Abstract
Within the Bangialean red algae the multi-copy small subunit nuclear ribosomal gene (SSU) has been shown to contain group-I introns at positions corresponding to 516 and 1506. Using the polymerase chain reaction (PCR) with primers flanking the 1506 intron we found that this intron was present in some copies of the rDNA in several North Atlantic Porphyra species. However, when the combination of a flanking and internal primer was used to amplify across the intron the percentages of individuals containing the intron increased. The likely explanation for these results was that, when flanking primers were used for PCR, template competition favoured the amplification of the intronless rDNA fragments. A combination of internal and flanking primers used to screen for the 516 intron amplified the intron in every accession of five taxa (Porphyra dioica, P. leucosticta, P. suborbiculata, P. umbilicalis and P. yezoensis) and in most samples of P. amplissima, P. linearis, and P. purpurea. When PCR primers were optimized for the 1506 intron and SSU of P. umbilicalis, the intron was detected in 28/28 samples. Such observations suggest that the 1506 intron is ubiquitous in Porphyra and is present in some, but not all, copies of the ribosomal DNA (rDNA) repeat. Multiple size 1506 introns were detected in individuals of P. umbilicalis. Four of the larger size variants were shown to encode a putative His–Cys box that was associated with mobility of other group-I introns. Our observations suggest that the 1506 group-I intron may still be mobile within the Porphyra lineage.
Introduction
The nuclear ribosomal small subunit RNA (SSU) and the chloroplast ribulose bisphosphate carboxylase oxygenase (rbcL) genes have been used to clarify phylogenetic relationships for many Porphyra species (Freshwater et al., Citation1994; Ragan et al., Citation1994; Oliveira et al., Citation1995; Kunimoto et al., Citation1999 a, Citation b ; Broom et al., Citation2002; Klein et al., Citation2003; Lindstrom & Fredericq, Citation2003). The more variable ribosomal nuclear internal transcribed spacer (ITS) and/or ribosomal group-I introns have been utilized to resolve relationships among more closely related species (Kunimoto et al., Citation1999 b; Müller et al., Citation2001; Broom et al., Citation2002).
Using primers designed to anneal to the conserved coding regions, polymerase chain reaction (PCR) amplification can be directed across the more variable intron regions (Slade et al., Citation1993). The method has been called Exon Primed Intron Crossing PCR (EPIC-PCR; Palumbi & Baker, Citation1994) and has been the primary method employed for intron detection and amplification.
The red algal order Bangiales contains introns in the nuclear-encoded SSU genes (Oliveira & Ragan, Citation1994; Oliveira et al., Citation1995; Müller et al., Citation1998, Citation2001; Milstein & Oliveira, Citation2005). These introns encode self-splicing RNAs (also known as ribozymes or group-I introns) or their remnants that have been extensively studied in diverse organisms, including eubacteria, fungi, plants, and protists (Belfort, Citation1991; Damberger & Gutell, Citation1994; Yamada et al., Citation1994; Gargas et al., Citation1995; Hibbett, Citation1996; Booton et al., Citation2004). The two most frequently found intron insertion sites of the SSU gene are at base pairs 516 and 1506 [according to SSU rDNA gene sequence numbering of Escherichia coli (Migula) Castellani & Chalmers]; these also correspond to those predicted at helices 21 and 50 in the secondary structure of this RNA (Brimacombe et al., Citation1988; Neefs et al., Citation1993). Group-I introns are characterized by a conserved primary and secondary RNA structure (Kruger et al., Citation1982; Cech, Citation1990), which has allowed further classification of these introns into subgroups (Michel & Westhof, Citation1990; Suh et al., Citation1999). Oliveira & Ragan (Citation1994) described the occurrence of a group-IC1 intron in the 18S ribosomal RNA genes at 1506 of Porphyra spiralis var. amplifolia Oliveira et Coll that differed in size between three distinct populations.
The sporadic and broad distribution of group-I introns in the small and large subunits of the rDNA repeats suggests that intron sequences are highly successful at invading and maintaining themselves in eukaryotic genomes (Bhattacharya et al., Citation1994, Citation1996; Bhattacharya, Citation1998; Friedl et al., Citation2000). A study by Bhattacharya et al. (Citation2001) on the diverse phylogenies of eukaryotic group-IC1 introns showed that the 516 introns in Bangialean red algae and a brown alga (Aureoumbra lagunensis Stockwell, DeYoe, Hargraves et Johnson) were closely related. Bhattacharya and co-workers (2001) concluded that it is unlikely that the group-IC1 introns in the SSU were inherited vertically from a common ancestor of the red and brown algae because these algal lineages do not share a close evolutionary relationship.
Müller et al. (Citation2001) surveyed the SSU 516 and 1506 (helices 21 and 50) group-ICI introns in a large number of Bangialian species in order to evaluate their utility for phylogenetic and biogeographic studies. They used EPIC primers to amplify both introns, which were subsequently sequenced for phylogenetic analysis. Many individuals lacked either one or both of the group-I introns and there were both inter- and intra-specific size variants. Inter-specific sequence variation between taxa for both introns ranged from 0–44.3%. Based on the intron and rRNA phylogenies of the same accessions, Müller et al. (Citation2001) hypothesized that the ribosomal group-I introns were inherited vertically and frequently lost in recently radiated taxa. In contrast to the proposal by both Oliveira & Ragan (Citation1994) and Kunimoto et al. (Citation1999 a, Citation b ), that the SSU introns would be useful to differentiate populations in Porphyra or Bangia for biogeographic studies, Müller et al. (Citation2001) concluded that the SSU introns were not useful for such purposes.
Hibbett (Citation1996) examined the utility of internal primers when amplifying group-I introns in members of the homobasidiomycetes (mushroom-forming fungi). While developing a PCR assay for intron detection, he found that an internal intron primer paired with a flanking primer in the SSU allowed detection of introns that would otherwise go undetected by EPIC PCR. Hibbett (Citation1996) described two instances of false negative results for intron detection in Lentinellus ursinus (Fries) R. Kühner and L. montanus O. K. Miller. He attributed the failure of the intron amplification (using EPIC primers) to low copy numbers of intron-containing rDNA repeats and a PCR artefact called template preference (Sefc et al., Citation2003). Hibbett (Citation1996) concluded that, for any study focussing on the distribution of introns, it was essential to use intron-specific PCR primers to assess their presence or absence, especially when related taxa were known to contain an intron.
The present study builds on an earlier investigation of intra- and inter-specific diversity of North Atlantic Porphyra (Klein et al., Citation2003) by surveying a large collection of Porphyra for the presence of group-I introns in the SSU and characterizing their size, structure, and utility for phylogeographic studies. Both flanking (EPIC) and internal ‘intron-specific’ PCR primers were developed and used to screen for the 1506 SSU nuclear ribosomal intron previously described in Porphyra.
The present paper summarizes the occurrence of ribosomal group-I introns in several North Atlantic Porphyra taxa and the Asiatic species P. yezoensis Ueda; it shows how the application of intron-specific primers enhances the detection of introns within the SSU gene. This study addresses discrepancies between the conclusions of previous researchers (Oliveira & Ragan, Citation1994; Kunimoto et al., Citation1999 b; Müller et al., Citation2001) about the utility of the 1506 group-I intron as a variable locus for population differentiation, phylogography and phylogenetics. Group-I introns were sequenced from representative Porphyra specimens and a detailed analysis of intron distribution in Porphyra umbilicalis Kützing performed to determine intra-specific variation within the 1506 intron.
Materials and methods
Sample collections and identification
Samples of Porphyra were collected from 88 sites along the coast of New England and the Canadian Maritimes as described in Klein et al. (Citation2003). Collections of all conspicuous Porphyra taxa at each site were made within the intertidal (on foot) and shallow subtidal zones (by SCUBA). Voucher specimens of each population were prepared (approximately 25 fronds/site) and deposited in the Albion R. Hodgdon Herbarium (NHA) at the University of New Hampshire in order to document temporal, spatial and biological characteristics of the different taxa (cf. Mathieson & Hehre, 1986). Mathieson has collected along the coast of New England USA and the Canadian Maritimes for more than 40 years and considers these sites to be representative of the range of habitats in which Porphyra occurs. Twenty-eight additional samples of P. umbilicalis were collected from various locations in the Northwest and Northeast Atlantic (). All samples were identified to species following morphological methods detailed in Klein et al. (Citation2003); these identifications were then confirmed using several molecular methods, including direct sequencing, species-specific PCR, or an RFLP assay using the chloroplast rbcL gene (Teasdale et al., Citation2002).
Table 1. A list of Porphyra containing the 1506 ribosomal introns sequenced for phylogenetic and intra-specific analysis.
DNA extraction and amplification, plus sequencing of introns
DNA was extracted by a modified cetyltrimethylammonium bromide (CTAB) method (Stiller & Waaland, Citation1993), as described in Klein et al. (Citation2003). Porphyra umbilicalis samples were extracted using the Puregene® DNA Isolation Kit for plant tissue (#D5500A; Gentra Systems, Minneapolis, USA).
Purified DNA samples (5–10 ng/µl) were transferred to 96-well microtitre plates for high throughput analysis. High throughput screening was carried out by transferring 4 µl of template DNA to separate 96-well plates and performing PCR using locus-specific primers (SSU group-I introns or the rbcL gene). In other assays, individual aliquots were added to 0.2-ml thin-walled tubes for polymerase chain reaction (PCR).
Flanking consensus primers for the 1506 intron were designed in Primer Select (Lasergene™, DNASTAR, Madison, WI) from approximately 950 bp of SSU rDNA sequence previously generated from Porphyra dioica Brodie et Irvine, P. linearis Greville P. leucosticta Thuret in Le Jol., P. miniata (C. Agardh) C. Agardh, P. purpurea (Roth) C. Agardh (Klein et al., Citation2003), P. spiralis var. amplifolia (GenBank Accessions L26175-L26177; Oliveira & Ragan, Citation1994), P. suborbiculata Kjellman and P. umbilicalis. Samples were screened for SSU introns using either a set of flanking primers (porint1: 5′-TGTACACACCGCCCGTC-3′; porint2: 5′-CTGATCCTTCTGCAGGTTCACCTAC-3′) or a combined flanking and internal primer set (porint5: 5′-TTGGGGGCATTCGTATTTCAT-3′; porint6: 5′-GGCTGCAAAGGCTTCGGTA-3′; see Supplemental Material A, available at http://www.informaworld.com/mpp/uploads/teasdale_et_al._supplemental_material.zip). Primers porint5 and porint6 were designed from a consensus of partial intron sequences from multiple Porphyra species. Primers were also designed to amplify the 516 intron: the internal intron primer Int5pr1: 5′-AGGCGAACCTTCAGAGACT-3′ and the SSU flanking primer Int5pr2: 5′-CAGACAAATCACTCCACCAA-3′. Species-specific primers were developed for P. umbilicalis: the SSU flanking primer was H50-1: 5′-GAAGGAGAAGTCGTAACAAGGTTT-3′; the 1506 intron internal primer was H50-2: 5′-CAGGGGACCGACTGTCTCTTA-3′. To determine the exact intron insertion points, which are located near the 3′ end of SSU, additional primers were designed for amplification or sequence of the P. umbilicalis 1506 intron: H50-FL 5′-GAGGAAGGAGAAGTCGTAACAA-3′ and UMB-ITS1-R: 5′-TATCCACCGTTAAGAGTTGTAT-3′.
Reagents for each 50-µl PCR reaction contained 1X Magnesium Free Reaction Buffer B (Promega, Madison WI), 0.2 mM each dNTP (Promega), 0.5 units of Taq DNA polymerase (Promega), 0.4 µM each primer, and variable MgCl2 concentrations (depending on primer set) given in . Amplification profiles for each primer set are given in and were carried out in an MJ Research PTC-100 Programmable Thermal Controller (Watertown MA). The PCR products were visualized after gel electrophoresis and staining with ethidium bromide.
Table 2. PCR profiles used for group-I intron amplification.
Sequences of the 1506 intron were determined for individual accessions of P. dioica, P. linearis, P. leucosticta, P. miniata, P. purpurea, P. suborbiculata, and 28 different geographic isolates of P. umbilicalis. The collection site of each sample, representative herbarium specimen (if available), and the corresponding GenBank Accession information are given in . The PCR products were separated by gel electrophoresis on 0.9% low melting point agarose (Life Technologies, Gaithersburg, USA), excised from the gel, and digested with agarase according to the manufacturer's directions (Sigma, St. Louis, USA). Cycle sequencing was done according to manufacturer's instructions using the ABI PRISM Taq DyeDeoxy Terminator Cycle Sequencing Kit with AmpliTaq DNA Polymerase (P/N 401384; Perkin Elmer, Foster City, CA) using 10 pmol of primer and 30–90 ng of template DNA. The reaction products were separated and analysed on an ABI PRISM 377 Automated Sequencer (UNH Sequencing Facility). Sequences were generated with ABI DNA Sequencing Software version 2.1.1 and edited using SeqEd Software (version 1.0.2; ABI, Foster City, USA). Both strands were sequenced for the 1506 intron for each species. Contiguous sequences were assembled and aligned using SeqMan (DNASTAR) algorithms. GenBank accessions for sequenced samples are given in .
Alignment of 1506 group-I introns and phylogenetic analyses
Initial alignments of the 1506 group-I intron sequences from select Porphyra taxa were made using the ClustalW algorithm in Megalign (DNASTAR). The intron sequences were manually aligned in Bioedit Vers. 5.0.6 (North Carolina State University), which is an alignment program that can incorporate secondary structure information. The computer alignments (Supplemental Material B; available at http://www.informaworld.com/mpp/uploads/teasdale_et_al._supplemental_material.zip) were compared with secondary-structure models with the aid of a group-I intron database (Damberger & Gutell, Citation1994), conserved base-paired segments or ‘P’ domains (Michel & Westhof, Citation1990), and the secondary structure folding program mfold version 3.0 (Mathews et al., Citation1999). The group-IC1 intron of Hildenbrandia rubra (Sommerfelt) Meneghini (GenBank accession #L19345, Ragan et al., Citation1993) was used as an outgroup in the phylogenetic analyses. Gaps were treated as missing data.
Phylogenetic inference was first performed by maximum parsimony (MP) analyses using PAUP* (Swofford, Citation2002), with a full heuristic search strategy that first involved 200 replicates with RANDOM taxon addition with the tree-bisection-reconnection (TBR) branch-swapping algorithm. Initial searches found the 10 shortest trees, which were then used as starting trees in searches that used TBR branch-swapping; only the most parsimonious tree was saved from this analysis. To assess support for the inferred relationships, bootstrap analyses (Felsenstein, Citation1985) were conducted with 1000 replicates. Maximum-likelihood (ML) trees were built using PAUP* (Swofford, Citation2002) assuming both a General Time Reversible (GTR) model and a non-coding gamma distributed rate estimation. The maximum parsimony tree (see above) was used to initiate a heuristic search for the most likely ML tree. Based on prior work by Wheeler & Honeycutt (Citation1988), who showed that paired nucleotides forming stem regions appear to undergo compensatory mutations that maintain secondary structure, a weighted parsimony analysis was done using the stem/loop definitions described in Soltis & Soltis (Citation1998) with stem bases assigned a weight of 1 and loop bases a weight of 2. A weighted bootstrap parsimony search was conducted as previously described with the equally weighted characters.
To test for data set incongruence between the 1506 group-I intron dataset and a previously published SSU phylogeny of North Atlantic Porphyra (Klein et al., Citation2003) the SSU dataset was re-analysed with those taxa used in the group-I phylogeny. A partition homogeneity test (PHT; Swofford, 1998), also known as incongruence-length difference (ILD; Farris et al., Citation1994, Citation1995), was performed using PAUP*. Only those taxa that were available for both data sets were examined. A random addition heuristic search was conducted using 100 replicates; the resulting p-value determined the probability of rejecting the null hypothesis of congruence (or homogeneity) of the two data sets.
Results
Amplification survey of group-I ribosomal introns in North Atlantic Porphyra
Flanking primers (EPIC) porint 1 + 2 were used to screen 742 individuals representing 88 collection sites and nine different species for the presence of the 1506 intron. All samples were scored according to the size of the intron, which was calculated from the size of the amplification product minus the number of SSU flanking bases (i.e. the 730-bp amplification product contained a 555-bp intron). Typical results of the PCR assay are shown in . Lane 1 shows the amplification products from a P. purpurea sample: a high molecular weight band corresponding to a 645 bp intron and a low molecular weight fragment representing primer-dimer. Sequencing confirmed placement of the intron at the expected insertion site, while the complementary 18S sequence was at the ends of the amplified fragments. Lane 2 (also P. purpurea) shows the primer-dimer band and a short fragment corresponding to the amplification product across an rDNA lacking the 1506 intron. For the 742 individuals sampled six different intron sizes were estimated from agarose gels: ∼425 bp, 555 bp, 645 bp, 725 bp, 825 bp and 1075 bp (). In some cases the intron size classes were clustered by species: the 645 bp intron was most common in P. purpurea (lane 1), whereas the 425 bp introns only amplified from P. yezoensis samples. Most species seemed to share size variants or had multiple size introns (data not shown) with the 555 bp intron being the most common size class found in six of the nine species surveyed. The EPIC amplification of P. umbilicalis DNA in this subset (; lanes 11–14; 17–19) produced fragments in the 174 bp range only, the amplification size predicted if no introns were present at the 1506 position in the SSU gene.
Fig. 1. An agarose gel (representing a subset of 742 Porphyra samples) illustrating an initial amplification screening for the presence of 1506 introns with flanking primers porint1 and porint2. The lanes show variable size introns or templates that lacked intron amplification. All fragments showing the 174 bp band size represent samples lacking the 1506 intron (shown as the top band of a doublet, the lower band represents primer dimer). The first lane (M) is a ΦX/Hae III DNA size marker. Numbers attached to arrows represent amplification size class and actual intron size (parentheses) in bp. Lane 1–2: P. purpurea (Orr's Island, Maine); 3–10: P. linearis (Rachel Carson Salt Pond Reserve, Bristol, Maine); 11–15, 17–19: P. umbilicalis (Peak's Island, Casco Bay, Maine); 16: Negative control; 20: P. linearis (Old Soaker, Mount Desert Island, Maine).
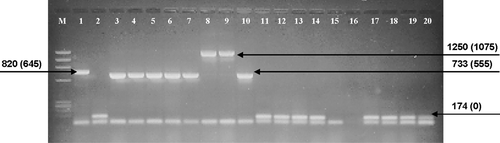
Table 3. Initial screening for the 1506 intron from North Atlantic Porphyra collections using flanking primers porint1 and porint 2.
Subsequently, a subset of the entire Porphyra collection (see Supplemental Materials A) was examined to evaluate whether the EPIC screen detected all 1506 introns (). In the second screen, PCR amplifications were carried out with an internal primer (porint6), designed against a consensus of Porphyra 1506 intron sequences plus a flanking primer (porint5) corresponding to the consensus of upstream SSU sequence. Of the two species with low intron occurrence in the EPIC screen, P. miniata and P. umbilicalis both showed a substantial increase in the detection of the 1506 intron when using the porint5 and porint6 primer combination: from 0 → 2 in P. miniata, and most dramatically 1 → 26 for P. umbilicalis. There were significant increases for introns detection for several other taxa (P. purpurea, P. linearis.)
Table 4. The number of 1506 intron amplifications in a sub-sample (total N = 181) from the Porphyra survey. Table shows the difference in amplifications between using only flanking primers (porint 1 & porint 2) or the combination of a flanking and intron specific primer combination (porint 5 and porint 6).
Using a subset of the same individuals from the 1506 EPIC screen, 88 individuals from all nine Porphyra species were screened for the 516 intron. The PCR primers represented an intron-specific (int5pr-1) and an rDNA-specific primer (int5pr-2) designed against a consensus sequence for multiple species. The 516 intron was detected at high levels in all species except P. miniata. In five of the nine species (P. dioica, P. leucosticta, P. suborbiculata, P. umbilicalis, and P. yezoensis) the 516 intron was detected in every individual screened ().
Table 5. Amplification results of 516 Group-I introns using the Int5pr1 and Int5pr2 (intron specific) primer pair from a survey of 88 Porphyra individuals.
The previous screens were carried out using primers designed against consensus sequences from multiple Porphyra species. New species-specific primers were designed against P. umbilicalis SSU (H50-1, flanking) and 1506 sequence (H50-2, internal). The primers were used to screen for the presence of the 1506 group-I intron in 28 individuals from the Northeast and Northwest Atlantic (). In every case the combination of an internal intron and flanking primer supported amplification of a fragment containing part of the 1506 intron. The sequences corresponding to the partial intron were at least 548 bp in length. Therefore, the 1506 SSU intron is present in at least one copy of the ribosomal repeat in every individual of P. umbilicalis.
The P. umbilicalis intron sequences (18 European, 10 North American) were easy to align because they exhibited considerable sequence conservation. In some samples PCR amplification with primers H50-1 and H50-2 also produced higher molecular weight bands (see below).
Presence of an open reading frame in large size variants of the 1506 intron
Using a second primer pair with a reverse 3′ primer in the adjacent internal transcribed spacer (ITS) region, full-length intron sequences for P. umbilicalis were obtained from four samples (ENG-1, IRE-2, NWY-7, NWY-8). After subtracting rDNA sequences and correcting for the overlap of the two partial 1506 intron fragments the full size of the intron was ∼710 bp. Amplification of the 1506 intron in three Northeast Atlantic P. umbilicalis specimens (NWY-7, NWY-8, ENG-1) using the H50-1 and H50-2 primers produced a second, larger amplification product for which the intron size was determined to be 1188 bp. Alignment of both the smaller and larger intron sequences showed that the difference was due to an indel of 478 bp near the 5′ end relative to the smaller intron. A pairwise sequence comparison between both size classes in all three individuals showed that the smaller intron had 96.2–100% sequence similarity and the larger one 99.8–100% sequence similarity. Strikingly, there was less sequence similarity (93.1–94.2%) between the two intron size classes. The fourth individual (IRE-2) contained the larger size intron variant but the smaller intron was not detected.
The Open Reading Frame (ORF) Finder at the National Center for Biotechnology Information (NCBI) (http://www.ncbi.nlm.nih.gov/gorf/gorf.html) was used to analyse the 1188-bp 1506 introns. A 155 amino acid ORF was found in the large 1506 intron for IRE-2 (AY613848) on the complementary strand to that encoding the SSU rRNA. The other three 1188-bp 1506 introns had a stop codon at Glutamate 105, but the last 1/3 of the ORF was conserved with several amino acid substitutions. The 1506 intron ORF was compared with other protein coding sequences in GenBank and encodes a putative His–Cys Box endonuclease. The amino acid sequences from all four of the large size variants were aligned to similar sequences predicted from the 1506 ribosomal intron in the Bangiophyceae (Porphyra spiralis, GenBank #L26177; and Bangia fuscopurpurea, GenBank #L36066) as well as the endonuclease I-PpoI (GenBank L03183) (). The amino acid sequence for IRE-2 (GenBank #AY613848) was identical in 14 amino acids with that of the I-Ppo I endonuclease His–Cys box. All nine residues proposed to be part of the Zn binding domain and amino residues in the active site of the I-PpoI were conserved in the Porphyra large intron ORF. The amino-acid sequences for the putative His–Cys box in the other three P. umbilicalis samples were less conserved, i.e. only 12 amino acids were identical.
Fig. 2. Similar putative endonuclease sequences from the Bangiophyceae within the 1506 ribosomal group-I intron. The amino acid sequence of the Porphyra umbilicalis intron ORF found in four individuals (Pumb) is aligned to the corresponding sequences from Porphyra spiralis (Psp 1), and Bangia atropurpurea (Bat 1) as given in Haugen et al. (Citation1999). Identical positions are indicated by dots, deletions by dashes. The His-Cys box motif ( bold line) is compared to the homing endonuclease I-PpoI. Conserved residues proposed to be directly involved in zinc binding (C100, C105, H110, C125, C132, H134, C138) and the active site (H98, N119) of the I-PpoI endonuclease (Flick et al., Citation1998) are indicated by shading.
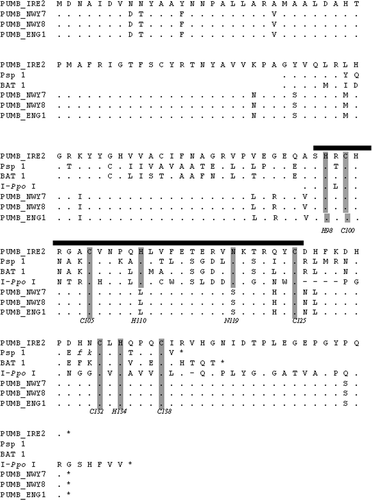
Phylogeny of seven North Atlantic Porphyra inferred from the 1506 intron
The 544 bp fragments of the smaller 1506 intron from seven Porphyra taxa were easily aligned against conserved secondary structure domains. The P, Q, R, and S functional core domains were highly conserved across all Porphyra species and only slightly different for the outgroup taxon, H. rubra. The conserved P domains or ‘stem’ regions showed minimal variation between species, except for the P8 region that showed high levels of variation between taxa (see Supplemental Materials B).
Of the 544 nucleotide positions aligned, 143 were parsimony-informative, primarily from the variable loop domains. Heuristic searches using equal weighting of all characters found one minimal-length tree of 495 steps (consistency index [CI] = 0.8566; retention index [RI] = 0.690). The strict consensus of the most parsimonious tree is given in . Using the red alga H. rubra as a phylogenetic outgroup, the seven North Atlantic Porphyra species formed two well-supported clades. Porphyra miniata, P. purpurea, and P. suborbiculata formed the first clade (bootstrap value = 99%), while the sister clade included P. dioica, P. leucosticta, P. linearis, and P. umbilicalis (bootstrap value = 80%).
Fig. 3. Phylogram of the 1506 Group-I Intron from seven North Atlantic Porphyra using Maximum Parsimony analysis. Numbers represent bootstrap support from resampling of 1000 replicates. Parentheses = bootstrap support from weighted analysis.
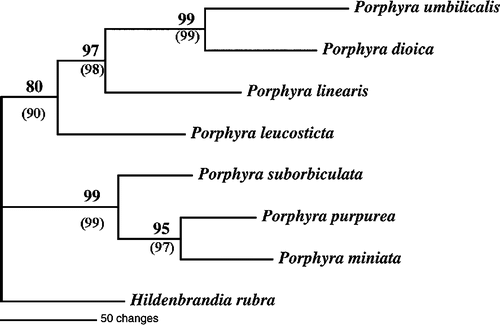
The tree topology was similar for both MP () and ML (not shown) analyses. Parsimony analyses that differentially weighted stem versus loop bases did not change the overall topology of the phylogenetic trees (data not shown). The major difference between the weighted and equal weighting trees was the stronger bootstrap support of species relationships in the weighted phylogram (). In general, differential weighting of stems versus loops did not substantially alter the results obtained using equal weighting of characters.
Discussion
The distributions of 1506 and 516 group-I ribosomal introns within North Atlantic Porphyra were assessed using two different strategies for PCR amplification. The 1506 introns were further characterized by sequencing. The results support the following conclusions: (i) PCR using EPIC and/or non-species specific primer design under-estimates the presence of group-I introns in members of the Bangiales; (ii) the rDNA repeats within an individual Porphyra sample are heterogeneous for the presence or absence of the 1506 group-I intron; and (iii) rDNA group-I introns probably exist in all species of Porphyra. Some of these results differ from an earlier assessment of ribosomal group-I intron distribution in the Bangiales by Müller et al. (Citation2001), suggesting that their results should be re-evaluated.
Several previous surveys for the presence of group-I introns in Porphyra used flanking or EPIC primers (Kunimoto et al. Citation1999 a, Citation b ; Müller et al., Citation2001; Broom et al., Citation2002). In each of these studies the presence of a 516 or a 1506 intron was reported in some but not all accessions of each taxon. In our initial survey of 742 Porphyra accessions from the Northwest Atlantic using EPIC primers designed against conserved regions of the SSU we observed similar results for the 1506 intron. The frequency of detecting the 1506 intron varied between 1.4 and 100% in the taxa surveyed. Notably the 1506 intron was observed in only three individuals among 218 accessions of P. umbilicalis. However, when a combination of intron-internal and intron-flanking (consensus) primers were used to screen DNAs from a subset of 88 of the 742 Porphyra accessions, the frequency of detecting the two helix introns (1506 and 516) increased. For example the 1506 intron was detected in 90% of the P. umbilicalis samples while the 516 intron was detected in 73% of the individuals.
While our observations suggest that a combination of flanking and intron-internal primers enhance the rate at which both 516 and 1506 introns are detected, comparing these results with EPIC primer screens of the same samples, suggests that the rDNA repeats in each Porphyra accession are heterogeneous. That is, some copies of the SSU in the rDNA repeat have either the 516 or 1506 introns or both, while other copies of the rDNA repeat in the same individual do not. A phenomenon called short allele dominance or template preference (Wattier et al., Citation1998; Sefc et al., Citation2003) may explain why several earlier investigators (Kunimoto et al., Citation1999 a, Citation b ; Müller et al., Citation2001; Broom et al., Citation2002) failed to detect the SSU group-I introns in various accessions using EPIC PCR. Thus, where two templates (heterogeneous rDNA repeats) with identical primer sites exist, the shorter template (no intron) out-competes the larger one during early rounds of PCR and only the shorter fragment is observed following amplification and gel fractionation. Although no quantitative analysis was done to determine how many copies of the small ribosomal subunit might contain a group-I intron, we speculate that intron detection by EPIC in an individual sample may indicate that certain Porphyra isolates have a higher level of intron-containing rDNA than rDNA that lacks the intron. In the original paper documenting the presence of group-I introns in the SSU of Porphyra, Oliveira & Ragan (Citation1994) report that by varying PCR conditions they could observe “intronless SSU rDNAs in at least some individuals of Porphyra spiralis var. amplifolia”.
When species-specific intron/flanking primers were developed for the 1506 intron of P. umbilicalis the frequency of detecting this intron increased to 100% of the samples screened (n = 28). Given that there is sequence variation in both the rDNA flanking sequences and within the rDNA group-I introns between Porphyra taxa, species-specific primers are needed to maximize intron amplification compared with the rDNA repeats in which the introns occur.
Larger size variants of the 1506 intron in Porphyra umbilicalis encode a His–Cys box similar to a PpoI endonuclease
As in this study, Oliveira & Ragan Citation1994 and Müller et al. (Citation2001) observed some Porphyra individuals with larger size variants of the 516 and 1506 introns. This suggests that gene conversion mechanisms are not homogenizing all rDNA cistrons. Our observations that, at a sequence level, 1506 introns of similar size are more similar between different algal accessions than different size 1506 introns within an individual may result from both horizontal transmission and pseudogene evolution as outlined below.
For horizontal transmission of introns to occur there must be mechanisms at either the RNA or DNA level to promote intron insertion. Intron spread at the DNA level depends on the expression of intron-encoded enzymes or Homing Endonuclease Genes (HEGs) found within some group-I introns (Lambowitz & Belfort, Citation1993; Belfort & Roberts, Citation1997; Johansen et al., Citation1997). The homing activity refers to the intron's ability to move horizontally into an intron-less allele of the same genome, or into a new genome. One group or ‘family’ of these endonucleases that is made up exclusively of nuclear-encoded enzymes is characterized by a conserved His–Cys box motif (Johansen et al., Citation1993). Putative pseudogenes for this homing endonuclease have been observed in larger size variants of the 1506 intron (Oliveira & Ragan, Citation1994; Müller et al., Citation2001; present study). Finally, if horizontal transfer is occurring within Porphyra or Eukaryota in general it must have a vector to allow such a transmission. Bhattacharya et al. (Citation1996) reported that based on phylogenetic evidence, the ribosomal introns at position 1512 of the SSU in green algae may be of viral origin. It is unclear how widespread viruses are among marine algae, but it has long been known that they may act as vectors of nucleic acids. If a virus is capable of infecting very closely related taxa such as different Porphyra species, it is most likely also capable of transferring nucleic acids horizontally between various hosts.
As in previous studies (Oliveira & Ragan, Citation1994; Müller et al., Citation2001) large size variants (+478 bp) of the 1506 intron were observed in four accessions of P. umbilicalis (IRE-2, ENG-1, NWY-7, NWY-8). The ORF in IRE-2 is 155 amino acids in length and encodes all of the conserved zinc-binding and active site residues associated with a homing-type endonuclease I-PpoI endonuclease. This contrasts with Müller et al. (Citation2001) who did not detect any large ORFs. The ORF in IRE-2 also appears to have an extended C-terminal end when compared with other endonuclease-like sequences (Haugen et al., Citation1999) from bangiophyceaen 1506 group-I introns. Unlike the sequences described in Haugen et al. (Citation1999), no frameshifts were apparent in IRE-2 or the remaining three large size variant 1506 intron P. umbilicalis sequences: ENG-1, NWY-7, NWY-8. However, unlike IRE-2 these three sequences have ‘in frame’ stop codons at position 105 (GLU) and may represent pseudogenes. The same three intron sequences showed polymorphisms compared with both IRE-2 and the His–Cys box motif of the endonuclease I-PpoI. These polymorphisms resulted in the loss of two I-PpoI conserved amino acids (H110, N119; Flick et al., Citation1998).
How widespread the putative homing endonucleases are in Porphyra is unclear. The large size variants of the 1506 introns were only detected in four of 28 P. umbilicalis accessions from the Eastern and Western North Atlantic. Larger size variants for the 1506 intron were also detected in several accessions of P. linearis (data not shown). Again the phenomenon of short allele dominance may mask the frequency at which these ORF or pseudogene-containing 1506 introns occur. Goddard & Burt (Citation1999) postulated that the HEG containing group-I introns can easily increase in frequency within a population until fixed. After fixation in the population there is reduced selection against mutations that affect the endonuclease activity. Therefore, functional forms of the HEGs are eventually reduced in frequency. Their data (Goddard & Burt, Citation1999) supported a constant cycle of invasion, degeneration, loss, and finally re-invasion, with each transition occurring roughly every 2 million years. Haugen et al. (Citation1999) came to a similar conclusion, based on reports that nuclear homing endonucleases generate double-strand breaks at intron-lacking rDNA alleles (Johansen et al., Citation1997; Elde et al., Citation1999) and are lethal when expressed in yeast (Lin & Vogt, Citation1998), and that selection occurs against functional forms of homing endonuclease genes. The-pair wise comparison between the different intra-individual intron types in IRE-2, ENG-8, NWY-7, NWY-8 (the 478-bp insertion or not) showed a higher sequence similarity (99.8–100%) in the larger 1506 introns between individuals when compared with shorter intron variants (96.2–100%). Such results suggest that the two intron size classes are diverging at different rates and should be considered different entities for phylogenetic purposes.
Non-congruence between phylogenies based on partial SSU sequences and the 1506 intron
In a previous study by Klein et al. (Citation2003), the relationships of the Northwest Atlantic Porphyra taxa were evaluated based on partial small subunit ribosomal sequences. Two clades with strong bootstrap values included one clade with P. miniata, P. leucosticta and P. suborbiculata and the other of P. dioica, P. linearis, P. purpurea and P. umbilicalis. The SSU phylogeny from Klein et al. (Citation2003) and the phylogeny based on the shorter size variant of the 1506 intron from this study () are not congruent; a partition homogeneity test between the group-I intron and SSU data sets found that they were incongruent with p = 0.01 (data not shown). This is consistent with the hypothesis that these group-I introns may still undergo occasional horizontal gene transfer. Our observations contrast with the conclusions drawn by Müller et al. (Citation2001) where the phylogenies based on SSU and intron sequence were largely congruent. They concluded that these group-I introns were largely immobile, inherited vertically, and showed subsequent loss from some lineages. We believe their conclusions were premature because (i) many of Müller et al. (Citation2001) accessions were misidentified, and (ii) they failed to detect introns in many of their specimens. Individual accessions from the same ‘morphological’ taxon are scattered across the phylogenies (cf. Müller et al. (Citation2001)). By contrast, work in our lab has demonstrated that SSU and rbcL sequences are species-specific (Teasdale et al. Citation2002; Klein et al. Citation2003). Using a combination of flanking and internal primers we detected the 1506 group-I intron at high frequencies (especially when the primers were species-specific). Our phylogenetic analyses, combined with the discovery of an intact His–Cys box in larger size variants, suggests that the group-I intron may have undergone rare horizontal transmission, resulting in incongruence between the SSU and intron phylogenies.
Conclusions
In order to obtain a true picture of the range and variation of group-I introns within the small ribosomal RNA gene in Porphyra, it is necessary to use both a species-specific intron primer and a flanking primer to detect these insertions among the heterogeneous copies of the rDNA repeat. Some copies of the larger size variant of the 1506 intron in P. umbilicalis may encode a functional homing-endonuclease. The larger size intron (with the His–Cys box) appears to be evolving independently of the smaller version of the 1506 intron.
Haugen et al. (Citation1999) proposed that the smaller size SSU introns, lacking the His–Cys box, are vertically inherited; sequence data for the shorter 1506 group-I introns from Porphyra umbilicalis are consistent with this proposal. The small SSU introns also exhibit sequence variation (96.2–100% similarity over geographically isolated accessions). Therefore, as originally proposed by Oliveira & Ragan (Citation1994), rapidly evolving group-I introns can provide a variable locus to evaluate population history within Porphyra species.
TEJP_A_359255_SUP_0001.zip
Download ()Acknowledgements
The research done in this paper was supported by the National Sea Grant College Program of the US Department of Commerce's National Oceanic and Atmospheric Administration under NOAA Grant #NA16RG1035, the Hubbard Endowment Fund of the UNH Marine Program, and the UNH Graduate School Summer Teaching Fellowships. Our thanks go to Dr John Burke and Dr Ken Hampel from the University of Vermont, who helped with the secondary structure analysis of the ribosomal group-I intron for Porphyra umbilicalis. The following individuals graciously contributed sample material used in this study: D.A. Birkett (Queens University, Ireland), T. Bray (University of New Hampshire (UNH), USA), J. Brodie (Natural History Museum, UK), E. Hehre (UNH), C. Neefus (UNH), A. Pedersen (Norwegian Institute for Water Research, Norway), R. Pereira (Centre for Marine and Environmental Research, Portugal), F. Rindisi (Alfred Wegener Institute, Germany), J. Rueness (University of Oslo, Norway), K. Sjotun (University of Bergen, Norway), and A. Wagner (Alfred Wegener Institute, Germany). The research described was submitted in partial fulfillment of the requirements for a PhD (BWT 2004) at the University of New Hampshire, USA.
References
- Belfort , M . 1991 . Self-splicing introns in prokaryotes: migrant fossils? . Cell , 64 : 9 – 11 .
- Belfort , M and Roberts , RJ . 1997 . Homing endonucleases: keeping the house in order . Nucl. Acids Res. , 25 : 3379 – 3388 .
- Bhattacharya , D . 1998 . The origin and evolution of protist group-I introns . Protist , 149 : 113 – 122 .
- Bhattacharya , D , Cannone , J and Gutell , R . 2001 . Group-I intron lateral transfer between red and brown algal ribosomal RNA . Curr. Genet. , 40 : 82 – 90 .
- Bhattacharya , D , Friedl , T and Damberger , S . 1996 . Nuclear-encoded rDNA group-I introns; origin and phylogenetic relationships of insertion site lineages in the green algae . Mol. Biol. Evol. , 13 : 978 – 989 .
- Bhattacharya , D , Surek , B , Ruesing , M , Damberger , S and Melkonian , M . 1994 . Group-I introns are inherited through common ancestry in the nuclear-encoded rRNA of Zygnematales (Charophyceae) . Proc. Natl Acad. Sci. USA , 91 : 9916 – 9920 .
- Booton , G , Floyd , G and Fuerst , P . 2004 . Multiple group-I introns detected in the nuclear small subunit rDNA of the autosporic green alga Selenastrum capricornutum . Curr. Genet. , 46 : 228 – 234 .
- Brimacombe , R , Atmadja , J , Stiege , W and Schüler , D . 1988 . A detailed model of the three-dimensional structure of Escherichia coli ribosomal RNA in situ in the 30S subunit . J. Mol. Biol. , 199 : 115 – 136 .
- Broom , JE , Nelson , WA , Yarish , C , Jones , WA , Aguilar Rosas , R and Aguilar Rosas , LE . 2002 . A reassessment of the taxonomic status of Porphyra suborbiculata, Porphyra carolinensis and Porphyra lilliputiana (Bangiales, Rhodophyta) based on molecular and morphological data . Eur. J. Phycol. , 37 : 217 – 226 .
- Cech , TR . 1990 . Self-splicing of Group-I introns . Annu. Rev. Biochem. , 59 : 543 – 568 .
- Damberger , SH and Gutell , RR . 1994 . A comparative database of group-I intron structures . Nucl. Acids Res. , 22 : 3508 – 3510 .
- Elde , M , Haugen , P , Willassen , NP and Johansen , S . 1999 . I-NjaI, a nuclear intron-encoded homing endonuclease from Naegleria, generates a pentanucleotide 3' cleavage-overhang within a 19 base-pair partially symmetric DNA recognition site . Eur. J. Biochem. , 259 : 281 – 288 .
- Farris , JS , Kallersjo , M , Kluge , AG and Bult , C . 1994 . Testing significance of incongruence . Cladistics , 10 : 315 – 319 .
- Farris , JS , Kallersjo , M , Kluge , AG and Bult , C . 1995 . Constructing a significance test for incongruence . Syst. Biol. , 44 : 570 – 572 .
- Felsenstein , J . 1985 . Confidence limits on phylogenies: an approach using the bootstrap . Evolution , 39 : 783 – 791 .
- Flick , KE , Jurica , MS , Monnat , RJJR and Stoddard , BL . 1998 . A binding and cleavage by the nuclear intron-encoded homing endonuclease I-Ppol . Nature , 394 : 96 – 101 .
- Freshwater , DW , Fredericq , S , Butler , BS , Hommersand , MH and Chase , MW . 1994 . A gene phylogeny of the red algae (Rhodophyta) based on plastid rbcL . Proc. Natl. Acad. Sci. USA , 91 : 7281 – 7285 .
- Friedl , T , Besendahl , A , Pfeiffer , P and Bhattacharya , D . 2000 . The distribution of group-I introns in lichen algae suggests that lichenization facilitates intron lateral transfer . Mol. Phylogenet Evol. , 14 : 342 – 352 .
- Gargas , A. , DePriest , P.T. and Taylor , J.W. 1995 . Positions of multiple insertions in SSU rDNA of lichen forming fungi . Mol. Biol. Evol. , 12 : 208 – 218 .
- Goddard , M and Burt , A . 1999 . Recurrent invasion and extinction of a selfish gene . Proc. Natl. Acad. Sci. , 96 : 13880 – 13885 .
- Haugen , P , Huss , V , Nielsen , H and Johansen , S . 1999 . Complex group-I introns in nuclear SSU rDNA of red and green algae: evidence of homing–endonuclease pseudogenes in the Bangiophyceae . Curr. Genet. , 36 : 345 – 353 .
- Hibbett , DS . 1996 . Phylogenetic evidence for horizontal transmission of group-I introns in the nuclear ribosomal DNA of mushroom-forming fungi . Mol. Biol. Evol. , 13 : 903 – 917 .
- Johansen , S , Elde , M , Vader , A , Haugen , P , Haugli , K and Haugli , F . 1997 . In vivo mobility of a group-I intron in nuclear ribosomal DNA of the myxomycete Didymium iridis . Mol. Microbiol. , 24 : 737 – 745 .
- Johansen , S , Embley , TM and Willassen , NP . 1993 . A family of nuclear homing endonucleases . Nucl. Acids Res. , 21 : 4405
- Klein , AS , Mathieson , AC , Neefus , CD , Cain , DF , Taylor , HA , West , AL , Hehre , EJ , Brodie , J , Yarish , C , Teasdale , BW and Wallace , AL . 2003 . Identifications of northwestern Atlantic Porphyra (Bangiaceae, Bangiales) based on sequence variation in nuclear small subunit ribosomal RNA and plastid ribulose bisphosphate carboxylase oxygenase large subunit genes . Phycologia , 42 : 109 – 122 .
- Kruger , K , Grabowski , P , Zaug , AJ , Sands , J , Gottschling , DE and Cech , TR . 1982 . Self-splicing RNA: autoexcision and autocyclization of the ribosomal RNA intervening sequence of Tetrahymena . Cell , 31 : 147 – 157 .
- Kunimoto , M , Kito , H , Kaminishi , Y , Mizukami , Y and Murase , N . 1999a . Molecular divergence of the ssu rRNA gene and internal transcribed spacer 1 in Porphyra yezoensis (Rhodophyta) . J. Appl. Phycol. , 11 : 211 – 216 .
- Kunimoto , M , Kito , H , Yamamoto , HY , Cheney , DP , Kammishi , Y and Mizukami , Y . 1999b . Discrimination of Porphyra species based on small subunit ribosomal RNA gene sequence . J. Appl. Phycol. , 11 : 203 – 209 .
- Lambowitz , AM and Belfort , M . 1993 . Introns as mobile genetic elements . Annu. Rev. Biochem. , 62 : 587 – 622 .
- Lin , J and Vogt , VM . 1998 . I-PpoI, the endonuclease encoded by the Group-I Group PpLSU3, is expressed from an RNA Polymerase I transcript . Mol. Cell Biol. , 18 : 5809 – 5817 .
- Lindstrom , SC and Fredericq , S . 2003 . rbcL gene sequences reveal relationships among north-east Pacific species of Porphyra (Bangiales, Rhodophyta) and a new species, P. aestivalis . Phycol. Res. , 51 : 211 – 224 .
- Mathews , DH , Sabina , J , Zuker , M and Turner , DH . 1999 . Expanded sequence dependence of thermodynamic parameters improves prediction of RNA secondary structure . J. Mol. Biol. , 288 : 911 – 940 .
- Mathieson , AC and Hehre , EJ . 1986 . A synopsis of New Hampshire seaweeds . Rhodora , 88 : 1 – 139 .
- Michel , F and Westhof , E . 1990 . Modeling of the three-dimensional architecture of Group-I Catalytic Groups Based on Comparative Sequence Analysis . J. Mol. Biol. , 216 : 585 – 610 .
- Milstein , D and Oliveira , MC . 2005 . Molecular phylogeny of Bangiales (Rhodophyta) based on small subunit rDNA sequencing: emphasis on Brazilian Porphyra species . Phycologia , 44 : 212 – 221 .
- Müller , KM , Cannone , JJ , Gutell , RR and Sheath , RG . 2001 . The structural and phylogenetic analysis of the group-IC1 introns in the order Bangiales (Rhodophyta) . Mol. Biol. Evol. , 18 : 1654 – 1667 .
- Müller , KM , Sheath , RG , Vis , ML , Crease , TJ and Cole , KM . 1998 . Biogeography and systematics of Bangia (Bangiales, Rhodophyta) based on the RUBISCO spacer, rbcL gene and 18S rRNA gene sequences and morphometric analyses. I. North America . Phycologia , 37 : 195 – 207 .
- Neefs , JM , Van De Peer , Y , De Rijk , P , Chapelle , S and De Wachter , R . 1993 . Compilation of small ribosomal subunit RNA structures . Nucl. Acids Res. , 21 : 3025 – 3049 .
- Oliveira , MC , Kurniawan , J , Bird , CJ , Rice , EL , Murphy , CA , Singh , RK , Gutell , RR and Ragan , MA . 1995 . A preliminary investigation of the order Bangiales (Bangiophycidae, Rhodophyta) based on the sequences of nuclear small-subunit ribosomal RNA genes . Phycol. Res. , 43 : 71 – 79 .
- Oliveira , MC and Ragan , MA . 1994 . Variant forms of a group-I intron in nuclear small-subunit rRNA genes of the marine red alga Porphyra spiralis var. amplifolia . Mol. Biol. Evol. , 11 : 195 – 207 .
- Palumbi , S and Baker , CS . 1994 . Contrasting population structure from nuclear intron sequences and mtDNA of Humpback Whales . Mol. Biol. Evol. , 11 : 426 – 435 .
- Ragan , MA , Bird , CJ , Rice , EL , Gutell , RR , Murphy , CA and Sing , RK . 1994 . A molecular phylogeny of the marine red algae (Rhodophyta) based on the nuclear small-subunit rRNA gene . Proc. Natl Acad. Sci., USA , 91 : 7276 – 7280 .
- Ragan , MA , Bird , CJ , Rice , EL and Singh , RK . 1993 . The nuclear 18S ribosomal RNA gene of the red alga Hildenbrandia rubra contains a group-I intron . Nucl. Acids Res. , 21 : 3898
- Sefc , KM , Payne , RB and Sorenson , MD . 2003 . Microsatellite amplification from museum feather samples: effects of fragment size and template concentration on genotyping errors . Auk , 120 : 982 – 989 .
- Slade , RW , Moritz , C , Heideman , A and Hale , PT . 1993 . Rapid assessment of single copy nuclear DNA variation in diverse species . Mol. Ecol. , 2 : 359 – 373 .
- Soltis , PS and Soltis , DE . 1998 . “ Molecular evolution of 18S rDNA in angiosperms: implications for character weighting in phylogenetic analysis ” . In Molecular Systematics of Plants II: DNA Sequencing , Edited by: Soltis , DE , Soltis , PS and Doyle , JJ . 188 – 210 . Boston, , USA : Kluwer .
- Stiller , JW and Waaland , JR . 1993 . Molecular analysis reveals cryptic diversity in Porphyra (Rhodophyta) . J. Phycol. , 29 : 506 – 517 .
- Suh , SO , Jones , KG and Blackwell , M . 1999 . A group-I intron in the nuclear small subunit rRNA gene of Cryptendoxyla hypophloia, an ascomycetous fungus: evidence for a new major class of group-I introns . J. Mol. Evol. , 48 : 493 – 500 .
- Swofford , DL . 2002 . PAUP*: Phylogenetic Analysis Using Parsimony (and other Methods), Version 4.0b10. , Sunderland, , USA : Sinauer Associates .
- Teasdale , B , West , A , Taylor , H and Klein , A . 2002 . A simple restriction fragment length polymorphism (RFLP) assay to discriminate common Porphyra (Bangiophyceae, Rhodophyta) taxa from the Northwest Atlantic . J. Appl. Phycol. , 14 : 293 – 298 .
- Wattier , R , Engel , CR , Saumitou-Laprade , P and Valero , M . 1998 . Short allele dominance as a source of heterozygote deficiency at microsatellite loci: experimental evidence at dinuclotide locus Gv1CT in Gracilaria gracilis (Rhodophyta) . Mol. Ecol. , 7 : 1569 – 1573 .
- Wheeler , WC and Honeycutt , RL . 1988 . Paired sequence difference in ribosomal RNAs: evolutionary and phylogenetic implications . Mol. Biol. Evol. , 5 : 90 – 96 .
- Yamada , T , Tamura , K , Aimi , T and Songsri , P . 1994 . Self-splicing group-I introns in eukaryotic viruses . Nucl. Acids Res. , 22 : 2532 – 2537 .