Abstract
We investigated salinity-induced developmental plasticity and difference in the ‘reaction norm’ of clonal cultures (genotype–environment [G × E] interaction) of Cyclotella meneghiniana, isolated from different locations, and their F1 offspring. Genetic distances between strains and the phylogenetic position of each strain were investigated by partial 28S rDNA sequencing. In addition, we investigated morphological differences between cells obtained from different sites along a tidal river, from the lower reaches to the river mouth. In laboratory culture, all strains exhibited a greater number of central fultoportulae (CFP = central strutted processes) in saline medium (salinity, 17 psu) than in freshwater medium; the number of striae on the valve and the diameter of the central area increased in all but three strains. A significant G × E interaction was detected in the number of CFP, the number of striae, the diameter of the central area and the valve diameter. Pair-wise comparison showed significant G × E interactions for these traits among strain pairs. In the field-collected material, all parameters (CFP number, stria number, central area diameter and valve diameter) were greatest at the estuary mouth.
Introduction
Developmental plasticity, i.e. the ability of a single genotype to alter its developmental processes and phenotypic outcomes in response to different environmental conditions (Moczek et al., Citation2011), is a common phenomenon in various taxa. Developmental plasticity has been suggested as crucial in the formation of evolutionary novelties (West-Eberhard, Citation2003) and promoting innovation (Moczek et al., Citation2011). In unicellular organisms at an individual cell level, developmental plasticity is associated with morphological changes (Lürling, Citation2003; Luo et al., Citation2006; Bollmann et al., Citation2010).
Theoretical and empirical studies agree that selection for plasticity can result in phenotypic adaptation to different environmental conditions (reviewed in Ghalambor et al., Citation2007). A genotype-specific response to environmental variation is called the ‘reaction norm’ (Windig et al., Citation2004). Variation among genotypes in response to environments is referred to as a genotype–environment (G × E) interaction (e.g. Windig et al., Citation2004), which can be visualized by plotting the reaction norms of multiple genotypes. Evidence suggests that plasticity has evolved and can be visualized as a change in the slope of the reaction norm between ancestral and derived population or species (Gotthard & Nylin, Citation1995). However, G × E interactions in diatoms have not yet been explored.
Diatoms are unicellular eukaryotic microalgae with cell walls consisting of two siliceous valves, whose fine structure is mostly species-specific and constant (Round et al., Citation1990). On the other hand, developmental plasticity is observed in Thalassiosira decipiens (McMillan & Johansen, Citation1988) and Cyclotella meneghiniana in response to salinity changes (Håkansson & Korhola, Citation1998; Håkansson & Chepurnov, Citation1999), in T. tumida in response to temperature changes (Fryxell, Citation1986), and in T. weissflogii in response to grazing (Pondaven et al., Citation2007).
In this study, we focused on the diatom, Cyclotella meneghiniana, which is a common euryhaline diatom occurring in environments ranging from freshwater to estuarine (e.g. Håkansson & Korhola, Citation1998) and can be grown in culture in a wide range of salinities (Rao, Citation1978; Kopczyńska, Citation1979; Roubeix & Lancelot, Citation2008). It is one of the commonest diatom species (Finlay et al., Citation2002) and exhibits high genetic variability, containing several, perhaps many, genetically distinct but apparently reproductively isolated groups (Beszteri et al., Citation2005a , Citation2007). In addition, it has been suggested that Thalassiosirales species, including C. meneghiniana, have repeatedly moved between freshwater and marine environments, accompanied by speciation events (Alverson et al., Citation2007). The valve of C. meneghiniana shows a marginal zone of costae clearly delimited from a central zone (e.g. Håkansson & Korhola, Citation1998), which differentiates this species from C. cryptica. However, this difference between the two species can be generated by environmental change, since saline conditions can induce C. meneghiniana to produce a morphology similar to that of C. cryptica (Desikachary & Rao, Citation1973; Hoops & Floyd, Citation1979). Others have reported that salinity changes can induce variation in the number of central fultoportulae (CFP), the length of the striae (Håkansson & Chepurnov, Citation1999) and valve thickness (Tuchman et al., Citation1984) in C. meneghiniana cultures. However, quantitative measurements of the degree of developmental plasticity and G × E interactions have not been performed. Therefore, considering the evolutionary and ecological significance of this phenomenon, we investigated salinity-induced developmental plasticity and G × E interactions in clonal cultures of C. meneghiniana, after characterizing their phylogenetic positions. In addition, we investigated the valve morphology of natural populations exposed to different saline environments in a tidal river.
Materials and methods
Cultures
Clonal cultures were identified as C. meneghiniana by morphology and genetic distance of 28S rDNA (). lists the cultures and their origins. Strains of C. meneghiniana were established as single-cell isolates by removing cells from net haul material with a micropipette and then passing them through several washes in sterile media. Strains SR-0, SR-2, SR-3, MG-0 and HK () were deposited in the NIES culture collection as NIES-2365, NIES-2367, NIES-2368, NIES-2364 and NIES-2363, respectively. Cells were cultivated in plastic cups (16 cm2 × 4 cm) containing approximately 20 ml sterile modified Bold's basal medium (BBM; Bischoff & Bold, Citation1963) at 19°C under continuous illumination, with a light intensity of approximately 30 µmol photons m−2 s−1. We used continuous illumination for two reasons, (1) to keep the clones’ environment as homogeneous as possible, and (2) to maintain a high growth rate. The modified BBM was prepared by adding 10 mg l−1 Na2SiO3.9H2O, 20 µg l−1 cyanocobalamin, 1 µg l−1 biotin and 10 µg l−1 thiamine to BBM diluted 1 : 4 with deionized water; the modified BBM was used as the freshwater medium in salinity experiments. To ensure monoclonality of the cultures, single cells were reisolated from established cultures. After size reduction and induction of sexual reproduction by incubation in 30% seawater medium (following Schultz & Trainor, Citation1968; for the composition of the seawater medium, see below, ‘Effect of salinity’), F1 strains were established from single initial cells (SR-1, SR-2 and SR-3 were obtained from SR-0, and MG-1 from MG-0). These F1 strains may have been generated by oogamous reproduction, because we observed sperm and eggs during sexual reproduction. When cells reached a density of approximately 4 × 104 cells ml−1 (SR-0 and MG-0) or 1 × 104 cells ml−1 (SR-1, SR-2, SR-3 and HK), they were transferred to new media for experimentation and cultivated until mid-to-late exponential growth phase. We confirmed the absence of bacterial contamination by using phase-contrast microscopy.
Fig. 1. Phylogenetic tree of Cyclotella species and the strains used to investigate developmental plasticity based on Bayesian analysis (TN + G) of partial 28S rDNA sequences (D1/D2 regions). The numbers at the nodes are posterior probabilities (PP) above 0.50. DNA sequences determined in this study are in bold. Scale bar represents 3 substitutions per 100 nucleotides.
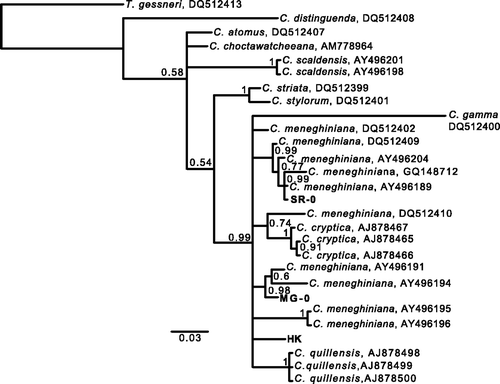
Table 1. Strain characteristics.
DNA extraction, polymerase chain reaction (PCR) and sequencing
Cells were harvested from cultures of SR-0, MG-0 and HK, and DNA was extracted using the DNeasy Plant Kit (QIAGEN, Germany). The D1–D2 regions of the nuclear 28S rDNA were amplified and sequenced using primers from Scholin et al. (Citation1994). PCR products were purified by ExoSap-IT (USB, OH, USA) and directly sequenced on both strands by using Big Dye Terminator v3.1 sequencing chemistry (Applied Biosystems, CA, USA). Sequencing products were electrophoresed on an ABI 3730 Avant sequencer (Applied Biosystems, CA, USA) by FASMAC DNA sequencing services (Atsugi, Japan).
Sequence assembly and analyses
High-confidence ambiguities (i.e. ambiguities at ‘the same position without sequencing noise around that position in both sequencing reactions’ and interpreted to be within-strain polymorphisms: Beszteri et al., Citation2005a ), were not found in the electropherograms. The 28S rDNA sequences of strains used in this study were compared with those of C. meneghiniana and other Cyclotella species (see Supplementary Table 1). For phylogenetic inferences for Cyclotella, we constructed data matrixes of 28S rDNA sequences (27 sequences, 10 species) (Supplementary Table 1). These sequences, including the out-group (T. gessneri AN02-08; GenBank accession number DQ512413), were aligned using the ClustalW build-in in Mega 5.0. The aligned sequences were trimmed to the same length (546 bases); the alignment has been deposited in TreeBase, accession 12757, and is also available here as a nexus file (Supplementary File 1). MEGA 5.0 was used to find the optimal model of DNA substitution for the Bayesian tree construction and selected the Tamura–Nei plus Gamma-distributed model (TN + G) (−lnL = −2261) by reference to the Akaike Information Criterion (AIC). For preparation of the phylogenetic tree, a Bayesian analysis was implemented with MrBayes 3.1.2 (Huelsenbeck & Ronquist, Citation2001). The Markov chain Monte Carlo (MCMC) process was set to two runs, four chains, default temperature (0.2) and one million generations. The sampling frequency was set to every 100 generations. After analysis, the first 2000 trees were deleted as burn-in and the consensus tree was constructed. The phylogenetic trees were visualized with FigTree v1.3.1 (available at http://tree.bio.ed.ac.uk/software/figtree/).
Effect of salinity
Three replicates of seven strains (SR-0, SR-1, SR-2, SR-3, MG-0, MG-1 and HK) were examined in freshwater and seawater media; the former contained 0% seawater (salinity 0), and the latter contained 50% seawater (final salinity, 17 psu), prepared by diluting f/2 medium (Guillard & Ryther, Citation1962) in freshwater medium. Daigo's artificial seawater (Wako Pure Chemical Industries, Osaka, Japan) was used for the preparation of f/2 medium. Approximately 2 × 103 cells ml−1 (SR-0 and MG-0) or 5 × 102 cells ml−1 (SR-1, SR-2, SR-3, MG-1 and HK) were inoculated into 20 ml media of different salinities in a plastic cup and incubated for 5 days (SR-1, SR-2, SR-3, MG-1 and HK) or 11 days (SR-0 and MG-0) until newly formed valves predominated in the experimental population. For labelling of the new daughter valves, the effect of salinity on the SR-1, SR-2, SR-3, MG-1 and HK strains was monitored in the presence of the fluorescent probe 2-(4-pyridyl)-5-((4-(2-dimethylaminoethylaminocarbamoyl)methoxy)phenyl)oxazole (100 nM PDMPO, LysoSensor™ DND-160 yellow/blue; Molecular Probes, Eugene, OR, USA), which is taken up during valve formation (Shimizu et al., Citation2001). We preliminarily confirmed that PDMPO had no effect on valve morphology. Although investigations of the effect of salinity on MG-0 and SR-0 were performed without PDMPO, most valves harvested after the experiment were thought to be newly formed because of the growth of these strains: the average numbers of divisions during the experiment were 4.453 (SR-0) and 4.009 (MG-0), giving average percentages of 95.4% (SR-0) and 93.0% (MG-0) of newly formed valves. The morphological traits (number of CFP, number of striae, diameter of the central area and valve diameter) were measured in 25 randomly selected valves from samples of each replicate experiment.
Measurements of valve morphology
The samples were boiled in 50% sulphuric acid with potassium dichromate to remove organic material. They were rinsed multiple times with distilled water, dried on coverslips, and mounted with Pleurax (Mount media; Wako Pure Chemical Industries, Osaka, Japan). Slides of strains (SR-0, SR-1, SR-2, SR-3, MG-0, MG-1 and HK) have been deposited in the National Museum of Nature and Science, Tokyo (as TNS-AL-57088, TNS-AL-57089, TNS-AL-57090, TNS-AL-57091, TNS-AL-57092, TNS-AL-57093, TNS-AL-57094 respectively). Phase-contrast microscopy was performed using a Leica DMLB (Leica Microscopy Systems, Heerbrugg, Switzerland) and ×100 phase-contrast objectives. The morphological traits were measured by capturing a digital image of each valve (DFC480 digital camera, Leica Microscopy Systems, Heerbrugg, Switzerland) and using the distance measurement function of ImageJ software (Abràmoff & Magalhães, Citation2004). The variables used for morphological analysis are shown in . The valve and central area diameters were measured in horizontal and vertical directions on the images and averaged to reduce measurement error. The number of CFP and striae were counted manually.
Observations of field samples
We collected samples from four sites located between the lower reaches and the estuary mouth of the Ara-kawa (Ara River) in Tokyo, Japan, on 15 October 2006 (). Samples of approximately 100 ml were obtained by hauling a 25 -µm plankton net four times horizontally over 10 m of the river surface. Surface water samples (shallower than 1 m) were taken simultaneously to measure salinity, pH and water temperature. Immediately after collection, the samples were fixed in 2% formaldehyde. Water quality at each collection site was assessed using the following parameters and equipment: salinity, optical refractometer (Is/ Mill-E: As-one, Osaka, Japan); pH, a glass electrode (MP225: Mettler Toledo, Tokyo, Japan); and water temperature, a glass mercury thermometer. Only valves with morphologies typical of C. meneghiniana were observed and any ambiguous morphology was excluded. Therefore, any C. meneghiniana valves that had C. cryptica-like morphologies were not measured. Morphological traits (number of CFP, number of striae, diameter of the central area and valve diameter) were measured for at least 45 randomly selected valves at each collection site.
Table 2. Collection sites and water quality data.
Statistical analysis
Statistical tests included Student's t-test and generalized linear model (GLM) and were conducted using R software, version 2.5.0. To examine the effect of salinity and strain on each morphological trait, GLM was used to generate an analysis of deviance table by using an F-test, with each trait as the dependent variable and strains and salinity as independent variables. To determine the effect of salinity and strain on the traits (G × E interaction), we generated an analysis of deviance table by using F-test for GLM fits, with traits as the dependent variable and salinity and strain as factors, and examined the significance of the interaction effect. We conducted pairwise multiple comparisons of G × E interaction in three strains that were originally isolated from field samples (SR-0, MG-0 and HK). In the field, there were sympatric differences in valve diameter and diatom morphological traits correlated with valve diameter (e.g. Johansen & Theriot, Citation1987; Wood et al., Citation1987; Theriot, Citation1988). Therefore, to compare morphological traits at each collection site while accounting for valve diameter, we conducted GLM analysis with collection site as a fixed-effects factor and valve diameter as a covariate. We examined the Akaike Information Criterion (AIC) to determine the best fitting model (Akaike, Citation1973). Consequently, a Poisson model was chosen for the number of CSP and a Gamma model was chosen for the number of striae, diameter of the central area, and valve diameter. For pairwise multiple comparisons after GLM, we adjusted the P value according to Holm's method (Holm, Citation1979). Differences were considered significant at P < 0.05. Pooled data are expressed as the mean ± standard error (SE).
Results
Strain identification and phylogenetic relationships
lists the cultures used in this study and their origins. Phylogenetic relationships between our strains and Cyclotella species were inferred from Bayesian phylogenetic analyses by using partial 28S rDNA sequences (). Deeper nodes including several Cyclotella species (C. atomus, C. choctawhatcheeana, C. scaldensis, C. striata, C. stylorum and C. distinguenda) had low posterior probability (PP) (<0.6). However, the strains obtained here were closely related to other C. meneghiniana strains and C. gamma, C. cryptica and C. quillensis with 0.99 PP; these species will be referred to as the ‘C. meneghiniana complex’. Cyclotella cryptica and C. quillensis formed a distinct group within the C. meneghiniana complex with 1.00 PP; no strains obtained in this study were included in the C. cryptica or C. quillensis clades. We observed 10–18 base differences in pairwise comparisons of the three strains (SR-0 vs. MG-0 showed a 10-base difference; SR-0 vs. HK showed 18; MG-0 vs. HK showed 17).
Developmental plasticity in clonal cultures
The effect of salinity for all traits (number of CFP, number of striae, diameter of the central area and valve diameter) was significant (, ). Pairwise multiple comparisons after GLM in seawater vs. freshwater medium showed that the numbers of CFP were significantly increased in all strains in seawater (). The number of striae, diameter of the central area, and valve diameter respectively increased, decreased and showed no response ().
Fig. 3. Morphological traits of seven clonal strains of C. meneghiniana in freshwater medium (FW: salinity 0) and 50% seawater medium (SW: salinity 17 psu). Reaction norms are shown for (a) the number of central fultoportulae (CFP), (b) the number of striae, (c) the diameter of the central area, and (d) the valve diameter. Bars indicate mean ± standard error (SE).
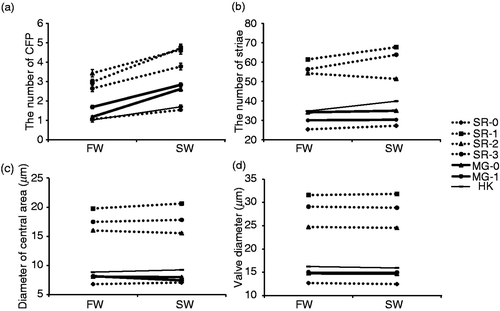
Table 3. The results of GLM summarizing the effect of salinity and strain on the traits of C. meneghiniana; d.f. = degrees of freedom.
Table 4. Pairwise comparison of the effect of salinity in each strain. ‘Increase’ and ‘decrease’ tabulate significant changes (P < 0.05) in seawater vs. freshwater; n.s. = not significant.
G × E interaction
We observed a significant interaction between strain and salinity for all traits (, ). The subsequent pairwise comparisons by GLM were conducted to focus on three strains isolated from different locations (SR-0, MG-0 and HK) (). A significantly different reaction norm for the number of striae was exhibited in SR-0 vs. MG-0, SR-0 vs. HK and MG-0 vs. HK (, ). Moreover, for the diameter of the central area, SR-0 vs. MG-0 and MG-0 vs. HK showed significantly different reaction norms (, ).
Table 5. Pairwise comparison of G × E interactions (SR-0, MG-0 and HK).
Field observations
Differences in morphological traits at each collection site along the Ara-kawa () are shown in . To compare the morphological traits at each collection site after accounting for valve diameter, we conducted GLM with diameter as a covariate. For all traits, collection site had a significant effect (). A post hoc pairwise comparison was conducted for each trait. All morphological traits had significantly higher mean values in samples from the estuary mouth (point D) than those in samples from the upper reaches of the river (Points A, B and C) (, ). In all other pairwise combinations by collection site, no significant difference in these traits was observed (, ); however, for the number of striae and diameter of the central area, interactions between collection site and valve diameter were significant ().
Fig. 4. Morphological traits of specimens at each collection site (A, B, C and D). (a) Number of central fultoportulae (CFP), (b) number of striae, (c) diameter of the central area, (d) valve diameter. Bars indicate mean ± standard error (SE).
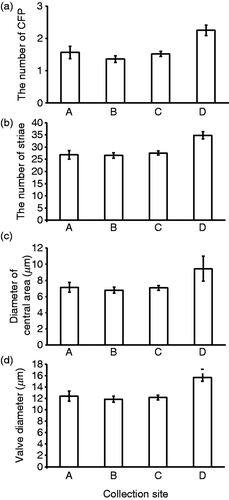
Table 6. The effect of collection site and valve diameter on C. meneghiniana traits. For ‘significant pairs’ the level of significance used was P = 0.05
Discussion
Species identification and genetic differences
The strains used in this study (SR-0, MG-0 and HK) were included in the clade of C. meneghiniana complex and were not in the C. cryptica or C. quillensis clades. SR-0, MG-0 and HK were established from different isolated localities (). In addition, these cultures had 10–18 base differences in their 28S r DNA sequences. Based on the locality and DNA sequence, we conclude that the populations of origin for these three strains have an extremely low rate of genetic exchange.
Laboratory and field results
In the laboratory, the number of CFP was higher in all strains in seawater, while in the field, the number of CFP was highest in samples from the estuary mouth. Moreover, in the laboratory, the number of striae and diameter of the central area increased in all but three strains in seawater. In the field, the number of striae and diameter of the central area were highest in the estuary mouth sample but qualified by the interaction between collection site and valve diameter (). The number of striae and the number of marginal fultoportulae are strongly related in C. meneghiniana, since 95% of the marginal costae separating the striae have marginal fultoportulae (Beszteri et al., Citation2005b ). Therefore, the number of fultoportulae will increase with the number of striae and costae under conditions of greater salinity. The fultoportula is a structure that extrudes β-chitin fibrils (McLachlan et al., Citation1965; Herth & Zugenmaier, Citation1977; Herth & Barthlott, Citation1979), which mediate aggregation (Herth & Barthlott, Citation1979) and cell buoyancy (Walsby & Xypolyta, Citation1977). Increase in the number of fultoportulae may therefore facilitate adaptation to buoyancy changes accompanying higher salinity by promoting chitin fibril formation. Formation of a local environment around cells by aggregation may help reduce salt stress associated with changing salinity. In addition, striae are composed of rows of pores (areolae) that mediate the flux of nutrients and exudates across the cell membrane (Round et al., Citation1990). Therefore, changes in the number of striae and the diameter of the central hyaline area in response to changes in salinity may also affect metabolic activity. At the population level, increasing salinity increases growth rate (with the maximum growth rate occurring at a salinity of 18 psu according to Roubeix & Lancelot, Citation2008), adhesion to valve faces of sister cells (Håkansson & Chepurnov, Citation1999), and aggregation of entangled chitin fibrils (Y. Shirokawa, unpublished data). We plan to investigate the relationship between these fine morphological changes and population-level responses.
In the laboratory, the valve diameter was slightly changed in three strains (, ). Changes in salinity can induce changes in valve thickness in C. meneghiniana cultures (Tuchman et al., Citation1984), and consequent increments in valve flexibility may allow distortion of the external shape and slight diameter changes. In the field, diatoms from the site of greatest salinity, site D, had a greater valve diameter than diatoms from other sites. However, these regional cell size differences could also reflect differences in the time elapsed since sexual reproduction in the different populations. In laboratory cultures of C. meneghiniana, salinity is one of the environmental cues that trigger sexual reproduction. Thus, Schultz & Trainor (Citation1968) observed that sexual reproduction of C. meneghiniana did not occur in 25% seawater, but did occur in 50% seawater (equivalent of salinity to that of site D in this study). Hence it is possible that sexual reproduction may have been induced at the river mouth, where salinity was high and have caused the greater valve diameter at site D. Salinity differences in the lower reaches and estuary mouth may be important factors in the morphological differences observed in our field study. However, further study of other environmental factors, such as water velocity, nutrient availability and light intensity and its seasonal changes should also be addressed, because these may have differed at our collection sites.
G × E interactions among strains
Many studies have reported G × E interactions in a variety of organisms, and the common observation of genetic variation for plasticity within populations implies that it can evolve in response to natural selection (Pigliucci, Citation2005). In this study, we observed significant G × E interactions (). However, to discuss whether G × E interactions maintain genetic variation, the changing rank order of fitness across environments must be investigated (Clausen et al., 1941; Via, Citation1991; Bennington & McGraw, Citation1995).
Significant G × E interactions were observed for different traits depending on the strain pairs (). Therefore, the degree of change in each trait may be regulated and selected individually. Although the mechanisms involved in the formation of microscale diatom structures (e.g. striae, fultoportulae) are not yet completely understood, microtubules and microfilamentous actin are known to play significant roles in the positioning of pattern elements of the valve. Tesson & Hildebrand (Citation2010) revealed that valve formation proceeds with actin and the silica deposition vesicle interdigitated. They also revealed that misalignment of ribs and mispositioning of portulae occurs under the influence of microtubule inhibitors in C. cryptica. Current research in developmental plasticity involves characterization of the molecular mechanisms that link variation in external environmental cues to the changes in organismal development that result in the production of different phenotypes (e.g. Beldade et al., Citation2011). In C. meneghiniana, differences in the reaction norms of various strains may be caused by differences in the genes or alleles that regulate the behaviour of microtubules and microfilamentous actin during salinity-induced developmental changes.
Evolutionary implications
Adaptive plasticity can promote establishment and persistence of organisms in a new environment and even non-adaptive plasticity can facilitate adaptive evolution in some situations (Ghalambor et al., Citation2007). Cyclotella species occur in a wide range of saline environments, and as many as three independent freshwater-to-marine colonizations have been postulated, two of which led to speciation events (Alverson et al., Citation2007). In addition, the traits investigated in this study (number of CFP, number of striae and diameter of central area) are important for the identification of Cyclotella species (e.g. Lowe, Citation1975; Håkansson & Kling, Citation1994; Muylaert & Sabbe, Citation1996). Further investigation of how saline-induced plasticity in these traits contributes to morphological innovation and evolution is planned.
Supplemental Material
Download Zip (17.7 KB)Acknowledgements
We are grateful to M. Shimada, A. Kuwata, D.H. Jewson and H. Iida for offering valuable comments on this study, to S. Nakagawa and O. Ishii for technical advice on DNA extraction and PCR, and to K. Sasakawa for advice on phylogenetic analysis. We also thank the Arakawa Karyu River Office for providing data on the distance of each collection site from the river mouth. Y. Shirokawa was a recipient of a Research Fellowship for Young Scientists from JSPS.
References
- Abràmoff , M and Magalhães , P . 2004 . Image processing with Image . J Biophotonics International , 11 : 36 – 42 .
- Akaike , H . 1973 . Maximum likelihood identification of Gaussian autoregressive moving average models . Biometrika , 60 : 255 – 265 .
- Alverson , AJ , Jansen , RK and Theriot , EC . 2007 . Bridging the Rubicon: phylogenetic analysis reveals repeated colonizations of marine and fresh waters by thalassiosiroid diatoms . Molecular Phylogenetics and Evolution , 45 : 193 – 210 .
- Beldade , P , Mateus , RA and Keller , RA . 2011 . Evolution and molecular mechanisms of adaptive developmental plasticity . Molecular Ecology , 20 : 1347 – 1363 .
- Bennington , CC and McGraw , JB . 1995 . Natural-selection and ecotypic differentiation in Impatiens pallida . Ecological Monographs , 65 : 303 – 323 .
- Beszteri , B , Ács , E and Medlin , L . 2005a . Ribosomal DNA sequence variation among sympatric strains of the Cyclotella meneghiniana complex (Bacillariophyceae) reveals cryptic diversity . Protist , 156 : 317 – 333 .
- Beszteri , B , Ács , E and Medlin , LK . 2005b . Conventional and geometric morphometric studies of valve ultrastructural variation in two closely related Cyclotella species (Bacillariophyta) . European Journal of Phycology , 40 : 89 – 103 .
- Beszteri , B , John , U and Medlin , L . 2007 . An assessment of cryptic genetic diversity within the Cyclotella meneghiniana species complex (Bacillariophyta) based on nuclear and plastid genes, and amplified fragment length polymorphisms . European Journal of Phycology , 42 : 47 – 60 .
- Bischoff , HW and Bold , HC . 1963 . Phycological studies IV. Some soil algae from Enchanted Rock and related algal species , University of Texas Publication no. 6318, Austin, Texas .
- Bollmann , J , Klaas , C and Brand , LE . 2010 . Morphological and physiological characteristics of Gephyrocapsa oceanica var. typica Kamptner 1943 in culture experiments: evidence for genotypic variability . Protist , 161 : 78 – 90 .
- CLAUSEN, J. (1949). Genetics of climatic races of Potentilla glandulosa. Proceedings of 8th International Congress of Genetics. Hereditas 35, Supplementary volume 1: 162–172
- Desikachary , TV and Rao , VNR . 1973 . Studies on Cyclotella meneghiniana Kütz. III. The frustule . Proceedings of the Indian Academy of Science , 77B : 78 – 91 .
- Finlay , B , Monaghan , E and Maberly , SC . 2002 . Hypothesis: the rate and scale of dispersal of freshwater diatom species is a function of their global abundance . Protist , 153 : 261 – 273 .
- FRYXELL, G.A. (1986). Polymorphism in relation to environmental conditions as exemplified by clonal cultures of Thalassiosira tumida (Janisch) Hasle. In Proceedings of the 9th International Diatom Symposium (Round, F.E., editor) 61–73. Biopress, Bristol and O. Koeltz, Koenigstein
- Ghalambor , C , McKay , JK , Carroll , SP and Reznick , DN . 2007 . Adaptive versus non-adaptive phenotypic plasticity and the potential for contemporary adaptation in new environments . Functional Ecology , 21 : 394 – 407 .
- Gotthard , K and Nylin , S . 1995 . Adaptive plasticity and plasticity as an adaptation: a selective review of plasticity in animal morphology and life history . Oikos , 74 : 3 – 17 .
- Guillard , RRL and Ryther , JH . 1962 . Studies of marine planktonic diatoms I. Cyclotella nana Hustedt, and Detonula confervacea (Cleve) Gran . Canadian Journal of Microbiology , 8 : 229 – 239 .
- Håkansson , H and Chepurnov , V . 1999 . A study of variation in valve morphology of the diatom Cyclotella meneghiniana in monoclonal cultures: effect of auxospore formation and different salinity conditions . Diatom Research , 14 : 251 – 272 .
- Håkansson , H and Kling , H . 1994 . Cyclotella agassizensis nov. sp. and its relationship to C. quillensis Bailey and other prairie Cyclotella species . Diatom Research , 9 : 289 – 301 .
- Håkansson , H and Korhola , A . 1998 . Phenotypic plasticity in the diatom Cyclotella meneghiniana or a new species? . Nova Hedwigia , 66 : 187 – 196 .
- Herth , W and Barthlott , W . 1979 . The site of β-chitin fibril formation in centric diatoms. I. Pores and fibril formation . Journal of Ultrastructure Research , 68 : 6 – 15 .
- Herth , W and Zugenmaier , P . 1977 . Ultrastructure of the chitin fibrils of the centric diatom Cyclotella cryptica . Journal of Ultrastructure Research , 61 : 230 – 239 .
- Holm , S . 1979 . A simple sequentially rejective multiple test procedure . Scandinavian Journal of Statistics , 6 : 65 – 70 .
- Hoops , HJ and Floyd , GL . 1979 . Ultrastructure of the centric diatom Cyclotella meneghiniana: vegetative cell and auxospore development . Phycologia , 18 : 424 – 435 .
- Huelsenbeck , JP and Ronquist , F . 2001 . MRBAYES: Bayesian inference of phylogenetic trees . Bioinformatics , 17 : 754 – 755 .
- Johansen , JR and Theriot , E . 1987 . The relationship between valve diameter and number of central fultoportulae in Thalassiosira wessflogii (Bacillariophyceae) . Journal of Phycology , 23 : 663 – 665 .
- Kopczyńska , EE . 1979 . Chloride effect on the growth of Cyclotella meneghiniana Kütz. and Melosira granulata (Ehr.) Ralfs . Polish Archives of Hydrobiology , 26 : 587 – 594 .
- Lowe , RL . 1975 . Comparative ultrastructure of the valves of some Cyclotella species (Bacillariophyceae) . Journal of Phycology , 11 : 415 – 424 .
- Luo , W , Pflugmacher , S , Pröschold , T , Walz , N and Krienitz , N . 2006 . Genotype versus phenotype variability in Chlorella and Micractinium (Chlorophyta, Trebouxiophyceae) . Protist , 157 : 315 – 333 .
- Lürling , M . 2003 . Phenotypic plasticity in the green algae Desmodesmus and Scenedesmus with special reference to the induction of defensive morphology . Annales de Limnologie – International Journal of Limnology , 39 : 85 – 101 .
- McLachlan , J , McInnes , AG and Falk , M . 1965 . Studies on the chitan (Chitin: poly-N-acetylglucosamine) fibers of the diatom Thalassiosira fluviatilis Hustedt. I. Production and isolation of chitan fibers . Canadian Journal of Botany , 43 : 707 – 713 .
- McMillan , M and Johansen , J . 1988 . Changes in valve morphology of Thalassiosira decipiens (Bacillariophyceae) cultured in media of four different salinities . European Journal of Phycology , 23 : 307 – 316 .
- MOCZEK, A.P., SULTAN, S., FOSTER, S., LEDÓN-RETTIG, C., DWORKIN, I., NIJHOUT, H.F., ABOUHEIF, E. & PFENNIG, D.W. (2011). The role of developmental plasticity in evolutionary innovation. Proceedings of the Royal Society of London, series B, 278: 2705–2713
- Muylaert , K and Sabbe , K . 1996 . Cyclotella scaldensis spec. nov. (Bacillariophyceae), a new estuarine diatom . Nova Hedwigia , 63 : 335 – 345 .
- Pigliucci , M . 2005 . Evolution of phenotypic plasticity: where are we going now? . Trends in Ecology and Evolution , 20 : 481 – 486 .
- Pondaven , P , Gallinari , M , Chollet , S , Bucciarelli , E , Sarthou , G , Schultes , S and Jean , F . 2007 . Grazing-induced changes in cell wall silicification in a marine diatom . Protist , 158 : 21 – 28 .
- Rao , VNR . 1978 . Studies on Cyclotella meneghiniana Kütz. IV. Progressive diminution in cell size . Proceedings of the Indian Academy of Science, section B , 87 : 1 – 15 .
- Roubeix , V and Lancelot , C . 2008 . Effect of salinity on growth, cell size and silicification of an euryhaline freshwater diatom: Cyclotella meneghiniana Kütz . Transitional Waters Bulletin , 1 : 31 – 38 .
- Round , F , Crawford , R and Mann , DG . 1990 . The Diatoms: Biology and Morphology of the Genera , Cambridge University Press, Cambridge .
- Scholin , C , Herzog , M , Sogin , M and Anderson , D . 1994 . Identification of group- and strain-specific genetic markers for globally distributed Alexandrium (Dinophyceae). 2. Sequence analysis of a fragment of the LSU rRNA gene . Journal of Phycology , 30 : 999 – 1011 .
- Schultz , ME and Trainor , FR . 1968 . Production of male gametes and auxospores in the centric diatoms Cyclotella meneghiniana and C. cryptica . Journal of Phycology , 4 : 85 – 88 .
- Shimizu , K , Amo , YD , Brzezinski , MA , Stucky , GD and Morse , DE . 2001 . A novel fluorescent silica tracer for biological silicification studies . Chemistry and Biology , 8 : 1051 – 1060 .
- Tesson , B and Hildebrand , M . 2010 . Dynamics of silica cell wall morphogenesis in the diatom Cyclotella cryptica: substructure formation and the role of microfilaments . Journal of Structural Biology , 169 : 62 – 74 .
- Theriot , E . 1988 . An empirically based model of variation in rotational elements in centric diatoms with comments on ratios in phycology . Journal of Phycology , 24 : 400 – 407 .
- Tuchman , ML , Theriot , E and Stoermer , EF . 1984 . Effect of low salinity concentrations on the growth of Cyclotella meneghiniana Kütz . Archiv für Protistenkunde , 128 : 319 – 326 .
- Via , S . 1991 . The genetic-structure of host plant adaptation in a spatial patchwork – demographic variability among reciprocally transplanted pea aphid clones . Evolution , 45 : 827 – 852 .
- Walsby , AE and Xypolyta , A . 1977 . The form resistance of chitan fibres attached to the cells of Thalassiosira fluviatilis Hustedt . European Journal of Phycology , 12 : 215 – 223 .
- West-Eberhard , MJ . 2003 . Developmental Plasticity and Evolution , Oxford University Press, New York .
- Windig , JJ , De Kovel , CGF and De Jong , G . 2004 . “ Genetics and mechanics of plasticity ” . In Phenotypic Plasticity , Edited by: Dewitt , TJ and Scheiner , SM . 31 – 49 . New York : Oxford University Press .
- Wood , AM , Lande , R and Fryxell , GA . 1987 . Quantitative genetic analysis of morphological variation in an Antarctic diatom grown at two light intensities . Journal of Phycology , 23 : 42 – 54 .
supplementary material
The following supplementary material is available for this article, accessible via the Supplementary Content tab on the article's online page at http://dx.doi.org/10.1080/09670262.2012.697580.
Supplementary Table 1. Origin of the strains of Cyclotella species and GenBank accession numbers for DNA sequences.