Abstract
The Verrucariaceae (Ascomycota) is a family of mostly lichenized fungi with a unique diversity of algal symbionts, including some algae that are rarely or never associated with other lichens. The phylogenetic position of most of these algae has not yet been studied and, because morphology-based identifications can often be misleading, molecular data is necessary to revisit their identity and to explore patterns of association between fungal and algal partners. For this reason, the diversity of photobionts in this lichen family was investigated using molecular markers (rbcL and nuSSU) amplified from DNA extracts of lichen thalli and cultured isolates. Although a single algal genus, Diplosphaera (Trebouxiophyceae), was associated with 12 out of the 17 sampled genera of Verrucariaceae, representatives of eight other genera in five orders of the Chlorophyta and one genus in the Xanthophyceae also form lichen associations with members of the family. Fungal genera with simple crustose thalli (e.g. Hydropunctaria, Wahlenbergiella, Bagliettoa) use a high diversity and unusual selection of photobionts. In contrast, fungal genera with more complex thalli (e.g. Placidium, Dermatocarpon) tend to have lower photobiont diversity. Habitat requirements and phylogenetic histories are both partly reflected in the observed patterns of associations between lichenized fungi from the family Verrucariaceae and their photobionts.
Introduction
Eukaryotic algae and cyanobacteria are common components of symbioses, especially in mutualistic associations, where they provide a constant and stable source of carbohydrates to their heterotrophic symbionts. Although approximately 40 phototrophic genera have been recorded as symbionts (photobionts) of lichens, most lichenized fungi associate primarily with only two groups of chlorophyte algae, the trebouxiophycean genera Trebouxia and Asterochloris and the ulvophycean order Trentepohliales (genera Trentepohlia, Phycopeltis and Cephaleuros), as well as two genera of Cyanobacteria, Nostoc and Rhizonema (Tschermak-Woess, Citation1988; Friedl & Büdel, Citation2008; Lücking et al., Citation2009; Skaloud & Peksa, Citation2010). As a result, broad trends of associations between lichen mycobionts and photobionts can be discerned at a high phylogenetic level (Tschermak-Woess, Citation1988; Rambold et al., Citation1998; Persoh et al., Citation2004; Miadlikowska et al., Citation2006; Friedl & Büdel, Citation2008). For example, in Lecanorales and Teloschistales, the most species-rich orders of lichenized fungi, Trebouxia and Asterochloris are the predominant photobionts. Similarly, nearly all Peltigerales species associate with Nostoc, and tropical lichen orders such as Arthoniales, Ostropales, Pyrenulales and Trypetheliales tend to associate preferentially with Trentepohliales.
A very different trend in mycobiont–photobiont associations can be found in the family Verrucariaceae, where a remarkable number of algal genera can be found. Morphology-based studies have shown that the most common lichen photobionts mentioned above are only rarely or never reported as symbiotic partners of the Verrucariaceae (summarized in Tschermak-Woess, Citation1988). Instead, the Verrucariaceae form associations with a plethora of other algae. Stichococcus-like green algae (‘Protococcus spp.’, Stichococcus spp. and Diplosphaera spp., Trebouxiophyceae) seem to be the most common photobionts in this lichen family, but the morphological circumscription of these algal taxa is unclear and their genetic diversity has never been studied. Other Trebouxiophyceae that have been reported to associate with species of Verrucariaceae include Auxenochlorella, Chlorella, Coccobotrys, Myrmecia and Trochiscia (Tschermak, Citation1941a ; Tschermak-Woess, Citation1988; Nyati et al., Citation2007). Amphibious Verrucariaceae from both saline and freshwater habitats have repeatedly been reported in association with Dilabifilum, a genus of Ulvophyceae (Tschermak-Woess, Citation1970, Citation1976; Thüs, Citation2002). In addition, the Verrucariaceae is the only mostly lichenized family to form associations with Xanthophyceae (Heterococcus caespitosus Vischer: Tschermak, Citation1941b ; Zeitler, Citation1954; Parra & Redon, Citation1977; Tschermak-Woess, Citation1988; Thüs & Schultz, Citation2008), Phaeophyceae [Petroderma maculiforme (Wollny) Kuckuck: Moe, Citation1997; Peters & Moe, Citation2001; Sanders et al., Citation2004, Citation2005; Ascophyllum nodosum (Linnaeus) Le Jolis and Pelvetia canaliculata (Linnaeus) Decaisne & Thuret: Kohlmeyer & Volkmann-Kohlmeyer, Citation1998 and Rhodophyta (Apophlaea sp.: Kohlmeyer & Volkmann-Kohlmeyer, Citation1998). In most lichens, the symbiotic alga is embedded in a fungal tissue forming a lichen thallus that is dominated by the mycobiont. However, in the Verrucariaceae several fungal species are found in association with thalloid algae, such as the brown algae Pelvetia and Ascophyllum (Kohlmeyer & Volkmann-Kohlmeyer, Citation1998), and the green alga Prasiola crispa (Lightfoot) Kützing (Pérez-Ortega et al., Citation2010). In these associations the mycobiont grows within the independently formed algal thallus. Although the Prasiola photobiont of the species of Verrucariaceae Mastodia tessellata (Hooker f. & Harvey) Hooker f. & Harvey has recently been characterized (Pérez-Ortega et al., Citation2010), little is known about the photobiont identity of its close relatives found in typical fungus-dominated lichen thalli.
The factors responsible for the high photobiont phylogenetic diversity observed in the Verrucariaceae have been addressed only briefly and with contradictory conclusions. Following the observation that several amphibious species of Verrucariaceae are associated with Dilabifilum, Tschermak-Woess (Citation1976) suggested that selection for the same photobiont could be a character reflecting phylogenetic relatedness between species of lichen-forming fungi. However, it has not been tested whether all Verrucariaceae species with a Dilabifilum photobiont belong to a monophyletic group. Whether photobiont selection in Verrucariaceae reflects coincident ecological requirements of the two symbionts or whether it results from clade-specific preferences of Verrucariaceae lineages for certain algae remains an open question.
Morphological identification of photobionts within intact lichen thalli is hampered by the fact that characters important for species or genus identification (e.g. cell size and shape, chloroplast and pyrenoid morphology) may be modified in the lichenized state (Tschermak-Woess, Citation1988; Nyati et al., Citation2007; Honegger, Citation2009). Furthermore, freshly collected material is needed for isolating living photobionts, isolation itself is often difficult, and many photobionts grow slowly in culture. It is not surprising, therefore, that the identity of many algal partners is still unknown. The application of molecular techniques has greatly facilitated the study of lichen photobionts in several ways. First, molecular data can provide quick and reliable identification of the algae living within the lichen thallus (Dahlkild et al., Citation2001; Helms et al., Citation2001; Lohtander et al., Citation2003). Second, the application of population genetics approaches to lichen-associated algae and cyanobacteria has allowed a revision of species concepts (Kroken & Taylor, Citation2000; O’Brien et al., 2005; Nelsen & Gargas, Citation2006; Yahr et al., Citation2006; Skaloud & Peksa, Citation2010; Fernández-Mendoza et al., Citation2011; Nelsen et al., Citation2011). Finally, DNA sequence data provide a phylogenetic framework for algal classification (Friedl & Büdel, Citation2008). In the Verrucariaceae, however, very few studies have included molecular data for the photobionts (Friedl & Zeltner, Citation1994; Peters & Moe, Citation2001; Nyati et al., Citation2007; Pérez-Ortega et al., Citation2010).
Most studies using molecular markers for the identification of lichen photobionts amplify algal genes directly from DNA extracts of the complete lichen thallus. These extracts however contain all organisms co-existing with the principal symbionts, such as algae growing on the lichen thallus surface or in cracks or cavities within the lichen (accessory algae), and it may therefore be difficult to distinguish the main symbiotic algal component of a lichen thallus from opportunistic cohabitants (Helms et al., Citation2001; Honegger, Citation2009). These risks can be minimized if symbiotic algae are isolated from the thallus and DNA extracts prepared from unialgal photobiont cultures (Beck & Koop, Citation2001; Beck et al., Citation1998, Citation2002).
Here we explore the unique phylogenetic diversity of photobionts associated with the Verrucariaceae using molecular phylogenetics. In order to maximize our taxon sampling of the photobionts, we combined molecular data from isolated algal strains with a larger number of sequences generated from lichen-thallus DNA from herbarium specimens. These data are complemented with morphological observations from cultured algal isolates that were not sequenced. We analyse the phylogenies of photobionts and mycobionts in order to (1) establish or confirm the identity of the photobionts associated with main clades of the Verrucariaceae and (2) explore the patterns of association between algae and lichen-forming fungi in the context of evolution, thallus complexity and ecology.
Materials and methods
For the fungal partner, sequences were obtained using previously described protocols (Zoller et al., Citation1999; Zhou & Stanosz, Citation2001; Gueidan et al., Citation2007; Savić et al., 2008; Thüs & Nascimbene, Citation2008). Algal sequences produced in this study were obtained using two methods, one culture-independent, the other culture-dependent (see details below). In addition, the correspondence between obtained algal sequences and photobionts was verified by morphological identification of isolated algal strains from a total of 12 lichen species (see ‘fungal associates’ in Supplementary Table 1a (see supplementary material, which is available on the supplementary context tab of the article's online page at http://dx.doi.org/10.1080/09670262.2011.629788)). Specimens for which sequence data was missing were also used to confirm morphological identifications. The presence of cellular contacts between the fungus and the algal cells – either via haustoria or through envelopment of the algal cells by fungal hyphae – was used as the criterion for detecting lichenized algae (Tschermak-Woess, Citation1976). Thallus fragments with these types of contact between the symbionts were selected under the microscope and used to inoculate culture media.
Algal DNA extraction: culture-independent method
Total DNA was extracted from the whole lichen thallus (including both fungal and algal genomic DNA) and algal markers amplified using primers specific for the algae. This method was used for 38 lichen species representing all major clades of the Verrucariaceae (Supplementary Table 1a). Genomic DNA was extracted using a modified protocol from Zolan & Pukkila (Citation1986) as detailed in Gueidan et al. (Citation2007), or following Nelsen et al. (Citation2011). The same DNA extracts were used to obtain sequences for the mycobionts.
Algal DNA-extraction: culture-dependent method
Algal cells were isolated from the fresh lichen thallus and cultured on agar in Petri dishes. This approach was used for 12 lichen species, with a focus on amphibious taxa because for these species the amplification of algal sequences from lichen thallus extracts often produced ambiguous results or failed. Whenever possible, the isolation of photobionts was replicated using several specimens from different localities, so that the 12 species were represented by a total of 21 specimens (Supplementary Table 1a).
The specimens were collected from sites in Europe and North America (Supplementary Table 1a). Specimens from dry environments were kept in air-dried paper packets until inoculation, while those from coastal and freshwater sites were kept at 4°C in moist and opaque polyethylene bags and transferred to the laboratory within a week of collection. To reduce the risk of contamination by epiphytic algae, the upper thallus surface was removed with a sterile razor blade. A drop of sterile water was placed on the decorticated area and the lichen tissues macerated with a sterile lancet. A diluted suspension of algal cells and fungal hyphae was then transferred to different agar-based culture media (1.5% agar). Photobionts from coastal lichens were cultured using an artificial seawater medium modified after Starr & Zeikus (Citation1993: protocol available at http://epsag.netcity.de) or filtered and pasteurized seawater (collected near Portsmouth, UK). Freshwater and terrestrial species were transferred to Bold Basal Medium (Bischoff & Bold, Citation1963), with addition of 2 ml l–1 soil extract (Ettl & Gärtner, Citation1995), and cultured at 16°C under a 12 : 12 hour light : dark regime. The photobiont isolates from Bagliettoa were cultured on Trebouxia medium (Ahmadjian, Citation1993) at 13–15°C in continuous light (Favero-Longo et al., Citation2009). After 1–3 weeks, algal colonies growing out of lichen thallus fragments were separated from the attached mycelia and transferred to fresh media. Cultures are kept at the Natural History Museum London (BM), the Department of Plant Biology of the University of Torino (TO) and EPSAG (SAG). Vouchers of lichen thalli have been deposited in the lichen herbaria at BG, BM, DUKE, F, GZU, LI, MARSSJ, NCU, NY, TO and TSB (Supplementary Table 1a). The algal genera and species investigated were identified morphologically using Ettl & Gärtner (Citation1995). Nomenclature follows AlgaeBase (Guiry & Guiry, Citation2011) and, for the lichenized fungi, Index Fungorum (CABI, CBS & Landcare Research Citation2011) and Gueidan et al. (Citation2009). For light microscopy, we used an Axioskop (Zeiss, Oberkochen, Germany) equipped with differential interference contrast optics.
Amplification and sequencing of algal markers
Genomic DNA was obtained from cultured algal isolates using the DNeasy Plant Mini Kit (Qiagen GmbH, Hilden, Germany). Two gene regions of the algal partner, the small subunit of the nuclear ribosomal RNA gene (nuSSU) and chloroplast-encoded large subunit of ribulose-bisphosphate carboxylase–oxygenase (rbcL), were amplified using published and newly designed primers (). One microlitre of undiluted, 1/10- or 1/100-diluted genomic DNA was added to the following PCR mix: 1× PCR buffer (buffer IV with 1.5 mM MgCl2, Abgene, Rochester, NY), 2.5 µl dNTPs (0.2 mM each), BSA (1 mg ml–1), primers (0.8 µM), 0.75 U Taq polymerase (Denville, South Plainfield, NJ), and water to a total volume of 25 µl. PCR was performed on a PTC-200 Peltier thermal cycler (MJ Research, Waltham, MA). For nuSSU, amplifications were run with an initial cycle of 3 min at 95°C, followed by 35 cycles of the following steps: 45 s at 95°C, 40 s at 52°C and 3 min at 72°C. For rbcL, one initial cycle of 5 min at 95°C preceded 35 cycles of the following steps: 45 s at 95°C, 90 s at 47°C and 2 min at 72°C, or alternatively with an annealing temperature of 50°C and 40 cycles. All amplifications ended with a final cycle at 72°C for 10 min.
Table 1. Amplification and sequencing primers used in this study to obtain rbcL and nuSSU sequences for Verrucariaceae photobionts. The abbreviations ‘amp’ and ‘seq’ indicate primers used for amplification and sequencing, respectively.
After examination with gel electrophoresis or on a spectrophotometer (NanoDrop 2000, Thermo Fisher), PCR products were purified using the Microcon PCR cleaning kit (Millipore, Billerica, MA) or QIAquick Purification Kit (Qiagen, Hilden/Germany). Sequencing was carried out in 10 µl reactions using: 3 µl of purified PCR product, 1 µM of primer, 1 µl of Big Dye (Big Dye Terminator Cycle sequencing kit, ABI PRISM version 3.1; Perkin-Elmer, Applied Biosystems, Foster City, CA), 3 µl of Big Dye buffer, and 2 µl of double-distilled water. Automated reaction clean up and visualization was performed at the Duke IGSP Genome Sequencing & Analysis Core Facility and at the Nano-Bio Centre of the Technical University Kaiserslautern, using ABI 3730xl DNA analysers (Applied Biosystems).
Alignments and phylogenetic analyses
Sequences were assembled and edited using BioEdit (Hall, Citation1999) or Sequencher 4.2.2 (Gene Codes Corporation, Ann Arbor, MI). Four datasets (three algal with concatenated nuSSU and rbcL data and one fungal with concatenated RPB1, nuSSU, nuLSU and mtSSU data) were assembled. The first dataset (the Heterococcus dataset: Supplementary Table 1b) was assembled to investigate the phylogenetic placement of lichenized Heterococcus (Xanthophyceae, Heterokontophyta). It included 13 isolates of Heterococcus (including six newly sequenced photobionts), as well as three other xanthophyte species as outgroups. The second dataset (the Chlorophyta dataset: Supplementary Table 1c) was produced to examine the phylogenetic positions of chlorophyte photobionts, in particular within the two classes Ulvophyceae and Trebouxiophyceae. It included 100 ingroup taxa, including 76 Trebouxiophyceae, 20 Ulvophyceae and four Chlorophyceae. Three species of Prasinophytes were used as outgroups. The third dataset (the Prasiola-group dataset; Supplementary Table 1d) focused on a particular clade of trebouxiophyte algae, the Prasiolales and closely related taxa of the genera Diplosphaera and Stichococcus. It included 81 ingroup taxa and used three species of Chlorellales (Trebouxiophyceae) as outgroups. The Microthamiales might be more closely related to the Prasiola-group but the basal relationships between these groups are not supported. All sources of newly isolated or sequenced algae are summarized in Supplementary Table 1. Finally, the mycobiont dataset (Supplementary Table 1e) comprised 114 taxa, among which were 112 Verrucariaceae representing most of the main genera within this family, while two species of Chaetothyriales were used as an outgroup (TreeBase ID11790). The four different data matrices and trees are accessible on TreeBase (Heterococcus dataset, Treebase ID11791; Chlorophyta dataset, TreeBase ID11792; Prasiola-group dataset, TreeBase ID11793).
Manual alignments were performed using MacClade 4.06 (Maddison & Maddison, Citation2003). Ambiguous regions (sensu Lutzoni et al., Citation2000) and introns were delimited manually and excluded from the alignments. The phylogenetic signals from different amplified regions (rbcL and nuSSU for the algal datasets and nuLSU, nuSSU, mtSSU and RPB1 for fungal dataset) were tested for congruence using a 70% reciprocal bootstrap criterion (Mason-Gamer & Kellogg, Citation1996). The topology comparison was undertaken manually based on trees obtained with 500 bootstrap pseudoreplicates using RAxML VI-HPC (Stamatakis et al., Citation2005, Citation2008) on the Cipres Web Portal (http://www.phylo.org/sub_sections/portal/). Taxa or sequences responsible for incongruence were removed from the dataset (Placidiopsis cinerascens CG585b and Verrucaria viridula CG587 were removed from the algal datasets and also the four algal nuSSU sequences obtained from Agonimia opuntiella L623, A. tristicula L469, Normandina acroglypta L618 and N. pulchella L616, and one rbcL sequence obtained from Stictis uceolatum AAsn), and the two markers were combined. Phylogenetic relationships and confidence were inferred using RAxML on the Cipres Web Portal. The ML search followed a GTRMIX model of molecular evolution applied to the four partitions for the algal datasets (rbcL first, second and third codon positions, and nuSSU) and six partitions for the fungal dataset (RPB1 first, second and third codon positions, and nuLSU, nuSSU and mtSSU). Support values were obtained in RAxML with bootstrap analyses of 1000 pseudoreplicates.
Results
The photobionts of the Verrucariaceae we investigated belonged to the Xanthophyceae (Heterokontophyta) and the two green algal classes Trebouxiophyceae and Ulvophyceae (Chlorophyta). Within the Xanthophyceae (Heterokontophyta), all of the lichen-associated algal isolates belonged to the same genus, Heterococcus (). In the Ulvophyceae, all the photobiont isolates belonged to the genus Dilabifilum. In the Trebouxiophyceae (Figs 2 and 3), the photobiont isolates belonged to several genera, which were delimited here according to Ettl & Gärtner (Citation1995), Skaloud & Peksa (Citation2010) and Guiry & Guiry (Citation2011).
Fig. 1. Phylogenetic placement of the xanthophycean photobionts of Verrucariaceae. The tree was obtained using a maximum-likelihood analysis of a two-gene dataset (rbcL–nuSSU). Lichenized algal strains are indicated in parentheses with the name of the corresponding lichen-forming fungus and its collection number. Bootstrap values >70% are indicated above or below the branches.
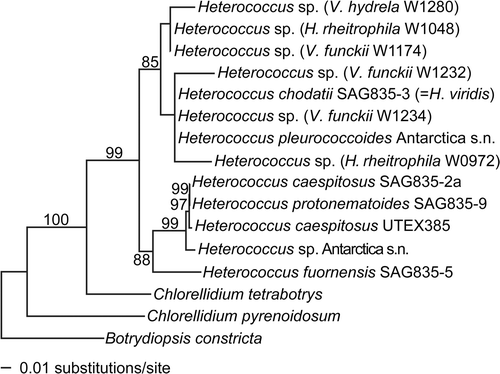
Phylogeny of the xanthophycean photobionts (Heterococcus spp., )
Sequences of all six photobiont isolates of Heterococcus clustered together with sequences of the authentic strain of H. chodatii Vischer (a synonym of H. viridis Chodat) and an isolate of H. pleurococcoides Pitschmann (85% bootstrap). Heterococcus caespitosus, the species thought to be the photobiont of the sampled species of Verrucariaceae belonged to a different well-supported clade, together with H. protonematoides Vischer and an unidentified specimen of Heterococcus (99% bootstrap). All our Heterococcus isolates were associated with freshwater Verrucariaceae from periodically or permanently submerged habitats. The mycobionts of these lichens [Hydropunctaria rheitrophila (Zschacke) C. Keller, Verrucaria funckii (Sprengel) Zahlbruckner and V. hydrela Acharius] do not belong to a unique lineage, but are members of three distantly related clades ().
The initial morphological characteristics in culture of these lichen-associated Heterococcus resembled H. caespitosus, with elongated and frequently branched filaments. However, after a few months our isolates underwent changes in cell morphology and thallus appearance: the filaments disintegrated and our isolates grew instead in short and weakly branched chains with rounded to ovoid cells. When transferred to fresh agar media, filaments with slender cells temporarily re-appeared for a few weeks (), but they never reached the lengths achieved by filaments of the young thalli when first isolated from wild-collected lichens. In liquid culture, cells always remained oval to spherical and grew in small aggregates or chains (). The morphology of the photobiont Heterococcus was therefore intermediate between H. pleurococcoides, which tends to have shorter chains or cell clusters, and H. viridis (syn. H. chodatii), which first develops branched thalli but soon separates into individual cells.
Phylogeny of ulvophycean photobionts (Dilabifilum spp., )
All our isolates of Dilabifilum from the Verrucariaceae belonged to a single well-supported clade within the Ulvophyceae (94% bootstrap; ). This clade was sister to a lineage including the genera Kornmannia and Blidingia (94% bootstrap). It contained the type strain of Dilabifilum arthopyreniae (Vischer & Klement) Tschermak-Woess, an accessory alga isolated from the shell-colonizing lichen Collemopsidium halodytes (Nylander) Grube & B.D. Ryan (syn. Arthopyrenia kelpii Körber). The clade also contained algal strains isolated from periodically or permanently submerged Verrucariaceae from freshwater and coastal intertidal habitats. Species from at least three different clades of amphibious Verrucariaceae (Hydropunctaria, Wahlenbergiella and Verrucaria aquatilis Mudd) were associated with these species of Dilabifilum (). In culture, none of our algal strains produced zoospores, which prevented further identification. Other relevant diagnostic characters, such as the shape of the terminal cells and the dimensions of cells in the filaments, were variable even within single thalli of our isolates ().
Fig. 2. Phylogenetic placement of chlorophyte photobionts of Verrucariaceae. The tree was obtained using a maximum-likelihood analysis of a two-gene dataset (rbcL–nuSSU). Lichenized algal strains are indicated by the addition, in parentheses, of the name of the corresponding lichen-forming fungus. They all belong to the family Verrucariaceae, except for Cladonia grayi, Icmadophila ericetorum, Lobaria linita, Racodium rupestre, Stictis urceolatum and Xanthoria parietina. For the Verrucariaceae, the collection numbers follow the fungal names. Bootstrap values >70% are indicated above or below the branches. Photobionts with identical sequences are represented by a single taxon in the phylogeny with the names and collection numbers of each lichen indicated.
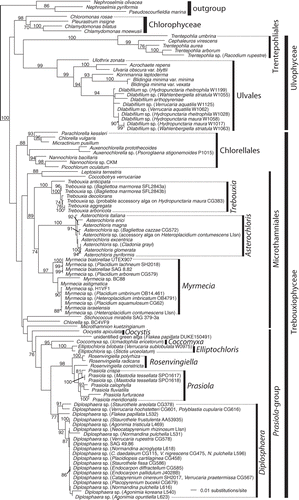
Fig. 3. Phylogenetic placement of the photobionts of Verrucariaceae related to the Prasiola-group. The tree was obtained using a maximum-likelihood analysis of a two-gene dataset (rbcL–nuSSU). Lichenized algal strains are indicated by the addition, in parentheses, of the name of the corresponding lichen-forming fungus. They all belong to the family Verrucariaceae, except for Icmadophila ericetorum, Lobaria pulmonaria, Phlyctis argena and Stictis urceolatum. For the Verrucariaceae, the collection numbers follow the fungal names. Bootstrap values >70% are indicated above or below the branches, except for values of 100%, which are indicated by a star. Photobionts with identical sequences are represented by a single taxon in the phylogeny with the names and collection numbers of each lichen indicated.
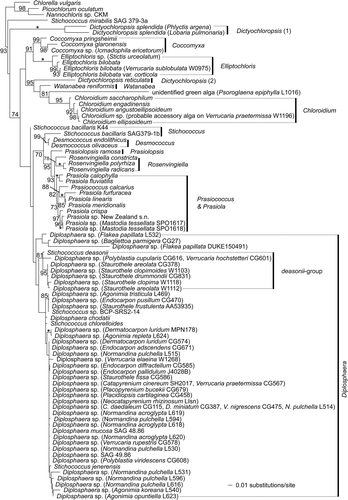
Fig. 4. Distribution of photobionts across a phylogeny of the Verrucariaceae obtained using a maximum-likelihood analysis of a four-gene dataset (RPB1–nuLSU–nuSSU–mtSSU). Lichen specimens for which molecular data are available for the photobionts are indicated in bold. For these specimens, the identity of the photobiont is shown after the taxon label using the following abbreviations: Ast = Asterochloris, Dil = Dilabifilum, Dip = Diplosphaera, Dip/dea = Diplosphaera deasonii-group (Stichococcus aff. mirabilis), Ell = Elliptochloris, Het = Heterococcus, Myr = Myrmecia, Pet = Petroderma maculiforme, Pra = Prasiola, Tre = Trebouxia. *For Hydropunctaria rheitrophila, two other specimens not included in this tree (W1048 and W0972) were associated with Heterococcus sp. (see ).
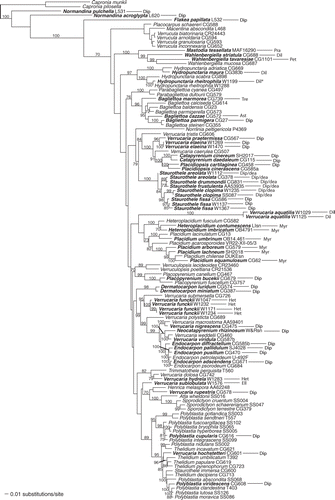
Figs 5–11. Light photomicrographs of algae isolated from crustose lichens of the family Verrucariaceae. 5. Filamentous growth in young culture of Heterococcus sp. isolated from Verrucaria funckii and cultured on 1.5% BBM-agar (BM H. Thüs W1174). 6. Cell aggregates of Heterococcus sp. isolated from V. funckii and cultured in liquid BBM medium (BM H. Thüs W1174). 7. Dilabifilum sp. isolated from V. aquatilis (BM H. Thüs W1125). 8. Chloroidium sp. isolated from V. praetermissa (BM H. Thüs W1198). 9. Elliptochloris bilobata isolated from V. sublobulata (BM H. Thüs W0975). 10. Diplosphaera sp. isolated from V. elaeina (BM H. Thüs W1269). 11. Stichococcus cf. mirabilis isolated from Staurothele clopima (BM H. Thüs W1118). Scale bars: 10 µm.
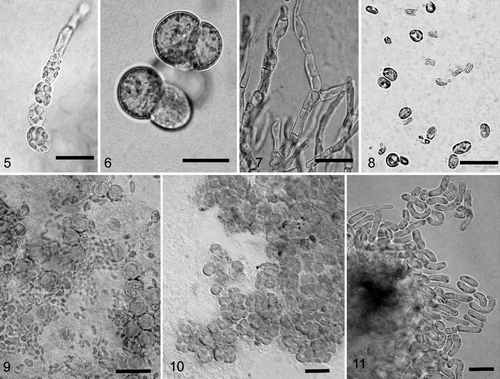
Phylogeny of trebouxiophycean photobionts ( and )
In our study, we found sequences of Trebouxia sensu stricto in only two species, Bagliettoa marmorea (Scopoli) Gueidan & Cl. Roux and Hydropunctaria maura (Wahlenberg) C. Keller, Gueidan & Thüs. It is very likely that the sequence of Trebouxia from H. maura represents an epiphyte instead of the actual photobiont, because this lichen species has repeatedly been found associated with a filamentous alga using morphological methods (including in this present study), and not with coccal Trebouxia-like algae. The coccal Trebouxia sensu stricto from B. marmorea has been found in two specimens (Favero-Longo et al., Citation2009; ). Other sampled species of Bagliettoa were associated with different photobionts: B. cazzae (Zahlbruckner) Vězda & Poelt was found with Asterochloris sp. and B. parmigera (J. Steiner) Vězda & Poelt with Diplosphaera sp. ( and ).
Sister to Trebouxia were the genera Myrmecia and Asterochloris (), which include both lichenized and free-living species. According to our taxon sampling, two species of Myrmecia, M. biatorellae J.B. Petersen and M. israelensis (S. Chantanachat & H. Bold) T. Friedl, can be found as photobionts of the Verrucariaceae. They are exclusively associated with the two closely related lichen genera Placidium and Heteroplacidium (), and are found in terricolous, saxicolous and corticolous species. In Asterochloris, our culture-independent method yielded one algal genotype found as an accessory alga on H. contumescens (Nylander) Breuss and involved in symbiosis with the fungal species Bagliettoa cazzae (). However, the identity of the photobiont of B. cazzae needs confirmation as our results are based on the observation of a single specimen.
The majority of trebouxiophycean photobionts were within the Prasiola-group ( and ). The sequence of one of our photobiont isolates, which came from the amphibious lichen Verrucaria praetermissa (Trevisan) Anzi, was nested within the genus Chloroidium Nadson (). The minute ellipsoidal cells with a single parietal chloroplast observed in our isolates of Chloroidium fit well the description of C. ellipsoideum (Gerneck) Darienko, Gustavs, Mudimu, C.R. Menendez, R. Schumann, U. Karsten, Friedl & Pröschold. However, our morphological observations suggested that Chloroidium in this lichen species may represent an accessory alga, and not the principal photobiont. Although we have isolated Chloroidium repeatedly from thalli of V. praetermissa, only in one case were the algal cells directly connected to fungal hyphal walls from the lichen thallus (). However, such cellular contacts were often observed with Diplosphaera cells in the same thalli.
In contrast, the molecular identification of the photobiont of Verrucaria sublobulata Servít as Elliptochloris bilobata Tschermak-Woess was reliable, as microscopical observations confirmed the presence of cellular contacts between the symbionts. Our isolates showed the characteristic morphology of E. bilobata, with a deeply divided parietal chloroplast, the formation of rod-like autospores, and numerous transparent droplets in the centre of the cell (). In our phylogeny, this photobiont was part of a well-supported clade together with lichenized and non-lichenized strains of Elliptochloris (100% bootstrap; ) and its sequence was almost identical to the epiphytic E. bilobata var. corticola Eliáš, Neustupa & Škaloud (1 bp difference in the nuSSU sequence; ).
A large number of Verrucariaceae species were associated with coccoid algae with a parietal unlobed chloroplast, which only covered up to two-thirds of the cell wall. These algal cells often formed characteristic three- to four-celled clusters in early growth stages after their isolation from the lichen thallus. A representative strain of this photobiont was our isolate W1269 from Verrucaria elaeina Borrer (). The sequence generated from this photobiont strain fell within a large unsupported group of taxa within the Prasiola group (), which can be assigned to the genus Diplosphaera sensu lato (including some species of Stichococcus). This group also included a large number of sequences from lichen thallus DNA-extracts of various Verrucariaceae. A lineage formed by the genera Desmococcus, Rosenvingiella and Prasiola was sister to the Diplosphaera clade, although this relationship was without statistical support. The type species of Stichococcus, S. bacillaris Nägeli, represented here by two published strains SAG 379-1b and K44, was not nested within Diplosphaera: one strain (SAG 379-1b) was sister to Desmococcus and the other (K44) lay at the base of a lineage including Desmococcus, Diplosphaera, Prasiococcus, Prasiola, Prasiolopsis, Rosenvingiella and S. bacillaris SAG 379-1b. The phylogenetic position of S. bacillaris is still problematic, because neither of the placements of these two strains was significantly supported in our phylogeny. The authentic strains of S. deasonii Neustupa, Eliáš & Šejnohová, S. jenerensis Neustupa, Eliáš & Šejnohová and Diplosphaera mucosa Broady were nested within Diplosphaera, as well as a strain of S. chlorelloides Grintzesco & Péterfi.
A high bootstrap support (95% bootstrap; ) was obtained in our phylogeny for a group of photobionts morphologically similar to Stichococcus mirabilis Lagerheim. This group was nested among lichenized strains of Diplosphaera and sister to S. deasonii and a genotype of Diplosphaera obtained from the lichen thalli of Polyblastia cupularis A. Massalongo and Verrucaria hochstetteri Fries. Photobiont cells isolated from Staurothele had rod-like cells at first, becoming more or less curved in culture, and one or more deeply lobed chloroplasts (). Based on their lichen associates and their morphology, this lineage most likely corresponds to S. mirabilis sensu Ahmadjian & Heikkila (Citation1970) although it is distinct from the nuSSU-sequence of the non-lichenized strain of S. mirabilis kept as SAG 379-3a, which forms here the earliest branching in the Prasiola-group ( and ).
Sequences of algae from lichen DNA-extracts of the species Normandina pulchella (Borrer) Nylander and N. acroglypta (Norman) Aptroot here represented by multiple specimens, nested within the Diplosphaera clade. Algal sequences obtained from the thallus of Flakea papillata O.E. Eriksson, a membranous-foliose lichen recently shown to belong to Verrucariaceae (Muggia et al., Citation2009), were also nested within this clade (). Because of an unusually thin sterile thallus composed of only one cell layer and the presence of rhizoids, this lichen has been attributed in the past to algal colonies, bryophytes or prothalli of ferns (Eriksson, Citation1992). Algal sequences from our lichen extracts of Flakea papillata are related to those of the Diplosphaera-group in our analysis (). The photobionts of both specimens of Flakea papillata (DUKE150491 and L532) belong to a clade also including the photobiont of Bagliettoa parmigera, although support is lacking for this relationship (). Further work will be necessary to confirm whether any of the two sequences obtained in our study truly belong to the photobiont of Flakea papillata.
Phylogeny of the lichen family Verrucariaceae ()
The multigene phylogeny (RPB1–nuLSU–nuSSU–mtSSU) was similar to previous studies (Gueidan et al., Citation2007, Citation2009; Savić et al., 2008; Muggia et al., Citation2009, Citation2010; Pérez-Ortega et al., Citation2010). Additionally, the phylogenetic position of several freshwater species was demonstrated here: Verrucaria elaeina was sister to V. praetermissa, and V. funckii to V. submersella Servít (); V. hydrela appeared as sister to V. dolosa Hepp. Verrucaria sublobulata is sister to a clade including Atla, Henrica, Sporodictyon and V. rupestris Schrader, although without support. The species V. aquatilis, represented here by two specimens, was on a long branch and its relationship to other Verrucariaceae was not supported.
Discussion
The family Verrucariaceae has long been of great interest to lichenologists and phycologists in determining the breadth of lichen photobiont diversity. Microscopical observations have produced a particularly long list of compatible photobionts for this lichen family (Tschermak-Woess, Citation1988). More recently, some studies have used molecular data to confirm the identity of some of these photobionts (Friedl & Zeltner, Citation1994; Peters & Moe, Citation2001; Nyati et al., Citation2007; Pérez-Ortega et al., Citation2010; Gueidan et al., 2011), but our study is the first to sample a large number of species across this family. The new data have allowed us to check the identity and phylogenetic position of photobionts from the Verrucariaceae and investigate the patterns of associations between the symbionts.
Patterns of photobiont associations in Verrucariaceae
The most common photobionts in the Verrucariaceae are from the genus Diplosphaera. They are associated with many lichen-forming fungal species, irrespective of their phylogenetic placement in the Verrucariaceae tree (). Both early diverging Verrucariaceae (e.g. Normandina) and more recently diverging ones (e.g. Endocarpon, Staurothele) have strains of Diplosphaera as photobionts. The algal genus Myrmecia, on the other hand, is restricted to a single lineage within the Verrucariaceae, which includes the two squamulose genera Heteroplacidium and Placidium ( and ). Although this lineage includes species from different substrata (bark, rock and soil), all sampled species have Myrmecia as a photobiont. It is therefore likely that photobiont associations in this group of lichens with complex thalli result mostly from common ancestry. Similarly, in the foliose genus Dermatocarpon, an amphibious species (D. luridum) and a species from exposed rock surfaces (D. miniatum) both have Diplosphaera as a photobiont ().
In contrast to lichens with complex thalli, species with simple crustose thalli (e.g. Wahlenbergiella, Hydropunctaria, Bagliettoa) and often lacking an upper cortex, are characterized by a high diversity of photobionts. In particular, the amphibious lineage that includes Wahlenbergiella and Mastodia is characterized by unusual photobionts: Mastodia tessellata is associated with Prasiola sp., W. tavaresiae with Petroderma maculiforme and W. striatula (Wahlenberg) Gueidan & Thüs with Dilabifilum sp. (). Other amphibious species, such as H. rheitrophila, are known to associate with different photobionts depending on the ecological conditions (Thüs & Schultz, Citation2008). Moreover, endolithic species of Bagliettoa are among the few Verrucariaceae associated with Trebouxia. For these amphibious and endolithic lineages, switches in photobionts are probably common and photobiont associations most likely result from ecological conditions.
In Verrucariaceae, genera with complex thalli seem to have a higher specificity for their photobionts than taxa with crustose thalli, which is consistent with observations in other groups of lichens (Helms, Citation2003; Blaha et al., Citation2006; Honegger, Citation2009). A complex thallus with a highly structured and developed cortex may prevent lichen-forming fungi from interacting with and adopting other photobionts from their environment, whereas a simple thallus lacking a cortex may perhaps allow greater freedom to develop alternative associations. Except for a few lineages of squamulose species most Verrucariaceae have simple crustose thalli and many lack a cortex. A simple morphology and a diverse ecology might therefore explain the high photobiont diversity found in this lichen family.
Revision of the identity of photobionts associated with Verrucariaceae
Heterococcus
In the past, H. caespitosus was the only species of this xanthophyte genus reported as a photobiont of Verrucariaceae, being described from Verrucaria elaeomelaena (A. Massalongo) Arnold, V. funckii (Tschermak, Citation1941b ; Zeitler, Citation1954; Tschermak-Woess, Citation1988) and V. cf. praetermissa (Zeitler, Citation1954, under the older synonym V. laevata auct.). It was also described from Hydropunctaria maura (Parra & Redon, Citation1977), but this record is most probably based on specimens representing an undescribed lichen species and not H. maura, because the reported ascospore size falls outside the usual range for this species. Our results demonstrate that the identity of Heterococcus species associated with amphibious Verrucariaceae has to be revised. None of our studied specimens of H. maura and V. praetermissa had Heterococcus as a photobiont. More importantly, none of the Heterococcus isolates sequenced here form a monophyletic clade with Heterococcus caespitosus, but they are more closely related to two other species, H. pleurococcoides and H. chodatii.
The confusion between H. caespitosus and the actual Heterococcus photobionts may have arisen because of the morphological changes that occur with time when the photobionts are grown in culture. The longer cells and branched filaments present in the initial isolation phase led Thüs & Schultz (Citation2008) to identify H. caespitosus as a photobiont of Verrucariaceae, but we show here that the same strains do not in fact belong to this algal species. The challenges caused by changes in cell morphology in Heterococcus have been noted previously (e.g. Darling et al., Citation1987; Lokhorst, Citation1992; Ettl & Gärtner, Citation1995). The genetic distances between our isolates and either H. pleurococcoides or H. chodatii are small and further studies with several more rapidly evolving markers (e.g. ITS rDNA) are required in order to find out if this well-supported clade consists of more than one species or represents a single but morphologically variable taxon. Given the problematic morphological circumscription of species in the genus Heterococcus, the earlier record of H. caespitosus from Verrucaria elaeomelaena sensu stricto (Tschermak, Citation1941b ) will require confirmation by molecular data.
Dilabifilum
In a previous study, Friedl & Büdel (Citation2008) showed that Dilabifilum arthopyreniae belonged to the Ulvales (Ulvophyceae). Our results confirm this phylogenetic placement with eight additional strains of Dilabifilum. There is considerable genetic diversity within the Dilabifilum clade but neither the habitat (freshwater vs. saline environments) nor the association with specific lichen taxa corresponds to the observed phylogenetic structure. The morphology of the isolated strains changed considerably over time. Characters affected by these changes, such as the width: length ratio of the cells and the form of terminal cells of the filaments, are regarded as diagnostic at the species level (Johnson & John, Citation1990). A revision of the genus Dilabifilum, including a molecular study of authentic strains of D. incrustans (Vischer) Tschermak-Woess, D. printzii (Vischer) Tschermak-Woess and D. prostratum Broady & Ingerfeld, is needed before a name can be attributed to our isolates.
Auxenochlorella
The photobiont of the granulose Psoroglaena stigonemoides (Orange) Henssen has previously been shown to belong to the genus Auxenochlorella (Nyati et al., Citation2007). This is the only representative of the Chlorellales associated with any of the Verrucariaceae.
Asterochloris and Trebouxia
Algae from the genera Asterochloris and Trebouxia are among the most common symbionts in most lichenized fungi but are rare in the Verrucariaceae. In addition to the Myrmecia rbcL sequence, a sequence of Asterochloris was obtained for Heteroplacidium contumescens with the culture-independent method. Because all other members of the Heteroplacidium–Placidium lineage associate with Myrmecia, this record most likely corresponds to an accessory alga. Our results suggest that species of Bagliettoa associate with three different photobiont genera, Asterochloris, Trebouxia and Diplosphaera ( and ). Further studies are needed in order to confirm these records and whether there is low photobiont specificity at the generic level for the endolithic genus Bagliettoa.
Myrmecia
Myrmecia biatorellae has previously been isolated from various squamulose Verrucariaceae and the amphibious crust Verrucaria submersella (Zeitler, Citation1954). Our data confirm M. biatorellae as a photobiont of squamulose Verrucariaceae (Heteroplacidium and Placidium) and, for the first time, M. israelensis has been identified as another photobiont from this lichen family.
Elliptochloris
Elliptochloris had been reported as an occasional photobiont in the Verrucariaceae (Thüs & Schultz, Citation2008). Although most of the amphibious species of Staurothele cited in earlier reports of Elliptochloris have been included, we failed to find it in any of these lichens. It is therefore likely that E. bilobata is an occasional epi- or endophyte but not the principal photobiont of Staurothele. However, in our study E. bilobata was found in our study associated with Verrucaria sublobulata and this record suggests that Elliptochloris may have a limited inundation tolerance when occurring in lichens; all other reports of E. bilobata from Verrucariaceae refer to collections from rather shaded and humid or temporarily inundated habitats (Tschermak-Woess, Citation1985; Trembley et al., Citation2002; Thüs & Schultz, Citation2008).
Prasiola sp. and Petroderma maculiforme
Although our taxon sampling covered several species of the genus Wahlenbergiella, the very closely related genus Mastodia remains the only lichen with Prasiola as a photobiont. In M. tessellata, the fungus lives within the thallus of the alga. Wahlenbergiella, however, is often associated with various strains of Dilabifilum (Ulvales), which occur inside a typical crustose lichen thallus. Despite the fact that W. striatula often grows side by side with Mastodia tessellata in the Southern Hemisphere, and may sometimes even become overgrown by free-living Prasiola, its photobiont is always Dilabifilum. Verrucaria tavaresiae R.L. Moe, a species associated with the brown alga Petroderma maculiforme, was recently shown to belong to the genus Wahlenbergiella (Gueidan et al., 2011). In this association, the algal filaments are also embedded within a thallus formed by the fungus (Sanders et al., Citation2004, 2005).
Stichococcus deasonii group
With the exception of Staurothele fissa (Taylor) Zwackh and S. frustulenta Vainio, all other sampled species of Staurothele sensu stricto (species with an epilithic thallus, Gueidan et al., Citation2009) share a genetically and morphologically similar photobiont ( and ). Like Endocarpon the genus Staurothele is characterized by the presence of algal cells within the fruiting bodies. These algal cells are small (<7 µm) and either cube- or rod-like, whereas algal cells from the thallus are larger (usually >10 µm) and spherical. Ahmadjian & Heikkila (1977) showed using culture experiments that the algae in the fruiting bodies of Staurothele clopima (Wahlenberg) Th. Fries are the same as the larger rounded cells from the thallus and they identified them as Stichococcus mirabilis. This alga differs from the closely related S. deasonii in the absence of a pyrenoid, the longer and often slightly curved cells, a lobed chloroplast, and the absence of filaments when cultured on solid media. Our isolates, which include collections from Europe and North America, fit this description, although some cells are strongly curved. However, our isolates do not cluster with a North American strain of S. mirabilis (SAG 379-3a) for which a sequence is available in GenBank, so further work will be necessary to confirm the identity of the elongated photobionts of Staurothele sensu stricto.
Diplosphaera
Our molecular and morphological observations indicate that earlier reports of photobionts from the poorly defined genera Diplosphaera, Protococcus and Stichococcus all seem to correspond in fact to members of the genus Diplosphaera. Historically, and particularly in the context of lichen symbiosis, the genus Protococcus has been used to accommodate species lacking clear diagnostic characters (Stahl, Citation1874; Winter, Citation1875; Zeitler, Citation1954). Moreover, the genera Stichococcus and Diplosphaera have always been difficult to differentiate by morphological characters. Diplosphaera differs from Stichococcus by the formation of two-celled clusters (Ettl & Gärtner, Citation1995). Both species and genus delimitations in this group of algae are poorly known.
Řeháková (1968) regarded S. chlorococcoides and most of the strains reported as Protococcus spp. by earlier authors as synonyms of Diplosphaera chodatii, attributing to this species a wide concept. Her illustrations make it clear that she included strains producing large amounts of mucilage, which would usually be referred to as Diplosphaera mucosa. Based on her own culture experiments, Řeháková (1968) reported Diplosphaera chodatii as the photobiont of Dermatocarpon arnoldianum auct., D. luridum (Dillenius ex Withering) J.R. Laundon, D. miniatum (Linnaeus) W. Mann and D. rivulorum (Arnold) Dalla Torre & Sarnthein. She added Polyblastia nigella Krempelhuber, Staurothele caesia (Arnold) Arnold, S. clopima, S. clopimoides Baglietto & Carestia) J. Steiner, S. fissa, S. frustulenta, S. rufa (A. Massalongo) Zschacke, Verrucaria acrotelloides A. Massalongo, V. dufourii De Candolle, V. myriocarpa Lönnroth and V. tristis (A. Massalongo) Krempelhuber to her list of compatible mycobionts, based on illustrations from Winter (Citation1875), Stahl (Citation1874) and Zeitler (Citation1954). These observations agree with our results, as our sequences of Diplosphaera were found associated with the same groups of mycobionts as those cited by Řeháková (1968). As in other related algal genera (e.g. Rindi et al., Citation2007), the genetic variation among the strains of the Diplosphaera for the nuSSU and rbcL gene regions is low, but the morphological and ecological variations are important. Faster evolving markers are required in order to study species delimitation in this morphologically diverse group of lichen photobionts and free-living algae.
Nannochloris normandinae
Tschermak-Woess (Citation1988) described the isolated photobiont of Normandina pulchella as Nannochloris normandinae Tschermak-Woess, which differs from species of Stichococcus and Diplosphaera in the presence of a pyrenoid. In our study, none of the photobionts isolated from the ten specimens of Normandina pulchella and N. acroglypta belonged to Nannochloris. Instead, they were Diplosphaera species. Moreover, our phylogeny shows that Stichococcus jenerensis, another species with a well-developed pyrenoid, is part of the large Diplosphaera clade. The authentic strain of Nannochloris normandinae could not be located, so new culture experiments will be required in order to verify if N. normandinae belongs in fact to Diplosphaera or if species of Normandina have different photobionts than previously reported.
Culture-dependent versus culture-independent methods
According to our results, amphibious lichens seem to be particularly liable to produce erroneous records of photobionts when using PCR methods directly on environmental samples of thalli. All our attempts to amplify rbcL or nuSSU of species of Dilabifilum and Heterococcus from lichen thallus extracts (using the culture-independent method) have consistently failed, even with specific primers. With general primers, these markers were more easily amplified for other algae occurring as epiphytes or as photobionts of neighbouring lichens. This amplification bias in the culture-independent method can lead to confusing results. For example, in coastal intertidal environments, Dilabifilum-associated lichens often grow side by side with Caloplaca species, and are sometimes overgrown by them, such as C. microthallina Weddell on Hydropunctaria maura. Using the culture-independent method, we have recovered a sequence of Trebouxia from H. maura, whereas the culture-dependent method confirms that H. maura is associated with Dilabifilum. Similarly, a previous record of Trebouxia from Verrucaria praetermissa (Thüs, Citation2002) is refuted by our new data.
Fast-growing algae are occasionally found on the surface and in the cracks of the lichen thallus. These accessory algae can also contaminate photobiont cultures obtained from thallus fragments and can easily be mistaken for the photobiont unless the fragments are checked for the connection between algal cells and fungal hyphae. Accessory algae have been found in lichen thalli of various families of lichen-forming fungi (Beck et al., Citation1998, Citation2002; Helms et al., Citation2001; Thüs, Citation2002; Lakatos et al., Citation2004; Honegger, Citation2009) and their involvement in the lichen symbiosis merits further studies.
Conclusion
Our study provides the first molecular confirmation of the identity of photobionts from a large number of species within the Verrucariaceae and helps to identify areas of conflict between morphology- and molecular-based identifications. It also documents the value of complementing the fast and easy culture-independent method with the more time consuming culture-dependent method. Moreover, the use of cultures is essential for differentiating photobionts from accessory algae. Our results show that the link between ecology and photobiont patterns is strong for crustose Verrucariaceae, in particular in amphibious habitats where the diversity of associated algae is the highest. For Verrucariaceae with more complex thalli, the diversity of associated algae is lower and is best explained by common ancestry. Ecological preferences and phylogenetic relatedness are therefore both involved in shaping the patterns of photobiont associations in the lichen family Verrucariaceae.
tejp_a_629788_sup_22661181.xlsx
Download MS Excel (51.7 KB)Acknowledgements
We thank Anna Guttova, Christine Keller, Helmut Mayrhofer and Akira Peters for logistic support for the collection of some material used in this study. We are very grateful to Burkhard Büdel for helpful discussions and Louise Lewis for providing sequence alignments. We would also like to acknowledge Fabio Rindi and an anonymous reviewer for their useful comments on the manuscript. This work was partly financed by a grant from the German Science Foundation to H.T. (DFG TH840-2), by a SYNTHESYS grant to C.G., by an NSF DDIG grant (DEB-0508567) to F.L. and C.G, and by NSF AToL grants (AFTOL, DEB-0229725 and DEB-0732984) to F.L.
References
- Ahmadjian , V . 1993 . The Lichen Symbiosis, , 2nd , New York : John Wiley .
- Ahmadjian , V and Heikkila , H . 1970 . The culture and synthesis of Endocarpon pusillum and Staurothele clopima . Lichenologist , 4 : 259 – 267 .
- Beck , A and Koop , H-U . 2001 . Analysis of the photobiont population in lichens using a single-cell manipulator . Symbiosis , 31 : 57 – 67 .
- Beck , A , Friedl , T and Rambold , G . 1998 . Selectivity of photobiont choice in a defined lichen community: inferences from cultural and molecular studies . New Phytol. , 139 : 709 – 720 .
- Beck , A , Kasalicky , T and Rambold , G . 2002 . Myco-photobiontal selection in a Mediterranean cryptogam community with Fulgensia fulgida . New Phytol. , 153 : 317 – 326 .
- Bischoff , HW and Bold , HC . 1963 . Phycological studies IV. Some soil algae from Enchanted Rock and related algal species . Univ. Texas Publ. , 6318 : 1 – 95 .
- Blaha , J , Baloch , E and Grube , M . 2006 . High photobiont diversity associated with the euryoecious lichen-forming ascomycete Lecanora rupicola (Lecanoraceae, Ascomycota) . Biol. J. Linn. Soc. , 99 : 283 – 293 .
- CABI, CBS & LANDCARE RESEARCH (2011). Index Fungorum. http//www.indexfungorum.org, searched on 27/04/2011
- Dahlkild , Å. , Källersjö , M , Lohtander , K and Tehler , A . 2001 . Photobiont diversity in Physciaceae (Lecanorales) . Bryologist , 104 : 527 – 536 .
- Darling , RB , Friedmann , EI and Broady , PA . 1987 . Heterococcus endolithicus sp. nov. (Xanthophyceae) and other terrestrial Heterococcus species from Antarctica: morphological changes during life history and response to temperature . J. Phycol. , 23 : 598 – 607 .
- Eriksson , OE . 1992 . Psoroglaena cubensis and Flakea papillata gen. et sp. nov., two corticolous lichens with a pantropical distribution . Systema Ascomycetum , 11 : 11 – 27 .
- Ettl , H and Gärtner , G . 1995 . Syllabus der Boden-, Luft- und Flechtenalgen , Stuttgart : Gustav Fischer .
- Favero-Longo , SE , Borghi , A , Tretiach , M and Piervittori , R . 2009 . In vitro receptivity of carbonate rocks to endolithic lichen-forming aposymbionts . Mycol. Res. , 113 : 1216 – 1227 .
- Fernández-Mendoza , F , Domaschke , S , García , MA , Jordan , P , Martín , MP and Printzen , C . 2011 . Population structure of mycobionts and photobionts of the widespread lichen Cetraria aculeata . Mol. Ecol. , 20 : 1208 – 1232 .
- Friedl , T and Büdel , B . 2008 . “ Photobionts ” . In Lichen Biology, , 2nd , Edited by: Nash , T. H. III . 7 – 26 . Cambridge : Cambridge University Press .
- Friedl , T and Zeltner , C . 1994 . Assessing the relationships of some coccoid green lichen algae and the Microthamniales (Chlorophyta) with 18S ribosomal RNA gene sequence comparisons . J. Phycol. , 30 : 500 – 506 .
- Gueidan , C , Roux , C and Lutzoni , F . 2007 . Using a multigene analysis to assess generic delineation and character evolution in the Verrucariaceae (Eurotiomycetes, Ascomycota) . Mycol. Res. , 111 : 1147 – 1170 .
- Gueidan , C , Savić , S , Thüs , H , Roux , C , Keller , C , Tibell , L , Prieto , M , Heiðmarsson , S , Breuss , O , Orange , A , Fröberg , L , Amtoft Wynns , A , Navarro-Rosinés , P , Krzewicka , B , Pykälä , J , Grube , M and Lutzoni , F . 2009 . Generic classification of the Verrucariaceae (Ascomycota) based on molecular and morphological evidence: recent progress and remaining challenges . Taxon , 58 : 184 – 208 .
- GUEIDAN, C., THÜS, H. & PÉREZ-ORTEGA, S. (2011). Phylogenetic position of the brown algae-associated lichenized fungus Verrucaria tavaresiae (Verrucariaceae). Bryologist, DOI: 10.1639/0007-2745-114.3.xxx
- GUIRY, M.D. &; GUIRY, G.M. (2011). AlgaeBase. World-wide electronic publication, National University of Ireland, Galway. http://www.algaebase.org; searched on 27 April 2011
- Hall , TA . 1999 . BioEdit: a user-friendly biological sequence alignment editor and analysis program for Windows 95/98/NT . Nucl. Acid Symp. Ser. , 41 : 95 – 98 .
- Hamby , RK , Sim , LE and Zimmer , EA . 1988 . Direct RNA sequencing: optimisation of extraction and sequencing techniques for work with higher plants . Plant. Mol. Biol. Rep. , 6 : 179 – 197 .
- HELMS, G. (2003). Taxonomy and symbiosis in associations of Physciaceae and Trebouxia. Dissertation zur Erlangung des Doktortitels, Mathematisch-naturwissenschaftliche Fakultäten, Georg-August-Universität Göttingen
- Helms , G , Friedl , T , Rambold , G and Mayrhofer , H . 2001 . Identification of photobionts from the lichen family Physciaceae using algal-specific ITS rDNA sequencing . Lichenologist , 33 : 73 – 86 .
- Honegger , R . 2009 . “ Lichen-forming fungi and their photobionts ” . In Plant relationships V, The Mycota, , 2nd , Edited by: Deising , H.B. 305 – 333 . Berlin : Springer-Verlag .
- Johnson , LR and John , DM . 1990 . Observations on Dilabifilum (Class Chlorophyta, order Chaetophorales sensu stricto) and allied genera . Eur. J. Phycol. , 25 : 53 – 61 .
- Kohlmeyer , J and Volkmann-Kohlmeyer , B . 1998 . Mycophycias, a new genus for the mycobionts of Apophlaea, Ascophyllum and Pelvetia . Systema Ascomycetum , 16 : 1 – 7 .
- Kroken , S and Taylor , JW . 2000 . Phylogenetic species, reproductive mode, and specificity of the green alga Trebouxia forming lichens with the fungal genus Letharia . Bryologist , 103 : 645 – 660 .
- Lakatos , M , Lange-Bertalot , H and Büdel , B . 2004 . Diatoms living inside the thallus of the green algal lichen Coenogonium linkii in neotropical lowland rain forests . J. Phycol. , 40 : 70 – 73 .
- Lohtander , K , Oksanen , I and Rikkinen , J . 2003 . Genetic diversity of green algal and cyanobacterial photobionts in Nephroma (Peltigerales) . Lichenologist , 35 : 325 – 339 .
- LOKHORST, G.M. (1992). Taxonomic studies in the genus Heterococcus: (Tribophyceae, Tribonematales, Heteropediaceae); a combined cultural and electron microscopy study. In Cryptogamic Studies, vol 3 (Jülich, W., editor). Fischer, Stuttgart
- Lücking , R , Lawrey , JD , Sikaroodi , M , Gillevet , PM , Chaves , JL , Sipman , HJM and Bungartz , F . 2009 . Do lichens domesticate photobionts like farmers domesticate crops? Evidence from a previously unrecognized lineage of filamentous cyanobacteria . Am. J. Bot. , 96 : 1409 – 1418 .
- Lutzoni , F , Wagner , P , Reeb , V and Zoller , S . 2000 . Integrating ambiguously aligned regions of DNA sequences in phylogenetic analyses without violating positional homology . Syst. Biol. , 49 : 628 – 651 .
- MADDISON, W.P. &; MADDISON, D.R. (2003). MacClade: Analysis of Phylogeny and Character Evolution. Version 4.6. Sinauer, Sunderland, MA
- Manhart , JR . 1994 . Phylogenetic analysis of green plant rbcL sequences . Mol. Phylogenet. Evol. , 3 : 114 – 127 .
- Mason-Gamer , R and Kellogg , E . 1996 . Testing for phylogenetic conflict among molecular datasets in the tribe Triticeae (Graminae) . Syst. Biol. , 45 : 524 – 545 .
- Miadlikowska , J , Kauff , F , Hoftstetter , V , Fraker , E , Grube , M , Hafellner , J , Reebe , V , Hodkinson , BP , Kukwa , M , Lücking , R , Hestmark , G , Otalora , MG , Rauhut , A , Büdel , B , Scheidegger , C , Timdal , E , Stenroos , S , Brodo , I , Perlmutter , GB , Ertz , D , Diederich , P , Lendemer , JC , May , P , Schoch , CL , Arnold , AE , Gueidan , C , Tripp , E , Yahr , R , Robertson , C and Lutzoni , F . 2006 . New insights into classification and evolution of the Lecanoromycetes (Pezizomycotina, Ascomycota) from phylogenetic analyses of three ribosomal RNA- and two protein-coding genes . Mycologia , 98 : 1088 – 1103 .
- Moe , R . 1997 . Verrucaria tavaresiae sp. nov., a marine lichen with a brown algal photobiont . Bull. California Lichen Soc. , 4 : 7 – 11 .
- Muggia , L , Gueidan , C , Perlmutter , GB , Eriksson , OE and Grube , M . 2009 . Molecular data confirm the position of Flakea papillata in the Verrucariaceae . Bryologist , 112 : 538 – 543 .
- Muggia , L , Gueidan , C and Grube , M . 2010 . Phylogenetic placement of some morphologically unusual members of Verrucariales . Mycologia , 102 : 835 – 846 .
- Negrisolo , E , Maistro , S , Incarbone , M , Moro , I , Dalla Valle , L , Broady , PA and Andreoli , C . 2004 . Morphological convergence characterizes the evolution of Xanthophyceae (Heterokontophyta): evidence from nuclear SSU rDNA and plastidial rbcL genes . Mol. Phylogenet. Evol. , 33 : 156 – 170 .
- Nelsen , MP and Gargas , A . 2006 . Actin type I introns offer potential for increasing phylogenetic resolution in Asterochloris (Chlorophyta: Trebouxiophyceae) . Lichenologist , 38 : 435 – 440 .
- Nelsen , MP , Rivas Plata , E , Andrew , CJ , Lücking , R and Lumbsch , HT . 2011 . Phylogenetic diversity of trentepohlialean algae associated with lichen-forming fungi . J. Phycol. , 47 : 282 – 290 .
- Nyati , S , Beck , A and Honegger , R . 2007 . Fine structure and phylogeny of green algal photobionts in the microfilamentous genus Psoroglaena (Verrucariaceae, lichen-forming ascomycetes) . Plant Biol. , 9 : 390 – 399 .
- O'Brien , H , Miadlikowska , J and Lutzoni , F . 2005 . Assessing host specialization in symbiotic cyanobacteria associated with four closely related species of the lichen fungus Peltigera . Eur. J. Phycol. , 40 : 363 – 378 .
- Parra , OO and Redon , J . 1977 . Aislamento de Heterococcus caespitosus Vischer ficobionte de Verrucaria maura Wahlenb . Bol. Soc. Biol. Concepción , 51 : 219 – 224 .
- Pérez-Ortega , S , de los Ríos , A , Crespo , A and Sancho , LG . 2010 . Symbiotic lifestyle and phylogenetic relationships of the bionts of Mastodia tessellata (Ascomycota, incertae sedis) . Am. J. Bot. , 97 : 738 – 752 .
- Persoh , D , Beck , A and Rambold , G . 2004 . The distribution of ascus types and photobiontal selection in Lecanoromycetes (Ascomycota) against the background of a revised SSU nrDNA phylogeny . Mycol. Prog. , 3 : 103 – 121 .
- Peters , AF and Moe , RL . 2001 . DNA sequences confirm that Petroderma maculiforme (Phaeophyceae) is the brown algal phycobiont of the marine lichen Verrucaria tavaresiae (Verrucariales, Ascomycota) from central California . Bull. California Lichen Soc. , 8 : 41 – 43 .
- Rambold , G , Friedl , T and Beck , A . 1998 . Photobionts in lichens: possible indicators of phylogenetic relationships? . Bryologist , 101 : 392 – 397 .
- ŘEHÁKOVÁ, H. (1968). Lisejnikové rasy z rodu Trebouxia, Diplosphaera a Myrmecia (Flechten der Gattungen Trebouxia, Diplosphaera und Myrmecia). Candidate dissertation, Katedra Bot., University of Karlova, Praha
- Rindi , F , McIvor , L , Sherwood , AR , Friedl , T , Guiry , MD and Sheath , RG . 2007 . Molecular phylogeny of the green algal order Prasiolales (Trebouxiophyceae, Chlorophyta) . J. Phycol. , 43 : 811 – 822 .
- Sanders , WB , Moe , RL and Ascaso , C . 2004 . The intertidal marine lichen formed by the Pyrenomycete fungus Verrucaria tavaresiae (Ascomycotina) and the brown alga Petroderma maculiforme (Phaeophyceae): thallus organization and symbiont interaction . Am. J. Bot. , 91 : 511 – 522 .
- Sanders , WB , Moe , RL and Ascaso , C . 2005 . Ultrastructural study of the brown alga Petroderma maculiforme (Phaeophyceae) in the free-living state and in lichen symbiosis with the intertidal marine fungus Verrucaria tavaresiae (Ascomycotina) . Eur. J. Phycol. , 40 : 353 – 361 .
- Savić , S , Tibell , L , Gueidan , C and Lutzoni , F . 2008 . Molecular phylogeny and systematics of Polyblastia (Verrucariaceae, Eurotiomycetes) and allied genera . Mycol. Res. , 112 : 1307 – 1318 .
- Sherwood , AR , Garbary , DJ and Sheath , RG . 2000 . Assessing the phylogenetic position of the Prasiolales (Chlorophyta) using rbcL and 18S rRNA gene sequence data . Phycologia , 39 : 139 – 146 .
- Skaloud , P and Peksa , O . 2010 . Evolutionary inferences based on ITS rDNA and actin sequences reveal extensive diversity of the common lichen alga Asterochloris (Trebouxiophyceae, Chlorophyta) . Mol. Phylogenet. Evol. , 54 : 36 – 46 .
- Stahl , E . 1874 . Beiträge zur Entwicklungsgeschichte der Flechten (vorläufige Mittheilung) . Bot. Zeitung , 1874 : 177 – 180 .
- Stamatakis , A , Ludwig , T and Meier , H . 2005 . RAxML-III: A fast program for maximum likelihood-based inference of large phylogenetic trees . Bioinformatics , 21 : 456 – 463 .
- Stamatakis , A , Hoover , P and Rougemont , J . 2008 . A rapid bootstrap algorithm for the RAxML web-servers . Syst. Biol. , 75 : 758 – 771 .
- STARR, R.C. & ZEIKUS, J.A. (1993). UTEX – The culture collection of algae at the University of Texas at Austin. J. Phycol., Suppl. 29
- Thüs , H . 2002 . Taxonomie, Verbreitung und Ökologie silicoler Süsswasserflechten im ausseralpinen Mitteleuropa . Bibl. Lichenol. , 83 : 1 – 214 .
- Thüs , H and Nascimbene , J . 2008 . Contributions toward a new taxonomy of Central European freshwater species of the lichen genus Thelidium (Verrucariales, Ascomycota) . Lichenologist , 40 : 499 – 521 .
- Thüs , H and Schultz , M . 2008 . Freshwater Flora of Central Europe, Vol. 21/1: Fungi, Part 1: Lichens , Heidelberg : Spektrum .
- Trembley , ML , Ringli , C and Honegger , R . 2002 . Morphological and molecular analysis of early stages in the resynthesis of the lichen Baeomyces rufus . Mycol. Res. , 106 : 768 – 776 .
- Tschermak , E . 1941a . Beitrag zur Entwicklungsgeschichte und Morphologie der Protococcale Trochiscia granulata . Österr. Bot. Z. , 90 : 67 – 73 .
- Tschermak , E . 1941b . Untersuchungen über die Beziehungen von Pilz und Alge im Flechtenthallus . Österr. Bot. Z. , 90 : 233 – 307 .
- Tschermak-Woess , E . 1970 . Über wenig bekannte und neue Flechtengonidien V. Der Phycobiont von Verrucaria aquatilis und die Fortpflanzung von Pseudopleurococcus arthopyreniae . Österr. Bot. Z. , 118 : 443 – 455 .
- Tschermak-Woess , E . 1976 . “ Algal taxonomy and the taxonomy of lichens: the phycobiont of Verrucaria adriatica ” . In Lichenology: Progress and Problems , Edited by: Brown , D.H. , Hawksworth , D.L. and Bailey , R.H. 79 – 88 . London : Academic Press .
- Tschermak-Woess , E . 1985 . Elliptochloris bilobata kein ganz seltner Phycobiont . Herzogia , 7 : 105 – 116 .
- Tschermak-Woess , E . 1988 . “ The algal partner ” . In CRC Handbook of Lichenology , Edited by: Galun , M. Vol. I , 39 – 92 . Boca Raton : CRC Press .
- White , TJ , Bruns , T , Lee , SB and Taylor , JW . 1990 . “ Amplification and direct sequencing of fungal ribosomal RNA genes for phylogenetics ” . In A Guide to Methods and Applications, PCR Protocols , Edited by: Gelfand , M.A. , Sninsky , J.J. and White , T.J. 315 – 322 . San Diego : Academic press .
- Winter , G . 1875 . Ueber die Gattung Sphaeromphale und Verwandte. Ein Beitrag zur Anatomie der Krustenflechten . Pringsh. Jahrb. wiss. Bot. , 10 : 245 – 274 .
- Yahr , R , Vilgalys , R and DePriest , PT . 2006 . Geographic variation in algal partners of Cladonia subtenuis (Cladoniaceae) highlights the dynamic nature of a lichen symbiosis . New Phytol. , 171 : 847 – 860 .
- Zeitler , I . 1954 . Untersuchungen uber die Morphologie, Entwicklungsgeschichte und Systematik von Flechtengonidien . Österr. Bot. Z. , 101 : 453 – 487 .
- Zhou , S and Stanosz , GR . 2001 . Primers for the amplification of mtSSU rDNA, and a phylogenetic study of Botryosphaeria and associated anamorphic fungi . Mycol. Res. , 105 : 1033 – 1044 .
- Zolan , ME and Pukkila , PJ . 1986 . Inheritance of DNA methylation in Coprinus cinereus . Mol. Cell. Biol. , 6 : 195 – 200 .
- Zoller , S , Scheidegger , C and Sperisen , C . 1999 . PCR primers for the amplification of mitochondrial small subunit ribosomal DNA of lichen-forming Ascomycetes . Lichenologist , 31 : 511 – 516 .
Supplementary material
The following supplementary material is available for this article, accessible via the Supplementary Content tab on the article's online page at http://dx.doi.org/10.1080/09670262.2011.629788