Abstract
The vertical migratory behaviour of estuarine microphytobenthos, i.e. the biofilm-forming microalgae inhabiting intertidal sediments, is probably a significant factor for their success in this extreme and unstable environment. The present work aimed to assess the relative role of endogenous versus environmental control of benthic microalgal vertical migratory behaviour. This was done by comparing the patterns of vertical migration in undisturbed sediment samples kept under constant conditions of darkness and low light with those in ambient light conditions, by measuring the changes in the surface microalgal biomass during daytime low-tide periods. The results showed that the formation of a biofilm was a two-phase process. It began with a relatively small accumulation of cells at the surface, starting hours before the beginning of the light period and endogenously driven. However, the full formation of the biofilm required exposure to light by the expected beginning of the photoperiod, which further promoted upward migration and accelerated the cell accumulation at the surface. In the absence of light, upward migration was interrupted and the incipient biofilm began to disaggregate. The relative importance of the endogenously controlled behaviour varied during the spring–neap tidal cycle, reaching a maximum on those days when low tide occurred in the middle of the day, suggesting its entrainment by the duration of light exposure on previous days. The regulation of the surface cell concentration during daytime low tides was found to be strongly dependent on exogenous factors, particularly irradiance. The spontaneous disaggregation of the biofilm shortly before the end of the low-tide period (due to tidal flood or sunset), both under constant as well as ambient light conditions, suggested the presence of an endogenously controlled positive geotaxis.
Introduction
The intertidal areas of estuaries are often inhabited by communities of microalgae, the microphytobenthos, which are composed mostly of benthic diatoms, but also euglenophytes and cyanobacteria (Admiraal, Citation1984; MacIntyre et al., Citation1996; Underwood & Kromkamp, Citation1999). These photosynthetic communities form dense and highly productive biofilms at the surface of the sediment, detectable to the naked eye during daytime low tide (Consalvey et al., Citation2004). Microphytobenthic biofilms play an important role in estuarine ecosystem functioning, contributing to up to 50% of primary production (MacIntyre et al., Citation1996; Underwood & Kromkamp, Citation1999). They constitute an essential food source for grazers (Herman et al., Citation2000; Middleburg et al., Citation2000), regulate the exchange of nutrients across the sediment–water interface (MacIntyre et al., Citation1996; Tyler et al., Citation2003) and promote sediment biostabilization (Paterson, Citation1986; Yallop et al., Citation1994; de Brouwer et al., Citation2000; Gerbersdorf et al., Citation2009; Andersen et al., Citation2010; Stal, Citation2010).
The most remarkable feature of sedimentary microalgal biofilms is perhaps the rhythmic vertical migratory behaviour displayed by many species of microalgae, which is closely synchronized with solar and tidal cycles. This phenomenon has been known for a long time and consists of an upward migration of cells to the sediment surface during diurnal low tides and a downward migration shortly before tidal flood or night (Fauvel & Bohn, Citation1907; Palmer & Round, Citation1967; Admiraal, Citation1984; Consalvey et al., Citation2004; Du et al., Citation2010). This rhythmic behaviour has been considered a key adaptation to the sedimentary intertidal habitat (Serôdio & Catarino, Citation2000; Mitbavkar & Anil, Citation2004). Upward migration allows the microalgae to reach the illuminated layers of the sediment and photosynthesize, which is of crucial importance because of the periodic disturbance caused by tidal resuspension and subsequent burial in aphotic layers of the sediment (Herlory et al., Citation2004; Chul-Hwan et al., Citation2006; Mitbavkar & Anil, Citation2006). On the other hand, downward migration may help microalgae to avoid grazers (Buffan-Dubau & Carman, Citation2000), minimize resuspension into the water column (Kingston, Citation1999b ), promote access to carbon or nutrients (Barranguet et al., Citation1998; Kromkamp et al., Citation1998; Kingston, Citation1999b , Citation2002), or facilitate sexual reproduction (Saburova & Polikarpov, Citation2003). Vertical migration has also been considered as a unique form of behavioural regulation of light exposure and absorption (Admiraal, Citation1984; Perkins et al., Citation2002; Underwood, Citation2002; Serôdio et al., Citation2005). Furthermore, benthic microalgae are known to carry out what is known as ‘micro-migration’ (Kromkamp et al., Citation1998), which consists of the continuous replacement of cells within the top layers of the sediment (Perkins et al., Citation2002; Underwood et al., Citation2005).
The vertical migratory behaviour of benthic microalgae also has ecological importance, as the large changes in microalgal biomass in the photic zone of sediments are a major factor in controlling short-term variation in biofilm-level photosynthetic rates (Pinckney & Zingmark, Citation1991; Guarini et al., Citation2000; Serôdio et al., Citation2001). Moreover, migratory rhythms cause changes in the photophysiology of the cells, which affects the photosynthetic efficiency of biofilms (Forster & Kromkamp, Citation2004; Serôdio, Citation2004; Serôdio et al., Citation2005; Perkins et al., Citation2010).
Several studies have reported the endogenous nature of the vertical migratory behaviour of benthic microalgae, showing that the vertical movements continue for several days, synchronized with solar and tidal cycles, in the absence of external stimuli (Palmer & Round, Citation1967; Palmer, Citation1974; Serôdio et al., Citation1997). However, the motility of benthic microalgae also responds to exogenous cues, such as temperature (Cohn et al., Citation2003), the presence of sub-surface nutrients (Kingston, Citation2002), physical disturbance of the sediment (Hopkins, Citation1966) or wave action (Kingston, Citation1999b ), and sediment desiccation (Coelho et al., Citation2009). Of particular importance is the role of light, both incident intensity (Kingston, Citation1999a ; Serôdio et al., Citation2006; Kingston & Gough, Citation2009; McLachlan et al., Citation2009) and spectral composition (Cohn et al., Citation2004; McLachlan et al., Citation2009), which can induce upward migration and biofilm formation at the sediment surface, or conversely, promote downward movement and decrease surface biomass (Mitbavkar & Anil, Citation2004; Jesus et al., Citation2005; Serôdio et al., Citation2006; McLachlan et al., Citation2009; Perkins et al., Citation2010).
The purpose of our study was to evaluate the roles of endogenous control and exogenous environmental cues in determining the patterns of vertical migration of biofilm-forming microalgae. This was carried out by comparing the migratory behaviour of microphytobenthos samples kept under constant conditions (darkness and constant low light) with those exposed to ambient light conditions. Vertical migration was studied by monitoring the microalgal biomass present in the photic zone of the sediment over periods coinciding with daytime low tide. Two non-destructive optical techniques were used to estimate microalgal surface biomass: Pulse Amplitude Modulation (PAM) fluorometry (measuring dark fluorescence level, F o: see for notation) and Spectral Reflectance Analysis (calculating the Normalized Difference Vegetation Index, NDVI). We also investigated the entrainment of the endogenous migratory behaviour, comparing hourly patterns of vertical migration under dark, low light and ambient light conditions along spring–neap tidal cycles. The effects of vertical migration on biofilm-level photophysiology were evaluated through observation of changes in F v/F m, the maximum quantum efficiency of photosystem II (PSII) in dark and low light-exposed microphytobenthic assemblages.
Table 1. Notation used in the text.
Materials and methods
Sample collection and preparation
Samples of superficial sediment were collected during low tide on a muddy tidal flat at Vista Alegre (40° 35′ N, 8° 41′ W), Ria de Aveiro, Portugal. The sediments were composed of fine particles (97% < 63 µm) and were dominated by epipelic diatoms (Serôdio et al., Citation2008). Samples were collected on different days distributed along two spring–neap tidal cycles (five days per cycle) in October 2006 and March 2008. The upper 1 cm of sediment was collected and mixed thoroughly with a spatula before being deposited on plastic trays (20 × 40 cm) and on Plexiglas circular plates (10 cm internal diameter), to produce a slurry c. 5 cm deep. In the laboratory, the sediment samples were immersed overnight in filtered seawater in the dark. The next day, the overlying water was carefully removed so that all measurements were carried out in samples exposed to the air. Trays (three replicates) were placed outside under ambient light conditions and the plates (three replicates for each treatment) were kept in the laboratory in darkness or low light (150 µmol photons m−2 s−1). For the trays maintained outside the laboratory, measurements were begun at sunrise or at the expected arrival of the low tide at the sampling site. In the laboratory (darkness and low-light treatments), measurements were started c. 90 min before those in ambient light conditions. In all cases measurements continued throughout the period matching the daytime low tide.
Chlorophyll fluorescence
Variable chlorophyll fluorescence was measured using a fluorometer comprising a computer-operated PAM-Control Unit (Walz, Effeltrich, Germany) and a WATER-EDF-Universal emitter-detector unit (Gademann Instruments GmbH, Würzburg, Germany) (Serôdio et al., Citation2006). A blue LED-lamp (peaking at 450 nm, half-bandwidth of 20 nm) provided measuring, actinic and saturating light, conducted by a 6-mm diameter Fluid Light Guide fibre-optic. The fibre-optic was set perpendicularly to the sediment surface, at a fixed distance of 1 mm, and controlled with a micromanipulator (MM33, Märtzhäuher, Germany). Saturating pulses of 0.6 s were used to measure the minimum (F o) and maximum (F m) fluorescence levels, and subsequent determination of F v/F m. F o was used as a proxy for the microalgal biomass in the photic zone of the sediment (B) (Serôdio et al., Citation2001) in samples kept in the dark or under low light. Samples exposed to low light were dark-adapted for 2 min before the measurement of F o and F m. Fluorescence was measured every 15 min during the daytime periods corresponding to low tide at the sampling site. On each occasion, measurements were made on three separate, non-overlapping areas of sediment. On one of the samples, F o was measured continuously every 15 min during the whole night period prior to the beginning of the daytime measurements.
The relationship between F o and B (µg Chl a g−1 dry sediment) was determined using vertically homogenized sediment samples of increasing Chl a content as described by Serôdio et al. (Citation2006). A significant linear regression between the two variables (n = 40, r = 0.969; P < 0.001; after pooling data from the two sampling periods) allowed conversion of arbitrary F o signals into estimates of microalgal biomass.
Spectral reflectance
The microalgal surface biomass of the samples kept under ambient light conditions was estimated using spectral reflectance analysis. Reflectance spectra were recorded using a USB2000 spectrometer (USB2000-VIS-NIR, grating #3, Ocean Optics, Duiven, the Netherlands), connected to a 400-µm diameter fibre-optic (QP400-2-VIS/NIR-BX, Ocean Optics); measurements covered a 350–1000 nm bandwidth with a spectral resolution of 0.38 nm. Reference and dark spectra were recorded immediately before each measurement of the reflectance spectrum and spectrum measurements were carried out under variable ambient light conditions. Reference spectra were obtained by measuring the spectrum reflected by a surface of standard reflectance (WS-1-SL Spectralon Reference Standard, Ocean Optics) placed under identical sunlight exposure conditions. A dark reflectance spectrum was subtracted from both sample and reference spectra to account for the dark current noise of the spectrometer.
The incident solar irradiance at the time of each measurement was recorded using a PAR sensor (LI-193SA and LI-250 light meter, Li-Cor, Lincoln, Nebraska, USA). Three replicated spectra were measured every 15 min on separate, non-overlapping areas, and the mean spectrum was smoothed using a 10-point moving average filter before further analysis. The fibre-optic was positioned perpendicularly to the sample surface at such a distance that a circular area c. 10 cm in diameter was monitored. The surface microalgal biomass was estimated using the normalized vegetation index NDVI (Rouse et al., Citation1973), calculated by:
Migration amplitude
To compare migratory behaviour between samples, sampling dates and endogenous vs. environmental control, the pattern of vertical migration during each low tide period was characterized by calculating the maximum relative change in surface biomass, or migration amplitude, given by:
For those samples kept in the dark or under low light, the rate of variation of surface biomass, ΔB/Δt, was calculated for the periods of biofilm formation and disaggregation. ΔB/Δt was also calculated for the periods immediately before and after the biomass maximum in samples in the dark, while for the samples exposed to low light it was calculated for the periods after the beginning of the light period and before the end of the light period. ΔB/Δt was calculated from the slope of the variation of B over periods ranging from 45 min to 2 h.
Results
Endogenous control: darkness
In the samples kept in the dark, surface biomass varied according to a pattern consistently repeated throughout the whole spring–neap tidal cycle (). It was characterized by an asymmetrical peak resulting from a rapid increase in microalgal concentration at the sediment surface (varying from 62.8 to 463.3 µg Chl a g−1 dry sediment h−1; ) for at least 2–3 h, followed by a slower decrease (on average, 65.0% of the previous rate of accumulation) to virtually constant levels during the rest of the subjective photoperiod. No changes in microalgal surface biomass were observed during the night, including any periods of low tide (data not shown). Migration amplitude varied during the spring–neap cycle, with maximum values on days when low tide occurred in the middle of the day (), and minimum values when light exposure took place during the early morning or late afternoon (). Despite this fortnightly variation in migration amplitude, the maximum surface biomass was always reached at times (tB max) soon (<2 h) after the beginning of light exposure at the sampling site (t i). A significant correlation was found between tB max and t i (; r = 0.966; P < 0.001). Further analysis showed that the highest coincidence between tB max and t i took place when the low tide exposure began in the middle of the day. Furthermore, the maximum in surface biomass occurred later than the beginning of low tide (tB max > t i) when the low tide exposure started early in the morning, and slightly later (tB max < t i) when it started during the afternoon ().
Fig. 1. Hourly variation of surface microalgal biomass (B: µg Chl a g−1 dry sediment), and maximum quantum efficiency of PSII, F v/F m, in sediment samples kept in darkness and exposed to low light (150 µmol m−2 s−1), during five days during one spring–neap tidal cycle in October 2006. Vertical dotted lines define the exposure to low light. Mean values of three replicates. Error bars: 1 standard error.
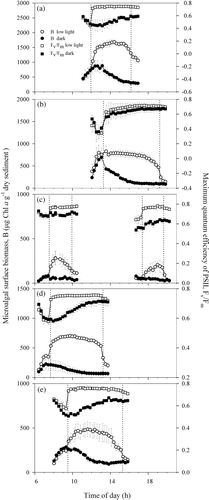
Fig. 2. Linear relationship between time for maximum in surface biomass, t Bmax, in samples kept in the dark (h) and t i, the expected time of the start of the daytime emersion period in the sampling site (h).
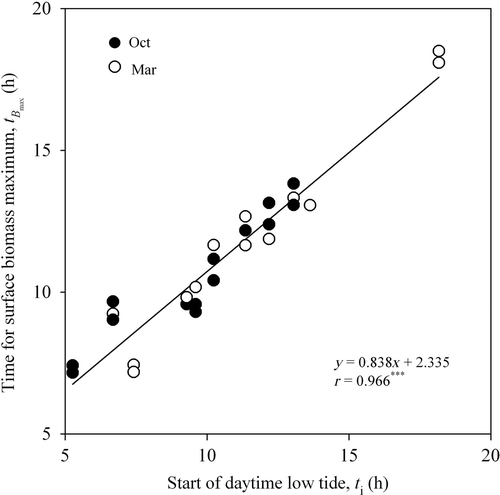
Table 2. Rates of variation of surface biomass (ΔB/Δt, µg Chl a g−1 dry sediment h−1) during upward and downward migration for the samples kept in the dark and exposed to low light shown in (days identified by the letter identifying each panel of figure). For the second low tide period of day c, rates could not be calculated as there was no initial increase in surface biomass.
Endogenous control: low light
The exposure of samples to low light at time t i caused a marked increase in the rate of microalgal accumulation at the sediment surface, to values of 65.0–1052.3 µg Chl a g−1 dry sediment h−1 (), 155% higher on average than the previous rate of increase in the dark. This caused a marked difference between the temporal patterns of biomass accumulation in dark- and low light-exposed samples (). The acceleration in microalgal accumulation at the surface was observed repeatedly during the spring–neap cycle, and was followed by a prolonged phase (>4 h) around the middle of the subjective low-tide period when microalgal biomass reached maximum values and remained approximately constant. The exceptions were on days when the period of light exposure was very short, due to low tide taking place in early morning or late afternoon (). The rates of decrease of surface biomass at the end of the light period were comparable to the rates of increase observed earlier in the same low-tide period, the difference being on average much smaller (17.0%) than those recorded for samples kept in the dark. Biomass started to decrease approximately 1–2 h before the end of low-tide light period (). As with samples kept in the dark, the migration amplitude under constant low light varied during the spring–neap cycle, with maximum values being recorded for those days when the light period occurred in the middle of the day ().
Endogenous control: effects on photosynthetic efficiency
In samples kept in the dark, F v/F m showed marked variation around t i, displaying a pattern of variation almost inversely symmetrical to the one displayed by biomass (). A rapid increase in surface biomass was generally followed by a steep decrease in F v/F m (from ∼0.75 to 0.4–0.6) at times closely matching the biomass maxima (e.g. ). The subsequent slow decrease in biomass was paralleled by an identically slow return of F v/F m to initial levels. The variation of F v/F m around t i varied between days, with larger decreases and subsequent recoveries being recorded for those days when low tide occurred at midday. However, despite this fortnightly variability, the inverse relationship between B and F v/F m was maintained throughout the spring–neap cycle, and the two parameters were found to be significantly correlated, either when all data were pooled (n = 153, r = −0.837; P < 0.001) or when treating each day separately (, n = 24, r = −0.957; , n = 31, r = −0.916; morning, n = 17, r = −0.779; afternoon, n = 16, r = −0.861; , n = 31, r = −0.980; , n = 34, r = −0.943; P < 0.001 in all cases).
In samples kept under constant low light, there was a rapid increase of F v/F m during transition from dark to light, reaching a maximum shortly after the beginning of the illumination period (e.g. ). This increase was always much faster than for surface biomass during the same periods. In dark-adapted samples F v/F m took several hours to recover to initial values, while in samples exposed to low light F v/F m remained virtually constant throughout the whole photoperiod, showing only a slight decrease when biomass started to decline (e.g. ).
Environmental control
shows the hourly variation of surface microalgal biomass and incident irradiance (PAR, µmol m2 s1) on selected days during the two studied spring–neap cycles. The highest PAR levels were recorded in March, with c. 1500 µmol m−2 s−1 being recorded on four out of five days. The surface microalgal biomass in those samples exposed to natural environmental conditions was generally more variable and irregular than in samples kept in the dark or low light (). In most cases, surface biomass reached values intermediate between dark- and low-light-treated samples (e.g. ). Some exceptions to this pattern were probably related to incident PAR. When PAR reached very high levels, biomass values remained below those of samples kept in the dark (). When PAR levels were low, biomass attained values higher than those of samples kept under low light (). The example shown in , where surface biomass under high irradiance levels transiently exceeded values recorded under low light, suggests a possible role of factors other than incident irradiance, such as desiccation or previous light history.
Fig. 3. Hourly variation of surface biomass (B: µg Chl a g−1 dry sediment) and PAR (µmol m−2 s−1) for the sediment samples kept under ambient light conditions and constant conditions (dark and low light), during five days during two spring–neap tidal cycles (a–e: October 2006; f–j: March 2008). Mean values of three replicates. Error bars: 1 standard error.
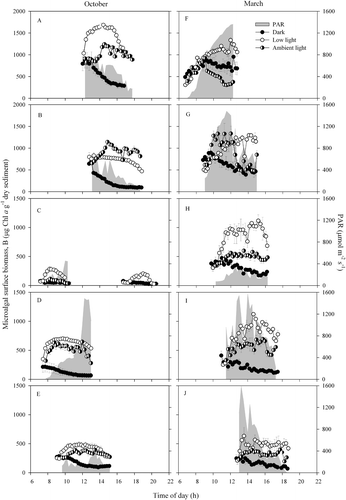
Variation over the spring–neap tidal cycle: entrainment of the migratory rhythm
Samples exposed to constant conditions (dark or low light) showed a well-defined fortnightly variation in migration amplitude, increasing as the time of low tide, t lt, shifted from sunrise to midday, and gradually decreasing as t lt occurred later in the day (). Maximum values of ΔB max were recorded around noon and the lowest were during early-morning and late-afternoon low tides, on days with two daytime emersion periods. In samples exposed to ambient light conditions, the pattern of variation during the spring–neap cycle was more irregular ().
Discussion
Biofilm formation
The results of this study indicate that the upward migration at the beginning of each diurnal low tide and the formation of the microalgal biofilm is a two-phase process, the first being fully endogenously controlled and the second being driven by environmental factors, especially light. The initial phase is an upward migration pulse beginning at least 2–3 h before the daytime emersion period and ending around the time when light exposure is expected to begin (t i). Previous results that showed a peak of surface biomass occurring sometime after the beginning of tidal exposure (tB max > t i) (Serôdio et al., Citation1997, Citation2005) appear to be a particular case of the general pattern described here, since both studies refer to days when light exposure started with sunrise.
Considering the lack of physical (light, temperature) or chemical gradients at the sediment–air interface (after prolonged darkness, the upper and lower layers both become uniformly anoxic), the accumulation of cells at the surface could be explained by an endogenous negative geotactic behaviour (Harper, Citation1977; Mitbavkar & Anil, Citation2004). This process would allow the microalgae to reach the vicinity of the photic zone, where they could ‘expect’ to become exposed to light as the sun rose or the tide ebbed. This would have an obvious adaptive value, allowing periods favourable for photosynthesis to be anticipated and fully exploited.
However, our results also show that, if no light reaches the sediment surface by t i, the initial surface accumulation of cells is reversed and the transient biofilm formed by then begins to disaggregate, as shown by experiments in continuous darkness. The rate of decrease of surface biomass observed during this period is low, compared to the rate of increase before t i (or to the downward migration at the end of the low-tide light period, see below), which suggests that cell movements are not synchronized or oriented downwards. The simplest explanation is that the observed decrease in surface biomass results from the randomly oriented movement of cells in the upper sediment layers.
The second phase of biofilm formation process is thus a switch from the initial endogenous stimulus to a strong (although light intensity-dependent) positive phototaxis, resulting in upward migration towards the sediment surface. In favourable conditions (such as low light) at time t i, microalgae continue to accumulate in the surficial layers, reaching maximum concentrations typically within 1–2 h. This finding apparently agrees with the results of Round & Palmer (Citation1966) who reported that a 2-h period of light exposure was required before biofilm formation. The acceleration often observed in surface biomass accumulation when the light was switched on (e.g. ) may have resulted in part from the sudden transition from darkness to the favourable light exposure. However, the light requirement for full formation of the biofilm is confirmed by the results found for those samples exposed to natural light ().
On the other hand, the hourly variation of biofilm-level F v/F m suggests that the exposure to light of cells arriving at the sediment surface induces important changes at the physiological level. The rapid and large increase of F v/F m to maximum (and thereafter virtually constant) values is consistent with the well-known dissipation of non-photochemical quenching (NPQ) induced by low light that can occur in diatoms after prolonged darkness (Arsalane et al., Citation1994; Olaizola et al., Citation1994; Muller et al., Citation2001; Jesus et al., Citation2006; Serôdio et al., Citation2006). NPQ induces a decrease in F v/F m and its build-up in diatoms in the dark has been attributed to processes leading to the formation of a proton gradient across the thylakoid membrane, probably chlororespiration (Ting & Owens, Citation1993; Jakob et al., Citation1999; Lavaud et al., Citation2002). The decrease in F v/F m that was observed as microalgae accumulated at the surface in the dark may be the result of the operation of chlororespiration as cells come in contact with atmospheric oxygen, or the manifestation of an endogenous physiological rhythm affecting the transthylakoid proton gradient (Serôdio et al., Citation2005). However, considering the strong agreement between the increase of surface biomass and the simultaneous decrease of F v/F m, as well as the long periods required for the build-up of significant NPQ in the dark (Jakob et al., Citation1999), it seems more likely that the observed sharp drop in biofilm-level F v/F m is caused by the accumulation in the photic zone of diatoms having a large NPQ, formed during the residence of these diatoms in dark layers of the sediment during the previous night or high tide. If so, the return of biofilm F v/F m to their initial values as surface biomass decreases would imply that the same cell population undergoes both upward and downward migration. The change in the transthylakoidal proton gradient as the microalgae cells reach the illuminated layers of the sediment may play a role in the detection of light gradients and the reinforcement of their phototactic movement.
Migratory responses of the biofilm during light exposure
Once close to the surface, microalgae become directly exposed to a range of highly variable or extreme conditions characteristic of intertidal habitats during low tide, including high levels of solar irradiance, extremes of temperature and salinity, and desiccation. Our results suggest that exogenous stimuli do strongly affect the patterns of microalgae vertical migration and can override the endogenous behaviour observed under constant conditions. This could allow the microalgae to respond quickly to adverse conditions by migrating downwards and seeking refuge in subsurface layers of sediment.
Although the individual effects of different environmental factors could not be ascertained, most of the differences between the migratory patterns recorded on samples exposed to ambient light conditions and to constant conditions could be related to ambient light intensity. Under low ambient light, early in the morning or late in the afternoon, or during cloudy days, benthic microalgae accumulated at the surface; biofilm biomass reached values comparable to those measured under constant low light in the laboratory and remained constant over the duration of these favourable periods. In contrast, during periods of high solar irradiance, surface biomass often decreased rapidly to levels below those measured in the laboratory, indicating strong negative phototaxis. The migratory response to changes in ambient light observed in this study, characterized by accrual of surface biomass under low light (c. 150 µmol m−2 s−1) and a gradual decrease for higher levels, is in agreement with results obtained using biomass light-response curves generated in the laboratory (Kingston, Citation1999a ; Serôdio et al., Citation2006; McLachlan et al., Citation2009), and illustrate the role of motility in regulating light exposure by diatoms (Cohn et al., Citation2001).
Occasionally, biomass levels remained relatively high, which may indicate the role of factors other than incident irradiance. Changes in the taxonomic composition of the biofilm could explain the differences in migratory behaviour that might have occurred within each fortnightly cycle. Alternatively, these results could be due to micro-migration, where microalgae migrate downwards to avoid locally unfavourable conditions and are replaced by others coming from subsurface layers (Kromkamp et al., Citation1998; Perkins et al., Citation2002; Underwood et al., Citation2005; Perkins et al., Citation2010). This process is expected when biofilms are exposed to high light for prolonged periods, causing limitation or photoinhibition of photosynthesis, and could explain the maintenance of high biomass levels at the surface during exposure to high light levels.
Biofilm disaggregation
The spontaneous disaggregation of the biofilm by synchronized downward migration of most of the cells, anticipating tidal flood or night, is a well-known feature of the migratory behaviour of microphytobenthos (Consalvey et al., Citation2004). The present observation that, under constant low light conditions, surface biomass starts to decrease shortly before the expected end of the subjective low tide period, strongly suggests the presence of an endogenous control, possibly associated with positive geotaxis. The reasons are, first, that the rate of decrease observed at the end of the daytime low tide was comparable to the initial increase in low light samples, suggesting downward migration rather than a dispersion resulting from randomly oriented cell movements. Second, the endogenous nature of this behaviour is supported by the consistent observation that it takes place under constant conditions, its timing being clearly an anticipation of incoming flood or sunset, independent of the duration of light exposure period (Paterson, Citation1986; Serôdio et al., Citation1997). Finally, the strongest response is induced when the biofilm is in the dark and no light gradients are present (Consalvey et al., Citation2004; Mitbavkar & Anil, Citation2004; Cartaxana & Serôdio, Citation2008). The alternative hypothesis, i.e. negative phototaxis, is unlikely because, in diatoms, it has only been documented in strong light (Serôdio et al., Citation2006; McLachlan et al., Citation2009).
Entrainment of the endogenous migratory rhythm
Assuming that, under constant conditions, migration amplitude is an indicator of the degree of entrainment of endogenous migratory behaviour, the pattern of variation of ΔB max indicates strongly that this entrainment is related to variations in the timing and duration of low-tide exposure periods during the spring–neap cycle. ΔB max varied periodically over the fortnightly tidal cycle, initially increasing with the length of light exposure period in the previous days, and decreasing as the light period available before sunset or tidal flood is shortened from each day to the next. The magnitude of ΔB max reached each day seems thus to be dependent on both the length of the light period until the time of day corresponding to ΔB max (tB max) is reached, and the time between tB max and flood or sunset.
The increase of migration amplitude with increased duration of light exposure periods during the preceding days indicates that the endogenous component of the migratory behaviour gets stronger as the time of low tide advances during the day. Exposure to longer periods of light as the time of low tide progresses during the spring–neap cycle causes progressive reinforcement of the endogenous behaviour, leading to a larger fraction of the microalgal population undergoing endogenously driven vertical movements.
On the other hand, when sunset begins to occur before the end of the low-tide periods, microalgae are induced to migrate downwards in response to darkening. This event seems to be memorized so that on the next day downward migration anticipates the approach of night. As a result, the amplitude of the endogenous migration decreases as the light period is increasingly truncated by sunset.
Acknowledgements
This work was supported by FCT – Fundação para a Ciência e Tecnologia through PhD grant SFRH/BD/23720/2005 (H. Coelho) and project ‘BenthicLink – Trophic links regulated by tidal and daily rhythms: benthic microflora and fauna in estuaries’, (POCI/BIA-BDE/61977/2004). Both financial supports were allocated by FCT under the Support Community Framework III, Operational Programme Science, Technology and Innovation. We thank two anonymous reviewers for critical comments on the manuscript.
References
- Admiraal , W . 1984 . The ecology of estuarine sediment-inhabiting diatoms . Progr. Phycol. Res. , 3 : 269 – 323 .
- Andersen , TJ , Lanuru , M , van Bernem , C , Pejrup , M and Riethmueller , R . 2010 . Erodibility of a mixed mudflat dominated by microphytobenthos and Cerastoderma edule, East Frisian Wadden Sea, Germany . Estuar. Coast. Shelf Sci. , 87 : 19 – 206 .
- Arsalane , W , Rousseau , B and Duval , J-C . 1994 . Influence of the pool size of the xanthophyll cycle on the effects of light stress in a diatom: competition between photoprotection and photoinhibition . Photochem. Photobiol. , 60 : 237 – 243 .
- Barranguet , C , Kromkamp , J and Peene , J . 1998 . Factors controlling primary production and photosynthetic characteristics of intertidal microphytobenthos . Mar. Ecol. Prog. Ser. , 173 : 117 – 126 .
- Buffan-Dubau , E and Carman , KR . 2000 . Diel feeding behavior of meiofauna and their relationships with microalgal resources . Limnol. Oceanogr. , 45 : 381 – 395 .
- Cartaxana , P and Serôdio , J . 2008 . Inhibiting diatom motility: a new tool for the study of the photophysiology of intertidal microphytobenthic biofilms . Limnol. Oceanogr. Methods , 6 : 466 – 476 .
- Chul-Hwan , K , Jong Seong , K , Hiroyuki , A , Hiroyuki , Y , Hiroyuki , M and Kenichi , K . 2006 . Tidal resuspension of microphytobenthic chlorophyll a in a Nanaura mudflat, Saga, Ariake Sea, Japan: flood-ebb and spring-neap variations . Mar. Ecol. Prog. Ser. , 312 : 85 – 100 .
- Coelho , H , Vieira , S and Serôdio , J . 2009 . Effects of desiccation on the photosynthetic activity of intertidal microphytobenthos biofilms as studied by optical methods . J. Exp. Mar. Biol. Ecol. , 381 : 98 – 104 .
- Cohn , SA , Bahena , M , Davis , JT , Ragland , RL , Rauschenberg , CD and Smith , BJ . 2004 . Characterisation of the diatom photophobic response to high irradiance . Diatom Res. , 19 : 167 – 179 .
- Cohn , SA , Donat , PH and Breure , AM . 2001 . “ Photo-stimulated effects on diatom motility ” . In Comprehensive Series in Photosciences , Edited by: Häder , D-P and Breure , AM . 375 – 401 . Amsterdam : Elsevier .
- Cohn , SA , Farrell , JF , Munro , JD , Ragland , RL , Weitzell , RE and Wibisono , BL . 2003 . The effect of temperature and mixed species composition on diatom motility and adhesion . Diatom. Res. , 18 : 225 – 243 .
- Consalvey , M , Paterson , DM and Underwood , GJC . 2004 . The ups and downs of life in a benthic biofilm: migration of benthic diatoms . Diatom Res. , 19 : 181 – 202 .
- De Brouwer , JFC , Bjelic , S , De Deckere , E and Stal , LJ . 2000 . Interplay between biology and sedimentology in a mudflat (Biezelingse Ham, Westerschelde, the Netherlands) . Cont. Shelf Res. , 20 : 1159 – 1177 .
- Du , GY , Son , M , An , S and Chung , IK . 2010 . Temporal variation in the vertical distribution of microphytobenthos in intertidal flats of the Nakdong River estuary, Korea . Estuar. Coast. Shelf Sci. , 86 : 62 – 70 .
- Fauvel , P and Bohn , G . 1907 . Le rhythme des marées chez les diatomées litorales . C. R. Séanc. Soc. Biol. , 62 : 121 – 123 .
- Forster , RM and Kromkamp , JC . 2004 . Modelling the effects of chlorophyll fluorescence from subsurface layers on photosynthetic efficiency measurements in microphytobenthic algae . Mar. Ecol. Prog. Ser. , 284 : 9 – 22 .
- Gerbersdorf , SU , Bittner , R , Lubarsky , H , Manz , W and Paterson , DM . 2009 . Microbial assemblages as ecosystem engineers of sediment stability . J. Soil Sediment. , 9 : 640 – 652 .
- Guarini , JM , Blanchard , GF , Gros , P , Gouleau , D and Bacher , C . 2000 . Dynamic model of the short-term variability of microphytobenthic biomass on temperate intertidal mudflats . Mar. Ecol. Prog. Ser. , 195 : 291 – 303 .
- Harper , MA . 1977 . “ Movements ” . In The Biology of Diatoms , Edited by: Werner , D . 224 – 249 . Oxford : Blackwell Scientific Publications .
- Herlory , O , Guarini , JM , Richard , P and Blanchard , GF . 2004 . Microstructure of microphytobenthic biofilm and its spatio-temporal dynamics in an intertidal mudflat (Aiguillon Bay, France) . Mar. Ecol. Prog. Ser. , 282 : 33 – 44 .
- Herman , PMJ , Middelburg , JJ , Widdows , J , Lucas , CH and Heip , CHR . 2000 . Stable isotopes as trophic tracers: combining field sampling and manipulative labelling of food resources for macrobenthos . Mar. Ecol. Prog. Ser. , 204 : 79 – 92 .
- Hopkins , JT . 1966 . The role of water in the behaviour of an estuarine mud-flat diatom . J. Mar. Biol. Assoc. U.K. , 46 : 617 – 626 .
- Jakob , T , Goss , R and Wilhelm , C . 1999 . Activation of diadinoxanthin de-epoxidase due to a chlororespiratory proton gradient in the dark in the diatom Phaeodactylum tricornutum . Plant Biol. , 1 : 76 – 82 .
- Jesus , B , Brotas , V , Marani , M and Paterson , DM . 2005 . Spatial dynamics of microphytobenthos determined PAM fluorescence . Estuar. Coast. Shelf Sci. , 65 : 30 – 42 .
- Jesus , B , Perkins , RG , Mendes , CR , Brotas , V and Paterson , DM . 2006 . Chlorophyll fluorescence as a proxy for microphytobenthic biomass: alternatives to the current methodology . Mar. Biol. , 150 : 17 – 28 .
- Kingston , MB . 1999a . Effect of light on vertical migration and photosynthesis of Euglena proxima (Euglenophyta) . J. Phycol. , 35 : 245 – 253 .
- Kingston , MB . 1999b . Wave effects on the vertical migration of two benthic microalgae: Hantzschia virgata var . intermedia and Euglena proxima. Estuaries , 22 : 81 – 91 .
- Kingston , MB . 2002 . Effect of subsurface nutrient supplies on the vertical migration of Euglena proxima (Euglenophyta) . J. Phycol. , 38 : 872 – 880 .
- Kingston , MB and Gough , JS . 2009 . Vertical migration of a mixed-species Euglena (Euglenophyta) assemblage inhabiting the high-intertidal sands of Nye beach, Oregon . J. Phycol. , 45 : 1021 – 1029 .
- Kromkamp , J , Barranguet , C and Peene , J . 1998 . Determination of microphytobenthos PSII quantum efficiency and photosynthetic activity by means of variable chlorophyll fluorescence . Mar. Ecol. Prog. Ser. , 162 : 45 – 55 .
- Lavaud , J , Rousseau , B , van Gorkom , HJ and Etienne , AL . 2002 . Influence of the diadinoxanthin pool size on photoprotection in the marine planktonic diatom Phaeodactylum tricornutum . Plant Physiol. , 129 : 1398 – 1406 .
- MacIntyre , HL , Geider , RJ and Miller , DC . 1996 . Microphytobenthos: The ecological role of the “Secret Garden” of unvegetated, shallow-water marine habitats. I. Distribution, abundance and primary production . Estuaries , 19 : 186 – 201 .
- McLachlan , DH , Brownlee , C , Taylor , AR , Geider , RJ and Underwood , GJC . 2009 . Light-induced motile responses of the estuarine benthic diatoms Navicula perminuta and Cylindrotheca closterium (Bacillariophyceae) . J. Phycol. , 45 : 592 – 599 .
- Middleburg , JJ , Barranguet , C , Boschker , HTS , Herman , PMJ , Moens , T and Heip , CHR . 2000 . The fate of intertidal microphytobenthos carbon: an in situ 13C-labeling study . Limnol. Oceanogr. , 45 : 1224 – 1234 .
- Mitbavkar , S and Anil , A . 2006 . Diatoms of the microphytobenthic community in a tropical intertidal sand flat influenced by monsoons: spatial and temporal variations . Mar. Biol. , 148 : 693 – 709 .
- Mitbavkar , S and Anil , AC . 2004 . Vertical migratory rhythms of benthic diatoms in a tropical intertidal sand flat: influence of irradiance and tides . Mar. Biol. , 145 : 9 – 20 .
- Muller , P , Li , X-P and Niyogi , KK . 2001 . Non-photochemical quenching. A response to excess light energy . Plant Physiol. , 125 : 1558 – 1566 .
- Olaizola , M , Roche , J , Kolber , Z and Falkowski , PG . 1994 . Non-photochemical fluorescence quenching and the diadinoxanthin cycle in a marine diatom . Photosynth. Res. , 41 : 357 – 370 .
- Palmer , JD . 1974 . Biological clocks in marine organisms: the control of physiological and behavioural tidal rhythms , New York : John Wiley .
- Palmer , JD and Round , FE . 1967 . Persistent, vertical-migration rhythms in benthic microflora. VI. The tidal and diurnal nature of the rhythm in the diatom Hantzschia virgata . Biol. Bull. ( Woods Hole ) , 132 : 44 – 55 .
- Paterson , DM . 1986 . The migratory behaviour of diatom assemblages in a laboratory tidal micro-ecosystem examined by low-temperature scanning electron microscopy . Diatom Res. , 1 : 227 – 239 .
- Perkins , RG , Oxborough , K , Hanlon , ARM , Underwood , GJC and Baker , NR . 2002 . Can chlorophyll fluorescence be used to estimate the rate of photosynthetic electron transport within microphytobenthic biofilms? . Mar. Ecol. Prog. Ser. , 228 : 47 – 56 .
- Perkins , RG , Lavaud , J , Serôdio , J , Mouget , J-L , Cartaxana , P , Rosa , P , Barrillé , L , Brotas , V and Jesus , BM . 2010 . Vertical cell movement is the primary response of intertidal benthic biofilms to increasing light dose . Mar. Ecol. Prog. Ser. , 416 : 93 – 103 .
- Pinckney , J and Zingmark , RG . 1991 . Effects of tidal stage and sun angles on intertidal benthic microalgal productivity . Mar. Ecol. Prog. Ser. , 76 : 81 – 89 .
- Round , FE and Palmer , JD . 1966 . Persistent, vertical-migration rhythms in benthic microflora.: II. Field and laboratory studies on diatoms from the Banks of the River Avon . J. Mar. Biol. Assoc. U.K. , 46 : 191 – 214 .
- Rouse , JW , Haas , RH , Schell , JA and Deering , DW . 1973 . “ Monitoring vegetation systems in the great plains with ERTS ” . In Third Earth Resources Technology Satellite Symposium , Edited by: Freden , SC , Mercanti , EP and Becker , M . 309 – 317 . Washington, DC : NASA .
- Saburova , MA and Polikarpov , IG . 2003 . Diatom activity within soft sediments: behavioural and physiological processes . Mar. Ecol. Prog. Ser. , 251 : 115 – 126 .
- Serôdio , J . 2004 . Analysis of variable chlorophyll fluorescence on microphytobenthos assemblages: implications of the use of depth-integrated measurements . Aquat. Microb. Ecol. , 36 : 137 – 152 .
- Serôdio , J and Catarino , F . 2000 . Modelling the primary productivity of intertidal microphytobenthos: time scales of variability and effects of migratory rhythms . Mar. Ecol. Prog. Ser. , 192 : 13 – 30 .
- Serôdio , J , Coelho , H , Vieira , S and Cruz , S . 2006 . Microphytobenthos vertical migratory photoresponse as characterised by light-response curves of surface biomass . Estuar. Coast. Shelf Sci. , 68 : 547 – 556 .
- Serôdio , J , da Silva , JM and Catarino , F . 1997 . Nondestructive tracing of migratory rhythms of intertidal benthic microalgae using in vivo chlorophyll a fluorescence . J. Phycol. , 33 : 542 – 553 .
- Serôdio , J , da Silva , JM and Catarino , F . 2001 . Use of in vivo chlorophyll a fluorescence to quantify short-term variations in the productive biomass of intertidal microphytobenthos . Mar. Ecol. Prog. Ser. , 218 : 45 – 61 .
- Serôdio , J , Vieira , S and Cruz , S . 2008 . Photosynthetic activity, photoprotection and photoinhibition in intertidal microphytobenthos as studied in situ using variable chlorophyll fluorescence . Cont. Shelf Res. , 28 : 1363 – 1375 .
- Serôdio , J , Vieira , S , Cruz , S and Barroso , F . 2005 . Short-term variability in the photosynthetic activity of microphytobenthos as detected by measuring rapid light curves using variable fluorescence . Mar. Biol. , 146 : 903 – 914 .
- Stal , LJ . 2010 . Microphytobenthos as a biogeomorphological force in intertidal sediment stabilization . Ecol Eng. , 36 : 236 – 245 .
- Ting , CS and Owens , TG . 1993 . Photochemical and nonphotochemical fluorescence quenching processes in the diatom Phaeodactylum tricornutum . Plant Physiol. , 101 : 1323 – 1330 .
- Tyler , AC , McGlathery , KJ and Anderson , IC . 2003 . Benthic algae control sediment: water column fluxes of organic and inorganic nitrogen compounds in a temperate lagoon . Limnol. Oceanogr. , 48 : 2125 – 2137 .
- Underwood , GJC . 2002 . Adaptations of tropical marine microphytobenthic assemblages along a gradient of light and nutrient availability in Suva Lagoon, Fiji . Eur. J. Phycol. , 37 : 449 – 462 .
- Underwood , GJC , Perkins , RG , Consalvey , MC , Hanlon , A , Oxborough , K , Baker , N and Paterson , DM . 2005 . Patterns in microphytobenthic primary productivity: Species-specific variation in migratory rhythms and photosynthetic efficiency in mixed-species biofilms . Limnol. Oceanogr. , 50 : 755 – 767 .
- Underwood , GJC and Kromkamp , J . 1999 . “ Primary production by phytoplankton and microphytobenthos in estuaries ” . In Advances in Ecological Research , Edited by: Woodward , G . 93 – 153 . Amsterdam : Elsevier .
- Yallop , ML , de Winder , B , Paterson , DM and Stal , LJ . 1994 . Comparative structure, primary production and biogenic stabilization of cohesive and non-cohesive marine sediments inhabited by microphytobenthos . Estuar. Coast. Shelf Sci. , 39 : 565 – 582 .