low irradiances . FEMS Microbiol. Ecol. , 58 : 14 – 22 .
- Walsby , AE and Schanz , F . 2002 . Light-dependent growth rate determines changes in the population of Planktothrix rubescens over the annual cycle in Lake Zürich, Switzerland . New Phytol. , 154 : 671 – 687 .
- Walsby , AE , Avery , A and Schanz , F . 1998 . The critical pressures of gas vesicles in Planktothrix rubescens in relation to the depth of winter mixing in Lake Zürich, Switzerland . J. Plankt. Res. , 20 : 1357 – 1375 .
- Walsby , AE , Ng , G , Dunn , C and Davis , PA . 2004 . Comparison of the depth where Planktothrix rubescens stratifies and the depth where the daily insolation supports its neutral buoyancy . New Phytol. , 162 : 133 – 145 .
- Walsby , AE , Schanz , F and Schmid , M . 2006 . The Burgundy-blood phenomenon: a model of buoyancy change explains autumnal waterblooms of Planktothrix rubescens in Lake Zürich . New Phytol. , 169 : 109 – 122 .
- Zotina , T , Köster , O and Jüttner , F . 2003 . Photoheterotrophy and light-dependent uptake of organic and organic nitrogenous compounds by Planktothrix rubescens under low irradiance . Freshwater Biol. , 48 : 1859 – 1872 .