Abstract
Planar optodes and microelectrodes have been used to locate photosynthetic activity in calcified Rivularia biasolettiana and R. haematites. Maximum photosynthesis, measured as oxygen concentration and pH, occurred in the upper 1–3 mm of the colony, coinciding with a region of calcification. Stoichiometric calculations indicate that photosynthesis could account for almost all of the calcification within this region but further calcification was identified deeper within the colonies where photosynthesis was negligible. Overall, it was estimated that a maximum of 14% of the calcium carbonate within the colonies could have been formed as a direct result of photosynthesis.
Introduction
Stromatolites are remarkable organically derived sediments formed largely through the agencies of cyanobacteria. Structures identified as stromatolites feature among Earth's earliest fossils (Riding, Citation1999) and persist today, though with a restricted distribution. Although most stromatolites appear to be marine in origin, freshwater representatives are also known and date back as far as the Precambrian (Bolhar et al., Citation2007; Handley et al., Citation2008). Modern stromatolites have proved difficult to investigate. They frequently grow in remote areas such as Shark Bay, W. Australia and the caldera lakes of the Pacific islands (Kazmierczak & Kempe, Citation2006), and the associated cyanobacteria are difficult to grow axenically. Experimental work can be confounded by their heavy mineralization, making high-resolution studies of their internal structure difficult or impossible.
Although stromatolites are best regarded as microbial communities rather than individual stromatolite-building species, in many cases a small number of organisms are responsible for most of their biomass. A few of these freshwater and marine stromatolite-forming species belonging to the genera Phormidium and Rivularia have proved to be amenable to experimentation. While none has been brought into pure culture, samples can be returned to the laboratory or examined in the field undamaged and subject to repeated experimentation. Previous work with the calcite-encrusted stromatolitic cyanobacterium Rivularia haematites (de Candolle) Bornet et Flahault has shown that the calcite crystals grow parallel to the trichomes and nucleate on the fibrous outer layer of the mucilaginous sheath (Caudwell et al., Citation2001) and are subject to bacterial biomicritization (Caudwell, Citation1987). Growth is seasonal, and individual colonies may attain an age of 10 yr or more, with zoned patterns of calcification (Pentecost, Citation1987; Pentecost & Spiro, Citation1990). Field and laboratory studies have shown that calcification is the result of photosynthesis and evaporation during the warmer seasons, while it is thought to be entirely abiogenic during winter as a result of CO2 evasion (Pentecost, Citation1987; Pentecost & Whitton, Citation2000). In the case of Rivularia, water levels are sufficiently low that during dry periods, the colonies became exposed to the air and evaporation of the water within and upon the colonies leads to some calcium carbonate precipitation. In other cyanobacteria, photosynthesis has also been found to be responsible for calcification in freshwater (Shiraishi et al., Citation2008), hypersaline (Ludwig et al., Citation2005) and marine settings (Visscher et al., Citation1998), while sulphate reduction (Dupraz & Visscher, Citation2005), ammonification and denitrification (Krumbein, Citation1979) are potential mechanisms for calcification in stromatolites. The exopolymer layer consisting of extracellular polymeric substances (EPS) is especially well developed in Rivularia (Sabater, Citation2000) where it may act as both a nucleation site (Shiraishi et al., Citation2008) or a trapping and binding medium for calcite crystals (Pentecost & Riding, Citation1986). The ease with which these colonies may be collected and maintained makes them prime experimental organisms and they are frequently recognized in the fossil record. While their rate of growth, mineralization, and their response to environmental factors (Whitton, Citation1987) are gradually becoming understood, studies of stromatolite ecophysiology lag behind work on non-mineralized mats. In the latter, much use has been made of microelectrodes to determine flux rates of major metabolites. These devices can probe soft mats and reveal important information about rates of photosynthesis, respiration, sulphide metabolism and major element cycling (Revsbech, Citation1994; Jonkers et al., Citation2003). In highly mineralized stromatolites, microelectrode probing is not always possible owing to the resistance to penetration and resultant damage to the sensors, although electrodes enclosed within steel needles have met with success in some of the less heavily mineralized forms (Visscher et al., Citation1998). While the surface of these stromatolites has been briefly investigated (Merz-Preiss, Citation2000), less is known of the chemical environment within them, yet this is of importance in understanding the mineralizing process. In 2003 the senior author discovered weakly cemented stromatolites of Rivularia in a calcareous hill stream in northern England, into which microelectrodes can be inserted without damage, and a planar optode was used to provide two-dimensional information on photosynthesis within the more heavily mineralized Rivularia colonies for the first time.
Materials and methods
Sampling
Mature Rivularia biasolettiana (Meneghini) Bornet et Flahault and R. haematites microstromatolites 2–7 cm in diameter were picked respectively from two karst streams in the Yorkshire Dales, UK: Gordale (Nat. grid. ref. 34/913644, alt. 330 m) and Kettlewell (34/974736, alt. 270 m). The species were identified according to Whitton (Citation2002) who found them to be widespread in the streams of this region. The colonies consisted of almost pure growths of Rivularia with less than 1% contamination by other cyanobacteria (mainly Lyngbya sp.). Samples were transported and maintained in cool stream water in transparent plastic containers with the water replaced every 24 h, and kept illuminated at room temperature under a 12 h daily photoperiod. While the R. haematites samples were heavily calcified throughout, a small number of poorly calcified R. biasolettiana were chosen for comparison and further characterization.
Biovolume estimates
Rivularia colonies consist of a large number of narrow (1–5 µm) radiating or parallel trichomes embedded in mucilage (). Cellular biovolume of the trichomes was determined since the bulk of the colonies appeared to consist of extracellular polymeric substances (EPS). To estimate cellular biovolume, colonies were divided into blocks measuring approximately 5 mm × 5 mm × 1 mm under a dissection microscope (). These were frozen (−20°C) then 20–40 µm thick slices were cut transversely with a razor and examined under a light microscope fitted with a calibrated graticule. For each 1 mm slice, 20 random fields were scanned to provide a total of c. 400 trichome counts with a precision of ±10%. Mean trichome width was determined from 50 random measurements on the same colonies under a magnification of ×750 with a light microscope with an estimated precision of ±5%. These measurements allowed the trichome biovolume to be recorded in 1 mm slices throughout the colony as the percentage area of colony occupied by trichomes in a plane perpendicular to the direction of growth.
Fig. 1. Features of Rivularia colonies. (a) Diagram of a vertical section through a mature Rivularia colony showing orientation of the trichomes and two bands of calcification. (b) Sampling block for biovolume and CaCO3 content. (c) Part of the Rivularia colony showing trichomes enclosed by a branched mucilaginous sheath. (d) Vertical view of a Rivularia colony showing trichomes (filled circles) surrounded by firm mucilaginous sheaths (stippled) enclosed in highly hydrated and diffluent mucilage in which calcification occurs (white).
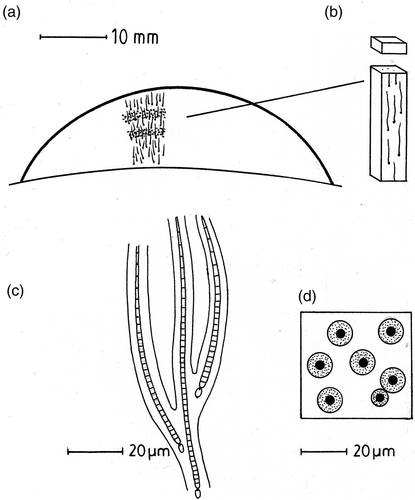
Calcium carbonate content and water chemistry
Another series of small frozen blocks was cut with a razor saw and homogenized with 5 ml water in a hand-held glass homogenizer. The suspension was mixed with 10 ml 0.1 N HCl for 3 h at room temperature, then centrifuged and the pellet resuspended and washed in a further two changes of water. The clear solution was neutralized with dilute NaOH and calcium determined titrimetrically with EDTA using the indicator Eriochrome Blue Black R (Greenberg et al., Citation1985). The calcium content was converted into vol % calcium carbonate as calcite in the sample at 1 mm depth intervals. Water samples were taken from the collecting sites and analysed within 24 h for major cations, anions and pH. Standard methods were used as given in Pentecost (Citation1992). The calcite saturation index, Ω, was determined with an iterative computer program (Pentecost, Citation1992): Ω = (Ca2+)()s/(Ca2+)(
)c where s refers to the sample and c the ion activity product for calcite at the measured temperature and pressure. When Ω = 1 the water sample is at equilibrium, i.e. saturated with respect to calcite, and when Ω > 1 the sample is supersaturated with respect to calcite (Howard et al., Citation1984).
Light attenuation in Rivularia microstromatolites
Colonies were cored with a 5 mm diameter metal cork-borer and the uppermost 0.5 mm of the core extruded then frozen at –20°C. The extruded end was sliced off with a razor saw, gently homogenized as above, and the suspension loaded into a chamber seated upon a Licor 185B Quantum Meter. The suspension was allowed to settle and a strong light source illuminated the suspension from above. The transmittance of light was then measured with the sensor. This was repeated down the core so that transmission values were obtained both for individual 0.5 mm sections, and cumulatively.
Planar oxygen optode
The heavily calcified R. haematites colonies were too dense to permit the safe penetration of glass microelectrodes, so a porphyrin-based optically isolated planar oxygen optode was used to measure photosynthesis rates within whole colonies.
The optodes used consisted of three layers: a transparent polyester support foil (125 µm thick, Goodfellow), a sensing layer and an optical insulation. Details of the sensing layer are described elsewhere (Franke et al., Citation2006). An additional layer of black silicone was added on top of the sensing matrix for optical insulation. The thicknesses of the dry sensing layer and the black silicone layer were ∼15–30 µm and ∼20–40 µm, respectively. Single colonies were carefully sliced vertically with a razor saw and one half of the colony placed with its vertical sectioned surface in contact with the planar optode. The planar optodes were glued with transparent silicone (Elastosil® E4, Wacker) onto the inside of a transparent wall of a perspex cell through which water, containing 2 mmol l−1 bicarbonate at pH 8 (to simulate stream conditions), was circulated at a controlled temperature (15°C) and oxygen concentration. The colony was sealed into the cell using a solution of warm 2% agar buffered at pH 8.0 with 2 mmol l−1 NaHCO3 so that the surface of the colony was in direct contact with oxygen-saturated water. Once the agar had set, the cell was gently pressed against a modular luminescence lifetime imaging system or MOLLI and water circulated through the Rivularia cell to provide continuous oxygen saturation. Oxygen imaging was facilitated by the modular luminescence lifetime imaging (MOLLI) system (Holst et al., Citation1998; Holst & Grunwald, Citation2001). The optodes were excited by blue LEDs (HLMP-CB 15, λmax = 475 nm, Agilent). The red luminescence light (λmax = 647 nm) emitted by the optode passed through a red optical filter (Deep Golden Amber, LEE-Filters) before being detected by a fast gateable CCD Camera (SensiCam, PCO, image size 640 × 480 pixels).
The rapid lifetime determination (RLD) method (Ballew & Demas, Citation1989; Liebsch et al., Citation2000) was employed to measure the luminescence lifetime distributions. Lifetime values were converted into oxygen concentrations using a modified two-component model of the Stern–Volmer equation (Klimant et al., Citation1995; Holst et al., Citation1998). Further image processing was carried out with a computer program developed in IDL® (Holst & Grunwald, Citation2001). Calibration was carried out before and after the experiments, whereby the lifetime values corresponding to 0 and 100% air saturation (AS) were taken as an average of many pixels (n > 100) from the areas of the planar optode which were known to be anoxic (near the base of the Rivularia colony shown to be anoxic using a microelectrode) and air saturated (bubbled with air for 1 h), respectively. Resolution of the oxygen images (640 × 480 pixels) was 25 × 25 µm per pixel. This resolution should not be compromised by the distance between the Rivularia and optode surface since they were in close contact. Output was provided as a colour-coded two-dimensional oxygen image as well as one-dimensional vertical or horizontal oxygen profiles with a precision of about 5% oxygen saturation. Measurements were undertaken at a Rivularia surface irradiance of 500 µmol m−2 s−1. The Rivularia was allowed to equilibrate with the water circulation system for 5 h at the above irradiance prior to measurement.
Microelectrodes
Oxygen microelectrodes with an external diameter of 10 µm were fabricated at the Max Plank Institute, Bremen. They were mounted on a micromanipulator, attached to a suitable picoammeter, and calibrated with water of known oxygen concentration as recommended by Gieseke & De Beer (Citation2004). The electrodes were inserted into water-saturated colonies of poorly calcified Rivularia biasolettiana and measurements taken every 50 µm throughout the depth of the colony. Measurements were made in triplicate at each level under an irradiance of 500 µmol m−2 s−1 provided by a fibre-optic light source. Equilibration conditions were the same as described above. pH microelectrodes were obtained from Unisense Ltd, Aarhus, Denmark and calibrated at pH 8.0 and 10.0 using standard National Bureau of Standards buffers. They were mounted and equilibrated as described above and measurements taken at 100 µm depth intervals within the Rivularia colony.
Results
Water chemistry
The water chemical data are listed in . At both of the sampling sites, the major dissolved constituents were Ca2+ and . The waters were mildly alkaline with pH > 8. Dissolved sulphate and nitrate concentrations were low. The calcite saturation index Ω exceeded unity at both sites indicating supersaturation of these waters with respect to calcite.
Table 1. Water chemical composition at the two sampling sites. Measurements, excluding pH and temperature, in mmol l−1 unless otherwise stated.
Rivularia biovolume and calcium carbonate content
In , the numbers of trichomes per mm2 of colony of surface are plotted against depth. Large differences are apparent between R. biasolettiana and R. haematites, with the latter having almost four times as many trichomes per unit area as the former. In both types of colony, however, the form of the depth-distribution is similar. Numbers at the colony surface are significantly lower than those encountered at depths of 1–3 mm. In R. biasolettiana, maximum numbers were recorded at a depth of 2 mm after which they declined to low numbers at 5 mm depth. In R. haematites, maximum numbers were recorded at 6 mm depth, after which they declined irregularly to 10 mm. Intact trichomes were encountered throughout the colonies but their density varied with depth. A different picture emerges for the estimated cellular biovolumes. These were determined as the percentage area of a transverse section of colony occupied by the trichomes (). Here it is apparent that the maximum biovolumes are similar for both species at around 2.5% reflecting large differences in the trichome diameters of the two taxa. Rivularia biasolettiana had a mean trichome diameter of almost 4 µm, compared with 7 µm for R. haematites. For both species, the remaining space (≥97.5%) was occupied by EPS, crystals of calcite and aqueous voids.
Fig. 2. Trichome density in Rivularia colonies. (a) Number of trichomes per mm2 of surface vs depth within colony (mm). (b) Trichome relative biovolume vs depth. Filled circles, R. haematites colonies, open circles, R. biasolettiana.
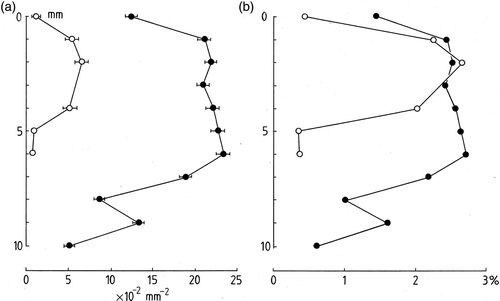
In both species the calcium carbonate content ranged from 10–35 vol%, with an overall mean of 21 vol%, equivalent to 42% by weight of the hydrated colony (). The degree of mineralization increased with depth in the colonies, but the deepest layers were too lax to allow accurate sampling and measurements. Microscopic examination also suggested that the uppermost 0.5 mm of both colony types was weakly mineralized. The calcite crystals were distributed in bands and this probably accounts for much of the variation in CaCO3 content with depth, but the banding was usually on a sub-millimetre scale so individual bands were not resolved using the above technique.
Light penetration
Log-linear plots of irradiance vs colony depth are shown in . Both species gave similar slopes and R. biasolettiana gave a good exponential trend below a depth of 2 mm. However, the two taxa showed signs of deviation from the Bouguer–Lambert Law of light attenuation near the surface since the best fit lines do not pass through the origin. This results from the high transparency of the upper 0.25 mm of the colonies due to the lower biovolume of the trichomes and weaker calcification in this region. Extinction values were estimated from the relevant regression equations (P < 0.001) as 3.06 and 3.13 mm−1 for R. biasolettiana and R. haematites respectively in the log-linear regions. Estimates of irradiance at the level of maximum photosynthesis were made by application of the above coefficients, ignoring the upper 0.25 mm of the colony which was almost transparent.
Optode and microelectrode data
Studies of R. haematites sections using a planar optode demonstrated photosynthesis as O2 concentration in the upper 2.5 mm of the colony. Although oxygen concentration varied vertically () little lateral variation was found. Photosynthetic activity was recorded in the surface layer of the mat with an incident irradiance of 500 µmol m−2 s−1 in both the hard and soft colonies, and the depth of the photosynthesizing layer was similar in both taxa. When the upper 5 mm of mat was removed with a scalpel, and illumination resumed, no photosynthesis was detected in the colonies suggesting that cells below this depth were moribund.
Fig. 5. Dissolved oxygen concentration in a R. haematites colony. Porphyrin planar optode at a surface irradiance of 500 µmol m−2 s−1. The thin black line indicates the colony surface. Silhouette of colony section shown below.
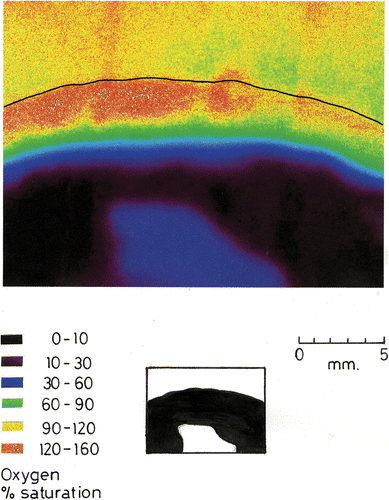
Oxygen penetrated the lower region of the colonies by diffusion (, ). At a depth of 5 mm, concentrations were about 10–30% saturation. Deeper still, levels dropped to zero. The deep blue region at bottom centre of consists of the agar support that contains a low concentration of oxygen depleted by the respiring Rivularia, probably with associated heterotrophic microbes. In R. haematites, maximum photosynthesis, corresponding to oxygen saturations of 160–180%, occurred at a depth of 1.5–3 mm with estimated irradiance levels between 2% and 0.02% of the surface values.
Fig. 6. Oxygen profile in a R. biasolettiana colony. Mean of three replicate runs showing standard errors at 1 mm intervals. Ordinate gives distance from colony surface in mm. For oxygen, only alternate datapoints are shown on this figure; the original resolution was 50 µm. Filled circles: microelectrode % oxygen. Open circles: pH at a resolution of 100 µm. Irradiance 500 µmol m−2 s−1 for both measurements.
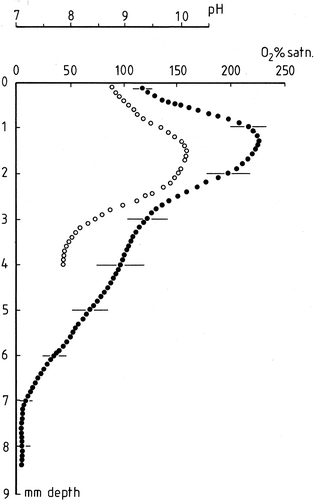
In R. biasolettiana, a microelectrode was used to measure oxygen production (). In this colony, maximum photosynthesis corresponded to a depth of 1.4 mm where the diminution of light intensity was estimated to correspond to the same range as that found for R. haematites.
The pH profile shown in demonstrates a pH rise associated with photosynthesis. At the surface of the colony the pH is close to 8.5 but rises rapidly with depth to a maximum of 10.2 at a depth of 1.5 mm. Thereafter, pH declines to a value of 7.9 at a depth of 4.0 mm below the surface.
Discussion
Optodes were originally designed for medical applications (Rumsey et al., Citation1988) but soon found use in microbial ecology (Glud et al., Citation1996; Wenzhöfer & Glud, Citation2004). Our study has demonstrated the potential for their use in understanding the distribution and concentration of major metabolites within heavily mineralized cyanobacterial colonies. Sectioning of colonies with a razor saw probably introduced some minor irregularities in the Rivularia surface which might alter the diffusion path of oxygen to the optode. However, we do not consider this to be significant since the colonies were sufficiently elastic that the slight pressure used to attach the colonies to the optode would have ensured that the entire surface came into contact with the optode.
Previous studies of Rivularia calcification have demonstrated that both organic (photosynthetic) and inorganic processes occur within the colonies. In UK populations, Pentecost (Citation1987) found that an abiogenic crust of calcite formed at the surface of the colonies during winter when growth was shown to be negligible. During the warmer seasons however, narrow bands of calcification were attributed to a combination of low-water events permitting surface evaporation, and photosynthesis within the colonies. The latter has received additional support from other observations. Casanova (Citation1986) found zoned crystals of calcite within Rivularia, attributed to daily variations of mineralization directly linked to photosynthesis–respiration cycles although these have not been reported in other studies (e.g. Pentecost, Citation1987; Obenlüneschloss & Schneider, Citation1991).
Measurements of the stable carbon isotope ratios of calcite deposited in summer and winter growth layers also demonstrated that a relationship with photosynthetic activity was likely (Pentecost & Spiro, Citation1990). This present study has shown that photosynthesis in mineralizing colonies occurs within layers in which calcification is actively taking place and provides support for its role in mineralization. It also provides evidence for further abiogenic or possibly non-photosynthetic biomineralization at depth. An estimate of the organic carbon content of the Rivularia colonies can be obtained from the dry mass: wet mass ratio of the decalcified colonies. This ratio was found to be 1 : 60 so that 1 mm3 of colony had an average dry mass of 17 µg. The carbon content of the dry mass is assumed to be 43% (Jorgensen, Citation1979) so that a cubic millimetre of fully hydrated Rivularia contains about 7 µg organic carbon.
A stoichiometric precipitation of calcium carbonate based on the photosynthesis equation (Equation1):
The rate of heterogeneous calcite nucleation is positively correlated to the calcite supersaturation (Reddy, Citation1995) so that any process leading to increased supersaturation should increase the nucleation rate. Water pH rise increases the supersaturation by raising the carbonate ion activity, so a pH rise in the zone of maximum photosynthesis of Rivularia is bound to increase the nucleation rate of calcite (Borowitzka, Citation1989). This increased nucleation rate could also result in increased calcite precipitation, but both nucleation and crystal growth can be reduced or even prevented by inhibitors. Nucleation inhibitors have been detected in a wide range of algae and cyanobacteria, including Rivularia where they may act to prevent precipitation at undesirable locations on the cell surface (Pentecost, Citation1974, Citation2004; Kawaguchi & Decho, Citation2001). In the case of cyanobacteria, inhibitors might prevent direct calcification of the cell wall, since nucleation seems to be confined to the EPS in the species so far investigated (Pentecost & Bauld, Citation1988). Crystal growth, and therefore nucleation, is most apparent in the outer layers of the Rivularia sheath, and photosynthesis probably plays an important role in the creation of nucleation sites for calcite in cyanobacteria. The maintenance of a moderately high pH below the zone of maximum photosynthesis may also explain why further calcification may occur deeper within the colony. In an earlier investigation using 14CO2 uptake into Rivularia, the amount of carbonate precipitated stoichioimetrically was estimated as around 5% of that actually observed (Pentecost, Citation1974). This lower estimate may have resulted from the loss of some hydrolysed EPS during the acid-washing procedure employed. With an average calcium carbonate content of 42% by weight in the colonies investigated here, and with 6% of the total colony mass consisting of photosynthetic calcite, about 14% of the total carbonate would appear to be the result of photosynthesis, more than previously estimated using the 14C method.
Calcium carbonate is only precipitated providing sufficient Ca is present and the mineral (calcite) solubility product is exceeded. In the stream waters considered here, Ca is the major dissolved cation and is approximately stoichiometric with as shown in . The waters overlying these colonies have been shown on several occasions to be already supersaturated with respect to calcite via inorganic degassing, particularly during summer with higher water temperatures (Spiro & Pentecost, Citation1991; Pentecost, Citation1992). This year-round supersaturation probably accounts for the ‘abiogenic’ layers of calcite laid down in winter as well as some of the calcite deposited at other times of the year. Water-suspended calcite, known to occur in similar nearby streams (Pentecost, Citation1990), could also be trapped by the Rivularia mucilage and may also contribute. Maximum CaCO3 contents were found at depths well exceeding the photosynthesizing layer, where respiration predominated. This is surprising given that respiration will lead to CO2 evolution, reversing (1) and removing CaCO3 and suggests that inorganic processes, particularly the diffusion of calcite-supersaturated water, could predominate over both photosynthesis and respiration. For inorganic precipitation to occur in the Rivularia colonies, calcium and bicarbonate ions must diffuse through the overlying biomass while the water must remain supersaturated with calcite. It is evident, from the pH profile, that high supersaturations are likely in the region of maximum photosynthesis (cf. Merz-Preiss, Citation2000) and that supersaturation is probably maintained, at least to depths of about 6 mm in the colony. Although the diffusion rate of these ions has not been measured in Rivularia it is not likely to differ greatly from that in water, providing the calcification in the upper region is low. In the Yorkshire Dales where water temperatures are usually in the range of 5–15°C, low respiration rates are to be expected and these will help maintain calcite supersaturation within the colonies. However, the anoxic environment deep within the Rivularia colonies could also promote sulphate-reduction and denitrification leading to carbonate precipitation (Krumbein, Citation1979; Visscher et al., Citation1998; Dupraz & Visscher, Citation2005) although the concentration of sulphate at our sites was ten times lower than that of seawater () and the characteristic odour of hydrogen sulphide was not evident. Nitrate concentrations were also low and unlikely to lead to much mineralization. Finally, it is possible that our samples are atypical in their increased calcification with depth and this aspect of Rivularia calcification requires further investigation.
Photosynthesis rates of Rivularia colonies have been studied in situ using the 14CO2 method (Livingstone et al., Citation1984). Their diel study suggested that light saturation for Rivularia was only attained at high incident intensities (∼1500 µmol m−2 s−1). Since cyanobacteria are normally intolerant of intense light, this again suggests that maximum photosynthetic activity is occurring at depth in the colony where irradiance is lower.
The estimated levels of irradiance in the zone of maximum photosynthesis are low and they may be underestimates. The method of obtaining the extinction values was based on the assumption that the absorption of light did not vary with trichome orientation. This may not be the case, and the radially arranged trichomes within the colonies could have acted as wave-guides leading to increased light transmission at depth. The two species of Rivularia investigated can be distinguished by their form of calcification and their trichome diameters. However, some populations of R. biasolettiana are more intensely calcified than those investigated here and field observations suggest that a continuity of forms may exist, even within the same stream. Recent work by Berrendero et al. (Citation2008) employing molecular fingerprinting also indicates a high morphological and genetic diversity within the genus as currently recognized.
Conclusions
(1) Hemispherical colonies of two freshwater Rivularia species consisted of an average of 2.5% trichome by volume with the remaining 97.5% consisting of EPS, calcium carbonate deposits and voids. (2) Optode and microelectrode measurements of photosynthesis and pH in sections of Rivularia colonies showed a well-defined region of maximum photosynthesis, 1.4–3 mm below the colony surface. (3) Measurements of light attenuation in the colonies indicated that maximum photosynthesis rates occurred at irradiance levels ranging from 0.02–2% of the surface irradiance value of 500 µmol m−2 s−1.
(4) The calcium carbonate content by volume of the colonies ranged from 10–35%. About 14% of this was estimated to result from photosynthetic CO2 uptake and the remainder from other biogenic and abiogenic processes. (5) The two species of Rivularia differed in their trichome diameter and density of calcification but it was concluded that a continuum of colony form probably occurs between these taxa. (6) Further studies on the calcification processes are required in the lower non-photosynthesizing regions of Rivularia microstromatolites.
Acknowledgements
We are grateful to The Central Research Fund, University of London for the provision of a research grant to the senior author and to the German Federal Ministry of Education and Research (BMBF, project number 03F0284A), and the Max Planck Society (MPG). Ingo Klimant (Institute for Analytical Chemistry, Micro- and Radiochemistry, Technical University of Graz, Austria) and Claudia Schröder (Institute for Analytical Chemistry, Bio- and Chemo-Sensors, University of Regensburg, Germany) are thanked for the preparation of the planar oxygen optodes, and Gaby Eickert, Ines Schröder and Ingrid Dohrmann for the preparation of microsensors.
References
- Ballew , RM and Demas , JW . 1989 . An error analysis of the rapid lifetime determination method for the evaluation of single exponential decays . Analyt. Chem. , 61 : 30 – 33 .
- Berrendero , E , Perona , E and Mateo , P . 2008 . Genetic and morphological characterization of Rivularia and Calothrix (Nostocales, Cyanobacteria) from running water . Int. J. Evol. Microbiol. , 58 : 447 – 460 .
- Bolhar , R and Van Kranedonk , MJ . 2007 . A non-marine depositional setting for the northern Fortescue Group, Pilbara Craton, inferred from trace element geochemistry of stromatolitic carbonates . Precambrian Res. , 155 : 229 – 250 .
- Borowitzka , MA . 1989 . “ Carbonate calcification in algae – initiation and control ” . In Biomineralization: Chemical and Biochemical Perspectives , Edited by: Mann , S , Webb , J and Williams , RJP . 63 – 94 . Weinheim : VCH Verlag .
- Casanova, J. (1986). Les stromatolites continentaux: paleoecologie, paleohydrologie, paleoclimatologie. Application au rift Gregory. PhD Thesis. Université d’Aix Marseille.
- Caudwell , C . 1987 . Étude experimentale de la formation de micrite et de sparite dans les stromatolites d’eau douce à Rivularia . Bull. Soc. Geol. Fr. , 8 : 299 – 306 .
- Caudwell , C , Lang , J and Pascal , A . 2001 . Lamination of swampy-rivulets Rivularia haematites stromatolites in a temperate climate . Sediment. Geol. , 143 : 125 – 147 .
- Dupraz , C and Visscher , PT . 2005 . Microbial lithification in marine stromatolites and hypersaline mats . Trends Microbiol. , 13 : 429 – 435 .
- Franke , U , Polerecky , L , Precht , E and Huettel , M . 2006 . Wave tank study of particulate organic matter degradation in permeable sediments . Limnol. Oceanogr. , 51 : 1084 – 1096 .
- Gieseke , A and De beer , D . 2004 . “ Use of microelectrodes to measure in situ microbial activities in biofilms, sediments, and microbial mats ” . In Molecular Microbial Ecology Manual,, , 2nd , Edited by: Akkermans , ADL and van Elsas , K . 1581 – 1612 . Dordrecht : Kluwer .
- Glud , RN , Ramsing , NB , Gundersen , JK and Klimant , I . 1996 . Planar optodes: a new tool for fine scale measurements of two-dimensional O2 distribution in benthic communities . Mar. Ecol. Progr. Ser. , 140 : 217 – 226 .
- Greenberg, A.E., Trussell, R.R. & Clesceri, L.S. (1985). Standard Methods of the Examination of Water and Wastewater. American Public Health Association, Washington DC.
- Handley , KM , Turner , SJ , Campbell , KA and Mountain , BW . 2008 . Silicifying biofilm exopolymers on a hot-spring microstromatolite: templating nanometer-thick laminae . Astrobiology , 8 : 747 – 770 .
- Holst , G and Grunwald , B . 2001 . Luminescence lifetime imaging with transparent oxygen optodes . Sensors and Activators B-Chemical , 74 : 78 – 90 .
- Holst , G , Kohls , O , Klimant , I , Konig , B , Kühl , M and Richter , T . 1998 . A modular luminescence lifetime imaging system for mapping oxygen distribution in biological samples . Sensors and Actuators B-Chemical , 51 : 163 – 170 .
- Howard , JR , Skirrow , G and House , WA . 1984 . Major ion and carbonate system chemistry of a navigable freshwater canal . Freshwat. Biol. , 14 : 515 – 532 .
- Jonkers , HK , Ludwig , R , De Wit , R , Pringault , GM , Muyzer , G , Niemann , H , Finke , N and De beer , D . 2003 . Structural and functional analysis of a microbial mat ecosystem from a unique permanent hypersaline inland lake: ‘La Salada de Chiprana’ (NE Spain) . FEMS Microbiol. Ecol. , 44 : 175 – 189 .
- Jorgensen, S.E. (1979). Handbook of Environmental Data and Ecological Parameters. International Society for Ecological Modelling, Oxford, New York.
- Kawaguchi , T and Decho , AW . 2001 . Potential role of extracellular polymeric secretions (EPS) in regulating calcification. A study of marine stromatolites, Bahamas . Thalassas , 17 : 11 – 19 .
- Kazmierczak , J and Kempe , S . 2006 . Genuine modern analogues of Precambrian stromatolites from caldera lakes of Niuafa’ou Island, Tonga . Naturwissenschaften , 93 : 119 – 126 .
- Klimant , L , Meyer , V and Kühl , M . 1995 . Fiber-optic oxygen microsensors, a new tool in aquatic biology . Limnol. Oceanogr. , 40 : 1159 – 1161 .
- Krumbein , W . 1979 . “ Calcification by bacteria and algae ” . In Biogeochemical Cycling of Mineral-forming Elements , Edited by: Trudinger , PA and Swaine , PA . 47 – 68 . New York : Elsevier .
- Liebsch , G , Klimant , I , Frank , B , Holst , G and Wolfbeis , OS . 2000 . Luminescence lifetime imaging of oxygen, pH, and carbon dioxide distribution using optical sensors . Appl. Spectrosc. , 54 : 548 – 559 .
- Livingstone , D , Pentecost , A and Whitton , BA . 1984 . Diel variations in nitrogen and carbon dioxide fixation by the blue-green alga Rivularia in an upland stream . Phycologia , 23 : 125 – 133 .
- Lübbers , DW . 1995 . Optical sensors for clinical monitoring . Acta Anaesthesiol. Scand. , 39 : 37 – 54 .
- Ludwig , R , Al-horani , FA , De beer , D and Jonkers , HM . 2005 . Photosynthesis-controlled calcification in a hypersaline microbial mat . Limnol. Oceanogr. , 50 : 1836 – 1854 .
- Merz-Preiss , M . 2000 . “ Calcification in cyanobacteria ” . In Microbial Sediments , Edited by: Riding , RE and Awramik , SM . 50 – 56 . Berlin : Springer .
- Obenlüneschloss , J and Schneider , J . 1991 . Ecology and calcification patterns in Rivularia (Cyanobacteria) . Arch. Hydrobiol. Suppl. Bd. , 92 : 489 – 582 .
- Pentecost, A. (1974). Calcium carbonate deposition by blue-green algae. Unpublished PhD thesis, University College of North Wales, Bangor.
- Pentecost , A . 1978 . Blue-green algae and freshwater carbonate deposits . Proc. R. Soc. Lond. B. , 200 : 43 – 61 .
- Pentecost , A . 1987 . Growth and calcification of the freshwater cyanobacterium Rivularia haematites . Proc. R. Soc. Lond. B. , 232 : 125 – 136 .
- Pentecost , A . 1990 . The identity of suspended particles in a calcite-depositing stream and their signficance in trapping and binding phenomena . Limnologica , 21 : 251 – 255 .
- Pentecost , A . 1992 . Carbonate chemistry of surface waters in a temperate karst region: the southern Yorkshire Dales, U.K . J. Hydrol. , 139 : 211 – 232 .
- Pentecost , A . 2004 . Significance of marine algal calcification inhibitors in the global carbon cycle . Thalassas , 20 : 45 – 49 .
- Pentecost , A and Bauld , J . 1988 . Nucleation of calcite on the sheaths of cyanobacteria using a simple diffusion cell . Geomicrobiol. J. , 6 : 129 – 135 .
- Pentecost, A. & Riding, R. (1986). Calcification in Cyanobacteria. In Biomineralisation of Lower Plants and Animals (Leadbeater, B.S.C. & Riding, R., editors), 73–90. Oxford University Press, Oxford.
- Pentecost , A and Spiro , B . 1990 . Stable carbon and oxygen isotope composition of calcites associated with modern freshwater cyanobacteria and algae . Geomicrobiol. J. , 8 : 17 – 26 .
- Pentecost , A and Whitton , BA . 2000 . “ Limestone ” . In The Ecology of Cyanobacteria , Edited by: Whitton , BA and Potts , M . 257 – 279 . Dordrecht : Kluwer .
- Reddy , MM . 1995 . “ Carbonate precipitation in Pyramid Lake, Nevada ” . In Mineral Scale Formation and Inhibition , Edited by: Amjad , Z . 21 – 32 . New York : Plenum .
- Revsbech, N.P. (1994). Analysis of microbial mats by use of electrochemical microsensors: recent advances. In Microbial Mats (Stal, L.J. & Caumette, P. editors), 135–147. NATO ASI Series Vol. G35. Springer, Berlin.
- Riding , R . 1999 . The term stromatolite: towards an essential definition . Lethaia , 32 : 321 – 330 .
- Rumsey , WL , Vanderkooi , JM and Wilson , DF . 1988 . Imaging of phosphorescence – a novel method for measuring oxygen distribution in perfused tissue . Science , 241 : 1649 – 1651 .
- Sabater , S . 2000 . Structure and architecture of a stromatolite from a Mediterranean stream . Aquatic Microb. Ecol. , 21 : 161 – 168 .
- Shiraishi , F , Bissett , A , De beer , D , Reimer , A and Arp , G . 2008 . Photosynthesis, respiration and exopolymer calcium binding in biofilm calcification (Westerhöfer and Deinschwenger Creek, Germany) . Geomicrobiol. J. , 25 : 83 – 94 .
- Spiro , B and Pentecost , A . 1991 . One day in the life of a stream – a diurnal inorganic carbon mass balance for a travertine-depositing stream (Waterfall Beck, Yorkshire) . Geomicrobiol. J. , 9 : 1 – 11 .
- Visscher , PT , Reid , RP , Bebout , BM , Hoeft , SH , Macintyre , IG and Thompson , JA . 1998 . Formation of lithified micritic laminae in modern marine stromatolites (Bahamas); the role of sulfur cycling . Amer. Mineral. , 83 : 1482 – 1493 .
- Wenzhöfer , F and Glud , RN . 2004 . Small-scale spatial and temporal variability in benthic O2 dynamics of coastal sediments: effects of fauna activity . Limnol. Oceangr. , 49 : 1471 – 1481 .
- Whitton , BA . 1987 . “ The biology of the Rivulariaceae ” . In The Cyanobacteria, a Comprehensive Review , Edited by: Fay , P and van Baalen , C . 513 – 534 . Amsterdam : Elsevier .
- Whitton , BA . 2002 . “ Phylum Cyanophyta ” . In The Freshwater Algal Flora of the British Isles , Edited by: John , DM , Whitton , BA and Brook , AJ . 25 – 122 . Cambridge : Cambridge University Press .