Abstract
Alexandrium is detected throughout Scottish coastal waters on a near annual basis, and corresponding paralytic shellfish poisoning (PSP) toxins are found in Scottish shellfish. Previous studies at selected Scottish sites have shown diversity within the genus Alexandrium. In order to examine the distribution, diversity and toxicity of Alexandrium populations around the Scottish coast, historic cyst and cell data were compiled and cultures established from sediment and water samples. Historic data showed high cell densities of Alexandrium in Shetland, Orkney, the Western Isles and mainland east coast. Low abundances of Alexandrium cysts were observed along the west coast. Four species of Alexandrium (A. tamarense, A. ostenfeldii, A. minutum and A. tamutum) were established in laboratory culture and identified using morphological criteria. Sequencing of LSU rDNA from isolates of A. ostenfeldii, A. minutum and A. tamutum confirmed their identification and showed them to be similar to other European strains. Alexandrium tamarense, identified by morphological criteria, was observed to have a widespread distribution around the coast. Both toxin- and non toxin-producing strains of this species were isolated, suggesting the presence of A. tamarense Groups I and III. Alexandrium ostenfeldii was isolated from the east coast and Shetland Isles and was observed to produce both spirolide and PSP toxins. Alexandrium tamutum was identified from cultures isolated from Shetland and Orkney, the most northerly observation of this species to date. PSP toxins were not detected in isolates of A. minutum from the east coast and Orkney or of A. tamutum under the culture conditions used. This study has highlighted the diversity of Alexandrium in Scottish waters and reveals the requirement for temporal and regional studies on a species level in order to understand the variation in cell densities and PSP toxicity that is observed on an annual and decadal scale.
Introduction
Members of the dinoflagellate genus Alexandrium are observed as part of the summer phytoplankton community in Scottish coastal waters on an annual basis. Associated paralytic shellfish poisoning (PSP) toxins are regularly detected in mussels (Mytilus edulis) during the summer months (Bresnan et al., Citation2008). Monitoring for the presence of PSP toxins in the UK began in 1968 along the north-east coast of England (Ayres & Cullum, Citation1978). In 1990, the level of monitoring was expanded along the Scottish east and west coasts and also included sites in Northern Ireland. PSP toxin concentrations exceeding 1000 µg STX eq. 100 g−1 (1000 µg saxitoxin 100 g−1 shellfish flesh) were observed in Mytilus edulis from the Scottish and Northern Irish coasts (Marine Scotland Science (MS-S), unpublished data) and for the first time PSP toxins were detected in crustaceans including crabs, lobsters and prawns, albeit in trace amounts (Wyatt & Saborido-Rey, Citation1993). In response to the increased detection of PSP toxins in 1990, PSP toxin monitoring was extended around the Scottish coast and monitoring of coastal waters for the presence of Alexandrium began in 1996.
Analysis of data from these monitoring programmes revealed areas around Orkney, Shetland and the Western Isles (see for locations of island groupings), as well as the east coast mainland, as ‘hot-spots’ for the detection of both Alexandrium cells in the water column and PSP toxicity in shellfish (Bresnan et al., Citation2008). Examination of sediments from the east coast showed Alexandrium cyst beds to be prevalent, with high densities of Alexandrium cf. tamarense identified in Scapa Flow, Orkney (Joyce, Citation2001) and Alexandrium spp. offshore of Aberdeen, the Firth of Forth and offshore from the rivers Tyne and Tees in the north-east of England (Lewis et al., Citation1995). Previous investigations into species diversity identified A. tamarense (Group I, as defined by Lilly et al., Citation2007), A. tamutum, A. minutum and A. ostenfeldii in this east coast region (Medlin et al., Citation1998; Higman et al., Citation2001; John et al., Citation2003; Alpermann et al., Citation2008; Collins et al., Citation2009). Until recently, little investigation into Alexandrium diversity on the west coast had been undertaken with only A. tamarense (Group I) reported from one site (Higman et al., Citation2001). Recently, a more comprehensive survey revealed the presence of A. tamarense (Group III, as defined by Lilly et al., Citation2007) at the west coast as well as in the Orkney and Shetland Isles (Collins et al., Citation2009).
Fig. 1. Distribution of Alexandrium cysts (square root transformed) collected from 1992 to 1998. Cyst data obtained from Lewis et al. (Citation1995) are included. Symbol areas linearly scaled to cyst concentrations.
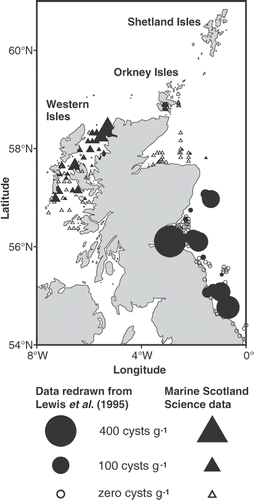
A correlation between Alexandrium cell numbers and PSP toxicity in shellfish flesh was observed in Scottish waters in the past, with relatively low Alexandrium cell densities of 1000 to 2000 cells l−1 associated with concentrations of PSP toxins exceeding the regulatory limit (80 µg STX eq. 100 g−1) in shellfish (Bresnan et al., Citation2005; Stobo et al., Citation2008). This contrasts with very high concentrations of Alexandrium cell numbers in English waters that can occur without associated toxicity detected in shellfish (Higman et al., Citation2001). Previous molecular analyses of the D1/D2 region of the LSU rDNA showed the distribution of toxin-producing A. tamarense (Group I) to be restricted to Scottish waters (Medlin et al., Citation1998; Higman et al., Citation2001; John et al., Citation2003; Collins et al., Citation2009) while the non-toxic A. tamarense Group III had a more widespread distribution around the British Isles (Medlin et al., Citation1998; Higman et al., Citation2001; John et al., Citation2003; Neale et al., Citation2007; Collins et al., Citation2009).
Spirolides have been detected in plankton net tow samples taken near the east coast (Luckas et al., Citation2005; Krock et al., Citation2008) and in shellfish samples from the west coast (Stobo et al., Citation2006), however Alexandrium ostenfeldii has yet to be confirmed as a potential spirolide producer in these areas.
In order to aid understanding of the distribution of Alexandrium in Scottish waters, historic data were compiled to examine the location of Alexandrium cyst beds and the occurrence of vegetative cells in the water column. To further investigate the diversity of Alexandrium, cultures were established from a wide geographical area and were identified to species level using fluorescence microscopy and sequence analyses of the LSU rDNA. In addition, the toxicity of the Alexandrium cultures was investigated using the Jellet Rapid Test (JRT) kit (Jellet Rapid Testing Ltd, Nova Scotia, Canada) and liquid chromatography mass spectrometry (LC-MS/MS) in order to assess potential toxin production in Scottish waters.
Materials and methods
Regional examination of Alexandrium in Scottish waters
To construct a regional distribution map of Alexandrium cysts from around the Scottish coast in the 1990s, information from two sources (Lewis et al., Citation1995) and MS-S (unpublished data) was collated. MS-S data were collected on three cruises in November 1996, December 1996 and September 1998 and analysed by MS-S (). Sediment samples were collected using a Day grab sampler during both studies. Lewis et al. (Citation1995) also used a hand corer for collection of coastal samples. All samples were processed and analysed using the method described in Lewis et al. (Citation1995).
During a 10-year period (1996–2005) Alexandrium cells from weekly phytoplankton samples collected from around the Scottish coast were enumerated (). Samples were collected and analysed using the method described in Bresnan et al. (Citation2008).
Sample collection and isolation of Alexandrium
In order to generate Alexandrium cultures, sediment and water samples were collected between 2006 and 2008 from locations around the Scottish coast including the Orkney, Shetland, Western Isles and mainland east and west coasts (). Samples were collected during autumn, winter and spring months. Subsamples of sediment were extracted and added to 50 ml filtered seawater (gravity-filtered through a 0.45 µm cellulose Whatman nitrate filter (VWR)), sonicated to break up particles and filtered through an 80 µm and 20 µm mesh sieve (Endecotts Ltd). The remaining sediment slurry was backwashed into a 15 ml centrifuge tube. A 500 µl aliquot was added to a single well of a 12-well culture plate. Each well was inoculated with 10% L1 minus silicate (L1-Si) phytoplankton growth media (Guillard, Citation1995). Plates were incubated under a 12:12 light:dark cycle at saturating light intensity (110 µmol m−2 s−1) at 15°C to encourage hatching of cysts. In addition 5 ml aliquots from 1 l unpreserved seawater samples were examined for the presence of free-swimming Alexandrium cells. Single cells were isolated by micropipette into single wells in 24-well culture plates inoculated with 10% L1-Si growth media. Once established, cultures were grown in 100% L1-Si growth media to encourage high cell densities, at 15°C under continuous light. These cultures were maintained in this light regime by monthly subsampling. Full protocols of sample processing, cell isolation and culture maintenance are detailed in Collins et al. (Citation2009). It was not possible to count Alexandrium cysts in sediment samples.
Morphological identification of Alexandrium cells
Alexandrium cells were identified using thecal plate dissection with Calcofluor White M2R (Sigma-Aldrich) (Fritz & Triemer, Citation1985). All cultures were examined under a Leica DMLB fluorescence microscope using a BG 38 blue filter setting, bandpass 420–490 nm emission and an H3 filter cube. Images of the relevant thecal plate structure were recorded using a Hamamatsu colour chilled 3CCD camera. Images were recorded using Leica software IM1000. Literature was consulted to assist in identification (Balech, Citation1995; Steidinger & Tangen, Citation1997; Taylor et al., Citation2003; Gribble et al., Citation2005; Lim et al., Citation2005; Gayoso & Fulco, Citation2006; Orlova et al., Citation2007; Wang et al., Citation2008).
Molecular identification of Alexandrium
Amplification of partial large subunit ribosomal DNA (LSU rDNA) of single Alexandrium cells obtained from a selection of clonal cultures () was carried out as described in Collins et al. (Citation2009) using the primers D1R (5′-ACCCGCTGAATTTAAGCATA-3′) and D2C (5′CCTTGGTCCGTGTTTCAAGA-3′) (Scholin et al., Citation1994). Alexandrium cultures (CCAP 1119/13 and CCAP 1119/15) originally isolated from Orkney and identified to genus level were obtained from the Culture Collection of Algae and Protozoa (Oban, Scotland) and included in the analysis. PCR products were visualized on agarose gels containing ethidium bromide, under UV illumination.
Table 1. Details of Alexandrium cultures from Scotland generated as part of this study.
PCR products were excised from the gels, purified using MinElute gel purification kits (Qiagen Ltd., Crawley, UK) according to the manufacturer's instructions and their concentrations estimated following agarose gel electrophoresis alongside mass markers (Low DNA Mass Ladder, Invitrogen). Purified products were either sequenced directly or were cloned into pCR4-TOPO plasmid vectors (TOPO TA Cloning Kit for sequencing) (Invitrogen Ltd., Paisley, UK) and were used to transform One Shot TOP10 chemically competent cells (Invitrogen Ltd., Paisley, UK), following manufacturer's instructions. Plasmids were purified using the QIAprep Spin Miniprep Kit (Qiagen Ltd.) according to the manufacturer's instructions and were quantified as described previously for PCR products.
Purified PCR product (40 to 80 ng) or purified plasmid (200 to 250 ng) was used as template in sequencing reactions as described by Collins et al. (Citation2009) using the GenomeLab DTCS – Quick Start Kit (Beckman Coulter) and a Beckman Coulter CEQ 8800 DNA Analysis system (Beckman Coulter). Forward and reverse sequencing reactions were performed with the same primers as used to generate PCR products, and sequences were checked using Sequencher software (Intelligenetics/Gene Codes Corporation).
To identify Alexandrium species from the sequences, a search was performed through the NCBI database using BLAST nucleotide search (Zhang et al., Citation2000).
Partial LSU rDNA consensus sequences from the Scottish isolates were aligned with available A. minutum, A. ostenfeldii and A. tamutum sequences retrieved from GenBank. Alexandrium ostenfeldii, A. minutum and A. tamutum sequences from Irish waters, supplied by Dr N. Touzet from NUI Galway, Ireland, were also included in the analysis. Identical sequences were identified and duplicates removed, leaving one representative sequence for each. The alignments were manually edited using the BioEdit programme (Hall, Citation1999). All gaps and ambiguous positions were removed resulting in a final alignment of 519 nucleotides for the A. tamarense LSU rDNA, using A. tropicale as the outgroup (Collins et al., Citation2009) and 538 nucleotides for the A. minutum, A. ostenfeldii and A. tamutum LSU rDNA, using Alexandrium andersonii as the outgroup. Alexandrium tamarense isolates were not included in the analysis as these have already been presented in Collins et al. (Citation2009).
Phylogenetic relationships were inferred using PAUP v. 4.0B10 PPC (Swofford, Citation1999) and Maximum Likelihood (ML) and neighbour joining (NJ) analyses. Modeltest v. 3.01 (Posada & Crandall, Citation1998) was used to determine the best-fit model. Bootstrap analysis using 1000 replicates was performed to determine support for branching in phylogenetic trees.
Analysis of PSP toxins using the Jellet Rapid Test (JRT)
All Alexandrium cultures were screened for PSP toxicity using the JRT kit. Cells (approximately 250 000) from Alexandrium cultures were concentrated onto a 10 µm isopore membrane filter (Millipore, Ireland) under vacuum. Filtrate (400 µl) was used to wash cells from the filter into a sterile plastic universal bottle and 500 µl of 0.1 M acetic acid was added to extract the PSP toxins as sonication has not been observed to improve the extraction (Laycock et al., Citation2010). Phytoplankton buffer (400 µl), as supplied by the manufacturer, was added to 100 µl of extract and mixed by pipetting up and down three times. One hundred microlitres of each mixture was then added to the sample pad on JRT strips and after 20 min each strip was visually examined for the presence or absence of test (T) and control (C) lines. Tests were scored according to the kit instructions. The colour intensity of the T line compared to the C line determines if a sample is PSP toxin positive (absence of T line) or negative (T line intensity equal to C line). Sensitivity of the test varies with different analogues of PSP toxins (Laycock et al., Citation2010), however Laycock (pers. comm.) suggests a sensitivity to STX of 0.3 ng ml−1 which indicates a limit of detection of 0.006 pg STX cell−1.
Detection of toxins using LC-MS/MS
Certified reference calibration solutions for PSP toxins containing gonyautoxins (GTX1, GTX2, GTX3, GTX4, GTX5), decarbamoyl-gonyautoxins (dcGTX2, dcGTX3), saxitoxin (STX), neosaxitoxin (NEO), decarbamoyl-STX (dcSTX), decarbamoyl-NEO (dcNEO), N-sulfocarbamoyl toxins (C1 and C2) and spirolide 13-desMethyl C (SPX1) were purchased from the National Research Council Institute for Marine Biosciences (NRC-IMB), part of the Canadian National Research Council (CNRC), Halifax, Nova Scotia, Canada. All solvents were purchased from Rathburns Chemicals Ltd. (Walkerburn, UK). All solvents and reagents were of analytical grade and were used without further purification.
PSP toxins and spirolides were determined using a 3200 QTrap® hybrid triple quadrupole-linear ion trap mass spectrometer (MS/MS) equipped with an atmospheric pressure ionization fitted with an electrospray ionization source (Turbo V® Source) supplied by Applied Biosystems (Warrington, UK). The MS/MS was coupled to an Agilent 1200 series LC system comprising a degasser, binary pump, column oven and autosampler.
Following the JRT screen, cells (approximately 250 000) from Alexandrium cultures, selected on the basis of sufficient cell density being present (), were obtained by centrifugation at 3000 rpm for 20 min for PSP toxin detection. Limits of detection per cell for the various PSP toxins examined ranged from 0.2 fg STX diHCl eq. cell−1 (C1) to 35.0 fg STX diHCl eq. cell−1 (NEO). Acetic acid (500 µl, 0.5 M) was added to the cell pellet with toxin extraction undertaken by freezing (−80°C for 30 ± 5 min) and thawing (room temperature for 30 ± 5 min) three times. The samples were subsequently mixed using a vortex mixer and centrifuged at 10 000 rpm for 20 ± 5 min using a microcentrifuge. The sample extracts were transferred to a Millipore UltraFree MC 0.2 µm filter unit and centrifuged at 6000 rpm for 3 ± 1 min. An aliquot of each extract (50 µl) was combined with 50 µl of acetonitrile in order to improve the chromatography prior to analysis by LC-MS/MS (5 µl injection volume).
Multiple reaction monitoring (MRM) transitions and instrument settings used with the 3200 Qtrap MS/MS are provided in Turrell et al. (Citation2009). Calibration standard solutions ranging from 0.05 to 1.0 (for GTX1, GTX2, GTX5, dcGTX2, STX, NEO, dcSTX and dcNEO) were used for toxin quantification. Limits of detection ranged from 9 nM (dcGTX3) to 147 nM (NEO). Analyses were carried out in the positive ion mode. A ZIC-HILIC analytical column (150 × 2.1 mm, 3.5 µm particle size, Sequant, Sweden) was used for PSP toxin separation attached to a ZIC-HILIC guard column (14 × 1.0 mm). All compounds were separated in a single 35-min analysis which included an equilibration period. Correction was made for any ion suppression/enhancement from the phytoplankton extracts via the use of standard addition.
Alexandrium ostenfeldii culture (S06/013/01) was examined for the presence of spirolides. Spirolides were extracted from A. ostenfeldii cells using the micro-extraction procedure described and validated by Cembella et al. (Citation1999). Samples (0.5 ml) of cultures containing 250 000 cells were filtered by centrifugation (3 min at 3000 rpm) through a 0.2 µm spin filter pre-washed with methanol (Ultrafree-MC, Durapore membrane, Millipore, Watford, UK). The filtrate was discarded. Spirolides were extracted from the cell fraction by incubation with methanol (100 µl) for 5 min directly on the spin cartridge membrane, followed by centrifugation for 30 s at 3000 rpm. To ensure complete extraction of any spirolides, this procedure was repeated with a further 100 µl methanol filtrate through the spin cartridge. The filtrates were combined and transferred to 350 µl insert amber vials and analysed by LC-MS/MS.
Screening, confirmation and quantification of known spirolides were performed simultaneously in a single analysis using MRM experiments with selected transitions characteristic of the presence of known spirolides (A, B, C, D, E, F, G, 13-desMeC, 13-desMeD and 20-MeG) and by performing enhanced mass spectra (EMS) scans for confirmation. In this scan mode the third quadrupole Q3 was used as a linear ion trap. Ions were transferred directly from the ion source and orifice region to Q3, collected and stored before being scanned out, giving increased sensitivity compared to a full scan analysis.
The instrument was optimized for spirolides using SPX1 standard (NRC) by infusion and flow injection analysis in positive ion mode. This optimization process was performed manually and automatically using Analyst 1.4.2 software. The 13-desMeC single charged [M + H]+ ion at m/z 694.2 (precursor ion) was monitored as well as the product ion at m/z 444.2 in both SPX1 calibration standards (ranging from 1.0 µg ml−1 to 50.0 µg ml−1) and culture extracts. The spirolide analogue present was quantified by direct comparison of the peak area of the respective most abundant transition with that obtained by injection of SPX1 calibration standards assuming the response factor for the spirolides to be identical. This approximation was used due to the lack of commercially available certified calibration solutions for other spirolide analogues at the time of the study.
Chromatographic separation was achieved by attaching two columns to improve separation and resolution. The first column was a Hypersil BDS C8, 3 µm packing, 50 mm by 2.1 mm internal diameter attached with a dead volume connector to a Phenomenex Luna C18(2) HST, 2.5 µm packing, 100 × 2.0 mm internal diameter column. A BDS Hypersil C8 guard column, 3 µm packing, 10 × 2.1 mm internal diameter was attached to the combined columns. The mobile phase was composed of phase A: deionized water and phase B: acetonitrile, both containing 2 mM ammonium formate and 50 mM formic acid. An elution gradient was used as follows: 35% B for the first 2 min then gradient to 100% B over 10 min, hold at 100% B for 3 min, back to 35% B in 0.1 s and equilibration for 6.9 min. The flow rate was 200 µl min−1, the injection volume was 5 µl and the column compartment temperature was maintained at 35°C throughout the analyses.
Results
Distribution of Alexandrium in Scottish waters
Collation of data on the distribution of Alexandrium cysts in the late 1990s confirms the presence of Alexandrium cysts on the west coast of Scotland (). Cyst densities from this region ranged from 10 to 100 cysts g−1 sediment with the exception of one sea loch (Eddrachillis Bay, 58.5° N) on the north-west coast where 373 cysts g−1 was recorded. Lewis et al. (Citation1995) reported high Alexandrium cyst densities of 400 cysts g−1 of sediment on the east coast, from the River Tees in the North East of England (55° N) to the Firth of Forth (56° N) and offshore of Aberdeen (57° N) (). In contrast, cyst densities offshore within the Moray Firth (58° N) and Orkney Isles were lower than the densities observed in the Firth of Forth with the maximum being less than 100 cysts g−1. No cysts were observed in the sediment of many areas sampled within the Moray Firth.
High Alexandrium cell densities (maximum annual average > 200 cells l−1) in water samples were observed in the Orkney, Shetland and Western Isles. Maximum annual average cell densities on the east coast were higher (100 cells l−1) than those observed in sea lochs along the west coast (<100 cells l−1) (). Highest Alexandrium maximum annual average cell densities were observed in the Shetland Isles, where high concentrations of PSP toxins (>80 µg STXeq. 100 g−1 shellfish flesh) are regularly detected (Stobo et al., Citation2008; Swan & Davidson, Citation2008). Alexandrium cells were not identified to species level. No cyst data are available from this region.
Diversity and toxicity of Alexandrium in Scottish waters
A total of 24 cultures were established during the course of this study. Locations and information on isolates are presented in Figs and . Thecal plate examination of cultured cells revealed 15 A. tamarense, five A. ostenfeldii, three A. minutum and one A. tamutum culture (). Alexandrium tamarense cultures had the most widespread distribution amongst all species cultured. These A. tamarense cultures were established from the east and west coasts and Western, Orkney and Shetland Isles (). Isolates were identified using thecal plate morphology only and were grouped on the basis of their toxin production detected using JRT under the culture conditions detailed in the methods section. Using these criteria, they have been termed ‘toxic’ and ‘non-toxic’. To present a comprehensive regional distribution, locations of toxic Group I and non-toxic Group III A. tamarense isolates, identified using molecular techniques as detailed in Collins et al. (Citation2009), are also included. A small number of isolates of A. ostenfeldii, A. minutum and A. tamutum were established into laboratory culture from the east coast, Orkney and Shetland Isles (). The identification of two previously unidentified isolates obtained from CCAP was confirmed during this project as A. tamutum and locations of these isolates are also shown in .
Fig. 4. Locations of toxin- and non toxin-producing Alexandrium tamarense established in culture during this study. Cultures of A. tamarense Groups I and III detailed in Collins et al. (Citation2009) are included.
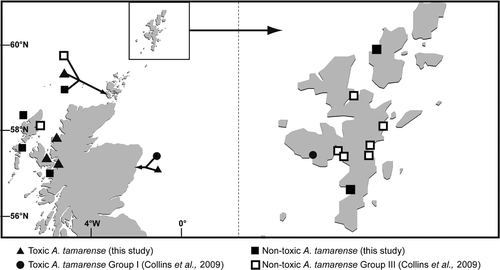
Alexandrium tamarense
Cell morphology was typical of the A. tamarense form in current literature (Figs ). Light microscopy analysis demonstrated that the general cell shape of the Scottish isolates varied significantly, ranging from slightly rounded, to more hexagonal to slightly oval, all showing a deeply excavated cingulum (, ). Cell size ranged from 24.5 to 39.2 µm in diameter and 24.5 to 44.1 µm in length. Cells were observed singly or as two-celled chains. Less frequently, chains of three and four cells were observed. Visual pigmentation varied throughout the cultures, with some being slightly darker than others. Calcofluor staining revealed that the apical pore complex (APC) was always attached to the first apical plate (1′) (, ). The apical pore was typical of A. tamarense (). An obvious ventral pore (Vp) was present on the 1′ plate in all cultures (, , ). Variation occurred in the presence and absence of a posterior attachment pore (Pap) on the posterior sulcal plate (Sp) (, ). This variation was apparent between and within cultures; the attachment pore was seen on the Sp of some cells in cultures listed in .
Figs 6–15. Alexandrium tamarense from Scottish waters, identified using light microscopy with epifluorescence. . General cell shape in dorsal view showing well developed cingulum. . Ventral-apical view showing attachment of APC with 1′, small ventral pore (Vp) on 1′ and 6′′. . Detailed view of apical pore (Po). . Ventral view showing 1′ and 6′′ and sulcal plates. , . Detailed view of 1′ with small Vp and 6. . Antapical view of Sp with no posterior attachment pore (Pap). . Antapical view showing Sp Pap. Scale bars represent: Figs , , 10 µm; , 5 µm.
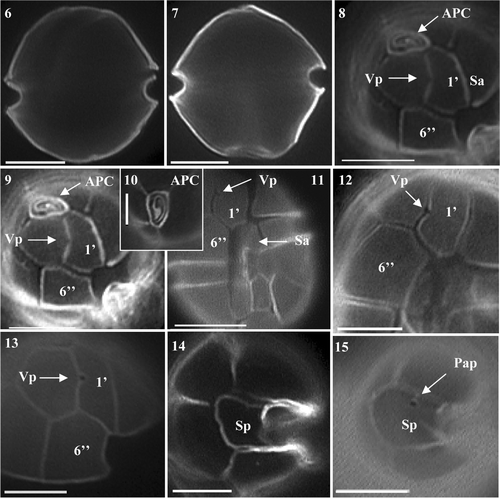
Toxin testing by JRT revealed positive PSP production in six of the 15 A. tamarense isolates (). LC-MS/MS analysis of these isolates showed profiles to be dominated by the N-sulfocarbamoyl C2 toxin and the potent carbamate toxins NEO, STX, GTX-4, GTX-3. Lower concentrations of corresponding epimeric C and GTX toxins including GTX-5 were observed (, –) as well as trace levels of dcGTX-3 and dcSTX and dcGTX 2 in some cultures. Quantification of the PSP toxins in cell extracts was carried out by comparisons with the standards.
Fig. 16. MRM chromatograms (with variable intensity) from LC-MS/MS analysis of extracts of Alexandrium tamarense isolated from Scottish coastal waters.
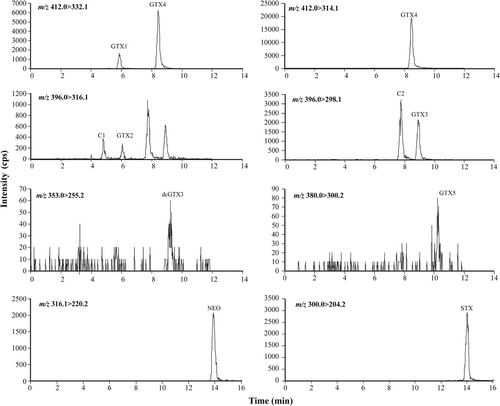
Fig. 17. Histogram detailing percentage contribution of PSP toxin analogues detected in Scottish A. tamarense isolates by LC-MS/MS.
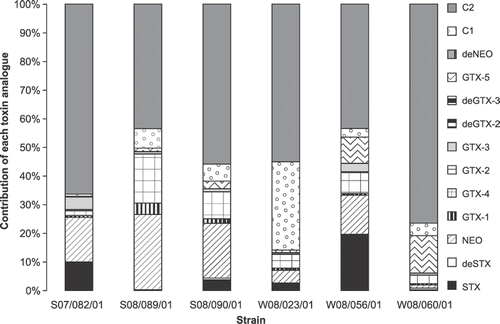
Table 2. Toxins produced by Alexandrium maintained in batch culture in L1 media.
Alexandrium ostenfeldii
Alexandrium ostenfeldii exhibited the greatest variation in cell size among the four species and was the slowest growing species established. Cell size ranged from 29.4 µm to 58.8 µm in diameter and 24.5 to 58.8 µm in length. Cells were almost spherical in shape and appeared as solitary individuals (). Only very occasionally were chains of two cells observed. Cells did not possess a deeply excavated cingulum, instead it was rather shallow (). Calcofluor staining revealed a typical APC connecting to the characteristic 1′ plate containing a very large Vp (, ). The 1′ was a typically long and narrow rectangular-shaped plate. Although similar to the 1′ of A. peruvianum, the right margin of the plate was straighter and more angular than the smoother, curved nature of A. peruvianum (Figs ). The 6′′ plate was wider than long, again a feature shared with A. peruvianum, however the difference was more pronounced in A. ostenfeldii (Figs ). The shape of the anterior sulcal plate (Sa) was found to be wider than long and more rectangular (, ) in comparison to the more triangular shape seen in A. peruvianum. Its margins appeared more rounded (, ) contrasting with the sharp outline of A. peruvianum. The Sp was asymmetrical and did not display a great difference in the length to width ratio, appearing more box-shaped than rectangular (, ). The plate lacked a Pap in all cultures (, are representative of this plate).
Figs 18–26. Alexandrium ostenfeldii from Scottish waters, identified using light microscopy with epifluorescence. . General cell shape. . Epithecal plates showing clear contact between APC and 1′, with large ventral pore and wide 6′′. . Apical view of APC. . Ventral view showing 1′ with large ventral pore and wide 6′′. , . 1′ with large ventral pore, Sa plate and partial view of 6′′. . Epithecal plates showing APC 1′ and wide 6′′. , . Antapical view showing Sp with no Pap. Scale bars represent: 10 µm.
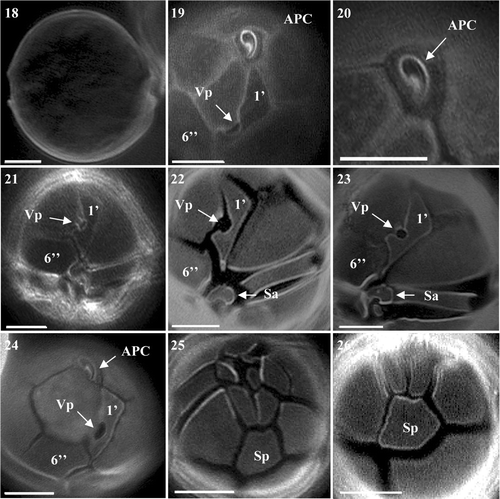
The BLAST search identified the LSU rDNA consensus sequences obtained from isolates S06/013/01 and S06/015/01 as A. ostenfeldii. These isolates had identical LSU rDNA sequences. Phylogenetic analysis of the available A. ostenfeldii and A. peruvianum LSU sequences divided the A. peruvianum isolates IEO-VGOAMD12 and IEO-VGOAM10C from Spain from A. ostenfeldii. It then separated the A. ostenfeldii strains AOTVA4 and AOTVA1 from Finland as sister to two relatively well-supported clades, a New Zealand clade (bootstrap support, BP, 96%) and a Northern European/North American clade (BP 92%) (). The Northern European/North American clade was further divided into two clades, giving a Northern European group (BP 99%) consisting of the Scottish isolates, the two Danish strains K0287 and K0324 and the Irish strain BY.K04, and a weak North American group (BP 56%). The Northern European strains are genetically distinct from the Finnish strains even though they are geographically close ().
LC-MS/MS was used to simultaneously collect quantitative data with confirmatory information using methanol extracts from an isolate of A. ostenfeldii (S06/013/01). MRM was used for quantification and EMS for confirmation. A single peak (RT = 10.6 min) was present in chromatograms (). The mass spectrum obtained from the EMS scan showed the characteristic fragmentation pattern of a spirolide (). Typically, the successive losses of water (loss of m/z 18) firstly from the precursor ion MH+ at m/z 706.7 (m/z 706.7 → 688.8 → 670.9 → 652.6), secondly from a cluster of ions (m/z 392.3 → 374.3 → 346.3) and the presence of an intense fragment ion at m/z 164.2 are associated with the cyclic imine system of a spirolide. The fragmentation pattern of the A. ostenfeldii extract matches the fragmentation pattern of 20-methyl spirolide G as proposed by Aasen et al. (Citation2005). Using the response factor from spirolide desMeC, A. ostenfeldii produced an estimated concentration of 19 500 fg 20-methyl spirolide G cell−1, while other spirolide derivatives were not detected (). Although the isolate tested negative for PSP toxins using the JRT (), it proved positive for the production of PSP toxins when examined using LC-MS/MS. Low concentrations of both STX and NEO were detected in exponentially growing cells resulting in a low cell-specific PSP toxicity of 81 fg STX eq. cell−1 ().
Alexandrium minutum
Identification of A. minutum was straightforward. Using light microscopy (LM), the obvious small size, general oval shape and light colouration of cells suggested that cultures were of the A. minutum group (A. minutum, A. andersonii and A. tamutum). Cells were spherical to slightly oval in shape, always with a pronounced cingulum (Figs ). Only solitary cells were observed in all three cultures. Cell diameter ranged from 14.7 to 24.5 µm and length varied from 14.7 to 27.0 µm. The distinctive plates 1′, 6′′, Sa and Sp were typical of A. minutum, being characteristically long and narrow (Figs ). The APC was found attached to the 1′ plate and a small ventral pore was present in all A. minutum cultures (Figs ). No variation was observed in the degree of reticulation in the hypotheca or areolation of plates. Only one culture, S07/020/01 generated from east coast offshore sediment, possessed a Pap present on the Sp (, ). Both cultures from Scapa, Orkney (W07/001/01 and W07/025/01) lacked this pore. The Sp plate was again typical of A. minutum, being wider than long ().
Figs 30–38. Alexandrium minutum from Scottish waters, identified using light microscopy with epifluorescence. . General cell shape. . Dorsal view of cell. . Ventral view showing 1′, 6′′ and Sa. . Ventral view of cell showing sulcal plates and descending cingulum. . Ventral view showing descending cingulum. . Long and narrow 1′ with clear ventral pore and 6′′ plate. , . Narrow 1′ and 6′′ plates. . Antapical view showing Sp with attachment pore. Scale bars represent: Figs , , 10 µm; , 5 µm.
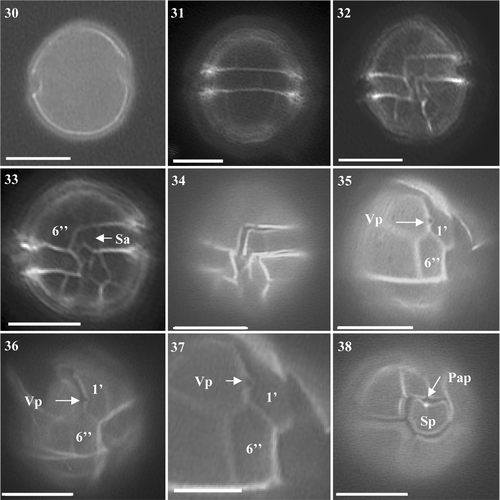
The BLAST search identified the Scottish isolates S07/020/01 and W07/001/01 as A. minutum based on their LSU rDNA consensus sequences. These isolates gave identical LSU rDNA sequences. Phylogenetic analysis revealed that they fell into the global clade ribotype as described by Lilly et al. (Citation2005) ().
Toxicity analysis of extracts from cultured cells using Jellet Rapid Test (limit of detection of 0.006 pg STX cell−1) and LC-MS/MS (limits of detection: 0.2 fg STXdiHCl eq. cell−1 (C1) to 35.0 fg STXdiHCl eq cell−1 (NEO) of cultures S07/020/02 and W07/001/01 showed no evidence of the presence of any PSP toxins under the culture conditions used in this study ().
Alexandrium tamutum
There were no obvious differences between A. tamarense and A. tamutum under LM. Alexandrium tamutum was more similar in size to A. tamarense than to A. minutum, although it was slightly more oval in shape (Figs ). Sizes of cells varied between 24.5 to 34.3 µm in diameter and 24.5 to 36.8 µm in length, lying within the ranges found for the A. tamarense and A. minutum isolates. General cell shape was spherical with the cingulum not as pronounced as in A. tamarense or A. minutum (Figs ). Cells did not appear as heavily pigmented as A. tamarense or A. minutum. The 1′ plate was wider and the 6′′ and Sp plates were wider than long (Figs ). A further difference was the position of the ventral pore on the anterior right margin of the 1′ plate. The pore of A. tamutum was found to be more centrally located within the margin of the 1′ plate (Figs ), a similarity with A. tamarense, whereas on A. minutum it was positioned further down the margin towards the sulcal plates. The Sp did not possess a Pap ().
Figs 39–47. Alexandrium tamutum from Scottish waters, identified using light microscopy with epifluorescence. . General cell shape. . Ventral view showing sulcal plates and descending shallow cingulum. , . Dorsal view of cell. . Ventral view showing 1′ with ventral pore and wide 6′′. , . Epithecal plates showing contact between the APC and 1′. . Epitheca showing 1′ and 6′′. . Antapical view showing Sp. Scale bars represent: 10 µm.
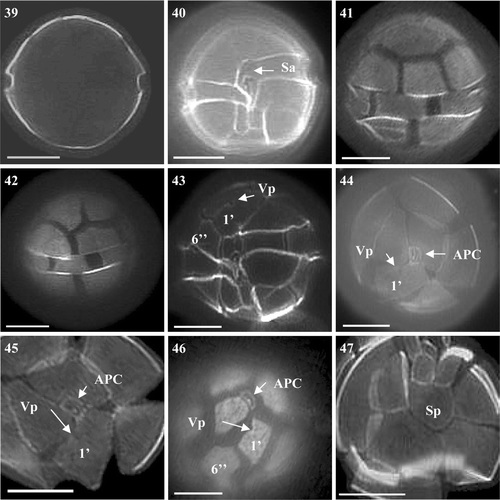
The BLAST search identified the LSU rDNA consensus sequences obtained from Scottish isolate S07/036/01 as A. tamutum and showed it to cluster with other European isolates as illustrated by the positioning on . Additional cultures obtained from the CCAP (1119/15 and 1119/13) were also identified as A. tamutum. These cultures originated from Orkney, with CCAP 1119/15 being identical to the A. tamutum culture generated in this study. Phylogenetic analysis placed the Scottish isolates with other A. tamutum strains isolated from the Mediterranean and Ireland ().
PSP toxin production was not detected in isolate S07/036/01 when analysed by JRT () and LC-MS/MS, under the culture conditions used.
Discussion
This study confirms Alexandrium in Scottish water as being a diverse genus, with four species identified (A. tamarense, A. ostenfeldii, A. minutum and A. tamutum). Compilation of historic data reveals a regional distribution to both Alexandrium cysts and cells, with higher cyst densities (400 cysts g−1) observed along the east coast of Scotland, and high cell densities (maximum annual averages of 100–300 cells l−1) observed along the east coast, Orkney, Shetland and Western Isles. In contrast, low maximum annual averages of Alexandrium cells were recorded in sea lochs along the west coast of Scotland (<100 cells l−1). This distribution of Alexandrium cells is reflected in the high incidences of shellfish toxicity recorded in those areas during the 1990s (>80 µg STX eq. 100 g−1 shellfish flesh) (Bresnan et al., Citation2008). The low cyst abundance (<100 cysts g−1) observed along the west coast, excluding Eddrachillis Bay in the northwest, may contribute to the low cell abundances routinely observed in this area as cyst beds are known to act as a seed bed for Alexandrium blooms (Anderson et al., Citation2005; Martin et al., Citation2009). Both Joint et al. (Citation1997) and Brown et al. (Citation2001) have used hydrographic models to identify the source of the east coast Alexandrium bloom and PSP events of the early 1990s. They could not come up with a definitive source for these blooms although Joint et al. (Citation1997) suggested warmer temperatures and earlier stratification may have had an influence.
Historic Alexandrium data comprise genus level LM cell counts. In this study Scottish Alexandrium species showed similar or identical genotypes to the same species from other European locations, based on LSU rDNA sequences, however for some species such as A. ostenfeldii and A. tamutum, molecular comparisons were limited by the low number of European sequences in the GenBank database (). Some morphological variability was observed in the thecal plate structure of each species but in general observations were in keeping with descriptions in the scientific literature.
Both toxin-producing and non toxin-producing A. tamarense were isolated. JRT testing gave positive/negative results consistent with those obtained by LC-MS/MS for all A. tamarense cultures tested by both methods. Although toxin profiles were similar, a large variation in the concentration of individual toxin analogues and overall toxicity was observed (; , ). Toxin analogues were dominated by C2 and NEO and to a lesser extent, C1 and STX. Toxic Scottish and Irish A. tamarense isolates have shown similar toxin profiles, with high contributions of C2, NEO and STX (Touzet et al., Citation2008; Collins et al., Citation2009). Cultures for PSP toxin analysis were sampled once they had achieved a high cell density. It is not known if variation in toxin profiles between isolates is due to variation between individual strains or an artefact of culture conditions. Without molecular analysis it is not possible to determine if the A. tamarense isolates belong to Group I and Group III strains, however previous studies have shown PSP toxin production to be restricted to Group I strains while Group III strains have not demonstrated toxin production under the laboratory culture conditions in which they were grown (Scholin et al., Citation1994; Lilly et al., Citation2007; Collins et al., Citation2009). Analysis of the LSU rDNA of Scottish A. tamarense Groups I and III also places them with other European strains (Collins et al., Citation2009). This study confirms a more widespread distribution of toxin-producing A. tamarense in Scottish waters and provides the first record of PSP-producing species from sea lochs along the northwest Scottish coast (Lochs Ainort, Ewe and Duich).
This study confirms A. ostenfeldii as an additional PSP producer in Scottish waters. Although the JRT gave consistent results with LC-MS/MS analysis for A. tamarense, it failed to detect PSP toxins in the A. ostenfeldii isolate that were detected by LC-MS/MS. This is most likely due to the very small toxin analogue concentrations found in the A. ostenfeldii extract compared with the A. tamarense extracts (). It is also confirmed to produce spirolide toxins (, , ). Spirolide and PSP toxin production by A. ostenfeldii appear to be highly variable throughout different geographic regions. Strains from Danish waters and a strain isolated from the south coast in England have also been observed to produce low amounts of both groups of toxins (Hansen et al., Citation1992; Percy, Citation2006). In contrast, Touzet et al. (Citation2008) report on spirolide production by isolates from Irish waters, but did not find evidence for the production of PSP toxins. Interestingly, PSP toxin production has been detected in strains isolated from the Åland Archipelago on the south-west coast of Finland, however no spirolide production was observed (Kremp et al., Citation2009). Low concentrations of NEO (55 fg cell−1) and STX (16 fg cell−1) were detected in A. ostenfeldii exponentially growing cells in batch culture compared with concentrations produced by A. tamarense (). The concentration of NEO determined was just above the limit of detection of the LC-MS/MS instrumentation (Turrell et al., Citation2009) and not detected using the JRT kit. The production of 20-methyl spirolide G by the A. ostenfeldii culture correlates with recent findings by Krock et al. (Citation2008), who detected 20-methyl spirolide G and to a lesser extent 13-desmethyl-spirolide C in North Sea plankton field samples. Spirolides A, B, C, D and G identified as part of the toxin profile of Danish, Canadian and Irish A. ostenfeldii strains were not detected in this Scottish isolate (S06/013/01).
Molecular analysis using the LSU region of A. ostenfeldii showed Scottish strains to cluster with European strains from Denmark and Ireland (). In contrast, Kremp et al. (Citation2009) have demonstrated that English strains appear to be more closely related to A. ostenfeldii isolates from the Baltic and to A. peruvianum isolates from the Mediterranean.
The presence of a ventral pore on the first apical plate of the A. minutum cultures confirms the presence of two morphotypes of this species in Scottish waters. Alexandrium minutum without a ventral pore was reported from field material from the Western Isles (Hansen et al., Citation2003). This feature has been recorded as variable throughout isolates investigated from the Fleet Lagoon, on the south coast of England (Nascimento et al., Citation2005). Molecular analysis showed A. minutum to fall into the global clade containing strains from France, Spain, Italy, Ireland and Denmark (). PSP toxin production was not observed in the A. minutum cultures examined using JRT or LC-MS/MS. This is in contrast to toxic A. minutum detected in the Fal Estuary on the south coast of England (Percy, Citation2006). The culture conditions used during this study were optimized to ensure healthy growth of the cultures and the generation of sufficient biomass for toxin testing. Cultures were therefore not subject to nutrient stresses such as phosphate limitation which has been indicated as an inducer of PSP toxin production in Alexandrium (Anderson et al., Citation1990; Flynn, Citation2002). To date there are very few records of non-toxic A. minutum. Isolates not observed to produce toxins have been reported from the Gulf of Trieste in the Adriatic Sea (Tillmann & John, Citation2002) and from Cork harbour on the south coast of Ireland (Touzet et al., Citation2008). As these Scottish isolates were shown to belong to the global clade which includes toxin-producing isolates from other locations in Europe, it is evident that LSU sequencing is not sufficient to discriminate between toxin- and non toxin-producing strains. This is supported by Touzet et al. (Citation2007).
This study reports the most northerly observation of the recently described A. tamutum (Montresor et al., Citation2004) in European waters. Cells identified in this study provide an addition to the small number of reports of the species (Figueroa et al., Citation2007; Alpermann et al., Citation2008; Touzet et al., Citation2008). LSU analyses cluster the Scottish isolates with the Italian and Irish isolates previously examined by Montresor et al. (Citation2004). This species was not observed to produce PSP toxins under the culture conditions used, which correlates with toxin analyses by Montresor et al. (Citation2004) and Touzet et al. (Citation2008).
Considerable variation has been observed in the abundance of Alexandrium cells in the water column and in PSP toxicity in Scottish shellfish (Bresnan et al., Citation2008). Previous analysis of cell counts from a site on the east coast revealed considerable interannual differences in the cell density and timing of Alexandrium maximal abundance from year to year (Bresnan et al., Citation2009). Data from long-term Alexandrium time series from other regions show trends in cell abundance and toxicity on a decadal scale (Wyatt & Jenkinson, Citation1997; Anderson et al., Citation2005; Martin et al., Citation2009), however the physical environment of the coastal waters around Scotland including the North Sea is changing (Beaugrand et al., Citation2002; Edwards et al., Citation2002; Hughes, Citation2007). Improved monitoring of Alexandrium using the molecular methods required to identify cells to species/strain level will help provide the necessary data to understand if temporal and spatial variation in cell numbers and variation in PSP toxin concentrations are a result of natural variation on a decadal scale or due to changes in species composition as a result of natural range expansion, climate change or introductions.
This is the first comprehensive study on the distribution, diversity and toxin composition of the genus Alexandrium in Scottish waters. It highlights a wide geographical distribution of Alexandrium cysts and vegetative cells and identifies the diversity of both toxic and non-toxic Alexandrium species present. The co-occurrence of both toxin-producing and potentially non toxin-producing Alexandrium in the water column has implications for using phytoplankton counts to predict the occurrence of PSP events. Implementation of molecular methods such as whole-cell fluorescent in situ hybridization (FISH) probes (John et al., Citation2005; Touzet & Raine, Citation2007) or quantitative polymerase chain reaction (PCR)-based assays (Penna et al., Citation2007) will allow a more accurate assessment of the potential for PSP intoxication of shellfish.
Acknowledgements
The authors would like to thank the crews and staff of FRV Scotia and Clupea for collection of sediment samples and volunteers in the ecosystem monitoring programme for collection of water samples. Thanks to Professor Jane Lewis (University of Westminster, London) for her historic cyst data, Elspeth MacDonald and Russell Davidson (previous MS-S staff, Scotland) for their cyst analysis, Dr Nicolas Touzet (National University of Ireland, Galway) for advice/provision of sequence data and Professor Barrie Dale (University of Oslo, Norway) for advice on cyst methodologies and identification. This work was performed under the Scottish Government Marine Directorate ROAME AE1193, Schedule of Service AE11p and AE11q, Scottish Government and Food Standard Agency Toxic Phytoplankton Monitoring programmes (1996–2005) and the Sixth Framework Programme of the European Community (Collective Research Project SPIES-DETOX 0302790-2). The authors appreciate the input from three anonymous reviewers whose comments improved this manuscript.
References
- Aasen , J , Mackinnon , SL , Leblanc , P , Walter , JA , Hovgaard , P , Aune , T and Quilliam , MA . 2005 . Detection and identification of spirolides in Norwegian shellfish and plankton . Chem. Res. Toxicol. , 18 : 509 – 515 .
- ALPERMANN, T.J., BESZTERI, B., TILLMANN, U., CEMBELLA, A.D. & JOHN, U. (2008). Species discrimination in the genus Alexandrium by amplified fragment length polymorphism. In Proceedings of the 12th International Conference on Harmful Algae (Moestrup, Ø., Doucette, G., Enevoldson, H., Godhe, A., Hallegraeff, G., Luckas, B., Lundholm, N., Lewis, J., Rengefors, K., Sellner, K., Steidinger, K., Tester, P., Zingone, A., editors), 51–54. Intergovernmental Oceanographic Commission of UNESCO
- Anderson , DM , Kulis , DM , Sullivan , JJ , Hall , S and Lee , C . 1990 . Dynamics and physiology of saxitoxin production by the dinoflagellates Alexandrium spp . Mar. Biol. , 104 : 511 – 524 .
- Anderson , DM , Stock , CA , Keafer , BA , Bronzino Nelson , A , Thompson , B , Mcgillicuddy , Jr, DJ , Keller , M , Matrai , PA and Martin , J . 2005 . Alexandrium fundyense cyst dynamics in the Gulf of Maine . Deep-Sea Res. II. , 52 : 2522 – 2542 .
- AYRES, P.A. & CULLUM, M. (1978). Paralytic shellfish poisoning: an account of investigations into mussel toxicity 1968–77. Fisheries Research Technical Report, MAFF Directorate of Fisheries Research, Lowestoft
- BALECH, E. (1995). The genus Alexandrium Halim (Dinoflagellata). Sherkin Island Marine Station, Sherkin Island
- Beaugrand , G , Reid , PC , Ibanez , F , Lindley , A and Edwards , M . 2002 . Reorganization of North Atlantic marine copepod biodiversity and climate . Science , 296 : 1692 – 1694 .
- BRESNAN, E., FRYER, R., HART, M. & PERCY, P. (2005). Correlation between algal presence in water and toxin presence in shellfish. FRS Contract Report 04/05 (http://www.frs-scotland.gov.uk/Delivery/Information_resources/information_resources_view_document.aspx?resourceId=31137&documentId=2829)
- Bresnan , E , Hay , S , Hughes , SL , Fraser , S , Rasmussen , J , Webster , L , Slesser , G , Dunn , J and Heath , MR . 2009 . Seasonal and interannual variation in the phytoplankton community in the north east of Scotland . J. Sea Res. , 61 : 17 – 25 .
- BRESNAN, E., TURRELL, E. & FRASER, S. (2008). Monitoring PSP and Alexandrium hotspots in Scottish waters. In Proceedings of the 12th International Conference on Harmful Algae (Moestrup, Ø., Doucette, G., Enevoldson, H., Godhe, A., Hallegraeff, G., Luckas, B., Lundholm, N., Lewis, J., Rengefors, K., Sellner, K., Steidinger, K., Tester, P., Zingone, A., editors), 76–79. Intergovernmental Oceanographic Commission of UNESCO
- Brown , J , Fernand , L , Horsburgh , KJ , Hill , AE and Read , JW . 2001 . Paralytic shellfish poisoning on the east coast of the UK in relation to seasonal density-driven circulation . J. Plankton Res. , 23 : 105 – 116 .
- Cembella , AD , Lewis , NI and Quilliam , MA . 1999 . Spirolide composition in micro-extracted pooled cells isolated from natural plankton assemblages and from cultures of the dinoflagellate Alexandrium ostenfeldii . Nat. Tox. , 8 : 1 – 10 .
- Collins , C , Graham , J , Brown , L , Bresnan , E , Lacaze , J-P and Turrell , EA . 2009 . Identification and toxicity of Alexandrium tamarense (Dinophyceae) in Scottish waters . J. Phycol. , 45 : 692 – 703 .
- Edwards , M , Beaugrand , G , Reid , PC , Rowden , AA and Jones , MB . 2002 . Ocean climate anomalies and the ecology of the North Sea . Mar. Ecol. Prog. Ser. , 239 : 1 – 10 .
- Figueroa , RI , Garcés , E and Bravo , I . 2007 . Comparative study of the life cycles of Alexandrium tamutum and Alexandrium minutum (Gonyaulacales, Dinophyceae) in culture . J. Phycol. , 43 : 1039 – 1053 .
- Flynn , KJ . 2002 . Toxin production in migrating dinoflagellates: a modelling study of PSP producing Alexandrium . Harmful Algae , 1 : 147 – 155 .
- Fritz , L and Triemer , RE . 1985 . A rapid simple technique utilizing calcofluor white M2R for the visualization of dinoflagellate thecal plates . J. Phycol. , 21 : 662 – 664 .
- Gayoso , A and Fulco , VK . 2006 . Occurrence patterns of Alexandrium tamarense (Lebour) Balech populations in the Gulfo Nuevo (Patagonia, Argentina), with observations on ventral pore occurrence in natural and cultured cells . Harmful Algae , 5 : 233 – 241 .
- Gribble , KE , Keafer , BA , Quilliam , MA , Cembella , AD , Kulis , DM , Manahan , A and Anderson , DM . 2005 . Distribution and toxicity of Alexandrium ostenfeldii (Dinophyceae) in the Gulf of Maine, USA . Deep-Sea Res. II. , 52 : 2745 – 2763 .
- Guillard , RRL . 1995 . “ Culture methods ” . In Manual on Harmful Marine Microalgae , Edited by: Hallegaeff , GM , Anderson , DM and Cembella , AD . 45 – 62 . Intergovernmental Oceanographic Commission of UNESCO .
- Hall , TA . 1999 . BioEdit: a user-friendly biological sequence alignment editor and analysis program for Windows 95/98/NT . Nucleic Acids Symp Ser. , 41 : 95 – 98 .
- Hansen , PJ , Cembella , AD and Moestrup , Ø . 1992 . The marine dinoflagellate Alexandrium ostenfeldii: Paralytic shellfish toxin concentration, composition and toxicity to a tintinnid ciliate . J. Phycol. , 28 : 597 – 603 .
- Hansen , G , Daugbjerg , N and Franco , JM . 2003 . Morphology, toxin composition and LSU rDNA phylogeny of Alexandrium minutum (Dinophyceae) from Denmark, with some morphological observations on other European strains . Harmful Algae , 2 : 317 – 335 .
- Higman , WA , Stone , DM and Lewis , JM . 2001 . Sequence comparisons of toxic and non-toxic Alexandrium tamarense (Dinophyceae) isolates from UK waters . Phycologia , 40 : 256 – 262 .
- HUGHES, S. L. (2007). Scottish Ocean Climate Status Report 2004 and 2005. Fisheries Research services, Aberdeen (http://www.frs-scotland.gov.uk/Delivery/Information_Resources/information_resources_view_document.aspx?contentid=2174)
- John , U , Cembella , A , Hummert , C , Elbrachter , M , Groben , R and Medlin , L . 2003 . Discrimination of the toxigenic dinoflagellates Alexandrium tamarense and A. ostenfeldii in co-occurring natural populations from Scottish coastal waters . Eur. J. Phycol. , 38 : 25 – 40 .
- John , U , Medlin , LK and Groben , R . 2005 . Development of specific rRNA probes to distinguish between geographic clades of the Alexandrium tamarense species complex . J. Plankton Res. , 27 : 199 – 204 .
- Joint , I , Lewis , J , Aitken , J , Proctor , R , Moore , G , Higman , W and Donald , M . 1997 . Interannual variability of PSP outbreaks on the north east UK coast . J. Plankton Res. , 19 : 937 – 956 .
- JOYCE, L. B. (2001). An investigation into the occurence of harmful algal blooms in Scapa Flow, Orkney. PhD, Heriot-Watt University, UK
- Kremp , A , Lindholm , T , Dreßler , N , Erler , K , Gerdts , G , Eirtovaara , S and Leskinen , E . 2009 . Bloom forming Alexandrium ostenfeldii (Dinophyceae) in shallow waters of the Åland Archipelago, Northern Baltic Sea . Harmful Algae , 8 : 318 – 328 .
- Krock , B , Tillman , U , John , W and Cembella , A . 2008 . LC-MS-MS aboard ship: tandem mass spectrometry in the search for phycotoxins and novel toxigenic plankton from the North Sea . Anal. Bioanal. Chem. , 392 : 797 – 803 .
- Laycock , MV , Donovan , M and Easy , DJ . 2010 . Sensitivity of lateral flow tests to mixes of saxitoxins and applications to shellfish and phytoplankton monitoring . Toxicon , 55 : 597 – 605 .
- Lewis , J , Higman , W and Kuenstner , S . 1995 . “ Occurrence of Alexandrium sp. cysts in sediments from the north east coast of Britain ” . In Harmful Marine Algal Blooms , Edited by: Lassus , P , Arzul , G , Erard , E , Gentien , P and Marcaillou , C . 175 – 180 . Paris : Lavoisier .
- Lilly , EL , Halanych , KM and Anderson , DM . 2005 . Phylogeny, biogeography and species boundaries within the Alexandrium minutum group . Harmful Algae , 4 : 1004 – 1020 .
- Lilly , EL , Halanych , KM and Anderson , DM . 2007 . Species boundaries and global biogeography of the Alexandrium tamarense complex (Dinophyceae) . J. Phycol. , 43 : 1329 – 1338 .
- Lim , PT , Usup , G , Leaw , CP and Ogata , T . 2005 . First report of Alexandrium taylori and Alexandrium peruvianum (Dinophyceae) in Malaysia waters . Harmful Algae , 4 : 391 – 400 .
- Luckas , B , Dahlmann , J , Erler , K , Gerdts , G , Wasmund , N , Hummert , C and Hansen , PD . 2005 . Overview of key phytoplankton toxins and their recent occurrence in the North and Baltic seas . Environ. Toxicol. , 20 : 1 – 17 .
- Martin , JL , Hanke , AR and Legresley , MM . 2009 . Long term monitoring, including harmful algal blooms, in the Bay of Fundy, eastern Canada . J. Sea Res. , 61 : 76 – 83 .
- Medlin , LK , Lange , ML , Wellbrock , U , Donner , G , Elbrachter , M , Hummert , C and Luckas , B . 1998 . Sequence comparisons link toxic European isolates of Alexandrium tamarense from the Orkney Islands to toxic North America stocks . Eur. J. Protistol. , 34 : 329 – 335 .
- Montresor , M , John , U , Beran , A and Medlin , LK . 2004 . Alexandrium tamutum sp. Nov. (Dinophyceae): a new nontoxic species in the genus Alexandrium . J. Phycol. , 40 : 398 – 411 .
- Nascimento , SM , Purdie , DA , Lilly , EL , Larsen , J and Morris , S . 2005 . Toxin profile, pigment composition, and large subunit rDNA phylogenetic analysis of an Alexandrium minutum (Dinophyceae) strain isolated from the Fleet Lagoon, United Kingdom . J. Phycol. , 41 : 343 – 353 .
- Neale , K , Percy , L and Lewis , J . 2007 . Using sediment to help map Alexandrium distribution in UK coastal waters . Shellfish News , 24 : 20 – 23 .
- Orlova , TY , Selina , MS , Lilly , EL , Kulis , DM and Anderson , DM . 2007 . Morphogenetic and toxin composition variability of Alexandrium tamarense (Dinophyceae) from the east coast of Russia . Phycologia , 46 : 534 – 548 .
- Penna , A , Bertozzini , E , Battocchi , C , Galluzzi , L , Grazia Giacobbe , M , Villa , M , Garces , E , Luglie , A and Magnani , M . 2007 . Monitoring of HAB species in the Mediterranean Sea through molecular methods . J. Plankton Res. , 29 : 19 – 38 .
- PERCY, L.A. (2006). An investigation of the phytoplankton of the Fal Estuary, UK and the relationship between the occurrence of potentially toxic species and associated algal toxins in shellfish. PhD, University of Westminster, UK
- Posada , D and Crandall , KA . 1998 . Testing the model of DNA substitution . Bioinformatics , 14 : 817 – 818 .
- Scholin , CA , Herzog , M , Sogin , M and Anderson , DM . 1994 . Identification of group- and strain-specific genetic markers for globally distributed Alexandrium (Dinophyceae). II. Sequence analysis of a fragment of the LSU rRNA gene . J. Phycol. , 30 : 999 – 1011 .
- Steidinger , KA and Tangen , K . 1997 . “ Dinoflagellates ” . In Identifying Marine Phytoplankton , Edited by: Tomas , CR . 387 – 584 . San Diego : Academic Press .
- STOBO, L., GALLACHER, S., LEWIS, J., QUILLIAM, M. & SMITH, E. (2006). Development of methods for and survey of recently discovered toxins in UK shellfish. FRS Contract Report No. 06/06. (http://www.frs-scotland.gov.uk/Delivery/Information_resources/information_resources_view_document.aspx?resourceId=31137&documentId=2846)
- Stobo , L , Lacaze , J-P , Scott , A , Petrie , J and Turrell , EA . 2008 . Surveillance of algal toxins in shellfish from Scottish waters . Toxicon , 51 : 635 – 648 .
- SWAN, S.C. & DAVIDSON, K. (2008). Monitoring programme for the presence of toxin producing plankton in shellfish production areas in Scotland. Contract Reference SO1007/PAU179A. Food Standards Agency Scotland
- SWOFFORD, D.L. (1999). PAUP*: Phylogenetic Analysis using Parsimony (*and Other Methods). Version 4.0. Sinauer Associates, Sunderland, MA
- Taylor , FJR , Fukuyo , Y , Larsen , J and Hallegraeff , GM . 2003 . “ Taxonomy of harmful dinoflagellates ” . In Manual on Harmful Marine Microalgae , Edited by: Hallegraef , GM , Anderson , DM and Cembella , AD . 389 – 432 . Paris : UNESCO Publishing .
- Tillmann , U and John , U . 2002 . Toxic effects of Alexandrium spp. on heterotrophic dinoflagellates: an allelochemical defence mechanism independent of PSP-toxin content . Mar. Ecol. Prog. Ser. , 230 : 47 – 58 .
- Touzet , N , Franco , JM and Raine , R . 2007 . Characterization of nontoxic, toxin-producing strains of Alexandrium minutum (Dinophyceae) in Irish coastal waters . App. Environ. Microbiol. , 73 : 3333 – 3342 .
- Touzet , N , Franco , JM and Raine , R . 2008 . Morphogenetic diversity and biotoxin composition of Alexandrium (Dinophyceae) in Irish coastal waters . Harmful Algae , 7 : 782 – 797 .
- Touzet , N and Raine , R . 2007 . Discrimination of Alexandrium andersoni and A. minutum (Dinophyceae) using LSU rDNA-targeted oligonucleotide probes and fluorescent whole-cell hybridization . Phycologia , 46 : 168 – 177 .
- Turrell , E , Stobo , L , Lacaze , J-P , Piletsky , S and Piletska , E . 2009 . Optimisation of hydrophilic interaction liquid chromatography-mass spectrometry and development of solid phase extraction for the analysis of paralytic shellfish poisoning toxins . J. AOAC Int. , 91 : 1372 – 1386 .
- Wang , DZ , Lin , L , Gu , HF , Chan , LL and Hong , HS . 2008 . Comparative studies on morphology, ITS sequence and protein profile of Alexandrium tamarense and A. catenella isolated from the China Sea . Harmful Algae , 7 : 106 – 113 .
- Wyatt , T and Jenkinson , IR . 1997 . Notes on Alexandrium population dynamics . J. Plankton Res. , 19 : 551 – 575 .
- Wyatt , T and Saborido-Rey , F . 1993 . “ Biogeography and time-series analysis of British PSP records, 1968 to 1990 ” . In Toxic Phytoplankton Blooms in the Sea , Edited by: Smayda , TJ and Shimizu , Y . 73 – 78 . Amsterdam : Elsevier Publishing .
- Zhang , Z , Schwartz , S , Wagner , L and Miller , W . 2000 . A greedy algorithm for aligning DNA sequences . J. Comput. Biol. , 7 : 203 – 14 .