Abstract
This work reports the characterization of a unicellular cyanobacterium (LEGE 06123) isolated from an intertidal diazotrophic mat at Luz, a rocky beach in southern Portugal. This organism aggregates to form colonies held together by extracellular polymeric substances (EPS). Cells or cell groups are surrounded by a laminated sheath, and division occurs by binary fission, often in irregular planes. Dispersion is achieved by liberation of daughter cells from the ruptured mother sheath. LEGE 06123 is halotolerant, withstands a considerable range of iron concentrations, and favours growth at moderately high temperatures (35, 40°C). According to the criteria defined in 1998 by Komárek and Anagnostidis, LEGE 06123 could belong to the species Gloeocapsopsis crepidinum (Thuret) Geitler ex Komárek 1993. However, our phylogenetic analyses clearly show that LEGE 06123 is closely related to three cyanobacteria isolated from the Great Yellowstone and Zerka Ma’in hot springs, one of them (5.2 s.c.1) being described as a new genus and a new species – Chroogloeocystis siderophila Brown, Mummey et Cooksey. The polyphasic characterization performed here has exposed the unusual features of LEGE 06123, which along with the three thermophilic strains, forms a distinct clade deserving further investigation.
Introduction
Cyanobacteria are ubiquitous photosynthetic prokaryotes that perform oxygenic photosynthesis, a process that has radically changed the atmosphere and living conditions on Earth. This group of microorganisms has evolved by fast radiation; it is believed that they originally developed from mats and stromatolites in coastal oceanic ecosystems, and then gradually dispersed and colonized new environments (Nisbet & Sleep, Citation2001; Cavalier-Smith, Citation2006; Tomitani et al., Citation2006; Shi & Falkowski, Citation2008). During this evolution, a complexity and variety of morphologies as well as ecophysiological properties arose, turning cyanobacteria into the most diverse group of prokaryotes (Shi & Falkowski, Citation2008). They occupy a wide spectrum of environments, including extreme ecosystems such as hot springs, hypersaline lakes and deserts (Potts, Citation1999; Miller & Castenholz, Citation2000; Nübel et al., Citation2000). Cyanobacteria play a major role in the global carbon cycle as important primary producers and diazotrophic taxa are fundamental to the nitrogen cycle, particularly in oceans (Knoll, Citation2008). Many marine studies have focused on picocyanobacteria, a major component in the planktonic community of the open oceans (Waleron et al., Citation2007; Zwirglmaier et al., Citation2008). Nevertheless, some near-shore and coastal studies, mainly in tropical or subtropical areas, have provided information on the ecology and diversity of cyanobacteria in these ecosystems (Hoffmann, Citation1999; Abed et al., Citation2007; Díez et al., Citation2007; Ohki et al., Citation2008). In marine coastal regions, benthic cyanobacteria grow along the shore, mainly in the intertidal zone, forming cohesive mats. In these habitats, cyanobacteria are exposed to a range of daily stresses such as nutrient limitation, high UV-radiation and desiccation, as well as temperature and salinity fluctuations (Abed et al., Citation2007; Díez et al., Citation2007). A thorough identification of the taxa present in cyanobacterial mats is required to properly understand their structure and composition. Historically, the identification of cyanobacteria has been based mainly on morphological characteristics, but over the last few decades modern ecological investigations, electron microscopy, and particularly the advent of molecular methods, have substantially influenced our views on the taxonomy of these prokaryotes (Castenholz, Citation2001; Hoffmann et al., Citation2005). However, the classification of cyanobacteria and its revision are complex tasks due to the existence of numerous species exclusively defined by morphology, as well as many cyanobacterial gene sequences without a corresponding morphological description (Komárek & Anagnostidis, Citation1998; Wilmotte & Herdman, Citation2001; Oren & Tindall, Citation2005). Therefore, a polyphasic characterization merging the results of molecular analysis, cytomorphological and ecological evaluation is preferred, and the isolation of the organism into culture is also recommended (Nichols, Citation2007; Garcia-Pichel, Citation2008).
In this study, a unicellular cyanobacterium (LEGE 06123) collected from a diazotrophic mat in the intertidal zone of the rocky beach at Luz (southern Portugal) was isolated, and a polyphasic approach was used to characterize it. This organism belongs to the species Gloeocapsopsis crepidinum (Thuret) Geitler ex Komárek, according to botanical classification. However, phylogenetically, LEGE 06123 is closely related to three unicellular thermophilic cyanobacteria, one of which has been proposed as the type of a new genus based on molecular data – Chroogloeocystis siderophila Brown, Mummey et Cooksey. The work presented here will contribute to clarifying the taxonomy of this distinct clade of cyanobacteria.
Materials and methods
Site and habitat description
The Algarve coast is in the southernmost region of mainland Portugal, which has a Mediterranean climate (according to the Köppen classification, see Kottek et al., Citation2006), with an annual rainfall average of 452.6 mm and a mean annual air temperature of 17.9°C. Seawater temperature ranges from 21°C to 24°C in the summer, while in winter it is 15–19°C. The sampling site is located in the upper region of the intertidal zone of the rocky beach at Luz (37°05′04″N, 8°44′01″W), which is in a coastal plain outcrop known as ‘Luz sandstone’ formed in the Early Cretaceous (Rey, Citation1983). Seawater samples for physical and chemical analyses () were collected near-shore, and from puddles in the intertidal zone (where the cyanobacterium was found), transported and processed according to the standards required by the national reference laboratory, Instituto Nacional Ricardo Jorge, where the physicochemical parameters were measured ().
Table 1. Physico-chemical parameters of water samples from inshore and intertidal pools at Luz beach, Portugal.
Sampling and isolation
Samples were collected in December 2006, and a subsequent survey was carried out in May 2008, confirming the presence of the cyanobacterium described in this work. Sampling was performed by scraping the surface of green seaweeds and by collecting dark mats in shallow puddles that retain seawater during low tide. The isolation procedure included a liquid culture enrichment of the biological sample in MN medium (Kotai, Citation1972; Rippka, Citation1988), followed by streaking the cells on agar medium plates, and transferring single cells or colonies back into liquid medium using a Pasteur pipette (Rippka, Citation1988). Isolate 06123 is deposited at LEGE (Laboratório de Ecotoxicologia, Genómica e Evolução, CIIMAR, Porto), and the Culture Collection of Algae and Protozoa (CCAP 1425/1).
Morphological and ultrastructural characterization
Mats and isolated cells were observed using a Leica DMLB microscope (Leica Microsystems GmbH, Wetzlar, Germany). Images were captured with a Leica ICCA Camera System, and processed using Qwin Leica software (Leica Microsystems GmbH, Wetzlar, Germany). Cell dimensions were determined using the same software. For transmission electron microscopy studies, cells (from a 10-day old culture) were collected by centrifugation and processed as described by Seabra et al. (Citation2009). Sections were examined using a Zeiss EM C10 electron microscope operating at 80 kV. Morphological identification was performed following the criteria of Komárek & Anagnostidis (Citation1998), and by using Bergey's Manual of Systematic Bacteriology (Castenholz, Citation2001).
Growth experiments
For the growth experiments, LEGE 06123 cells were cultured in the artificial seawater defined medium ASNIII (Rippka, Citation1988), buffered with 10 mM HEPES (pH = 8.2), under continuous illumination (15 µmol m−2 s−1). Batch cultures were subjected to different temperatures (20–50°C), salinities (0–100‰ NaCl) and iron concentrations (0–400 µM FeCl3 · 6H2O). Growth was monitored by measuring the OD at 730 nm, with three replicates per treatment. Salinity and iron concentration experiments were performed at 25°C. The capacity of LEGE 06123 to fix nitrogen was evaluated by growing the cells in ASNIII with gradually reduced concentrations of nitrate down to zero (ASNIII0).
Molecular characterization
Cells were harvested by centrifugation and DNA was extracted using the Maxwell® 16 System (Promega Corporation, Madison, WI, USA), and the Cell DNA Purification Kit for Gram- bacteria (Promega Corporation, Madison, WI, USA) according to the instructions of the manufacturer. The following intra- or intergenic regions totalling 4405 bp were amplified using the oligonucleotide primers listed in : most of the 16S rRNA (small subunit ribosomal) gene, the internal transcribed spacer (ITS), and most of the 23S rRNA (large subunit ribosomal) gene; 600 bp within the rpoB (RNA polymerase β subunit); 658 bp comprising part of the two bilin subunit genes cpcB and cpcA, and the intergenic spacer (IGS); 1129 bp within nifK (dinitrogenase β subunit) and 353 bp within nifH (dinitrogenase reductase). PCR was carried out in a MyCycler™ thermal cycler (Bio-Rad laboratories Inc., Hercules, CA, USA) using the methodology described previously (Tamagnini et al., Citation1997). The profiles were: 94°C for 5 min, followed by 35 cycles of 94°C for 1 min, 50 or 60°C (for rRNA ITS: ITSc) for 1 min, 72°C for 1–1.25 min, and a final extension of 72°C for 7 min. For amplification of nifH the reagents and conditions were as described by Omoregie et al. (Citation2004).
Table 2. Target genes/sequences and oligonucleotide primers used in this study.
Agarose gel electrophoresis was performed with standard protocols using 1 × TAE buffer (Sambrook & Russell, Citation2001) and the DNA fragments were isolated from gels using the GFX™ PCR DNA and Gel Band Purification Kit (GE Healthcare, Buckinghamshire, UK). Purified PCR products were cloned into pGEM®-T Easy vector (Promega, Madison, WI, USA), and transformed into Escherichia coli DH5α competent cells following the instructions of the manufacturer. Colonies were screened for the presence of the insert by colony PCR, and subsequently grown overnight, in liquid LB medium supplemented with 100 µg ml−1 of ampicillin, at 37°C with shaking. Plasmid DNA was isolated from E. coli cultures using the GenElute™ Plasmid Miniprep Kit (Sigma-Aldrich, Saint Louis, MO, USA), and the insert was sequenced at STAB Vida (Lisbon, Portugal).
Novel sequences associated with this study were deposited in GenBank (Accession numbers: FJ589716, FJ536846, FJ536847, FJ536848, GU597364).
Phylogenetic analysis
To assess the position of the new strain LEGE 06123 within the cyanobacteria, three different methods were employed to reconstruct tree topologies: Neighbour-Joining (Saitou & Nei, Citation1987), Maximum Likelihood (Felsenstein, Citation1981) and Maximum Parsimony (Kluge & Farris, Citation1969; Fitch, Citation1971). The 16S rRNA gene sequence from LEGE 06123 was compared with the NCBI database (in November 2009), and the ten closest sequences (ordered by their e-value) were retrieved. In order to include full-length sequences and to achieve a reliable representation of cyanobacterial diversity, 77 extra sequences from reference strains (Castenholz, Citation2001) were retrieved from GenBank and/or from the quality-controlled sequence database RDP-II (Cole et al., Citation2007). The Chloroflexus aurantiacus J-10-fl 16S rRNA gene sequence (D38365.1) was included as outgroup. A multiple alignment of the sequences mentioned above was performed with ClustalX2 (Thompson et al., Citation1997), using the default parameters, and visually inspected. The same software was used to compute the NJ tree, for which the robustness of the nodes was assessed by 10 000 bootstrap resamplings. MP and ML trees were constructed using the PHYLIP 3.68 package (Felsenstein, Citation1989). For the MP analysis, a thorough search was chosen and up to 10 000 trees saved for future inspection. The ML procedure was applied with a transition/transversion ratio of 2, with or without global rearrangements. All trees were visualized by NJPLOT (Perrière & Gouy, Citation1996).
Results
Habitat, morphology, ultrastructure and identification of the isolate
The cyanobacterium isolated was present on the surface of green seaweeds as well as in mats growing in shallow intertidal puddles which receive an input of freshwater from surrounding groundwater (). The smooth dark mats in which LEGE 06123 was found were dominated by a filamentous heterocystous cyanobacterium, probably Calothrix sp., and were widespread in the bases of puddles along Luz beach (). The filamentous cyanobacteria form a dense entangled mass in which unicellular organisms (notably LEGE 06123) and particles are trapped. LEGE 06123 is a euryhaline organism, as can be seen from the differences in salinity of two of the puddles in which this organism was found (see ). It can occur in subaerophytic or immersed environments, mainly on rocky substrates, but also growing epiphytically on seaweeds.
Figs 1–8. Sampling site and samples of the colonial cyanobacterium LEGE 06123. Figs 1, 2. The sampling site at Luz beach, showing location in southern Portugal (arrowhead). . Unicellular cyanobacterium (arrowhead) in mat collected from shallow puddle. . Isolate LEGE 06123 showing sub-spherical cells which divide irregularly in various planes with dispersion through disintegration of colonies after intensive cell division (arrow). . Firm sheath (arrow) and amorphous polysaccharide layer (arrowhead) surround cells stained with Alcian blue. . Ultrastructure (TEM) showing the laminated nature of the sheath and the concentric arrangement of thylakoids which can exhibit invaginations towards the centre of the cell (sh, sheath, th, thylakoids). Scale bars: 10 µm (light micrographs); 1 µm (TEM).
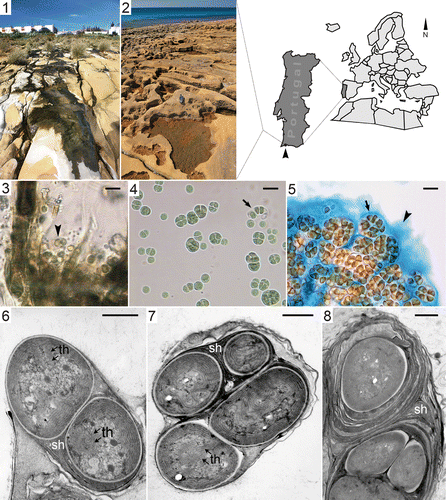
To identify LEGE 06123, we took into account its morphological characteristics and ecological properties. This isolate is a unicellular cyanobacterium that aggregates to form colonies held together by extracellular polymeric substances (EPS). The cells exhibit different shapes (ranging from spherical to polyhedral) and sizes depending on the developmental stage (see ). Each cell is surrounded by a firm, often stratified, colourless sheath with an average thickness of about 0.6 µm (), as well as an abundant amorphous layer of slime, which is visible when stained with Alcian blue (, arrowhead). The TEM images revealed the laminated nature of the sheath (Figs ). Light and TEM micrographs indicate that cells divide by binary fission, often in irregular planes, and do not necessarily grow into the original shape and size before the next fission. Dispersion is achieved by liberation of daughter cells from the ruptured mother sheath (, arrow). Electron micrographs also show that the thylakoids are arranged parallel to one another and to the cell wall (), and occasionally convoluted towards the centre of the cell () where DNA fibrils and cell inclusions can be observed ().
Table 3. Morphometric measurements of cyanobacterial strain LEGE 06123.a
Based on the descriptions of Komárek & Anagnostidis (Citation1998) and following their identification criteria, LEGE 06123 could be assigned to the type species Gloeocapsopsis crepidinum (Thuret) Geitler ex Komárek 1993. In Bergey's Manual of Systematic Bacteriology (Castenholz, Citation2001) there is no corresponding taxon to which this cyanobacterium could be assigned.
We were successful in sequencing the 16S rRNA gene, the internal transcribed spacer (ITS) between the 16S and the 23S rRNA genes, the 23S rRNA gene, the rpoB, the region between the cpcB and cpcA (including the intergenic spacer – IGS), and nifH and nifK. However, due to limited information available in databases, the only informative locus to corroborate the morphological identification of this organism is, at the moment, the 16S rRNA gene. Comparison of our sequence with GenBank showed that LEGE 06123 exhibits highest similarities (97%) with thermophilic cyanobacteria (AY380791.1, DQ471449.1, DQ471448.1), being particularly close to an isolate displaying a high tolerance for iron (Brown et al., Citation2005).
Culture growth experiments
In general, the 75 ml batch cultures reached stationary phase about 1 month after the beginning of the experiment, with a long lag phase of about 10–12 days (Figs ). Although this cyanobacterium was isolated from a beach in which the average sea temperature is 17°C in the winter and 22.5°C in the summer, it exhibited faster growth at higher temperatures (35°C and 40°C, see ). At 50°C LEGE 06123 did not survive, at least in an experiment without acclimation.
Fig. 11. Growth curves of LEGE 06123 in ASNIII medium supplemented with various concentrations of iron (FeCl3 · 6H2O).
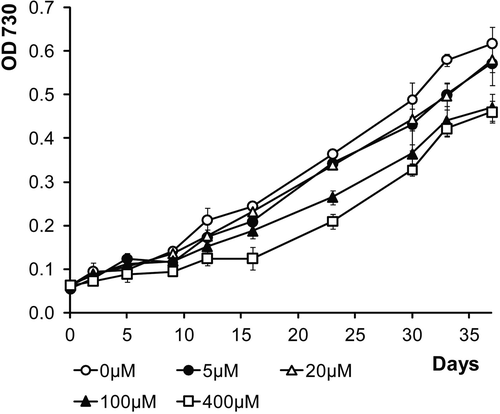
The exclusion of NaCl from the culture medium led to faster growth. With increasing salt concentration growth gradually decreased. At NaCl concentrations of 50‰ and above, the isolate failed to grow () but the cells could recover when re-cultured at lower salinities (data not shown).
LEGE 06123 exhibited better growth without any additional iron (FeCl3 · 6H2O), and growth was negatively affected by the increase in iron concentration (). However, the cultures were able to withstand iron concentrations up to 400 µM, and accumulated considerable amounts of iron in the EPS ().
Fig. 12. Transmission electron micrograph of a 14-day-old culture of the cyanobacterium LEGE 06123 in liquid medium ASNIII supplemented with 400 µM of iron (FeCl3 · 6H2O). The arrow shows the metal accumulation outside the cell. Scale bar: 0.5 µm.
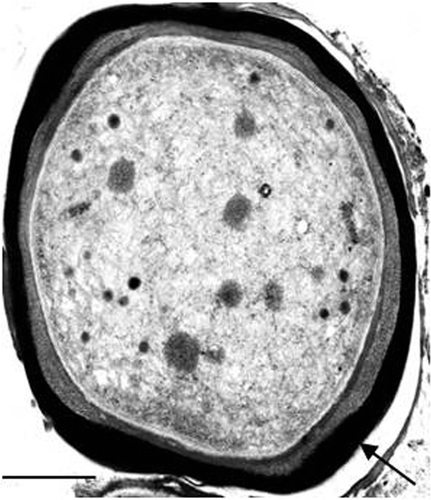
Even though at least two nitrogen fixation genes are present in LEGE 06123 (nifH and nifK), attempts to grow this cyanobacterium under N2-fixing conditions failed. Nevertheless, a small amount of combined nitrogen in the medium (0.1875 g l−1 NaNO3) supported growth.
Phylogenetic analysis
Multiple alignment and phylogenetic reconstructions were performed on the new isolate LEGE 06123. Tree topologies computed using a distance-based method, NJ (), or using a character-based method, ML (data not shown), indicate that LEGE 06123 is closely related to three unicellular thermophilic cyanobacteria, isolated from hot springs at Zerka Ma’in, Jordan (DQ 471449.1 and DQ4714481), and the Great Yellowstone, USA (AY38079.1). The clade was also resolved in all eight equally parsimonious trees (data not shown). This clade of unicellular cyanobacteria is placed within a weakly supported (67.2%) larger clade that includes filamentous heterocystous cyanobacteria.
Fig. 13. Phylogenetic tree of 16S rRNA gene sequences from several cyanobacteria generated by NJ analysis. LEGE 06123 is shown in bold. The tree was rooted with the outgroup Chloroflexus aurantiacus J-10-fl (D38365.1), which was later pruned from the tree. Bootstrap values >50% are indicated beside the nodes. Each taxon is identified by the accession number (brackets) and whenever possible by the name.
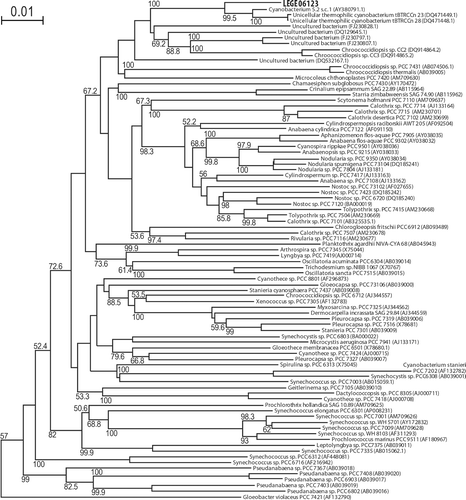
Discussion
Polyphasic characterization of LEGE 06123 has exposed the unusual nature of this cyanobacterium. Its 16S rRNA gene indicates a possible relationship with thermophilic cyanobacteria from two geographically distant hot springs, one from the Great Yellowstone Area, located in the USA (Brown et al., Citation2005), and two from Zerka Ma’in, in Jordan (Ionescu et al., Citation2007). Of these cyanobacteria, isolate 5.2 s.c.1 has also been extensively characterized (see Brown et al., Citation2005), allowing the comparison of its morphological, ecophysiological and ultrastructural characteristics with LEGE 06123. Both are unicellular cyanobacteria, dividing by binary fission, with no baeocyte formation observed. Cells of the two isolates are similar in morphology and size, and a firm sheath surrounds the cell or group of cells (see Figs , and ; Brown et al., Citation2005). Unlike our isolate, 5.2 s.c.1 does not seem to reach a many-celled (more than four) colony stage. Ultrastructural analyses showed that both cyanobacteria exhibit a multilayered sheath and a concentric arrangement of thylakoids (see Figs ; Brown et al., Citation2005), the latter considered to be an important taxonomic character (Komárek & Anagnostidis, Citation1998).
At the temperature range evaluated, LEGE 06123 grows faster at 35°C and 40°C, values considerably higher than the annual average seawater and air temperatures. Nevertheless, it is not uncommon that the optimal growth temperature in laboratory experiments does not coincide with those occurring in their natural environment (e.g. Abed et al., Citation2002; Taton et al., Citation2006). LEGE 06123 failed to grow at 50°C and above, values more likely to occur in hot springs inhabited by the thermophilic cyanobacteria related to LEGE 06123 (Brown et al., Citation2005; Ionescu et al., Citation2007).
LEGE 06123 can be considered a euryhaline organism since it can withstand a wide range of salinities like many other cyanobacteria (Abed et al., Citation2007; Waleron et al., Citation2007). However, it exhibits faster growth in the absence of salt or at low salt concentrations. A freshwater origin for this organism cannot be excluded, since it was isolated from the intertidal zone which has a groundwater input.
LEGE 06123 has low iron requirements, growing better in standard ASNIII medium () but it is capable of tolerating significant amounts of this metal, up to 400 µM FeCl3 · 6H2O. This contrasts with the high necessity for iron of the isolate 5.2 s.c.1 (Brown et al., Citation2005), yet both strains accumulate iron outside the cells through sorption in EPS (). In marine environments, iron is known to be a limiting nutrient (Stal & Zehr, Citation2008), and for this reason, some cyanobacteria often produce Fe-binding siderophores in order to scavenge the metal from seawater (Leão et al., Citation2007). It is also possible that the EPS might act as a reservoir of iron that becomes readily available to the cells whenever necessary. Given the near insolubility of Fe(III) in seawater (Leão et al., Citation2007), and the similarities between these two cyanobacteria, one can argue that LEGE 06123 might have an active role on the sequestration of iron in benthic coastal mats, making it available to other primary producers.
Concerning the capacity to fix N2, LEGE 06123 most probably possesses all the genetic machinery for this process (confirmed by the presence of both nifH and nifK), but it was not possible to grow it in a medium without combined nitrogen. Since LEGE 06123 was isolated from a diazotrophic mat dominated by a heterocystous cyanobacterium, it may have lost the ability to fix nitrogen due to the presence of sufficient combined nitrogen in its environment, as has been reported for cyanobacteria cultured long-term (Bolhuis et al., Citation2010).
The morphological characteristics of LEGE 06123 indicate that it could fit the species Gloeocapsopsis crepidinum (Thuret) Geitler ex Komárek 1993, according to Komárek & Anagnostidis (Citation1998). However, the phylogenetic analysis clearly shows that LEGE 06123 is closely related to the organisms isolated from the Great Yellowstone and Zerka Ma’in hot springs. Brown et al. (Citation2005) proposed that the isolate 5.2 s.c.1 is a new genus and species, named Chroogloeocystis siderophila Brown, Mummey et Cooksey, since, at that time, this cyanobacterium was physiologically and phylogenetically unique – a ‘loner’ sequence (Wilmotte & Herdman, Citation2001) – in the cyanobacterial tree. Our analysis revealed that LEGE 06123 and the three thermophilic strains are placed in a clade quite distant from other reference unicellular cyanobacteria. The closest reference strains are Chroococcidiopsis spp. but they are in a clade without bootstrap support. At present, there is only one 16S rRNA gene sequence available for the genus Gloeocapsopsis, G. crepidinum BDU 20372 (GenBank GU186891), which is quite distant from the clade of organisms discussed here. Moreover, the 16S sequence of G. magma, an aerophytic cyanobacterial colonizer of caves (Roldán & Hernández-Mariné, Citation2008) is also distantly related to our isolate (Hernández-Mariné, personal communication). As stated previously, the nifH sequence could not be used for phylogenetic analysis due to the lack of close relatives in databases, with the closest reference strain being Chroococcidiopsis thermalis sp. PCC 7203, with 84% similarity.
Acknowledgements
This work was financially supported by FCT (POCTI/CTA/46733/2002; SFRH/BD/29106/2006), ESF (III Quadro Comunitário de Apoio). We are grateful to Prof. Jiří Komárek for assistance with morphological identification, Dr Agostinho Antunes for assistance with the initial phylogenetic studies, and Prof. Paulo Fernandes for help with the geological description/characteristics of the sampling site.
References
- Abed , R , Garcia-Pichel , F and Hernández-Mariné , M . 2002 . Polyphasic characterization of benthic, moderately halophilic, moderately thermophilic cyanobacteria with very thin trichomes and the proposal of Halomicronema excentricum gen. nov., sp. nov . Arch. Microbiol. , 177 : 361 – 370 .
- Abed , RMM , Kohls , K and De Beer , D . 2007 . Effect of salinity changes on the bacterial diversity, photosynthesis and oxygen consumption of cyanobacterial mats from an intertidal flat of the Arabian Gulf . Environ. Microbiol. , 9 : 1384 – 1392 .
- Bolhuis , H , Severin , I , Confurius-Guns , V , Wollenzien , UIA and Stal , LJ . 2010 . Horizontal transfer of the nitrogen fixation gene cluster in the cyanobacterium Microcoleus chthonoplastes . ISME J. , 4 : 121 – 130 .
- Brown , II , Mummey , D and Cooksey , KE . 2005 . A novel cyanobacterium exhibiting an elevated tolerance for iron . FEMS Microbiol. Ecol. , 52 : 307 – 314 .
- Castenholz , RW . 2001 . “ Phylum BX. Cyanobacteria. Oxygenic photosynthetic bacteria ” . In Bergey's Manual of Systematic Bacteriology, , 2nd , Edited by: Boone , DR , Castenholz , RW and Garrity , GM . Vol. 1 , 473 – 599 . New York : Springer-Verlag .
- Cavalier-Smith , T . 2006 . Cell evolution and Earth history: stasis and revolution . Philos. Trans. R. Soc. Lond. B Biol. Sci. , 361 : 969 – 1006 .
- Cole , JR , Chai , B , Farris , RJ , Wang , Q , Kulam-Syed-Mohideen , AS , Mcgarrell , DM , Bandela , AM , Cardenas , E , Garrity , GM and Tiedje , JM . 2007 . The ribosomal database project (RDP-II): introducing myRDP space and quality controlled public data . Nucleic Acids Res. , 35 : D169 – D172 .
- Díez , B , Bauer , K and Bergman , B . 2007 . Epilithic cyanobacterial communities of a marine tropical beach rock (Heron Island, Great Barrier Reef): diversity and diazotrophy . Appl. Environ. Microbiol. , 73 : 3656 – 3668 .
- Felsenstein , J . 1981 . Evolutionary trees from DNA sequences: a maximum likelihood approach . J. Mol. Evol. , 17 : 368 – 376 .
- Felsenstein , J . 1989 . PHYLIP – Phylogeny Inference Package (Version 3.2) . Cladistics , 5 : 164 – 166 .
- Fitch , WM . 1971 . Toward defining the course of evolution: minimum change for a specified tree topology . Syst. Zool. , 20 : 406 – 416 .
- Garcia-Pichel , F . 2008 . “ Molecular ecology and environmental genomics of cyanobacteria ” . In Cyanobacteria: Molecular Biology, Genomics and Evolution , Edited by: Herrero , A and Flores , E . 59 – 87 . Norfolk : Caister Academic Press .
- Hoffmann , L . 1999 . Marine cyanobacteria in tropical regions: diversity and ecology . Eur. J. Phycol. , 34 : 371 – 379 .
- Hoffmann , L , Komárek , J and Kaštovský , J . 2005 . System of Cyanoprokaryotes (Cyanobacteria) – state in 2004 . Arch. Hydrobiol./Algol. Stud. , 117 : 95 – 115 .
- Ionescu , D , Oren , A , Hindiyeh , MY and Malkawi , HI . 2007 . “ The thermophilic cyanobacteria of the Zerka Ma’in thermal springs in Jordan ” . In Algae and Cyanobacteria in Extreme Environments , Edited by: Seckbach , J . 411 – 424 . Dordrecht : Springer .
- Janse , I , Kardinaal , WEA , Meima , M , Fastner , J , Visser , PM and Zwart , G . 2004 . Toxic and nontoxic Microcystis colonies in natural populations can be differentiated on the basis of rRNA gene internal transcribed spacer diversity . Appl. Environ. Microbiol. , 70 : 3979 – 3987 .
- Kluge , AG and Farris , JS . 1969 . Quantitative phyletics and the evolution of anurans . Syst. Zool. , 18 : 1 – 32 .
- Knoll , AH . 2008 . “ Cyanobacteria and earth history ” . In Cyanobacteria: Molecular Biology, Genomics and Evolution , Edited by: Herrero , A and Flores , E . 1 – 19 . Norfolk : Caister Academic Press .
- Komárek , J and Anagnostidis , K . 1998 . “ Cyanoprokaryota 1. Teil: Chroococcales ” . In Süsswasserflora von Mitteleuropa , Edited by: Ettl , H , Gartner , G , Heynig , H and Mollenhauer , D . Vol. 19/1. , 548 Stuttgart : Gustav Fischer .
- KOTAI, J. (1972). Instructions for preparation of modified nutrient solution Z8 for algae. Norwegian Institute for Water Research. B–11769. Blindern, Oslo, 5
- Kottek , M , Grieser , J , Beck , C , Rudolf , B and Rubel , F . 2006 . World map of the Köppen–Geiger climate classification updated . Meteorol. Z. , 15 : 259 – 263 .
- Leão , PN , Vasconcelos , MT and Vasconcelos , VM . 2007 . Role of marine cyanobacteria in trace metal bioavailability in seawater . Microb. Ecol. , 53 : 104 – 109 .
- Miller , SR and Castenholz , RW . 2000 . Evolution of thermotolerance in hot spring cyanobacteria of the genus Synechococcus . Appl. Environ. Microbiol. , 66 : 4222 – 4229 .
- Neilan , BA , Jacobs , D and Goodman , AE . 1995 . Genetic diversity and phylogeny of toxic cyanobacteria determined by DNA polymorphisms within the phycocyanin locus . Appl. Environ. Microbiol. , 61 : 3875 – 3883 .
- Nichols , D . 2007 . Cultivation gives context to the microbial ecologist . FEMS Microbiol. Ecol. , 60 : 351 – 357 .
- Nisbet , EG and Sleep , NH . 2001 . The habitat and nature of early life . Nature , 409 : 1083 – 1091 .
- Nübel , U , Garcia-Pichel , F and Muyzer , G . 1997 . PCR primers to amplify 16S rRNA genes from cyanobacteria . Appl. Environ. Microbiol. , 63 : 3327 – 3332 .
- Nübel , U , Garcia-Pichel , F and Muyzer , G . 2000 . The halotolerance and phylogeny of cyanobacteria with tightly coiled trichomes (Spirulina Turpin) and the description of Halospirulina tapeticola gen. nov., sp nov . Int. J. Syst. Evol. Microbiol. , 50 : 1265 – 1277 .
- Ohki , K , Kamiya , M , Honda , D , Kumazawa , S and Ho , KK . 2008 . Morphological and phylogenetic studies on unicellular diazotrophic cyanobacteria (cyanophytes) isolated from the coastal waters around Singapore . J. Phycol. , 44 : 142 – 151 .
- Omoregie , EO , Crumbliss , LL , Bebout , BM and Zehr , JP . 2004 . Comparison of diazotroph community structure in Lyngbya sp. and Microcoleus chthonoplastes dominated microbial mats from Guerrero Negro, Baja, Mexico . FEMS Microbiol. Ecol. , 47 : 305 – 318 .
- Oren , A and Tindall , BJ . 2005 . Nomenclature of the cyanophyta/cyanobacteria/cyanoprokaryotes under the International Code of Nomenclature of Prokaryotes . Arch. Hydrobiol./Algol. Stud. , 117 : 39 – 52 .
- Perrière , G and Gouy , M . 1996 . WWW-Query: an on-line retrieval system for biological sequence banks . Biochimie , 78 : 364 – 369 .
- Potts , M . 1999 . Mechanisms of desiccation tolerance in cyanobacteria . Eur. J. Phycol. , 34 : 319 – 328 .
- Rajaniemi , P , Hrouzek , P , Kastovska , K , Willame , R , Rantala , A , Hoffmann , L , Komárek , J and Sivonen , K . 2005 . Phylogenetic and morphological evaluation of the genera Anabaena, Aphanizomenon, Trichormus and Nostoc (Nostocales, Cyanobacteria) . Int. J. Syst. Evol. Microbiol. , 55 : 11 – 26 .
- Rey , J . 1983 . Le Cretacé de l'Algarve: essai de Synthèse . Comunicações dos Serviços Geológicos de Portugal , 69 : 87 – 101 .
- Rippka , R . 1988 . “ Isolation and purification of cyanobacteria ” . In Methods in Enzymology , Edited by: Packer , L and Glazer , AN . Vol. 167 , 3 – 27 . San Diego : Academic Press .
- Roldán , M and Hernández-Mariné , M . 2008 . The influence of green light on cyanobacterial fine structure: applicability for dim environments . Algological Studies , 126 : 159 – 171 .
- Saitou , N and Nei , M . 1987 . The neighbor-joining method: a new method for reconstructing phylogenetic trees . Mol. Biol. Evol. , 4 : 406 – 425 .
- Sambrook , J and Russell , DW . 2001 . Molecular Cloning: A Laboratory Manual, , 3rd , New York : Cold Spring Harbor Laboratory Press .
- Seabra , R , Santos , A , Pereira , S , Moradas-Ferreira , P and Tamagnini , P . 2009 . Immunolocalization of the uptake hydrogenase in the marine cyanobacterium Lyngbya majuscula CCAP 1446/4 and two Nostoc strains . FEMS Microbiol. Lett. , 292 : 57 – 62 .
- Sherwood , AR and Presting , GG . 2007 . Universal primers amplify a 23S rDNA plastid marker in eukaryotic algae and cyanobacteria . J. Phycol. , 43 : 605 – 608 .
- Shi , T and Falkowski , PG . 2008 . Genome evolution in cyanobacteria: the stable core and the variable shell . Proc. Natl. Acad. Sci. USA , 105 : 2510 – 2515 .
- Stal , LJ and Zehr , JP . 2008 . “ Cyanobacterial nitrogen fixation in the ocean: diversity, regulation, and ecology ” . In Cyanobacteria: Molecular Biology, Genomics and Evolution , Edited by: Herrero , A and Flores , E . 423 – 446 . Norfolk : Caister Academic Press .
- Tamagnini , P , Troshina , O , Oxelfelt , F , Salema , R and Lindblad , P . 1997 . Hydrogenases in Nostoc sp. strain PCC 73102, a strain lacking a bidirectional enzyme . Appl. Environ. Microbiol. , 63 : 1801 – 1807 .
- Taton , A , Grubisic , S , Ertz , D , Hodgson , DA , Piccardi , R , Biondi , N , Tredici , MR , Mainini , M , Losi , D , Marinelli , F and Wilmotte , A . 2006 . Polyphasic study of Antarctic cyanobacterial strains . J. Phycol. , 42 : 1257 – 1270 .
- Thompson , JD , Gibson , TJ , Plewniak , F , Jeanmougin , F and Higgins , DG . 1997 . The ClustalX windows interface: flexible strategies for multiple sequence alignment aided by quality analysis tools . Nucleic Acids Res. , 24 : 4876 – 4882 .
- Tomitani , A , Knoll , AH , Cavanaugh , CM and Ohno , T . 2006 . The evolutionary diversification of cyanobacteria: molecular-phylogenetic and paleontological perspectives . Proc. Natl. Acad. Sci. USA , 103 : 5442 – 5447 .
- Waleron , M , Waleron , K , Vincent , WF and Wilmotte , A . 2007 . Allochthonous inputs of riverine picocyanobacteria to coastal waters in the Arctic Ocean . FEMS Microbiol. Ecol. , 59 : 356 – 365 .
- Weisburg , WG , Barns , SM , Pelletier , DA and Lane , DJ . 1991 . 16S ribosomal DNA amplification for phylogenetic study . J. Bacteriol. , 173 : 697 – 703 .
- Wilmotte , A and Herdman , M . 2001 . “ Phylogenetic relationships among the cyabacteria based on 16S rRNA sequences ” . In Bergey's Manual of Systematic Bacteriology, , 2nd , Edited by: Boone , DR , Castenholz , RW and Garrity , GM . Vol. 1 , 487 – 493 . New York : Springer–Verlag .
- Zwirglmaier , K , Jardillier , L , Ostrowski , M , Mazard , S , Garczarek , L , Vaulot , D , Not , F , Massana , R , Ulloa , O and Scanlan , DJ . 2008 . Global phylogeography of marine Synechococcus and Prochlorococcus reveals a distinct partitioning of lineages among oceanic biomes . Environ. Microbiol. , 10 : 147 – 161 .