Abstract
Azaspiracids (AZAs) are a group of lipophilic marine biotoxins associated with human incidents of shellfish poisoning. During a research cruise to the North Sea, we analysed size-fractionated plankton for AZA by mass spectrometry and successfully isolated an AZA-producing dinoflagellate from the east coast of Scotland. As shown previously, an axenic culture of this dinoflagellate produces AZA 1, AZA 2 and an isomer of AZA 2. Here we give a taxonomic description of this new taxon Azadinium spinosum gen. et sp. nov., as a de novo producer of AZAs. Azadinium spinosum is a small (12–16 µm length and 7–11 µm width) peridinin-containing photosynthetic dinoflagellate with a superficial resemblance under light microscopy to gymnodinioids, but with a thin theca. The large nucleus is spherical and located posteriorly, whereas the single chloroplast is parietal, lobed, and typically extends into both the epi- and hyposome. The Kofoidian thecal tabulation is APC, 4′, 3a, 6″, 6C, 5?S, 6′″, 2″″. This plate pattern has an epithecal affinity to the Peridiniales and a hypothecal affinity to the Gonyaulacales, but is distinctly different from described dinoflagellate genera. The assignment of A. spinosum to the dinoflagellates is supported by molecular phylogenetic analysis of four genes, SSU rDNA, LSU rDNA (D1/D2 region), ITS and cytochrome oxidase (sub-unit 1) (COI). In agreement with the morphological description, phylogenetic analysis did not show any particularly close affiliation to the Peridiniales or Gonyaulacales, nor to any other dinoflagellate order represented in molecular databases. Consequently, we erected a new genus, Azadinium, for this taxon. However the ordinal affiliation of the genus is uncertain. This study represents the first description and confirmation of a new dinoflagellate species capable of producing AZA and is thus an important advance in surveillance programmes for toxigenic microalgae and toxins of human health significance.
Introduction
In the Netherlands in 1995 several cases of human poisoning resulted from the consumption of cultivated blue mussels (Mytilus edulis) from Killary Harbour, Ireland (McMahon & Silke, Citation1996). The symptoms (nausea, vomiting, severe diarrhoea and stomach cramps) were typical of the well-known diarrhetic shellfish poisoning (DSP) syndrome, caused mainly by toxic lipophilic compounds produced by dinoflagellates of the genus Dinophysis Ehrenberg. Yet subsequent chemical analysis of contaminated mussels found only insignificant levels of DSP toxins. The causative toxin was later isolated and structurally characterised from toxic mussels from Ireland (Satake et al., Citation1998), and later structurally revised (Nicolaou et al., Citation2006; Frederick et al., Citation2007), as a nitrogen-containing polyether with a unique spiral ring assembly, a cyclic amine, and a carboxylic acid. This novel marine biotoxin was named azaspiracid (AZA). Since its discovery this compound and numerous analogues have been detected in a variety of bivalve species (Furey et al., Citation2003; Rehmann et al., Citation2008), primarily from European countries including Ireland, United Kingdom, Norway, Netherlands, France, Spain, Portugal and Italy (Satake et al., Citation1998; Ito et al., Citation2002; James et al., Citation2002; Magdalena et al., Citation2003; Vale et al., Citation2008) and from Africa (Taleb et al., Citation2006).
In addition to increasing efforts to characterise toxicity and mode of action of azaspiracids (Twiner et al., Citation2005, 2008; Ito et al., Citation2006), clarification of the aetiology of AZP has been an important task in recent years. As is the case with several other marine biotoxins, accumulation of AZAs in molluscs strongly suggests food web transfer from lower trophic levels. Due to their polyether structural features containing a biologically active secondary amine moiety, AZA was suspected to be a dinoflagellate metabolite. Based upon chemical analysis by liquid chromatography coupled to mass spectrometry (LC-MS) of manually collected phytoplankton specimens from net hauls, Yasumoto (Citation2001) first indicated that a species of the dinoflagellate genus Protoperidinium Bergh was the primary source of AZA. This work was later published in detail by James et al. (Citation2003), with the culprit described as Protoperidinium crassipes (Kofoid) Balech. The link between AZA and P. crassipes, however, has remained controversial because production of AZA by P. crassipes has not been verified despite numerous attempts based upon field surveys and laboratory investigations of cultured and isolated cells. The uncertainty about the association of AZA toxins with Protoperidinium as the primary source organism is also underlined by a number of indirect arguments. First, in contrast to other proven producers of phycotoxins, which are all primarily phototrophic, P. crassipes is a heterotrophic dinoflagellate, known to prey upon other dinoflagellates (Gribble & Anderson, Citation2006). Therefore, the likelihood that another dinoflagellate could produce AZA, which then accumulates in P. crassipes through normal feeding processes, must be considered. Indeed, a number of micrograzers, including tintinnids and heterotrophic dinoflagellates, have been shown to act as transfer vectors for other phycotoxins (Maneiro et al., Citation2000; Jeong et al., Citation2001, Citation2003; Miles et al., Citation2004). Second, recent occurrences of AZA off the coast of Ireland have not been well correlated with the presence of P. crassipes or other members of Protoperidinium (Moran et al., Citation2005). Finally, preliminary laboratory experiments using cultures of P. crassipes have failed to detect AZA (Gribble, Citation2006; Tillmann & Krock, unpublished data).
During a research cruise in the North Sea in June, 2007, we measured various phycotoxins in fractionated phytoplankton samples by LC-MS and found the highest AZA concentrations in the size-fraction 8–20 µm (Krock et al., Citation2008). Combined with other observations (Krock et al., Citation2008), this information excluded P. crassipes as a de novo producer of AZA. We were therefore motivated to isolate and screen plankton species in the lower size range, resulting in the successful isolation of an AZA-producing dinoflagellate. This dinoflagellate was shown to produce AZA-1, AZA-2 and an isomer of AZA-2 in unialgal and axenic culture (Krock et al., Citation2008). Here we report the taxonomic identity, as well as the genotypic and phenotypic characterisation and toxin profile, of this new small thecate photosynthetic dinoflagellate, belonging to the group Peridiniphycidae, as a de novo producer of AZA toxins.
Materials and methods
Isolation and culture of the AZA-producing dinoflagellate
The culture of Azadinium spinosum gen. et sp. nov., provisionally designated as dinoflagellate isolate 3D9, was established from a water sample collected in a Niskin bottle from a rosette sampler cast in the North Sea on the Scottish east coast at 57°3.9′N; 02°30.2′W in June 2007. Details of the isolation by serial dilution and capillary micropipette are described in Krock et al. (Citation2008). Subcultures of the original isolate were grown non-axenically at 15–20°C in half-strength K medium (Keller et al., Citation1987), supplemented with selenite (Dahl et al., Citation1989), but without addition of ammonium ion. The growth medium was prepared from sterile-filtered (VacuCap 0.2 µm Pall Life Sciences) natural North Sea water (salinity: 32 psu, pH adjusted to 8.0).
Light microscopy (LM)
Observation of live cultured cells was carried out first with a stereomicroscope (Olympus SZH-ILLD) and then with an inverted microscope (Axiovert 200 M, Zeiss, Germany) equipped with epifluorescence and differential interference contrast optics. The swimming pattern of the cells was recorded with a video CCD camera (Sony DSP 3-CCD). Light microscopic determination of the thecal plate tabulation was performed on formalin-fixed cells (1% final concentration) stained with calcofluor white (Fritz & Triemer, Citation1985). The shape and localisation of the nucleus was determined after staining formalin-fixed cells with 4′-6-diamidino-2-phenylindole (DAPI, 0.1 µg ml−1 final concentration) for 10 min. Photographs were taken with a digital camera (Axiocam MRc5, Zeiss, Germany) connected to the inverted microscope.
Scanning electron microscopy (SEM)
Best results for SEM were obtained with the following fixation and preparation protocol. Cells from 1.5 ml of a dense culture were collected in Eppendorf microtubes by centrifugation (5 min, 16,000 g, Eppendorf centrifuge 5415 R). The supernatant was removed and the cell pellet re-suspended in 60% ethanol for 1 h at 8°C to strip off the outer cell membrane. Subsequently, cells were pelleted by centrifugation and re-suspended in 40% seawater for 30 min at 8°C. After removing the seawater supernatant, cell pellets were re-suspended and fixed with 5% glutaraldehyde in 0.2 M cacodylate buffer for 3 h at 8°C. Cells were then collected on polycarbonate filters (Millipore, 25 mm Ø, 3 µm pore-size) in a filter funnel where all subsequent washing and dehydration steps were carried out. A number of washings (2 × 0.1 M cacodylate, 2 × 0.05 M cacodylate, 8 × MilliQ-deionized water) were followed by a dehydration series in ethanol (30, 50, 70, 80, 95, 100%). Filters were dehydrated with hexamethyldisilazane (HMDS), first in 1:1 HMDS:EtOH followed by 2 × 100% HMDS, and then stored under gentle vacuum in a desiccator. Finally, filters were mounted on stubs, sputter-coated (Emscope SC500, Ashford, UK) with gold-palladium and viewed under a scanning electron microscope (FEI Quanta FEG 200, Eindhoven, Netherlands). Some SEM micrographs were presented on a black background using Adobe Photoshop 6.0 (Adobe Systems, San Jose, USA).
Morphological description and taxonomic assignment
We have adopted the general dinoflagellate classification scheme proposed by Fensome et al. (Citation1993). In describing the thecal plate tabulation, we employed the nomenclature of Kofoid (Citation1909). The designation of the putatively sub-divided thecal plates is adopted from Morrill & Loeblich (Citation1981).
Photosynthetic pigment analysis
As a phenotypic characteristic for taxonomic analysis the pigment profile was analysed by liquid chromatography coupled to diode array detection (LC-DAD) by spectroscopy. For pigment analysis, a culture of A. spinosum was grown in a 500 ml Erlenmeyer flask at 15°C under a photon irradiance of 25 µmol m−2 s−1. Upon reaching early stationary phase, at a cell concentration of 1.30 × 105 cells ml−1, triplicate samples of 60 ml were gently filtered onto glass-fibre filters (GF/C, Whatman, Kent, UK). Filters were immediately shock-frozen in liquid nitrogen and stored at −20°C for 10 days before being analysed. Pigments were extracted by soaking the filter for 24 h at −30°C in 5 ml of 100% acetone. Afterwards each extract was syringe-filtered through 0.2 µm cellulose filters (Spartan, Whatman, Kent, UK) and poured into 2 ml LC auto-sampler vials maintained at 5°C in an auto-sampler unit.
The LC system consisted of a controller/pump, solvent de-gasser, ternary gradient unit, a column oven and an autosampler (all Jasco, Groß-Umstadt, Germany). Pigments were separated as described in Zapata et al. (Citation2000) on a reverse-phase C8 column (Symmetry, Waters Corporation, Milford, USA); 150 × 4.6 mm i.d., 3.5 µm particle size) protected by a guard column of the same material. The binary solvent gradient consisted of two eluants with the following composition: eluant A was a mixture of methanol:acetonitrile:0.25 M aqueous pyridine solution (50:25:25 v:v:v); eluant B was a mixture of acetonitrile:acetone (80:20 v:v). The solvent flow rate was constant at 1 ml min−1 and the column temperature was set to 25°C. The analytical gradient was 100, 60, 0, 0, 100% of eluant A at 0, 18, 22, 34, 37 min, respectively, for a total run time of 42 min. The pigments were detected by photodiode array spectroscopy (Jasco, Groß-Umstadt, Germany) over the spectrum from 350–750 nm (resolution: 1.2 nm). The absorbance chromatogram was extracted at 434 nm. Pigments were identified by co-chromatography of authentic standards (DHI, Horsholm, Denmark) and photo-diode spectroscopy. Each peak was checked for homogeneity using the Jasco Chrompass LC software and compared to a spectral library created from the standards.
Chemical analysis of azaspiracids
For AZA analysis, cultures of A. spinosum were grown in 500 ml Erlenmeyer flasks at 20°C under a photon irradiance of 25 µmol m−2 s−1. After 1 week of growth, subsamples at a cell concentration of 25 × 103 ml−1 were taken for toxin analysis. In triplicate, two aliquots of 500 µl cell culture were collected on 0.45 µm pore-size spin-filters (Millipore Ultrafree, Eschborn, Germany) by centrifugation at 800 g at 4°C for 30 s. Cells were extracted by incubation with 50 µl methanol for 30 min. The extracts were subsequently filtered by centrifugation at 3000 g at 4°C for 30 s and transferred to LC vials for analysis.
Azaspiracids were analysed by liquid chromatography coupled to tandem mass spectrometry (LC-MS/MS) according to the method described in detail by Krock et al. (Citation2008). Mass spectral experiments were performed on an ABI-SCIEX-4000 Q Trap® triple quadrupole mass spectrometer (Applied Biosystems, Darmstadt, Germany) equipped with a Turbo V™ ion source coupled to an liquid chromatograph (Agilent 1100 LC, Waldbronn, Germany). The LC equipment included a solvent reservoir, in-line degasser (G1379A), binary pump (G1311A), refrigerated autosampler (G1329A/G1330B) and temperature-controlled column oven (G1316A).
Separation of lipophilic toxins was performed by reverse-phase chromatography on a C8 stationary phase (50 × 2 mm Hypersil BDS, 3 µm 120 Å, Phenomenex, Aschaffenburg, Germany). Resolution was achieved by binary gradient elution after injection of 5 µl of dinoflagellate extract. Multiple reaction monitoring (MRM) experiments were carried out in positive ion mode by selecting the following transitions (precursor ion > fragment ion): (i) AZA-1: m/z 842 > 824 collision energy (CE): 40 V and m/z 842 > 672 CE: 70 V); (ii) AZA-2: m/z 856 > 838 CE: 40 V and m/z 856 > 672 CE: 70 V, and (iii) AZA-3: m/z 828 > 810 CE: 40 V and m/z 828 > 658 CE: 70 V.
A standard solution of AZA-1 and a mussel extract containing AZA-1, AZA-2 and AZA-3 were kindly donated by Philipp Hess, Marine Institute, Galway, Ireland for calibration and identification of AZA components by LC-MS/MS.
Molecular phylogenetics
Extraction of genomic DNA
A 10 ml sample of an exponentially growing culture of Azadinium isolate 3D9 was centrifuged (Eppendorf 5810R, Hamburg, Germany) at 3220 g for 15 min at room temperature. The cell pellet was frozen at −20°C for 20 min before being subjected to total DNA extraction with the DNeasy Kit (Mini) (Qiagen, Hilden, Germany) according to manufacturer's instructions. The purity and quantity of the DNA was checked by UV-spectroscopy with a NanoDrop ND-1000 system (Peqlab, Erlangen, Germany) and the integrity of DNA fragments of a molecular weight of about 20 kb was verified on a 0.8% agarose gel.
PCR amplification and sequencing
The extracted DNA from the Azadinium isolate 3D9 was subjected to polymerase chain reaction (PCR) amplification of the following genes: 18S ribosomal DNA, 28S ribosomal DNA (D1/D1 region), internal transcribed spacer (ITS) and cytochrome oxidase sub-unit 1 (COI). The forward and reverse primers for amplification of full length 18S rDNA were: 1F (5′-AAC CTG GTT GAT CCT GCC AGT-3′) and 1528R (5′-TGA TCC TTC TGC AGG TTC ACC TAC- 3′), respectively. The forward and reverse primers for amplification of 28S rDNA (D1–D2 regions) were: Dir-F (5′-ACC CGC TGA ATT TAA GCA TA-3′) and Dir-2CR (5′-CCT TGG TCC GTG TTT CAA GA-3′), respectively. The forward and reverse primers for amplification of the ITS region were: ITS a (5′-CCA AGC TTC TAG ATC GTA ACA AGG (ACT)TC CGT AGG T-3′) and ITS b (5′-CCT GCA GTC GAC A(GT)A TGC TTA A(AG)T TCA GC(AG) GG-3′), respectively. The following primers were used for amplification of the cytochrome oxidase 1 (COI) gene: COIF (5′-AAAAATTGTAATCATAAACGCTTAGG-3′) and COIR (5′-TGTTGAGCCACCTATAGTAAACATTA-3′) (Zhang et al., Citation2005).
For each 50 µl PCR reaction, HotMasterTaq (Eppendorf®) buffer 1X, 0.1 mM of dNTPs, 0.1 mM of each forward and reverse primer and 1.25 units of Taq polymerase were added to 10–30 ng of the extracted genomic DNA.
For 18S and 28S rDNA amplifications, the reactions were subjected to the following thermocycling conditions: an initial denaturation at a temperature of 95°C for 7 min was followed by 35 cycles of denaturation at 94°C for 45 s, annealing temperature at 54°C for 2 min and elongation temperature at 72°C for 1.5 min. A final extension step at 72°C was carried out or 10 min.
For ITS amplifications, the reactions were subjected to the following thermocycling conditions: an initial denaturation at a temperature of 94°C for 4 min was followed by 9 cycles of denaturation at 94°C for 50 s, annealing temperature at 60°C for 40 s and elongation temperature at 72°C for 1 min; then 29 cycles of denaturation at 94°C for 45 s, annealing temperature at 50°C for 45 s and elongation temperature at 72°C for 1 min. A final extension step at 72°C was carried out for 5 min.
For COI amplifications, the reactions were subjected to the following thermocycling conditions: an initial denaturation at 94°C for 3 min was followed by 39 cycles of denaturation at 94°C for 30 s, annealing temperature at 46°C for 30 s and elongation temperature at 70°C for 1 min. A final extension step at 70°C was carried out for 5 min.
The completed reactions for all of the above amplifications were kept at 10°C until the next step. The PCR amplicons were analysed on 1% agarose by electrophoresis.
Sequence alignment for phylogenetic analyses
Sequence alignment was done with CLUSTAL X software (Thompson et al., Citation1997), and improved manually for all sequences; ambiguous alignments positions were excluded for the analysis. Alignments are available upon request and sequences are available at GenBank under accession numbers 1133791 (SSU), 1133764 (LSU), 1133769 (ITS), and 1133771 (COI). Maximum likelihood (ML) phylogenetic trees for all genes were calculated with PhyML (Guindon & Gascuel, Citation2003) using a BIO-NJ (neighbor-joining; NJ) tree as a starting tree, the WAG evolutionary model (Whelan & Goldman, Citation2001), with a gamma distribution parameter estimated from the data. For the SSU, LSU, and ITS of rDNA and COI, we used Oxyrrhis marina Dujardin as outgroup. An optimal base substitution model was calculated with Modeltest (Posada & Crandall, Citation1998; Posada & Buckley, Citation2004) ().
Table 1. Selected substitution model parameters obtained with Modeltest version 3.7 (Posada & Crandall, Citation1998; Posada & Buckley, Citation2004) for each of the four marker regions utilized in this study.
Nodal support was estimated by bootstrap analyses (Felsenstein, Citation1985) using maximum parsimony (MP), and NJ with the Aikaike Information Criterion (AIC) model parameters described above (). The bootstrap analyses were done in 1000 replicates for MP and NJ, and with 100 replicates for the ML analysis.
Results
Formal descriptions
Azadinium Elbrächter et Tillmann gen. nov.
TYPE: Azadinium spinosum Elbrächter et Tillmann gen. et sp. nov.
DIAGNOSIS: Cellulae dinoflagellatae vegetativae armatae biflagellatae cum formula tabulatio: APC, 4′, 3a, 6″, 6C, 5?S, 6′″, 2″″. Differt de generae de ordo Peridiniales Peridinium Ehrenberg, Protoperidinium Bergh, Ensiculifera Balech, Heterocapsa Stein, Pentapharsodinium Indelicato et Loeblich, Scrippsiella Balech ex Loeblich in numero lamellae precingularia et postcingularia (7 resp. 5), de generae de ordo Gonyaulacaes cum 6 precingulares et 6 postcingulares Gonyaulax Diesing, Gambierdiscus Adachi et Fukuyo, Goniodoma Stein, Protoceratium Bergh, Spiraulax Kofoid in numero lamellae apicales et intercalares epithecales. Differt de Peridiniella Kofoid in numero lamellae precingulares: 7 in Peridiniella, 6 in Azadinium.
Vegetative cells are thecate biflagellate dinoflagellates with the tabulation: APC, 4′, 3a, 6″, 6C, 5?S, 6′″, 2″″. This differs from genera of the order Peridiniales such as Peridinium Ehrenberg, Protoperidinium Bergh, Ensiculifera Balech, Heterocapsa Stein, Pentapharsodinium Indelicato et Loeblich, Scrippsiella Balech ex Loeblich and similar genera in the number of precingular and postcingular plates (7 and 5, respectively). This tabulation also differs from genera of the Gonyaulacales having six precingular and six postcingular plates, such as Gonyaulax Diesing, Gambierdiscus Adachi et Fukuyo, Goniodoma Stein, Protoceratium Bergh, Spiraulax Kofoid, in the number of epithecal and anterior intercalary plates. Azadinium is similar in some respects to Peridiniella Kofoid but differs in the number of precingular plates – 7 in Peridiniella versus 6 in Azadinium.
ETYMOLOGY: The genus name Azadinium (neuter) denotes with the first syllable Aza, the secondary amine function of azaspiracid, believed to be the biologically active pharmacophore of this molecule. At least one strain of the type species of this dinoflagellate genus produces several analogues of the toxin azaspiracid, and this is therefore a phenotypic criterion.
Azadinium spinosum sp. nov. Elbrächter et Tillmann (Figs )
Fig. 1. Azadinium spinosum. Light-microscopy view of (A, B) live cells; (C) empty theca and (D, E) formalin-fixed cells. Abbreviations: APC: apical pore complex. N: nucleus. Arrow in D: antapical spine. Arrowhead in D: pyrenoid. Scale bars: 5 µm.
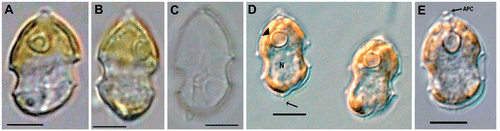
DESCRIPTION: Cellulae dinoflagellatae vegetativae armatae biflagellatae photosyntheticae cum 1 chloroplastis. Formula tabulatio: APC, 4′, 3a, 6″, 6C, 5?S, 6′″, 2″″. Porus ventralis in lamella primae apicalis. Longitudine circa 12 to 16 µm, latitudine circa 7 to 11 µm.
Fig. 2. Azadinium spinosum. SEM micrographs of thecae of different cells in (A) ventral view; (B) dorsal view; (C) right-lateral view. Scale bars: 5 µm.
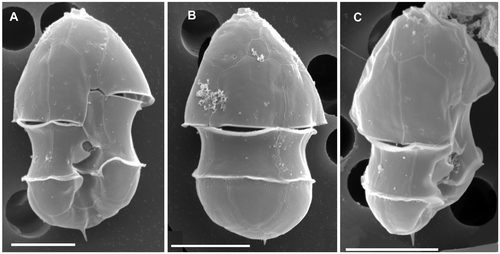
Biflagellate vegetative cells are photosynthetic with one chloroplast. The plate tabulation is: APC, 4′, 3a, 6″, 6C, 5?S, 6′″, 2″″. A ventral pore is present in the first apical plate. Cell length is about 12 to 16 µm, cell width about 7 to 11 µm.
Fig. 3. Azadinium spinosum. SEM micrographs of epithecal plates from different cells in (A) ventral view; (B) mid-dorsal view; (C) right dorsal view; (D) left dorsal view; (E) left-lateral view. Abbreviation: Sa: anterior sulcal plate. Scale bars: 2 µm.
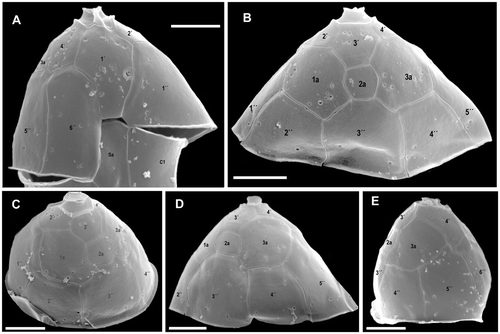
HOLOTYPE: SEM-stub (designation CEDiT 2008H1), deposited at the Senckenberg Research Institute and Natural History Museum, Centre of Excellence for Dinophyte Taxonomy, Germany.
Fig. 4. Azadinium spinosum. SEM micrographs of hypothecal plates of different cells in (A) ventral view; (B) ventro-antapical view; (C) dorso-right-lateral view; (D) right-lateral view. Abbreviation: Sp: posterior sulcal plate. Scale bars: 2 µm.
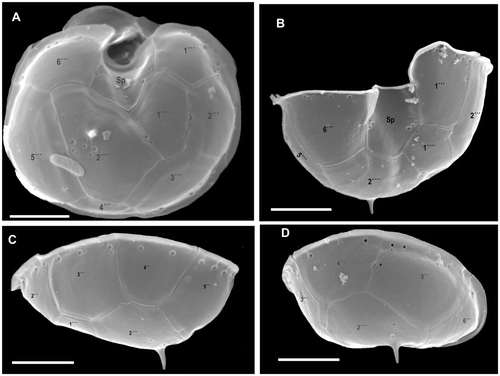
ISOTYPES: Formalin fixed sample (designation CEDiT 2008I2), deposited at the Senckenberg Research Institute and Natural History Museum, Centre of Excellence for Dinophyte Taxonomy, Germany; formalin fixed sample (designation B 40 0040631) and SEM-stub (designation B 40 0040632), deposited at the Botanical Museum, Berlin.
Fig. 5. Azadinium spinosum. SEM micrographs of different cells; (A) ventral view of the apical pore complex (APC) and part of plate 1′with ventral pore; (B) apical view of APC and apical plates; (C) ventral pore with slit (arrow) running to the suture between plate 1′and 1″; (D) hypotheca and cingulum in right dorsal view. White arrow points to the internal wing of sulcal plates; (E) detailed view of the sulcal region. Abbreviations: Sa: anterior sulcal plate; Sp: posterior sulcal plate; Ss: left sulcal plate; Sm: median sulcal plate; Sd: right sulcal plate. Scale bars: 2 µm (Fig. 5A, B, D, E) and 0.4 µm (Fig 5C).
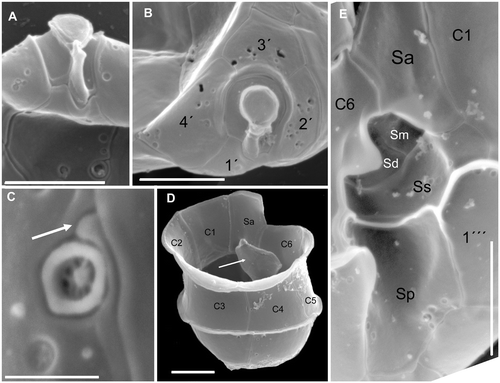
TYPE LOCALITY: 57°3.9′ N, 02°30.2′ W, North Sea off Scotland.
Fig. 6. Azadinium spinosum. Diagrammatic illustration of thecal plates; (A) ventral view; (B) left side; (C) right side; (D) dorsal view; (E) apical view; (F) antapical view (black triangle: antapical spine). Abbreviations: APC: apical pore complex; Sa, Sd, Sm, Sp, Ss: sulcal plates, as detailed in Fig. 5; vp: ventral pore).
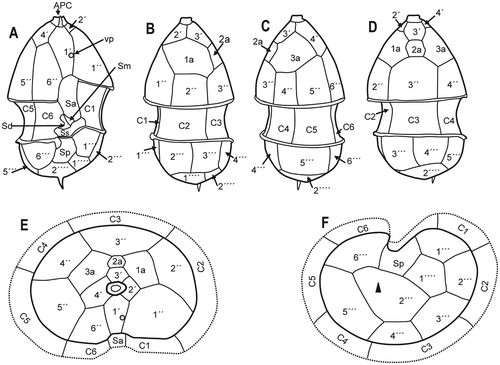
HABITAT: Marine plankton.
ETYMOLOGY: The epithet refers to the antapical spine that is almost invariably present.
Cell morphology
Cells of A. spinosum are elliptical, slightly elongated, and dorso-ventrally compressed. The conical episome with convex sides ends with a conspicuous apical pore complex (APC) and is larger than the hemispherical hyposome. The cingulum is deep and wide, accounting for approximately one quarter of total cell length. The hyposome is slightly asymmetrical, with the right side more convex than the left side. Cells are small and range from 12.3–15.7 µm in length and 7.4–10.3 µm in width (mean length: 13.8, mean width 8.8 µm, n = 73; theca measurement from SEM images). The large nucleus is spherical and located in the posterior part of the cell (). A single chloroplast is parietally arranged, lobed, and normally extends into both the epi- and hyposome. In old cultures or under high light conditions, however, the chloroplast may be restricted to the episome (). One large pyrenoid is located in the episome.
Under the light microscope, living cells resemble gymnodinioid dinoflagellates (, ). Azadinium cells, however, possess delicate thecal plates which can be seen when stressed cells (by agitation or upon the microscope stage under observation, etc.) shed their theca () or when cells are fixed in formalin (, ). A single, small and delicate antapical spine can also be seen, located slightly asymmetrically at the right side of the cell. The delicate theca can be stained with calcofluor white (not shown), but due to the small size and the delicate nature of the plates, detailed analysis of the plate pattern using fluorescence microscopy proved to be difficult. Nevertheless, the plate pattern resolved by SEM (Figs ) was in accordance with that analysed by calcofluor-stained cells. Generally, the surface of the plates is smooth but irregularly covered by pores of different sizes (ranging from 0.07 to 0.14 µm in diameter). A prominent row of pores with a mean diameter of 0.13 µm (±0.01 µm standard deviation, n = 20) is usually located below the lower cingulum list ().
The basic thecal plate arrangement was determined as: APC, 4′, 3a, 6″, 6C, 5?S, 6′″, 2″″ and is shown in . The apical pore is round or slightly ellipsoid, located in the centre of a pore plate and connected by a finger-like protrusion with the first apical pate 1′ (, ). From LM and SEM micrographs, it is not clear whether the apical pore is covered by a thecal plate or by cytoplasm. The same applies to the nature of the finger-like protrusion. The apical series is composed of four plates. Plate 1′ is of the ortho pattern, but slightly asymmetric, while the suture joining plate 6″ is shorter than that joining plate 1″. The shape of plate 1′ exhibits some variability, which is documented in Fig. S1 (see supplementary material available online at: http://www.informaworld.com/mpp/uploads/ejp_tillmann_et_al.pdf).
A distinct ventral pore is always present on the left margin of plate 1′. The ventral pore is about 300 nm in diameter and has a delicate substructure. If the pore is positioned inside plate 1′, it is connected by a narrow slit with the suture between plates 1′ and 1″ (see , and Fig. S1 supplementary material). Plates 2′ and 3′ are small. Plate 4′ is much larger and obvious in ventral view, whereas plate 2′ is displaced more dorsally, causing the asymmetry of plate 1′. The three intercalary plates are arranged more or less symmetrically on the dorsal side of the epitheca. The first and third intercalary plates are relatively large; the smaller plate 2a is four-sided (). The six precingular plates are of comparable size, but 1″ is distinctively wider than the 6″ plate. Moreover, plate 1″ is in contact with an intercalary plate (1a) and thus in contact with four epithecal plates, whereas plate 6″ is very narrow and five-sided, but only in contact with three apical plates (see ). The hypotheca has a plate arrangement consisting of six postcingular and two antapical plates (). All postcingular plates are of comparable sizes, but the four-sided plate 4′″ is the smallest, whereas plate 5′″ (also four-sided) is the largest. The two antapical plates are of markedly different size, with plate 1″″ displaced to the left and the larger 2″″ plate bearing a single short antapical spine.
The cingulum is wide, descending, displaced by about half of its width and composed of six plates of almost equal size (). Narrow cingular lists are present, particularly on the posterior cingulum fringe. In addition, a conspicuous lobe-shaped convexity of the sixth cingular plate partly covers the sulcal area and the flagellar pore region (). The plate pattern of the deeply concave sulcus is difficult to resolve and to interpret. We identified a large anterior sulcal plate (Sa), which partly invades the epitheca, whereas the large posterior sulcal plate (Sp) extends two-thirds of the way from the cingulum to the antapex. A left sulcal plate Ss is located anterior to Sp and abuts plates 1′″ and 1C. The right sulcal plate Sd abuts sulcal plates Ss and Sm as well as cingular plate 6C. The median sulcal plate Sm contacts sulcal plates Sa, Ss and Sd (). These plates apparently have a complicated three-dimensional structure, with large flanges invading into the hyposome (see , indicated by white arrow). The sulcal plates are covered with different sized pores (). We do not exclude the possibility that there are additional tiny sulcal plates but these could not be resolved by calcofluor-staining and epifluorescence microscopy or SEM. Dissection with hypochlorite did not resolve further platelets.
The plate pattern shown in Figs is the standard basic pattern. Several variations were, however, observed (see Fig. S2, http://www.informaworld.com/mpp/uploads/ejp_tillmann_et_al.pdf). Most variations can be interpreted as an extra division of a pre-existing plate to yield an additional plate, a modification that has been designated as a “complexum modification” by Lefevre (Citation1932). Although not statistically analysed, complexum modifications were most commonly observed on the epitheca, specifically by division of apical plates 2′ or 4′, the small intercalary plate 2a, or the dorsal precingular plate 3′ (Fig. S2, supplementary material). Very rarely, complexum modifications of other plates (e.g., 1′, 2″) or a reduction of epithecal plates (e.g. with the small medial intercalary plate 2a missing, see Fig. S2F, supplementary material) were observed. In one case, a particular cingular plate showed duplication in the horizontal plane, resulting in two rows of cingular plates (Fig. S2F, supplementary material). In two specimens, a reduction in hypothecal plates was observed yielding 5 postcingular and 1 antapical plate (Fig. S2I, supplementary material). When in ventral orientation and free of attached debris, all inspected specimens possessed the ventral pore.
The antapical spine is clearly visible in most specimens even under the LM, although the length of the spine is variable. In a few cases it is reduced to a small knob. Rarely (not analysed statistically), no antapical spine was detected, although lack of a spine could be a sample preparation artefact. One specimen was observed with two spines (Fig. S2J, supplementary material).
Swimming behaviour
At low magnification, cells of Azadinium attract attention by their conspicuous swimming pattern (see video clip “azamoving.mpg” available as supplementary material at www.informaworld.com/mpp/uploads/azamoving.mpg). Cells normally swim at low speed, interrupted by short, high-speed ‘jumps’ in various directions. These jumps are regularly observed when cells approach the glass bottom of the observation chamber. Rarely, larger distances may be travelled at higher speed.
Pigment composition
Pigment analysis by HPLC with diode array detection revealed that A. spinosum contained a pigment complement () that is typical for most photosynthetic dinoflagellates, with Chl c2 as the major accessory chlorophyll to Chl a and peridinin as the major carotenoid. In addition, the carotenoids diadinoxanthin, diatoxanthin, dinoxanthin, and prasinoxanthin were present.
Azaspiracid composition and content
The cultured 3D9 strain of A. spinosum produces several azaspiracid analogues, with AZA-1, AZA-2 and an isomer of AZA-2 present in decreasing order of concentration (). The AZA-1 cell quota, estimated by several measurements from various subcultures to be in the range of 5–40 fg cell−1 (data not shown), is quite variable in response to environmental condition and culture stage.
Phylogenetic analysis
Ribosomal DNA
Phylogenetic analysis of the three sequence data sets generated from the SSU, ITS and LSU (D1/D2 region) of the ribosomal rDNA operon () showed that A. spinosum sequences are unique and clearly separated from all known species, genera, and groups within the dinoflagellates (, and Figs S3, S4, supplementary material). The SSU dataset included 64 taxa and 1044 positions, of which 260 were parsimony informative. The AIC in Modeltest selected a GRT + I + G model (see ). The LSU dataset included 63 taxa and 449 positions, of which 314 were parsimony informative. The AIC in Modeltest selected a GRT + G model (see ). The trees inferred from the data and model settings showed that only the Gonyaulacales and Prorocentrales formed monophyletic groups, whereas the Gymnodiniales and Peridiniales formed several distinct clades, only some of which have bootstrap-support (see Figs S3, S4, supplementary material). The ITS dataset included 34 taxa and 538 positions, of which 424 were parsimony informative. The AIC in Modeltest selected a GRT + I + G model (see ). The tree inferred from the data and model settings showed monophyletic groups, with good to moderate bootstrap support. Azadinium spinosum was separated from all other groups, even when the branching order of groups such as the Gymnodiniales, Peridiniales, and Prorocentrales (GPP species [Saldarriaga et al., Citation2004) could not be resolved in the analysis ().
Cytochrome oxidase I (COI)
The COI dataset included 25 taxa and 620 positions of which 205 were parsimony informative. The AIC in Modeltest selected a GRT + G model (see ). The tree inferred from the data and model settings showed that the COI data set phylogeny () was in an agreement with published phylogenies using this and other markers (Daugbjerg et al., Citation2000; Saldarriaga et al., Citation2001; Zhang et al., Citation2007). COI resolved the Prorocentrales as a monophyletic group (even though represented by only two taxa), but the Gymnodiniales, Gonyaulacales, and Peridiniales appear at least polytomic, if not paraphyletic. Heterocapsa triquetra branched early from the base of this tree, just after the outgroup, Oxyrrhis marina. The remaining Peridiniales sequences formed a well-supported clade with sister-affiliations towards the Prorocentrales and A. spinosum.
Discussion
In this study, we followed the principles of modern taxonomy in combining morphological and other phenotypic descriptors with molecular phylogenetic characterisation based upon well-studied genes with generally conservative properties. We are therefore confident in ascribing A. spinosum to a new genus within the dinoflagellates and that it has not been previously described under another taxonomic designation. The available morphological and molecular descriptors did not, however, permit clear resolution of the higher level (ordinal) affinities of this organism. Uncertainties in classification of the genus at the family and ordinal level are best illustrated in the following discussion of the plate tabulation of Azadinium and its morphological affinities, combined with molecular phylogenetic analysis, particularly with respect to the Peridiniales and Gonyaulacales.
Morphological classification of the new taxon
With six latitudinal plate series (apical, apical intercalary, precingular, cingular, postcingular and antapical) this dinoflagellate clearly belongs to the subclass Peridiniphycidae. The configuration of the epitheca, with four apical plates, three apical intercalary plates and six precingular plates (4′, 3a, 6″), and of the cingulum with six cingular plates (6C) closely resembles that of genera assigned to the order Peridiniales, such as Peridinium Ehrenberg, Protoperidinium Bergh, Ensiculifera Balech, Heterocapsa Stein, Pentapharsodinium Indelicato et Loeblich, Scrippsiella Balech ex Loeblich. Nevertheless, these genera typically have seven precingular plates, with very few exceptions, e.g. Scrippsiella hexapraecingula Horiguchi et Chihara has only six precingular plates. However, assignment of this new taxon to Scrippsiella can be excluded because members of this genus always have five postcingular plates, in contrast to a consistent six plates in the new taxon.
Affinity with the genus Peridinium Ehrenberg can also be excluded, even though in the rare case of the freshwater species Peridinium gatunense Nygaard an aberrant number of six precingular plates may occur (Boltovskoy, Citation1973). The new taxon has six precingular and postcingular plates, and shares no close resemblance with Peridinium, for which all species have five postcingular plates.
The morphological relationship to Heterocapsa is unclear. The tabulation of the type species H. triquetra (Ehrenberg) Stein cannot be deduced from the figures provided by Stein (Stein, Citation1883, pl. 3 figs. 30–40). Balech (Citation1988) gave the tabulation formula: Po, 4′, 2a, 7″, 6C, 4S, 5′″, 2″″, but plate 7″ can also be interpreted as the anterior sulcal plate. This interpretation would yield six precingular plates, which is the same number as provided by Lindemann (Citation1928), giving 4ap + 2a + 6pr + 5pst + 0 p + 2at, corresponding to the Kofoidian pattern 4′, 2a, 6″, 5′″, 2″″. The same epithecal plate pattern can also be deduced from the SEM micrograph of Lewis & Dodge (Citation1990, figs 6.30, 6.31C) and from LM-photographs (M. Elbrächter, unpublished). This epithecal tabulation is in contradiction to that given by Morill & Loeblich (Citation1981) as 5′, 3a, 7″, 6C, 7S, 5′″, 1p, 2′″, which is very close to that of Cachonina Loeblich (Citation1968). A re-investigation of the type species from the type locality (Baltic Sea) is needed to resolve these problems. In all cases, published hypothecal tabulations of Heterocapsa indicate five postcingular plates, whereas the newly described taxon has six nearly equal-sized postcingular plates.
The only member of the Peridiniales having six postcingular plates is the genus Sphaerodinium Woloszynska (regarded by some authors as a later taxonomical synonym of Glenodinium Ehrenberg), but members of this genus have four more or less equal-sized apical intercalary plates and a conspicuous eyespot, which is missing in the new taxon. Some genera of the family Protoperidiniaceae Balech, such as Diplopsalis Bergh, Diplopelta Stein ex Joergensen, Lebouraia Abé (regarded by some authors as congeneric with Diplopelta) or Oblea Balech also have six precingular plates, but all of these have fewer apical intercalary plates and (more importantly) only four cingular plates; the first of the cingular plates, the ‘t’-plate is very short. The new taxon certainly does not belong to the family Protoperidiniaceae. In conclusion, there is no evidence that the taxon under discussion can be assigned to any described genus of the order Peridiniales.
Superficial resemblance to the Gonyaulacales is suggested by the presence of six precingular and six postcingular plates, as well as of six cingular plates, all of which is in accordance with the general tabulation of this order. A relatively large posterior sulcal plate and two antapical plates, with the left one smaller and slightly displaced towards the cingulum, as well as the insert configuration of the first apical plate (see Fensome et al., Citation1993, text fig. 62A) would also support classification into the Gonyaulacales. But so far, no genus of the Gonyaulacales has been described with almost symmetrical epithecal tabulation with four apical plates and three apical intercalary plates.
Some similarity to members of the suborder Cladopyxiineae is apparent. Whereas Cladopyxis Stein has only three apical plates (but four apical intercalary plates), Palaeophalacroma Schiller and Sinodinium Nie (regarded by some authors as congeneric with Palaeophalacroma) have four apical plates and three apical intercalary plates, which also have a symmetrical configuration. Nevertheless, atypically for the Gonyaulacales, members of the Cladopyxiineae have seven precingular plates, which excludes the assignment of the new taxon to this suborder. The main paratabulation of the genus Luehndea Morgenroth, exclusively known from the early Jurassic period, is very similar to the taxon described here, but nothing is known about an apical pore or the number of cingular plates. The proximochorate-chorate cysts of Luehndea have conspicuous spines and an epitractal archeopyle with a simple operculum. As cysts, and therefore also the type of archeopyle, are currently unknown for our taxon, and cysts resembling those of Luehndea are not known from recent plankton, we cannot justify assigning our taxon to Luehndea.
Strong similarity is apparent between this dinoflagellate and the genus Peridiniella Kofoid, classified as belonging to the Gonyaulacales, but of uncertain family affiliation. Nevertheless, all members of the genus Peridiniella have seven precingular plates (Balech, Citation1979; Dodge, Citation1987; Balech, Citation1988; Okolodkov & Dodge, Citation1995). In conclusion, there is no evidence that our taxon can be assigned to any described genus within the order Gonyaulacales.
On morphological grounds the only remaining group affinities to be discussed are the similarity to genera assigned to the suborder Peridiniphycidae (order affiliation uncertain) by Fensome et al. (Citation1993). The family Dollidiniaceae, known only as fossils from marine strata of late Jurassic to early Cretaceous age, is described as showing resemblance to both the Peridiniales and Gonyaulacales. Members of this family have six precingular, six cingular and six postcingular plates, as well as two antapical plates, which is in accordance with the taxon described here. But Dollidinium Helby et Stover has six apical and no apical intercalary plate.
To our knowledge, there is no genus in the Peridiniphycidae to which the new taxon can be assigned, although the strain investigated showed some plate pattern variability. As is usual in thecate dinoflagellates, the epithecal region of Azadinium exhibited some variability, whereas the hypothecal tabulation was quite stable. Fusion of plates is rare in this taxon, but plates are often sub-divided (see Fig. S2, supplementary material). Of course morphological variability must always be taken into account in describing new taxa. While, our cultures showed substantial variability in the number of thecal plates (around the standard basic pattern), the apparent healthiness and rapid growth of the cultures, and the relatively short time since isolation suggests that this represents natural variation rather than induced artefacts.
Consequently, we describe this taxon here as a new genus Azadinium gen. nov., which clearly fits into the Peridiniphycidae, but cannot be assigned to any existing order or family. Despite several characteristics of both the Peridiniales and Gonyaulacales, based on morphology alone, we cannot resolve whether Azadinium is intermediate between these two orders or is the ancestor from which both lineages are derived.
Morphological comparison at the species level
Some thecate dinoflagellates exhibit superficial resemblance to A. spinosum upon cursory microscopic analysis. For example, under LM, the overall shape including the broad cingulum may suggest allocation to species of the genus Heterocapsa. Nevertheless, as discussed above, species now assigned to this genus have a distinctly different tabulation. There are also some species of the genus Amphidoma Stein with a conspicuous broad cingulum, but all of these have six apical and no apical intercalary plates.
Small species assigned to the genus Gonyaulax have a similar broad girdle, like Gonyaulax parva Ramsfjell (Ramsfjell, Citation1959) and G. gracilis Schiller. Gonyaulax parva was described with the identical main tabulation: 4′, 3a, 6″, 6′″, 1p, 1″″, but plate 1p can be equally well regarded as the first antapical plate. This would be in accordance with the interpretation by Balech (Citation1989) of the hypothecal tabulation of Alexandrium, a member of the Gonyaulacales. The cingular and sulcal plates of G. parva have not been described or depicted in detail but it does not belong to the genus Gonyaulax as presently defined. This species should be reinvestigated. If it has six cingular plates, it likely belongs to the new genus Azadinium. Azadinium spinosum differs from G. parva by the following characters: plates 2′ and 4′ are equal in size and very small in G. parva, the intercalary plates are all very small and equal-sized, whereas in A. spinosum plate 2′ is much smaller than 4′, and plates 1a and 3a are large, whereas 2a is quadrangular and very small. In addition, the clearly visible spine in Azadinium is not present in Gonyaulax parva.
Gonyaulax gracilis Schiller (Schiller, Citation1935) was not validly described (ICBN ART. 32.1) but figures (pl. 90 ) provided by Bérard-Therriault et al. (Citation1999) under that name show an organism with a spine characteristic of A. spinosum. As the authors state in the text, the full tabulation was not established. Thus it remains uncertain, whether the organism they reported from eastern Canada is identical to our species described from the North Sea. Whether the organisms reported as G. gracilis from the Gulf of St. Laurence, Canada, is conspecific with the organism illustrated (but lacking a description) by Schiller, is also uncertain.
Molecular phylogeny
Most molecular phylogenetic studies of microalgae, and dinoflagellates in particular, refer to reconstructions based upon gene sequences of the ribosomal operon. We have attempted to mitigate some of the uncertainty in determining the phylogenetic position of Azadinium by avoiding dependence on a single gene, by comparing the SSU rDNA, LSU rDNA (D1/D2 region), as well as the ITS region. There is apparently an asymmetric rate of evolution among ribosomal genes in dinoflagellates, obviously pronounced for the SSU and for the hypervariable D1/D2 region of LSU, but less so for the ITS region (Daugbjerg et al., Citation2000; Saldarriaga et al., Citation2001, 2004; John et al., Citation2003; Zhang et al., Citation2007). Such incongruities make it difficult to generate consistent conclusions about the evolutionary affinities of the various orders. Thus, whereas the SSU and LSU rDNA data set generated polytomic or paraphyletic ordinal groups for the dinoflagellates (see Figs S3, S4, supplementary material), the ITS phylogeny showed monophyletic groups for the Gonyaulacales, Gymnodiniales, Peridiniales, and Prorocentrales, which were generally well-supported by bootstrap analysis. In all rDNA phylogenies, the Azadinium sequences were unique, with no close affiliation to any known species, genus, or order of dinoflagellates as described by molecular criteria.
As an alternative to the rDNA operon, gene phylogenies based upon conservative proteins such as COI have been helpful in providing important insights into deep branching patterns, and in establishing the evolutionary position of the dinoflagellates within the chromalveolates (Saldarriaga et al., Citation2004). Protein phylogenies have also been shown to have some potential for the resolution of dinoflagellates at the group, genus or species level (Lin et al., Citation2006; Zhang et al., Citation2007). In our case, the phylogenetic tree generated from the COI sequence is in general agreement with previous studies (Lin et al., Citation2006; Zhang et al., Citation2007), supporting the basal position of H. triquetra (Peridiniales) and C. cohnii (Gonyaulacales) in the tree topology (). Azadinium exhibits morphological characteristics of both the Peridiniales and Gonyaulacales, but protein phylogenetic analysis for the rDNA operon does not reveal close affiliation with either order, or to any other dinoflagellate order represented in molecular databases.
Conclusion
Both morphological and molecular data support the description of this dinoflagellate as a new species not assignable to any previously described genus, family or order. While generally supportive of the morphological assignment, molecular phylogenetic analysis was unable to provide sufficient evidence for unequivocal placement of A. spinosum at higher taxonomic levels. Our data set suggests that we may have a new order of dinoflagellates, but this can only be confirmed (or rejected) when more members of this group have been isolated, characterised, and added to the morphological and molecular datasets. The discovery of more dinoflagellate diversity can be expected when currently under-represented small size-fractions (e.g. <20 µm) are investigated (Groisillier et al., Citation2006).
For the moment, based upon morphological analysis, Azadinium must be placed in the subclass Peridiniphycidae of uncertain family and order affiliation. It thereby joins a large number of other dinoflagellate genera of uncertain affinities (Fensome et al., Citation1993). The systematic position will undoubtedly be clarified when molecular data are available from other strains/species of the genus.
The ecological and human health importance of A. spinosum is based on the fact that at least one strain produces azaspiracids. In addition to the results presented here, A. spinosum, as strain 3D9, was shown to produce azaspiracids in axenic cultures (Krock et al., Citation2008) and is thus a de novo producer of AZA toxins. However, we have not drawn any taxonomic or evolutionary significance from this observation. Toxigenicity is a highly variable phenotypic characteristic at the genus, specific and even population level among dinoflagellates (Cembella, Citation2003). Nevertheless, with the attribution of azaspiracids to a defined species and the confirmed occurrence of the producer at multiple field sites in the North Sea (Krock et al., Citation2008), the application of molecular genetic detection methods for detecting and quantifying A. spinosum in field samples will proceed rapidly. Available cultures of toxigenic A. spinosum open avenues for autecological characterisation relevant to genotypic mechanisms of toxin production and regulation, as well as toxin transfer and transformation in pelagic and benthic food webs.
Supplementary Data
Download Zip (36.9 MB)Acknowledgements
We thank Kerstin Heymann (GKSS, Geesthacht, Germany) for pigment analysis by liquid chromatography, Annegret Müller (AWI, Bremerhaven, Germany) for assistance with LC-MS/MS analysis of azaspiracids, and Katrin Kronberger, Hannelore Halliger and Friedel Hinz for their help with electron microscopy. We are grateful to Yean Sin Ong (AWI) for technical assistance with molecular analysis. Philipp Hess, Marine Institute, Galway, Ireland generously provided an AZA-1 standard and AZA-containing mussel reference material. Captain Horst Hansen, the entire FS Poseidon crew, and the AWI chemical ecology of marine protist group (Eva Käppel, Paul Korb, Tilman Alpermann, Sylke Wohlrab, Annegret Müller, Wolfgang Drebing) provided assistance and logistical support for the collection of field material used in this study. The oceanographic cruise NORCOHAB was endorsed by the SCOR-IOC Program GEOHAB as a contribution to the Core Research Project on Harmful Algal Blooms in Fjords and Coastal Embayments. Financial support was provided by the MARCOPOLI research program of the Alfred Wegener Institute as part of the Helmholz Foundation initiative in Earth and Environment.
References
- Balech , E . 1979 . Dinoflagellados. Campana Oceanografica Argentina “Islas Orcadas” 06/75 . Repubica Argentina, Armada Argentina Servico de Hidrografia Naval, Buenos Aires , 655 : 1 – 76 .
- Balech , E . 1988 . Los dinoflagellados del Atlantico sudoccidental . Publicaciones Especiales Instituto Español de Oceanografia , 1 : 1 – 310 .
- Balech , E . 1989 . Rediscription of Alexandrium minutum Halim (Dinophyceae) type species of the genus Alexandrium . Phycologia , 28 : 206 – 211 .
- Bérard-Therriault , L , Poulin , M and Bossé , L . 1999 . Guide d'identification du phytoplancton marin de l'estuaire et du golfe de Saint-Laurent incluant également certaines protozoaires . Publication spéciale canadienne des sciences halieutiques et aquatiques , 128 : 1 – 387 .
- Boltovskoy , A . 1973 . Peridinium gatunense Nygaard. Estructura y estereoultraestructura tecal (Dinoflagellida) . Physis, Sectión B , 32 : 331 – 344 .
- Cembella , AD . 2003 . Chemical ecology of eukaryotic microalgae in marine ecosystems . Phycologia , 42 : 420 – 447 .
- Dahl , E , Lindahl , O , Paasche , E and Throndsen , J . 1989 . “ The Chrysochromulina polylepis bloom in Scandinavian waters during spring 1988 ” . In Novel Phytoplankton Blooms: Causes and Impacts of Recurrent Brown Tides and other Unusual Blooms , Edited by: Cosper , EM , Bricelj , VM and Carpenter , EJ . 383 – 405 . Berlin, , Germany : Springer Verlag .
- Daugbjerg , N , Hansen , G , Larsen , J and Moestrup , Ø . 2000 . Phylogeny of some of the major genera of dinoflagellates based on ultrastructure and partial LSU rDNA data, including the erection of three new genera of unarmoured dinoflagellates . Phycologia , 39 : 302 – 317 .
- Dodge , JD . 1987 . An SEM study of Peridiniella shaeroidea and P. catenata (Dinophyceae) . Arch. Protistenkd. , 134 : 139 – 148 .
- Felsenstein , J . 1985 . Confidence limits on phylogenies: An approach using the bootstrap . Evolution , 39 : 783 – 791 .
- Fensome , RA , Taylor , FJR , Norris , G , Sarjeant , WAS , Wharton , DI and Williams , GL . 1993 . A classification of living and fossil dinoflagellates . Micropaleontology, Special Publication , 7 : 1 – 351 .
- Frederick , MO , Cole , KP , Pertovic , G , Loizidou , EZ and Nicolaou , KC . 2007 . “ Structural assignment and total synthesis of azaspirazid-1 ” . In Phycotoxins: Chemistry and Biochemistry , Edited by: Botana , LM . 297 – 309 . Oxford, UK : Blackwell Publishing .
- Fritz , L and Triemer , RE . 1985 . A rapid simple technique utilizing Calcofluor white M2R for the visualization of dinoflagellate thecal plates . J. Phycol. , 21 : 662 – 664 .
- Furey , A , Moroney , C , Magdalena , AB , Saez , MJF , Lehane , M and James , KJ . 2003 . Geographical, temporal, and species variation of the polyether toxins, azaspirazids, in shellfish . Environ. Sci. Technol. , 37 : 3078 – 3084 .
- Gribble , KE . 2006 . “ The ecology, life history, and phylogeny of the marine heterotrophic dinoflagellates Protoperidinium and Diplopsalidaceae (Dinophyceae) ” . In PhD thesis , USA : Massachusetts Institute of Technology, Woods Hole Oceanographic Institution .
- Gribble , KE and Anderson , DM . 2006 . Molecular phylogeny of the heterotrophic dinoflagellates, Protoperidinium, Diplopsalis and Preperidinium (Dinophyceae), inferred from large subunit rDNA . J. Phycol. , 42 : 1081 – 1095 .
- Groisillier , A , Massana , R , Valentin , K , Vaulot , D and Guillou , L . 2006 . Genetic diversity and habitats of two enigmatic marine alveolate lineages . Aquat. Microb. Ecol. , 42 : 277 – 291 .
- Guindon , S and Gascuel , O . 2003 . A simple, fast, and accurate algorithm to estimate large phylogenies by maximum likelihood . Syst. Biol. , 52 : 696 – 704 .
- Ito , E , Frederick , MO , Koftis , TV , Tang , W , Petrovic , G , Ling , T and Nicolaou , KC . 2006 . Structure toxicity relationship of synthetic azaspiracid-1 and analogs in mice . Harmful Algae , 5 : 586 – 591 .
- Ito , E , Satake , M , Ofuji , K , Higashi , M , Harigaya , K , Mcmahon , T and Yasumoto , T . 2002 . Chronic effects in mice caused by oral administration of sublethal doses of azaspirazid, a new marine toxin isolated from mussels . Toxicon , 40 : 193 – 203 .
- James , KJ , Furey , A , Lehane , M , Ramstad , H , Aune , T , Hovgaard , P , Morris , P , Higman , W , Satake , M and Yasumoto , T . 2002 . First evidence of an extensive northern European distribution of azaspirazid poisoning (AZP) toxins in shellfish . Toxicon , 40 : 909 – 915 .
- James , KJ , Moroney , C , Roden , C , Satake , M , Yasumoto , T , Lehane , M and Furey , A . 2003 . Ubiquitous “benign” alga emerges as the cause of shellfish contamination responsible for the human toxic syndrome, azaspiracid poisoning . Toxicon , 41 : 145 – 154 .
- Jeong , HJ , Kang , H , Shim , JH , Park , JK , Kim , JS , Song , JY and Choi , HJ . 2001 . Interactions among the toxic dinoflagellate Amphidinium carterae, the heterotrophic dinoflagellate Oxyrrhis marina, and the calanoid copepod Acartia spp . Mar. Ecol. Prog. Ser. , 218 : 77 – 86 .
- Jeong , HJ , Park , KH , Kim , JS , Kang , H , Kim , CH , Choi , HJ , Kim , YS , Park , JY and Park , MG . 2003 . Reduction in the toxicity of the dinoflagellate Gymnodinium catenatum when fed on by the heterotrophic dinoflagellate . Polykrikos kofoidii. Aquat. Microb. Ecol. , 31 : 307 – 312 .
- John , U , Fensome , RA and Medlin , LK . 2003 . The application of a molecular clock based on molecular sequences and the fossil record to explain biogeographic distributions within the Alexandrium tamarense “species complex” (Dinophyceae) . Mol. Biol. Evol. , 20 : 1015 – 1027 .
- Keller , MD , Selvin , RC , Claus , W and Guillard , RRL . 1987 . Media for the culture of oceanic ultraphytoplankton . J. Phycol. , 23 : 633 – 638 .
- Kofoid , CA . 1909 . On Peridinium steini Jörgensen, with a note on the nomenclature of the skeleton of the Peridinidae . Arch. Protistenkd. , 16 : 25 – 47 .
- Krock , B , Tillmann , U , John , U and Cembella , AD . 2008 . “ Characterization of azaspiracids in plankton size-fractions and isolation of an azaspiracid-producing dinoflagellate from the North Sea ” . In Harmful Algae , Epublication ahead of print, doi: 10.1016/j.hal.200806.003
- Lefevre , M . 1932 . Monographie des espèces d'eau douce du genre . Peridinium. Arch. Bot. , 2 : 1 – 208 .
- Lewis , J and Dodge , JD . 1990 . “ The use of the SEM in dinoflagellate taxonomy ” . In Scanning Electron Microscopy in Taxonomy and Functional Morphology , Edited by: Claugher , D . Vol. 41 , 125 – 148 . Oxford, UK : Clarendon Press . The Systematics Association
- Lin , S , Zhang , H and Jiao , N . 2006 . Potential utility of mitochondrial cytochrome b and its mRNA editing in resolving closely related dinoflagellates: A case study of Prorocentrum . J. Phycol. , 42 : 646 – 654 .
- Lindemann , E . 1928 . “ Abteilung Peridineae (Dinoflagellatae) ” . In Die natürlichen Pflanzenfamilien nebst ihren Gattungen und wichtigeren Arten insbesondere den Nutzpflanzen. 2. Auflage, Band. 2 , Edited by: Engler , A and Prantl , K . 3 – 104 . Leipzig, , Germnay : W. Engelmann .
- Loeblich , AR III . 1968 . A new marine dinoflagellate genus, Cachonina, in axenic culture from the Salton Sea, California with remarks on the genus Peridinium . Proc. Biol. Soc. Washington , 81 : 91 – 96 .
- Magdalena , AB , Lehane , M , Krys , S , Fernandez , ML , Furey , A and James , KJ . 2003 . The first identification of azaspiracids in shellfish from France and Spain . Toxicon , 42 : 105 – 108 .
- Maneiro , I , Frangópulos , M , Guisande , C , Fernández , M , Reguera , B and Rivero , I . 2000 . Zooplankton as a potential vector of diarrhetic shellfish poisoning toxins through the food web . Mar. Ecol. Prog. Ser. , 201 : 155 – 163 .
- McMahon , T and Silke , J . 1996 . West coast of Ireland; winter toxicity of unknown aetiology in mussels . Harmful Algae News , 14 : 2
- Miles , CO and Al. , E . 2004 . A novel pectenotoxin, PTX-12, in Dinophysis spp. and shellfish from Norway . Chem. Res. Toxicol. , 17 : 1423 – 1433 .
- Moran , S , Silke , J , Salas , R , Chamberlan , T , Lyons , J , Flannery , J , Thornton , V , Clarke , D and Devilly , L . 2005 . “ Review of phytoplankton monitoring 2005 ” . In 6th Irish Shellfish Safety Scientific Workshop , Galway, , Ireland : Marine Institute .
- Morrill , LC and Loeblich , AR III . 1981 . A survey for body scales in dinoflagellates and a revision of Cachonina and Heterocapsa (Pyrrhophyta) . J. Plankton Res. , 3 : 53 – 65 .
- Nicolaou , KC , Frederick , MO , Pertovic , G , Cole , KP and Loizidou , EZ . 2006 . Total synthesis and confirmation of the revised structures of azaspirazid-2 and azaspirazid-3 . Angew. Chem. Int. Ed. , 45 : 2609 – 2615 .
- Okolodkov , YB and Dodge , JD . 1995 . Redescription of the planktonic dinoflagellate Peridiniella danica (Paulsen) comb. nov. and its distribution in the N.E. Atlantic . Eur. J. Phycol. , 30 : 299 – 306 .
- Posada , D and Buckley , TR . 2004 . Model selection and model averaging in phylogenetics: advantages of the AIC and Bayesian approaches over likelihood ratio tests . Syst. Biol. , 53 : 793 – 808 .
- Posada , D and Crandall , KA . 1998 . Modeltest: testing the model of DNA substitution . Bioinformatics , 14 : 817 – 818 .
- Ramsfjell , E . 1959 . Two new phytoplankton species from the Norwegian Sea, the diatom Coscinosira poroseriata, and the dinoflagellate Gonyaulax parva . Nytt Magas. Botanikk , 7 : 175 – 177 .
- Rehmann , N , Hess , P and Quilliam , MA . 2008 . Discovery of new analogs of the marine biotoxin azaspirazid in blue mussels Mytilus edulis by ultra-performance liquid chromatography/tandem mass spectrometry. Rap . Commun. Mass Spektrom. , 22 : 549 – 558 .
- Saldarriaga , JF , Taylor , FJR , Cavalier-Smith , T , Menden-Deuer , S and Keeling , PJ . 2004 . Molecular data and the evolutionary history of dinoflagellates . Eur. J. Protistol. , 40 : 85 – 111 .
- Saldarriaga , JF , Taylor , FJR , Keeling , PJ and Cavalier-Smith , T . 2001 . Dinoflagellate nuclear SSU rRNA phylogeny suggests multiple plastid losses and replacements . J. Mol. Evol. , 53 : 204 – 213 .
- Satake , M , Ofuji , K , James , K , Furey , A and Yasumoto , T . 1998 . “ New toxic events caused by Irish mussels ” . In Harmful Algae , Edited by: Reguera , B , Blanco , J , Fernandez , ML and Wyatt , T . 468 – 469 . Spain : Xunta de Galicia and International Oceanographic Commission of UNESCO, Santiago de Compostela .
- Schiller , J . 1935 . “ Dinoflagellatae (Peridineae) in monographischer Behandlung ” . In Dr L. Rabenhorst's Kryptogamen-Flora von Deutschland, Österreich und der Schweiz , Edited by: Rabenhorst , L . 161 – 320 . Leipzig, , Germany : E. Kummer .
- Stein , F . 1883 . Der Organismus der Infusionsthiere. 3. Abt. Der Organismus der Arthrodelen Flagellaten nach eigenen Forschungen in systematischer Reihenfolge bearbeitet. 2. Hälfte. Einleitung und Erklärung der Abbildungen , Leipzig, , Germany : W. Engelmann .
- Taleb , H , Vale , P , Amanhir , R , Benhadouch , A , Sagou , R and Chafik , A . 2006 . First detection of azaspirazids in mussels in north west Africa . J. Shellfish Res. , 25 : 1067 – 1070 .
- Thompson , JD , Gibson , TJ , Plewniak , F , Jeanmougin , F and Higgins , DG . 1997 . The ClustalX windows interface: flexible strategies for multiple sequence alignment aided by quality analysis tools . Nucl. Acid Res. , 25 : 4876 – 4882 .
- Twiner , MJ , Rehmann , N , Hess , P and Doucette , GJ . 2008 . Azaspiracid shellfish poisoning: A review on the chemistry, ecology, and toxicology with emphasis on human health impacts . Mar. Drugs , 6 : 39 – 72 .
- Twiner , MJ , Hess , P , Dechraoui , MYB , Mcmahon , T , Samons , MS , Satake , M , Yasumoto , T , Ramsdell , JS and Doucette , GJ . 2005 . Cytotoxic and cytoskeletal effects of azaspiracid-1 on mammalian cell lines . Toxicon , 45 : 891 – 900 .
- Vale , C , Botelho , MJ , Rodrigues , SM , Gomes , SS and De M. Sampayo , MA . 2008 . Two decades of marine biotoxin monitoring in bivalves from Portugal (1986–2006): A review of exposure assessment . Harmful Algae , 7 : 11 – 25 .
- Whelan , S and Goldman , N . 2001 . A general empirical model of protein evolution derived from multiple protein families using a maximum likelihood approach . Mol. Biol. Evol. , 18 : 691 – 699 .
- Yasumoto , T . 2001 . The chemistry and biological function of natural marine toxins . Chemical Record , 1 : 228 – 242 .
- Zapata , M , Rodriguez , F and Garrido , JL . 2000 . Separation of chlorophylls and carotenoids from marine phytoplankton: a new HPLC method using reverse phase C8 column and pyridine-containing mobile phase . Mar. Ecol. Prog. Ser. , 195 : 29 – 45 .
- Zhang , H , Bhattacharya , D and Lin , S . 2005 . Phylogeny of dinoflagellates on mitochondrial cytochrome b and nuclear small rDNA . J. Phycol. , 41 : 411 – 420 .
- Zhang , H , Bhattacharya , D and Lin , S . 2007 . A three-gene dinoflagellate phylogeny suggests monophyly of Prorocentrales and a basal position for Amphidinium and Heterocapsa . J. Mol. Evol. , 65 : 463 – 474 .