Abstract
The carposporophyte life-history phase is unique to florideophycean algae and its morphological development has been thoroughly investigated. Carposporophytes are tiny, generally embedded in female gametophyte tissues, and difficult to investigate via traditional molecular methods. However, subtractive cloning of differentially expressed genes from small amounts of tissue is now possible thanks to recent methodological advances. We applied a cDNA subtraction method using magnetic beads and PCR to isolate carposporophyte-specific cDNAs from Gracilariopsis andersonii (Gracilariales, Rhodophyta). Messenger RNAs extracted from carposporophyte tissues and vegetative thalli of the gametophyte were captured on oligo-dT magnetic beads, followed by first-strand cDNA synthesis in situ. Subtractive hybridization between the amplified second-strand carposporophyte cDNA in solution and the first-strand gametophyte cDNA attached to magnetic beads was conducted to remove common genes present in both gametophyte and carposporophyte tissues. Of 795 cDNA clones examined, 117 showed up-regulation of genes in carposporophyte tissues. Among 32 clones sequenced, we found sequences homologous to glutathione S-transferase (Chondrus crispus) and ESTs from Gracilaria. Full-length sequences for two such genes were obtained by RACE and their functions are discussed.
Introduction
The carposporophyte phase is a reproductive feature unique to florideophycean red algae. After syngamy, the diploid zygote develops into a multicellular carposporophyte while remaining physically connected to the female gametophyte. In the carposporophyte, short filamentous gonimoblasts generate carpospores that develop into diploid tetrasporophytes after discharge. Morphological development of the carposporophyte phase and the entire cystocarp (carposporophyte and surrounding female tissue) is diverse among red algae and contains taxonomically meaningful characters useful for phylogenetic classification (Hommersand & Fredericq, Citation1990). Nutritional dependence of the carposporophyte on its maternal gametophyte has been suggested based on the absence of normal chloroplasts in carposporophyte tissues (e.g. Wetherbee, Citation1980; Delivopoulos, Citation2003a ) and unidirectional flow of photosynthetically fixed carbon from gametophyte to carposporophyte (Turner & Evans, Citation1978). Reduction in developmental rate, spore production and germination success for carposporophytes excised from gametophyte tissues also suggest physiological dependence of the carposporophyte phase (West & McBride, Citation1999; Kamiya & Kawai, Citation2002). Significant differences in development between carposporophyte and vegetative tissues suggest developmental, life-history phase-specific gene regulation, although specific molecular mechanisms have not been elucidated.
Isolation of differentially expressed genes can be achieved by subtractive hybridization between cDNA libraries constructed from target and reference tissues. Liu et al. (Citation1994) successfully isolated phase-specific cDNAs from Porphyra purpurea (Roth) C. Agardh sporophytes and gametophytes using this method. However, construction of cDNA libraries requires large amounts of mRNA, limiting applicability to larger tissue samples. Advances in gene amplification and cloning have improved subtractive hybridization methods, alleviating this problem – oligo-dT labelled magnetic beads allow isolation and concentration of mRNAs from small amounts of tissue or cells (e.g. Hara et al., Citation1991; Coche et al., Citation1994; Karrer et al., Citation1995; Mészáros & Morton, Citation1996; Coche, Citation1997; Heinrich et al., Citation1997; Huang et al., Citation2004). Test tissue cDNAs are hybridized to an excess of cDNAs from a reference tissue, prior to mechanical, magnetic separation of any unhybridized cDNAs that remain. An oligo-dT primer covalently linked to the beads captures mRNAs and allows first-strand cDNA synthesis on the beads. Thus, cDNAs are bound to a solid phase, enabling repeated synthesis of second strand cDNAs and easy separation of unhybridized cDNA targets from common hybrids using magnetic force.
Gracilaria and Gracilariopsis (Gracilariales, Rhodophyta) are economically important for agar extraction and as a food source (Santelices & Doty, Citation1989; Oliveira et al., Citation2000). Some species have recently been used for molecular studies because their life cycles are easily controlled under laboratory conditions (Sun et al., Citation2002, Citation2006; Ren et al., Citation2006, Citation2008; Teo et al., Citation2007; Ren & Zhang, Citation2008). We found that mature female thalli of Gracilariopsis andersonii (Grunow) Dawson (formerly recognized as G. lemaneiformis; Gurgel et al., Citation2003), are available year round along the Californian coast. Additionally, intact carposporophytes are easily isolated during cystocarp dissection. In this study we applied a subtractive hybridization method using oligo-dT magnetic beads and PCR to construct life-history phase-specific cDNA libraries from carposporophytes of G. andersonii. Subsequently, two full-length cDNA sequences were obtained and compared with available protein sequence data.
Materials and methods
Sampling
Several female thalli with numerous cystocarps were collected from the intertidal zone at Four Mile Beach, Santa Cruz, California, on 10 May 2001. Samples were immediately transferred to our laboratory at the University of California Santa Cruz and used to generate carposporophyte cDNAs for subtractive hybridization. Pericarps were removed using a disposable syringe needle and naked carposporophyte tissues were dissected out (). Over 100 carposporophyte tissue samples (c. 80 mg wet weight), seen to produce few coloured carpospores, were stored in RNAlater (Qiagen, Chatsworth, CA, USA) at 4°C for 24 hours. An additional 70 carposporophyte tissue samples (c. 60 mg) were collected from the same site on 27 May 2001, and used to synthesize solid-phase cDNA libraries. A single female gametophyte thallus was cut into 5 mm fragments (c. 400 mg), frozen in liquid nitrogen and stored at −80°C.
Synthesis of solid-phase cDNA libraries from carposporophyte and vegetative tissues
We synthesized and subtracted phase-specific cDNA libraries after Coche (Citation1997). From total RNA solution, mRNA isolation and synthesis of first-strand cDNAs were conducted via oligo-(dT)25 coupled magnetic beads (Dynabeads mRNA DIRECT Kit, Dynal, Oslo, Norway). Throughout the procedure, magnetic beads were concentrated with a magnetic-particle concentrator (Dynal) before supernatant removal. First strand cDNAs were synthesized on magnetic beads for carposporophyte (to concentrate carposporophyte-specific cDNAs) and gametophyte (to subtract common cDNAs) tissues. Total RNA was extracted using the RNeasy Plant Mini Kit (Qiagen) and first-strand cDNAs were directly synthesized on the beads using Superscript II RNase H− reverse transcriptase (Gibco-BRL, Invitrogen Corp., Carlsbad, CA, USA).
Preparation of carposporophyte cDNA fragments to be subtracted
An additional cDNA library was synthesized on fresh magnetic beads from the carposporophyte tissues collected on 10 May, in preparation for carposporophyte cDNA fragment subtraction. Second-strand cDNAs were synthesized using the Random Primers DNA Labeling System (Gibco-BRL) and then digested with RsaI. For PCR-amplification of digested cDNA fragments, a 23-mer, linker-1 (5′-ATCCTTCCCAGCCTGGGATCCCT-3′) and its complementary 22-mer, linker-2 (5′-AGGGATCCCAGGCTGGGAAGGA-3′), designed by Coche (Citation1997), were ligated to cDNA fragment ends. PCR reactions were conducted in 100 µl volumes containing 2.0 µl of cDNA solution, 100 pmol linker, 20 nmol of each dNTP, 1 × PCR buffer and 2 units of ExTaq DNA polymerase (TaKaRa Bio., Shiga, Japan). Small cDNA fragments (less than 50 bp) were removed from PCR products using CHROMA SPIN Column-200 (CLONTECH Laboratories, Palo Alto, CA, USA) and the flow-through (carposporophyte cDNA fragments) was used for the next hybridization.
First subtractive hybridization
PCR-amplified carposporophyte cDNA fragments were denatured at 94°C and then annealed back onto the carposporophyte cDNA library bound to beads suspended in 5.2 µl of 20 × SSC and 2 µl of 10 mM EDTA. After hybridization for 24 h at 55°C, the supernatant was discarded and hybridized cDNA fragments were denatured in 7.2 µl of 1.4 mM EDTA for 3 min at 94°C. A 30 µl aliquot of this cDNA solution (carposporophyte cDNA fragments annealed to the carposporophyte cDNA library) was hybridized to a gametophyte cDNA library bound to beads for 24 h at 55°C to subtract common cDNAs.
Preparation of carposporophyte-specific cDNA library on the beads
The subtracted supernatant (carposporophyte cDNA fragments that did not anneal to the gametophyte cDNA library; Target-1) and hybridized cDNA fragments (common to both carposporophyte and gametophyte; Common-1) were PCR amplified with the linker-1 primer and purified using QIAEX II (Qiagen). Another 5 µl aliquot of beads containing the carposporophyte cDNA library was annealed to amplified Common-1 fragments for 24 h at 55°C, and bead-bound double-stranded cDNAs were digested by RsaI and AluI for 2 h at 37°C. The latter step was preformed to eliminate common cDNA fragments from the carposporophyte cDNA library. Therefore, single-stranded cDNA (mainly carposporophyte-specific) eluded digestion and remained attached to the beads.
Second subtractive hybridization
To concentrate carposporophyte-specific cDNA fragments, amplified Target-1 fragments were annealed to a carposporophyte-specific cDNA library, and subtracted cDNA fragments (Target-2) were eluted by thermal denaturation. To further eliminate common cDNA fragments, Target-2 cDNA fragments were hybridized to a fresh, bead-bound gametophyte cDNA library. The final subtracted supernatant was PCR amplified and then size-fractionated using CHROMA SPIN Column-400. The final, subtracted cDNA library was constructed using pGEM-T Easy plasmid vectors and JM109 competent cells (Promega, Madison, WI, USA).
Screening of the subtracted cDNA library
To test the carposporophyte-specificity of the subtracted cDNA library, randomly selected clones were hybridized with labelled cDNA probes specific to both carposporophyte and gametophyte phases. Phase-specific cDNA probes were generated from carposporophyte and gametophyte total RNA extractions using the SMART PCR cDNA Synthesis Kit (CLONTECH Laboratories). After phenol/chloroform extraction and CHROMA Spin Column-1000 size-fractionation, flow-through was digested with RsaI and QIAEX II purified. Adaptors (Ad1-F: 5′-CTAATACGACTCACTATAGGGCTCGAGCGGCCGCCCGGGCAGGT-3′; Ad1-R: 5′-ACCTGCCCGG-3′) were then ligated to digested cDNA fragment ends. Primary and secondary (nested) PCRs were performed with primers P1 (5′-CTAATACGACTCACTATAGGGC-3′) and NP1 (5′-TCGAGCGGCCGCCCGGGCAGGT-3′), respectively. The PCR product was QIAEX II purified and functioned as a probe for subsequent screening.
cDNA inserts were PCR amplified from 795 randomly selected colonies of the subtracted library. One µl of 0.6 N NaOH was added to 1 µl of each PCR-amplified insert, and 1 µl of the mixture was blotted on two 6 × 5 cm positively charged nylon membranes (Hybond-N+, GE Healthcare Bio-Sciences, Uppsala, Sweden). Two replicate membranes containing 120 dot blotted PCR-amplified inserts were incubated in 1.5 ml of 0.5 M Tris buffer (pH 7.4) for 4 min, washed with deionized distilled water (DDW), and baked for 15 min at 120°C. Probes synthesized from carposporophyte and gametophyte cDNAs were applied to each replicate membrane, respectively. The Alkphos direct labelling and detection system with CDP-Star (GE Healthcare Bio-Sciences) was used for DNA probe labelling and detection.
Reverse northern blotting
To confirm the carposporophyte-specificity of cDNA clones showing a positive signal upon differential screening, reverse northern blotting was performed using PCR-amplified cDNAs from carposporophyte and gametophyte samples collected on 27 May. cDNAs synthesized with the SMART PCR cDNA Synthesis Kit were electrophoresed in agarose gel, transferred to a membrane filter, and exposed to colony PCR generated DNA probes.
Sequencing of full-length cDNAs
Twenty-one carposporophyte-specific cDNA clones were successfully sequenced on an ABI PRISM 3100 Genetic Analyzer (Applied Biosystems, Foster City, CA, USA). Of these, two clones were determined to contain complete gene sequences. 5′RACE (rapid amplification of cDNA ends) and 3′RACE were carried out using the SMART RACE cDNA Amplification Kit (CLONTECH Laboratories). Partial gene sequence data (accession numbers AB573693–AB573709) and complete gene sequences (accession numbers AB533272 and AB533273) were deposited in the DNA Data Bank of Japan sequence database. Sequence similarity searches of Genbank protein databases were performed with the tBLASTX and PSI-blast programs. The simple modular architecture research tool (Letunic et al., Citation2009) was used for the identification and annotation of protein domains. Transmembrane topology was predicted with the SOSUI program (http://bp.nuap.nagoya-u.ac.jp/sosui/). The presence of a signal peptide sequence was determined using SignalP 3.0 program (Bendtsen et al., Citation2004).
The identification of an intron in both complete gene sequences was accomplished by sequencing genomic DNAs extracted from a cultured female gametophyte thallus collected from Four Mile Beach, Santa Cruz, California, on 9 January 2001. Culture methods and conditions are described in Kamiya et al. (Citation1997). Genomic DNAs were extracted using the E.Z.N.A. Plant DNA Miniprep Kit (Omega Bio-tek, Doraville, GA, USA). Primers were designed based on cDNA sequences and PCR products were directly sequenced as described above.
Results
Before subtraction, RsaI-digested cDNA fragments measured 130–1200 bp in length, while subtracted cDNAs ranged from 150–500 bp post-hybridization series. Subtracted cDNA fragments were transformed and 117 of 795 colonies tested yielded greater carposporophyte DNA probe signal intensity than was seen for the gametophyte probe (). When a clone showing a similar signal intensity between carposporophyte and gametophyte was used as a probe in the reverse northern blotting, identical bands appeared in both carposporophyte and gametophyte cDNA lanes (49-8 in ). Among the 117 clones, 36 clones exhibiting remarkable signal difference between gametophyte and carposporophyte probes were subjected to reverse northern blotting, and all but one clone hybridized specifically to carposporophyte cDNAs (e.g. 47-33, 49-10 and 56-2.1 in ). In total 32 out of the 36 clones were successfully sequenced, and 21 unique sequences were obtained. Clones with the greatest similarity to known DNA or expressed sequence tag (EST) sequences are listed in .
Figs 3, 4. Results of differential screening. Two identical membranes blotted with PCR-amplified cDNA sequences from the subtracted library were detected with probes constructed from gametophyte () or carposporophyte cDNA fragments (). Circles indicate spots showing stronger signal intensity in response to the carposporophyte cDNA probe.
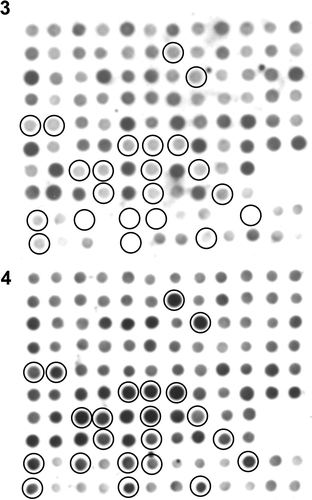
Fig. 5. Four results from reverse northern blotting. Amplified cDNAs from the gametophyte (lane 1) and carposporophyte (lane 2) were electrophoresed and hybridized to a probe produced from each subtractive cDNA clone.
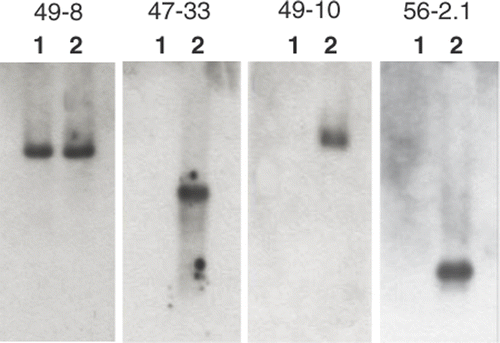
Table 1. Putative identities of subtracted cDNA fragments from Gracilariopsis andersonii.
5′ and 3′ RACE methods were applied to some of the 21 clones sequenced, and complete cDNA gene sequences were successfully obtained from two clones (47-33 and 56-2.1). In both cases the complete mRNA sequences were 3′ polyadenylated. These genes re-sequenced from genomic DNA templates showed identical sequences to their corresponding cDNAs, indicating a lack of introns for both genes.
The first complete cDNA gene sequence (Gand-carp1) was 1408 nucleotides in length (not including the poly-A tail) with an open reading frame of 852 nucleotides (encoding 284 amino acid residues), a 5′ untranslated region of 27 nucleotides and a 3′ non-coding region of 526 nucleotides (). The predicted protein had a calculated molecular mass of 31.2 kDa and a theoretical isoelectric pH of 9.4. Protein motif analysis indicated that this protein had two transmembrane domains (underlined in ) consistent with the prediction of hydrophobicity. The region from the 54th aa to the 120th aa (highlighted in ) was weakly homologous to the double-psi beta-barrel (DPBB) domain contained in Rare lipoprotein A (RlpA) (E-value = 9.1e−04). Results of BLAST searches showed high similarity to a putative lipoprotein of Ectocarpus siliculosus (Dillwyn) Lyngbye (accession no. CBN74372; E-value = 10e−46).
Fig. 6. Nucleotide and amino acid sequences for Gand-carp1. The hydrophobic regions are underlined and a region homologous to the DPBB domain is highlighted. The poly-A tail is not indicated.
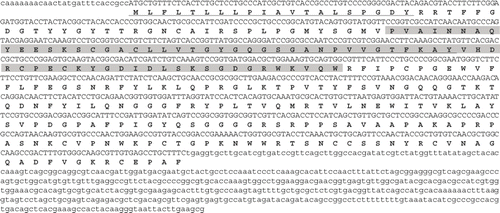
The second complete cDNA gene sequence (Gand‐carp2) was 1002 nucleotides in length (not including the poly-A tail) with an open reading frame of 582 nucleotides (encoding 194 amino acid residues), a 5′ untranslated region of 85 nucleotides and a 3′ non-coding region of 332 nucleotides (). The predicted protein had a calculated molecular mass of 21.6 kDa and a theoretical isoelectric pH of 8.5. A hydrophobic region at the N-terminal end was predicted to be a signal peptide (highlighted in ). The central hydrophilic region from amino acid position 85 to 130 exhibited a high probability of forming a coiled-coil structure (underlined in ). BLAST search results suggested this gene was partially homologous to an EST (DV966959) of Gracilaria changii (Xia & Abbott) Abbott shown in .
Discussion
Subtractive cDNA hybridization is a powerful method for identifying differentially expressed genes during biological processes such as alternation of life-history phases, cellular differentiation and physiological responses to environmental variables. In this study, isolation of mRNAs, synthesis of both primary and secondary strand cDNAs and subtractive hybridization were performed in situ on magnetic beads. Our approach allowed easy isolation of sufficient transcripts for amplification and sequencing from small amounts of carposporophyte tissue while effectively removing non-target cDNAs with minimal target loss. Approximately 15% of subtracted cDNA clones were up-regulated or only expressed in carposporophyte tissue samples. We attempted additional rounds of subtractive hybridization, but there was no remarkable change in the subtraction efficiency (data not shown).
Diatchenko et al. (Citation1996) developed a PCR-based cDNA subtraction method, called suppression subtractive hybridization (SSH), to selectively amplify target cDNA fragments and simultaneously suppress non-target DNA amplification. SSH includes a normalization step that equalizes the relative abundance of cDNA within a target population, a modification that enhances the probability of identifying low-abundance transcripts (Diatchenko et al., Citation1996; Rebrikov et al., Citation2004). This method has been applied to many kinds of organisms including seaweeds (Pearson et al., Citation2001; Ren et al., Citation2006; Peters et al., Citation2008; Cosse et al., Citation2009), though some limitations of the SSH technique have been pointed out (Rebrikov et al., Citation2002). Ren et al. (Citation2006) reported that 13 out of 768 clones examined using the SSH method had cDNA fragments differentially expressed between tetrasporophytes and gametophytes of Gracilariopsis lemaneiformis and only one clone was tetrasporophyte-specific. We applied SSH during a preliminary step, but found subtraction efficiency to be quite low (data not shown).
Some cDNA sequences showed significant similarity to several ESTs from Gracilaria changii (), fairly close phylogenetically to Gracilariopsis (Bird et al., Citation1992; Goff et al., Citation1994). Because EST analysis of G. changii was based on environmental samples (Teo et al., Citation2007), carposporophyte tissues probably accompanied field-collected materials. EST analyses have also been performed for other red algae, such as Gracilaria gracilis (Stackhouse) Steentoft, Irvine & Farnham (cultured thalli in Lluisma & Ragan, Citation1997), Porphyra yezoensis Ueda (field-collected gametophytes in Lee et al., Citation2000; cultured gametophytes in Nikaido et al., Citation2000; cultured sporophytes in Asamizu et al., Citation2003), Gracilariopsis lemaneiformis (as Gracilaria lemaneiformis; cultured gametophytes in Sun et al., Citation2002) and Griffithsia okiensis Kajimura (cultured gametophytes and sporophytes in Lee et al., Citation2007). Cyanidioschyzon merolae De Luca, Taddei & Varano is the only red alga for which complete nuclear genomic sequences are available (Matsuzaki et al., Citation2004). Furthermore, a homology search against genome sequences of Chondrus crispus Stackhouse, which are almost complete, was carried out individually (Jonas Collen, personal communication) but the results were similar to the blast search results in . There are several possible reasons why our cDNA sequences failed to show significant similarity to these ESTs and complete genome sequences. Firstly, our carposporophyte-specific genes may not be present in non-Florideophycean algae, such as Cyanidioschizon and Porphyra, which lack a carposporophyte phase. Secondly, these genes may have diverged beyond easy recognition in the above species. Thirdly, the gene transcripts examined in this study may be so rare (such as mRNAs encoding regulatory proteins) as to escape detection from EST libraries.
Clone 55-19.1 and 57-29.3 showed high similarity to glutathione S-transferase (GST) of Chondrus crispus, which is an enzyme that catalyses nucleophilic conjugation of the reduced form of tripeptide glutathione to a wide variety of hydrophobic, electrophilic and usually cytotoxic substrates. GST in land plants has been subdivided into eight classes with unique functions such as alleviating environmental stress, transport of endogenous natural products into vacuoles, hormone homeostasis, cell signalling, and regulation of apoptosis (Basantani & Srivastava, Citation2007). Although this enzyme has been found in red algae (Pflugmacher et al., 2000; Hervé et al., 2008), further investigation would be required to reveal its function in the carposporophyte phase.
Protein motif analysis indicated that Gand-carp1 contains the region weakly homologous to the DPBB domain in the lipoprotein RlpA. Homologous sequences of the RlpA gene are observed in various bacteria, but not generally in eukaryotes, except for some aphid species (Nikoh & Nakabachi, Citation2009). Although the function of RlpA is not well understood, it has been shown to act as a suppressor of prc mutant Escherichia coli (Bass et al., Citation1996) and the DPBB fold of this gene is suspected to be an enzymatic domain (Castillo et al., Citation1999). The amino acid sequence of Gand-carp1 showed a high similarity to a putative lipoprotein of Ectocarpus siliculosus. Considering the presence of two transmembrane domains (), it is possible that Gand-carp1 is an insoluble membrane protein.
The hydrophobic region at the N-terminal end of Gand-carp2 was predicted to be a signal peptide. Gand-carp2 is probably a soluble protein as it has no other hydrophobic regions, though it did not show similarity to any known proteins. Because this gene was detected in several other subtractive cDNA clones and was similar to an EST of Gracilaria changii, it may be highly expressed in carposporophyte tissues.
No introns were found in the Gand-carp1 nor Gand-carp2 genes. There are typically no introns in prokaryotic genomes (although one has been found in transfer and ribosomal RNA genes of archaebacteria; Jackson et al., Citation2002; Tocchini-Valentini et al., Citation2005), but the mRNAs of Gand-carp1 and Gand-carp2 were polyadenylated, characteristic of eukaryotic genes. Because only 5% of complete gene sequences were found to include introns in Cyanidioschyzon merolae (Nozaki et al., Citation2007), the lack of introns may be common in red algae.
One widely held notion is that retention of the carposporophyte and its nourishment by the female gametophyte allow florideophycean algae to enhance reproductive fecundity because a single fertilization event can lead to production of very large numbers of tetrasporophytes (Searles, Citation1980; Brawley & Johnson, Citation1992). Nevertheless, the degree of reliance upon the female gametophyte differs among florideophycean algae. For example, carposporophytes in some members of Acrochaetiales, Batrachospermales and Nemaliales contain mature plastids that are presumably photosynthetically functional (Ramm-Anderson & Wetherbee, Citation1982; Hommersand & Fredericq, Citation1990; Sheath et al., Citation1996; Delivopoulos, Citation2003b ). In some palmarialean algae, a fertilized carpogonium develops directly into a tetrasporophyte without production of a carposporophyte (van der Meer & Todd, Citation1980; Mitman & Phinney, Citation1985). Comparative analyses of carposporophyte-specific transcripts among these taxa may help us to further understand how functional genes regulate the carposporophyte life-history phase.
Goff & Coleman (Citation1987) suggest that the processes involved in post-fertilization development, such as transfer of a zygotic nucleus into gametophytic cells, formation of a heterokaryotic fusion cell, and packaging of the diploid nuclei into gonimoblast initials, are extraordinarily similar to processes found in red algal parasites. Parasites comprise 20% of all known red algal genera (Goff, Citation1982), and phylogenetic data suggest that many taxa acquired parasitism independently (Goff & Zuccarello, Citation1994; Kurihara et al., Citation2010). If the ability to parasitize red algal hosts originated from complex post-fertilization behaviour (Goff & Coleman, Citation1987), some genes expressed in the parasitic cells (and also in fusion cells) may be homologous to carposporophyte-specific genes. The parasite Gracilariophila oryzoides Setchell & Wilson is abundant on Gracilariopsis andersonii along the California coast (Abbott & Hollenberg, Citation1976; Silva, Citation1979), and therefore would represent an excellent candidate to survey for the presence of carposporophyte-specific transcripts in its genome.
In conclusion, we have shown that subtractive hybridization using oligo-dT magnetic beads and PCR is useful for isolating carposporophyte-specific transcripts. This method is effective given minimal tissue containing a small amount of mRNA, because isolation of mRNAs, synthesis of cDNAs and subtractive hybridization can be carried out on magnetic beads with little target loss. Many of the subtracted cDNAs were up-regulated or only expressed in carposporophyte samples. However, most carposporophyte-specific cDNA sequences analysed encoded proteins with unknown homologues. Further investigations such as quantitative analysis, cellular localization and expression timing for each protein are required for a better understanding of the mechanisms controlling the complicated process of post-fertilization development, the interrelationship between carposporophyte and gametophyte and the evolution of the unique, red algal carposporophyte life-history phase.
Acknowledgements
The authors would like to acknowledge Dr Julio Harvey for his help during the experimentation and writing phases of this project. We also thank Drs Jonas Collen and Yoo Kyung Lee for providing the information about Chondrus crispus genome sequence data and Griffithsia okiensis EST data, respectively. This project was supported in part by Grant-in-Aid for Scientific Researchers from the Ministry of Education, Science, Culture and Sport, Japan to MK.
References
- Abbott , IA and Hollenberg , GJ . 1976 . Marine Algae of California , Stanford, California : Stanford University Press .
- Asamizu , E , Nakajima , M , Kitade , Y , Saga , N , Nakamura , Y and Tabata , S . 2003 . Comparison of RNA expression profiles between the two generations of Porphyra yezoensis (Rhodophyta), based on expressed sequence tag frequency analysis . J. Phycol. , 39 : 923 – 930 .
- Basantani , M and Srivastava , A . 2007 . Plant glutathione transferases – a decade falls short . Can. J. Bot. , 85 : 443 – 456 .
- Bass , S , Gu , Q and Christen , A . 1996 . Multicopy suppressors of prc mutant Escherichia coli include two HtrA (DegP) protease homologs (HhoAB), DksA, and a truncated R1pA . J. Bacteriol. , 178 : 1154 – 1161 .
- Bendtsen , JD , Nielsen , H , Von Heijne , G and Brunak , S . 2004 . Improved prediction of signal peptides: SignalP 3.0 . J. Mol. Biol. , 340 : 783 – 795 .
- Bird , CJ , Rice , EL , Murphy , CA and Ragan , MA . 1992 . Phylogenetic relationships in the Gracilariales (Rhodophyta) as determined by 18S rDNA sequences . Phycologia , 31 : 510 – 522 .
- Brawley , SH and Johnson , LE . 1992 . Gametogenesis, gametes and zygotes: an ecological perspective on sexual reproduction in the algae . Br. Phycol. J. , 27 : 233 – 252 .
- Castillo , RM , Mizuguchi , K , Dhanaraj , V , Albert , A , Blundell , TL and Murzin , AG . 1999 . A six-stranded double-psi beta barrel is shared by several protein superfamilies . Structure , 7 : 227 – 236 .
- Coche , T . 1997 . “ Subtractive cDNA cloning using magnetic beads and PCR ” . In Methods in Molecular Biology, Vol. 67: PCR Cloning protocols, from Molecular Cloning to Genetic Engineering , Edited by: White , BA . 371 – 387 . Totowa, NJ : Humana Press Inc. .
- Coche , T , Dewez , M and Beckers , M-C . 1994 . Generation of an unlimited supply of a subtracted probe using magnetic beads and PCR . Nucleic Acids Res. , 22 : 1322 – 1323 .
- Cosse , A , Potin , P and Leblanc , C . 2009 . Patterns of gene expression induced by oligoguluronates reveal conserved and environment-specific molecular defense responses in the brown alga Laminaria digitata . New Phytol. , 182 : 239 – 250 .
- Delivopoulos , SG . 2003a . Ultrastructure of auxiliary and gonimoblast cells during carposporophyte development in the red alga Cryptopleura ruprechtiana (Delesseriaceae, Ceramiales, Rhodophyta) . Biol. Cell , 95 : 383 – 392 .
- Delivopoulos , SG . 2003b . Ultrastructure of post-fertilization development in the red alga Scinaia articulata (Galaxauraceae, Nemaliales, Rhodophyta) . Biol. Cell , 95 : 27 – 38 .
- Diatchenko , L , Lau , YFC , Campbell , AP , Chenchik , A , Moqadam , F , Huang , B , Lukyanov , S , Lukyanov , K , Gurskaya , N , Sverdlov , ED and Siebert , PD . 1996 . Suppression subtractive hybridization: A method for generating differentially regulated or tissue-specific cDNA probes and libraries . Proc. Natl. Acad. Sci. USA , 93 : 6025 – 6030 .
- Goff , LJ . 1982 . “ The biology of parasitic red algae ” . In Progress in Phycological Research. Vol. 1 , Edited by: Round , FE and Chapman , DJ . 289 – 369 . Amsterdam : Elsevier Biomedical Press .
- Goff , LJ and Coleman , AW . 1987 . “ Nuclear transfer from parasite to host. A new regulatory mechanism of parasitism ” . In Endocytobiology, , Edited by: Lee , JJ and Fredrick , JF . Vol. 3 , 402 – 423 . New York : Annals of the New York Academy of Sciences .
- Goff , LJ , Moon , DA and Coleman , AW . 1994 . Molecular delineation of species and species relationships in the red algal agarophytes Gracilariopsis and Gracilaria (Gracilariales) . J. Phycol. , 30 : 521 – 537 .
- Goff , LJ and Zuccarello , GC . 1994 . The evolution of parasitism in red algae: cellular interactions of adelphoparasites and their hosts . J. Phycol. , 30 : 695 – 720 .
- Gurgel , CFD , Liao , LM , Fredericq , S and Hommersand , MH . 2003 . Systematics of Gracilariopsis (Gracilariales, Rhodophyta) based on rbcL sequence analyses and morphological evidence . J. Phycol. , 39 : 154 – 171 .
- Hara , E , Kato , T , Nakada , S , Sekiya , S and Oda , K . 1991 . Subtractive cDNA cloning using oligo (dT)30-latex and PCR: isolation of cDNA clones specific to undifferentiated human embryonal carcinoma cells . Nucleic Acids Res. , 19 : 7097 – 7104 .
- Heinrich , T , Washer , S , Marshall , J , Jones , MGK and Potter , RH . 1997 . Subtractive hybridization of cDNA from small amounts of plant tissue . Mol. Biotech. , 8 : 7 – 12 .
- Hervé , C , de Franco , PO , Groisillier , A , Tonon , T and Boyen , C . 2008 . New members of the glutathione transferase family discovered in red and brown algae . Biochem. J. , 412 : 535 – 544 .
- Hommersand , MH and Fredericq , S . 1990 . “ Sexual reproduction and cystocarp development ” . In Biology of the Red Algae , Edited by: Cole , KM and Sheath , RG . 305 – 346 . New York : Cambridge University Press .
- Huang , G , Dong , R , Maier , T , Allen , R , Davis , EL , Baum , TJ and Hussey , RS . 2004 . Use of solid-phase subtractive hybridization for the identification of parasitism gene candidates from the root-knot nematode Meloidogyne incognita . Mol. Plant Pathol. , 5 : 217 – 222 .
- Jackson , S , Cannone , J , Lee , J , Gutell , R and Woodson , S . 2002 . Distribution of rRNA introns in the three-dimensional structure of the ribosome . J. Mol. Biol. , 323 : 35 – 52 .
- Kamiya , M and Kawai , H . 2002 . Dependence of the carposporophyte on the maternal gametophyte in three Ceramiacean algae (Rhodophyta), with respect to carposporophyte development, spore production and germination success . Phycologia , 41 : 107 – 115 .
- Kamiya , M , Tanaka , J and Hara , Y . 1997 . Comparative morphology, crossability and taxonomy within the Caloglossa continua (Delesseriaceae, Rhodophyta) complex from the western Pacific . J. Phycol. , 33 : 97 – 105 .
- Karrer , EE , Lincoln , JE , Hogenhout , S , Bennett , AB , Bostock , RM , Martineau , B , Lucas , WJ , Gilchrist , DG and Alexander , D . 1995 . In situ isolation of mRNA from individual plant cells: creation of cell-specific cDNA libraries . Proc. Natl. Acad. Sci. USA , 92 : 3814 – 3818 .
- Kurihara , A , Abe , T , Tani , M and Sherwood , AR . 2010 . Molecular phylogeny and evolution of red algal parasites: a case study of Benzaitenia, Janczewskia, and Ululania (Ceramiales) . J. Phycol. , 46 : 580 – 590 .
- Lee , EK , Seo , SB , Kim , TH , Sung , S-K , An , G , Lee , C-H and Kim , Y-J . 2000 . Analysis of expressed sequence tags of Porphyra yezoensis . Mol. Cells , 10 : 338 – 342 .
- Lee , H , Lee , HK , An , G and Lee , YK . 2007 . Analysis of expressed sequence tags from the red alga Griffithsia okiensis . J. Microbiol. , 45 : 541 – 546 .
- Letunic , I , Doerks , T and Bork , P . 2009 . SMART 6: recent updates and new developments . Nucleic Acids Res. , 37 : D229 – D232 .
- Liu , QY , van der Meer , JP and Reith , ME . 1994 . Isolation and characterization of phase-specific complementary DNAs from sporophytes and gametophytes of Porphyra purpurea (Rhodophyta) using subtracted complementary DNA libraries . J. Phycol. , 30 : 513 – 520 .
- Lluisma , AO and Ragan , MA . 1997 . Expressed sequence tags (ESTs) from the marine red alga Gracilaria gracilis . J. Appl. Phycol. , 9 : 287 – 293 .
- Matsuzaki , M , Misumi , O , Shin-I , T , Maruyama , S , Takahara , M , Miyagishima , SY , Mori , T Nishida , K . 2004 . Genome sequence of the ultrasmall unicellular red alga Cyanidioschyzon merolae 10D . Nature , 428 : 653 – 657 .
- Mészáros , M and Morton , DB . 1996 . Subtractive hybridization strategy using paramagnetic oligo(dT) beads and PCR . Biotechniques , 20 : 413 – 419 .
- Mitman , GG and Phinney , HK . 1985 . The development and reproductive morphology of Halosaccion americanum I. K. Lee (Rhodophyta, Palmariales) . J. Phycol. , 21 : 578 – 584 .
- Nikaido , I , Asamizu , E , Nakajima , M , Nakamura , Y , Saga , N and Tabata , S . 2000 . Generation of 10,154 expressed sequence tags from a leafy gametophyte of a marine red alga, Porphyra yezoensis . DNA Res. , 7 : 223 – 227 .
- Nikoh , N and Nakabachi , A . 2009 . Aphids acquired symbiotic genes via lateral gene transfer . BMC Biology , 7 : 12
- Nozaki , H , Takano , H , Misumi , O , Terasawa , K , Matsuzaki , M , Maruyama , S , Nishida , K , Yagisawa , F , Yoshida , Y , Fujiwara , T , Takio , S , Tamura , K , Chung , SJ , Nakamura , S , Kuroiwa , H , Tanaka , K , Sato , N and Kuroiwa , T . 2007 . A 100%-complete sequence reveals unusually simple genomic features in the hot-spring red alga Cyanidioschyzon merolae . BMC Biology , 5 : 28
- Oliveira , EC , Alveal , K and Anderson , RJ . 2000 . Mariculture of the agar-producing gracilarioid red algae . Rev. Fish. Sci. , 8 : 345 – 377 .
- Pearson , G , Serrão , EA and Cancela , ML . 2001 . Suppression subtractive hybridization for studying gene expression during aerial exposure and desiccation in fucoid algae . Eur. J. Phycol. , 36 : 359 – 366 .
- Peters , AF , Scornet , D , Ratin , M , Charrier , B , Monnier , A , Merrien , Y , Corre , E , Coelho , SM and Cock , JM . 2008 . Life-cycle-generation-specific developmental processes are modified in the immediate upright mutant of the brown alga Ectocarpus siliculosus . Development , 135 : 1503 – 1512 .
- Pflugmacher , S , Schröder , P and Sandermann , H . 2000 . Taxonomic distribution of plant glutathione S-transferases acting on xenobiotics . Phytochemistry , 54 : 267 – 273 .
- Ramm-Anderson , SM and Wetherbee , R . 1982 . Structure and development of the carposporophyte of Nemalion helminthoides (Nemalionales, Rhodophyta) . J. Phycol. , 18 : 133 – 141 .
- Rebrikov , D , Desai , S , Kogan , YN , Thornton , AM and Diatchenko , L . 2002 . Subtractive cloning: new genes for studying inflammatory disorders . Ann. Periodontol. , 7 : 17 – 28 .
- Rebrikov , DV , Desai , SM , Siebert , PD and Lukyanov , SA . 2004 . Suppression Subtractive Hybridization . Methods Mol. Biol. , 258 : 107 – 134 .
- Ren , X and Zhang , X . 2008 . Identification of a putative tetrasporophyte-specific gene in Gracilaria lemaneiformis (Gracilariales, Rhodophyte) . J. Ocean Univ. China , 7 : 299 – 303 .
- Ren , X , Zhang , X , Mao , Y , Sui , Z , Xu , D and Zang , X . 2008 . Cloning and characterization of a Rab11 homologue in Gracilariopsis lemaneiformis . J. Appl. Phycol. , 20 : 1103 – 1109 .
- Ren , X , Zhang , X and Sui , Z . 2006 . Identification of phase relative genes in tetrasporophytes and female gametophytes of Gracilaria/Gracilariopsis lemaneiformis (Gracilariales, Rhodophyta) . Electron. J. Biotechnol. , 9 : 127 – 132 .
- Santelices , B and Doty , MS . 1989 . A review of Gracilaria farming . Aquaculture , 78 : 98 – 133 .
- Searles , RB . 1980 . The strategy of the red algal life history . Am. Nat. , 115 : 113 – 120 .
- Sheath , RG , Müller , KM , Whittick , A and Entwisle , TJ . 1996 . A re-examination of the morphology and reproduction of Nothocladus lindaueri (Batrachospermales, Rhodophyta) . Phycol. Res. , 44 : 233 – 246 .
- Silva , PC . 1979 . “ The benthic algal flora of central San Francisco Bay ” . In San Francisco Bay: The Urbanized Estuary , Edited by: Conomos , TJ . 287 – 345 . San Francisco, CA : Pacific Division of the American Association for the Advancement of Science .
- Sun , X , Yang , G , Mao , Y , Zhang , X , Sui , Z and Qin , S . 2002 . Analysis of expressed sequence tags of a marine red alga, Gracilaria lemaneiformis . Prog. Nat. Sci. , 12 : 518 – 523 .
- Sun , X , Zhang , X , Mao , Y , Sui , Z and Qin , S . 2006 . Identification of phase and sex-related ISSR markers of red alga Gracilaria lemaneiformis . J. Ocean Univ. China , 5 : 82 – 84 .
- Teo , S-S , Ho , C-L , Teoh , S , Lee , W-W , Tee , J-M , Rahim , RA and Phang , S-M . 2007 . Analyses of expressed sequence tags from an agarophyte, Gracilaria changii (Gracilariales, Rhodophyta) . Eur. J. Phycol. , 42 : 41 – 46 .
- Tocchini-Valentini , GD , Fruscoloni , P and Tocchini-Valentini , GP . 2005 . Structure, function, and evolution of the tRNA endonucleases of Archaea: An example of subfunctionalization . Proc. Natl. Acad. Sci. USA , 102 : 8933 – 8938 .
- Turner , CHC and Evans , LV . 1978 . Translocation of photoassimilated 14C in the red alga Polysiphonia lanosa . Br. Phycol. J. , 13 : 51 – 55 .
- van der Meer , JP and Todd , ER . 1980 . The life history of Palmaria palmata in culture. A new type for the Rhodophyta . Can. J. Bot. , 58 : 1250 – 1256 .
- West , JA and McBride , DL . 1999 . Long-term and diurnal carpospore discharge patterns in the Ceramiaceae, Rhodomelaceae and Delesseriaceae (Rhodophyta) . Hydrobiologia , 398/399 : 101 – 113 .
- Wetherbee , R . 1980 . Postfertilization development in the red alga Polysiphonia 1. Proliferation of the carposporophyte . J. Ultrastr. Res. , 70 : 259 – 274 .