Abstract
A new species of raphid pennate diatom producing a blue pigment, Haslea karadagensis Davidovich, Gastineau & Mouget, sp. nov., was recently isolated from the Crimean coast of the Black Sea (Ukraine). This organism is very similar to the well-known Haslea ostrearia, the first described ‘blue’ diatom, which produces marennine, the pigment involved in the greening of oysters. The Ukrainian diatom, H. karadagensis, differs slightly from H. ostrearia in the structure of its frustule, and the two organisms are unable to interbreed. Two molecular markers, rbcL and the ITS1–5.8S–ITS2 sequences, showed 2% and >50% differences, respectively, between the two species. UV-visible spectrophotometry and in vivo confocal micro-Raman spectroscopy were used to compare the pigment of H. karadagensis with marennine. Both pigments showed absorption bands in the UV and red regions, but the positions of the maxima differ between the pigments. Significant differences were observed by micro-Raman spectroscopy in the 1000–1700 cm−1 wavenumber range, revealing that the pigments are different molecules. Haslea karadagensis is the first example of a new ‘blue’ diatom and produces a novel blue pigment.
Introduction
The raphid pennate marine diatom, Haslea ostrearia (Gaillon) Simonsen, is a tychopelagic or benthic organism, known for its ability to produce a water-soluble blue pigment, commonly called marennine, which accumulates predominantly in the apical regions of the cells. In oyster ponds of western France, where H. ostrearia can regularly become dominant, marennine is released into the seawater and is responsible for the ‘greening’ of oysters. This phenomenon, which has a marked economic impact locally, has long been known (Sprat, Citation1667). The involvement of a microscopic alga was recognized later but still long ago, and the organism was first described as Vibrio ostrearius (Gaillon, Citation1820; Dyer, Citation1877), then as Navicula ostrearia, and finally transferred to the genus Haslea (Simonsen, Citation1974). The name ‘marennine’ originates from the Marennes–Oléron region (Lankester, Citation1886), an area in south-western France long dedicated to bivalve production.
Over the past two centuries, diatoms with blue apices have been observed on all French coasts, from the Channel to the Mediterranean Sea, and they have all been ascribed to H. ostrearia (reviewed by Neuville & Daste, Citation1978). In the Mediterranean, they have been reported from France, near Banyuls (Sauvageau, Citation1906) and Boulouris (Gert Hansen, Scandinavian Culture Collection of Algae & Protozoa, Copenhagen); Sardinia (Solazzi & Tolomio, Citation1976; Tolomio, Citation1978); and Sicily, both in the harbour of Palermo (Barone, Citation1979) and on the north-western coast (Rossella Barone, personal communication). ‘Blue’ diatoms have also been reported from the Adriatic Sea (Molisch, Citation1903), the Suez canal (Madkour et al., Citation2007), and the Black Sea, in the north Caucasus (Proschkina-Lavrenko, Citation1963).
Diatoms with blue tips, recorded as H. ostrearia, have also been reported from almost all seas and oceans, in both northern and southern hemispheres, either from direct observation or by a greening effect on bivalves. In north-western Europe, records come from the coasts of Great Britain (Sprat, Citation1669; Hustedt & Aleem, Citation1951), Belgium (M’harzi et al., Citation1998), Norway (Hendey, Citation1964), Sweden (Wulff et al., Citation2000), Denmark (Limfjord; Petersen, Citation1916), and the Baltic Sea (Snoeijs & Kasperovičienė, Citation1996). In the northern Atlantic Ocean, ‘blue diatoms’ have been found in the Canary Islands (SCCAP, Gert Hansen), and different places along the North American coast, including North Carolina (Beaufort) and Rhode Island (Narragansett Bay) (Kennett & Hargraves, Citation1991; Gary Wickfors, personal communication); Virginia (Chesapeake Bay; Mitchell & Barney, Citation1918); New Jersey (Sullivan, Citation1977); Florida (Biscayne Bay; Julian Sprung, personal communication); and possibly in Honduras (Grunow, Citation1877). In the northern Pacific Ocean, H. ostrearia has been reported from the San Juan Islands, between Washington state and British Columbia (Hardy, Citation1973), and from Japan (Ranson, Citation1937). In the southern hemisphere, diatoms identified as H. ostrearia have been observed in the Indian Ocean (Simonsen, Citation1974), and reported from northern (Ricard, Citation1987), eastern (Tasmania, Volkman et al., Citation1994) and western Australia (Leschenault Estuary; Wasela Hosja, personal communication). These records suggested that the only microalga known to produce a blue pigment had a worldwide distribution.
In spring 2008, samples taken from the rocky shores of the Karadag Natural Reserve in the Crimea (Ukraine) were found to contain diatoms with dark bluish apices. Some of these cells were isolated and clones were cultured in controlled conditions. A protocol previously used for the induction of sexual reproduction in H. ostrearia (Davidovich et al., Citation2009; Mouget et al., Citation2009) was successfully applied to this diatom and auxospores and an F1 generation was obtained (Davidovich et al., Citation2012). The discovery of the Ukrainian Haslea raises concerns about the identity of other ‘blue’ diatoms. Most records of H. ostrearia have relied on species determination using light microscopy alone. Images from scanning electron microscopy (SEM) were published by Simonsen (Citation1974), who studied the frustule of H. ostrearia and transferred the species from Navicula to Haslea. It is highly unlikely that all the diatoms recorded as Navicula/Haslea ostrearia have been studied with SEM. Moreover, to the best of our knowledge, no publication has compared the chemistry of the blue pigments of any other strains, and the uniqueness of marennine, as the only blue pigment produced by diatoms, has never been questioned.
This paper will first focus on the identification and description of a new ‘blue diatom’, using biometry of the natural populations, and light and electron microscopy of cell and frustule morphology. A preliminary molecular characterization was undertaken to allow comparison with H. ostrearia. For this, we used two different markers: the ITS (Internal Transcribed Spacer) region, ITS1–5.8S–ITS1, a nuclear marker that has been widely used in diatoms (Alverson, Citation2008; Medlin & Kooistra, Citation2010), and rbcL, a chloroplast gene coding for the RubisCO large sub-unit, which has recently been receiving more interest (Hamsher et al., Citation2011). We also tested reproductive compatibility between the Ukrainian diatom and H. ostrearia. In addition, UV-visible spectrophotometry, and especially in vivo confocal micro-Raman spectroscopy, were used to investigate similarities and differences between the pigments of H. ostrearia and the new diatom. Raman spectroscopy can be used to provide a qualitative and quantitative analysis of numerous organic and mineral compounds (Spiro, Citation1974; Jolivet et al., Citation2008; Trinkler et al., Citation2010), and has been used to discriminate between pigments in organisms such as molluscs (Hedegaard et al., Citation2006) and corals (Fan & Yang, Citation2008; Nowak et al., 2009). It has also been used to measure in vivo pigment spectra of chlorophylls and carotenoids in the Prymnesiophyceae, Chlorophyceae, Bacillariophyceae and Cyanobacteria (Wu et al., Citation1998), and to quantify in vivo the amount of domoic acid synthesized by the diatom Pseudonitzschia multiseries, for comparison with HPLC results (Wu et al., Citation2000). We will demonstrate that micro-Raman spectroscopy is also a powerful tool for revealing differences between uncommon biomolecules, such as blue algal pigments. Compared with infra-red spectroscopy, this technique of vibrational spectroscopy has a distinctive advantage for samples and biomaterials immersed in water because of its low sensitivity to water content. Furthermore, Raman analyses do not require purified pigment, which in the case of marennine would require the generation of large biomass and time-consuming harvesting and purification processes (Pouvreau et al., Citation2006b ).
Materials and methods
Algal isolation and culturing
Naviculoid diatoms with blue tips were isolated from rocks collected from the shores of the Black Sea, Crimea (Ukraine), near the Karadag Biological Station (46° 53′ 40″ N, 35° 12′ 05″ E) in spring 2008. Isolates from natural populations were re-isolated at least five times before being considered monoclonal, and studied as H. karadagensis. For this work, different clones of H. karadagensis were used, ten derived from natural populations sampled in March–May 2008 (NCC 237 to NCC 244, Nantes Culture Collection), and in June 2010 (10607A and 10607C, Karadag Natural Reserve collection), and some other clones isolated from progenies obtained after heterothallic reproduction (NCC 313 to 316 isolated in January 2009). We also used different clones of H. ostrearia identified according to Simonsen (Citation1974), NCC 141, 148 and 234 isolated from phytoplankton sampled in the Bay of Bourgneuf (France, 46° 59′ 19″ N, 2° 14′ 14″ W), NCC 148.14c, 148.7, 148.75, 148.78, isolated from homothallic events happening spontaneously in NCC 148 cultures, and clones NCC 158.1, 158.4, resulting from sexual reproduction in the laboratory (crossing NCC 141 × NCC 148.7). Cultures were grown in Petri dishes or Erlenmeyer flasks containing artificial seawater (Perkins et al., Citation2006), under conditions of 15 ± 1°C, an irradiance of 60 µmol photons m−2 s−1, and a 14:10 h L:D cycle.
Light microscopy, biometry, and statistical analysis
For light microscopy (LM) of live cells, we used the ×40 objective of either an Olympus CH-2 microscope (Olympus, France) or a Biolar PI microscope (PZO, Poland), equipped with a JVC TK-C1381 or a PowerShot A95 digital camera, respectively. Photographs of cleaned frustules (see below) were taken using an Olympus AX70 and a Nikon DXM1200F digital camera (with LUCIA software). Measurements were made using ImageJ software (http://rsb/info/nih/gov/ij) and a micrometre slide as a scale. Densities of striae were measured on different cultures grown in the laboratory, five clones of H. karadagensis (NCC 237 to 241), and two clones of H. ostrearia (NCC 148.78 and NCC 158.4). A two-tailed Student's t-test was performed to compare transverse and longitudinal stria densities.
Scanning electron microscopy
Cleaned siliceous frustules were prepared for scanning electron microscopy (SEM) by digesting cells in a mixture of sulphuric and oxalic acids in potassium permanganate (Hendey, Citation1974). Cells were then dried onto aluminium stubs and coated with a thin film of platinum using a JUC5000 sputter coater. SEM observations were performed with a JEOL 7600F operating at 7 kV.
Mating experiments
Crossing experiments between strains were performed following Davidovich et al. (Citation2009) and Mouget et al. (Citation2009), a protocol designed to trigger maximal auxosporulation. According to this protocol, mating mixtures were prepared in sterile Petri dishes, by diluting parental cultures in exponential phase of growth at a final concentration of 2000 cells ml−1 and then placed in a culture box under low irradiance (28 ± 2 m−2 s−1 illumination) and a short photoperiod (8:16 h L:D cycle). Signs of auxosporulation were monitored after one week. To check for sexual compatibility, all the wild H. karadagensis clones (NCC 237 to NCC 244) were mated with H. ostrearia clones of the two opposite sexual types (see Davidovich et al., Citation2009), NCC 158.1 and NCC 234. Three different and separate experiments were performed with each combination of clones.
Molecular characterization
Total DNA of the clones NCC 148.75 (H. ostrearia) and NCC 313 (H. karadagensis) were extracted following Doyle & Doyle (Citation1990). Two markers were used: ITS1–5.8S–ITS2 (nuclear ribosomal DNA), amplified using universal primers ITS-1 and ITS-4 (White et al., Citation1990): ITS-1: 5′–TCCGTAGGTGAACCTGCGG–3′ (forward) and ITS 4: 5′–TCCTCCGCTTATTGATATGC–3′ (reverse), and rbcL (plastid DNA), amplified using DPrbcL1 and DPrbcL7 (Daugbjerg & Andersen, Citation1997) modified according to Jones et al. (Citation2005). These sequences are 5′–AAGGAGAAATHAATGTCT–3′ (forward) for DPrbcL1 and 5′-AARCAACCTTGTGTAAGTCTC–3′ (reverse) for DPrbcL7. The PCR amplifications were performed with 0.2 µM primers, and then 2.5 U GoTaq® Flexi DNA Polymerase (Promega). For a final volume of 25 µL, mixtures contained 1 × green GoTaq® flexi buffer, 2.5 mM MgCl2 25 mM, and 200 µM PCR nucleotide mix (Promega, France). Forty cycles of 60 s denaturation at 94°C, 60 s annealing at 52°C, and 90 s extension at 72°C were performed. PCR products were separated by agarose gel electrophoresis. UV visualization was made using a CN-1000 Darkroom from Vilber Lourmat (Marne-la-Vallée, France). Bands of interests were eluted with a Wizard® SV Gel and PCR Clean-up System (Promega). DNA was integrated into pGEM® T-Easy Vector (Promega). Competent Escherichia coli cells NEB-5-alpha from New-England Biolabs (Evry, France) were transformed with the recombined vector. Positive colonies were identified by white/blue selection. The sequencing reactions were carried out by Beckmann Coulter Genomics (UK, http://www.cogenicsonline.com) using SP6 and T7-promotor primers. For each marker, three transformed bacterial colonies were sent for sequencing. Homology of the ITS sequences with those of known diatoms was searched with BLAST (http://blast.ncbi.nlm.nih.gov/Blast.cgi), alignment was made using ClustalW2 (http://www.ebi.ac.uk/Tools/msa/clustalw2/) and visualized using Genedoc software (http://www.nrbsc.org/gfx/genedoc/). Translations were done using ExPASy Translate Tool (http://expasy.org/tools/dna.html).
UV-visible spectrophotometry
Coloured supernatants of H. ostrearia and H. karadagensis cultures were simultaneously collected in late exponential growth phase, at the beginning of the photoperiod. Supernatants were first clarified by filtration through 0.45 µm filters, then through 0.22 µm filters before measurement. The pH values were usually constant (8.0 ± 0.2) and no buffer was required for adjustment. A quartz cell (Hellma) was used for spectrum measurement. Absorbance spectra ranging from 200 nm to 800 nm were recorded using a Thermo electron Helios gamma UV-visible spectrophotometer (Cambridge, UK).
Micro-Raman spectroscopy
For in vivo confocal micro-Raman measurements, live algae were placed in Petri dishes. The coloured apices of cells in exponential phase of growth, coloured protoplasts in old cultures, gametes containing pigment obtained from crossing experiments, and parietal chloroplasts were studied using a wavelength of 514.5 nm from a coherent Argon–Krypton ion laser from Coherent Innova (Utrecht, the Netherlands). The Raman spectra were recorded in the back-scattering configuration on a T64000 from Horiba Jobin Yvon (Longjumeau, France) spectrometer coupled to a liquid-N2 -cooled CCD detector. All measurements were performed at room temperature using an Olympus microscope equipped with a 100× objective to focus the incident laser beam onto the sample and to collect the scattered light. The laser intensity was reduced to less than 0.5 mW at the sample to minimize possible sample deterioration. In order to obtain distinguishable peaks and Raman spectra with good signal-to-noise ratios, a series of 30 measurements was systematically averaged, with an integration time varying between 10 and 60 s (depending on the samples). The Raman spectra were recorded within the 250–2200 cm−1 wavenumber region and were shifted in the figures (without any baseline correction) for clarity.
Results
Haslea karadagensis Davidovich, Gastineau & Mouget sp. nov.
(, )
Figs 1–4. Haslea karadagensis and H. ostrearia, LM. 1. Live cell of H. karadagensis. 2. Live cell of H. ostrearia. 3. Cleaned frustule of H. karadagensis. 4. Cleaned frustule of H. ostrearia. Scale bars = 10 µm.
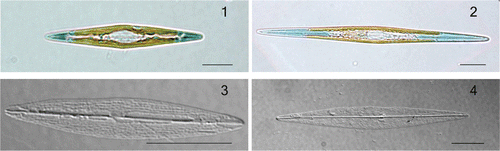
Figs 5–9. Haslea karadagensis, SEM. 5. External view of an entire valve. 6. External view of valve centre, showing the longitudinal slits and the straight proximal raphe fissures. 7. Internal view of valve centre, showing the rectangular areolae and the straight proximal raphe ends. 8. External view of apex showing the ± straight distal ending, longitudinal slits, and the continuous peripheral slit. 9. Internal view of apex showing the straight helictoglossa and the rectangular areolae. Scale bars = 10 µm () or 1 µm ().
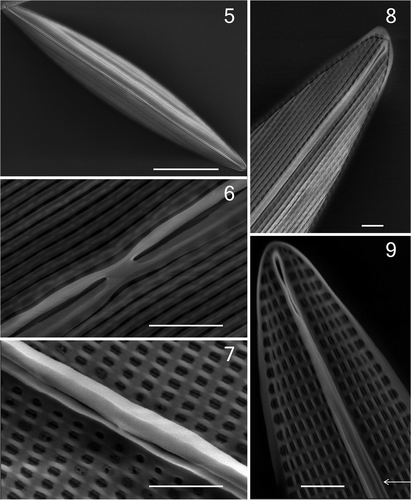
DESCRIPTION: Frustules narrowly rectangular in girdle view. Valves delicate, lanceolate with acute apices (), 28.9–84.0 µm long and 4.5–11.2 µm wide in natural populations. Two band-like plastids, each with several small pyrenoids, lie appressed to the girdle (, and ). At each apex, the cell contents appear dark blue in colour throughout the cell cycle. Raphe system central, only faintly visible in LM. External raphe fissures straight, with only very slightly unilaterally deflected, slightly expanded apical endings, and almost straight central endings. External valve surface covered by longitudinal siliceous strips parallel to the raphe (), merging with a peripheral slit as they abut the valve margin (seen particularly well near the valve apices: ). Raphe sternum constricted externally between the central raphe endings (). Internally, raphe fissures opening laterally on a slightly thickened rib (), except at the apices where they end in elongated helictoglossae (). A narrow accessory rib is present, more or less fused with the raphe sternum at the centre of the valve but closely adjacent to it for most of its length, ending a few micrometres from the apices (), where the raphe fissure opening is more lateral and hidden. Transapical striae difficult to detect in LM (), parallel, perpendicular to the raphe throughout the valve, 31.4 ± 0.2 in 10 µm (mean ± SE, n = 30), comprising uniseriate rows of rectangular areolae. The longer axes of the areolae are oriented longitudinally, parallel to the raphe () and there are 57.8 ± 0.3 areolae in 10 µm longitudinally (mean ± SE, n = 30). Hymenes were not found, but it is possible that they were removed by the cleaning process. The girdle bands are simple split rings, about 1 µm wide throughout, without pores (not illustrated). See also Figs S2 to S8 in the .
HOLOTYPE: BM 101627, syntype BM 101628, both at the National History Museum, London. The clone used for the holotype, NCC 244, has been used for the SEM plate ().
TYPE LOCALITY: Type material was collected from the sublittoral zone near the Biological Station (44° 54′ 40″ N, 35° 12′ 05″ E) of Karadag Natural Reserve, Crimea (Ukraine). Biofilms were collected from stones at a depth of 0.2–0.4 m by N.A. Davidovich, O.I. Davidovich, R. Gastineau and J.-L. Mouget in April 2008. Note that cells with characteristic blue tips were recorded in samples taken near the Karadag Biological Station every spring during the period of observation, starting from 2008. The species was also found in Feodosiya Bay (45° 01′ 25″ N, 35° 24′ 23″ E).
ETYMOLOGY: Named after Karadag, meaning ‘black mountain’ in the Tatar language, referring to the Jurassic mountain above the shores of this Crimean locality.
Comparison with H. ostrearia and biometry
show live cells of the ‘blue’ diatoms, H. karadagensis and H. ostrearia, respectively. Apart from the intensity and location of the blue colouration, which is darker and more strongly confined to the apices in the H. karadagensis, the two diatoms are very similar, although H. ostrearia is narrower and, as a result, has more acute apices. Both have lanceolate valves with two band-like plastids, and in both the cells are solitary and free living. The transapical striation of the valve is above the theoretical resolution limit of the light microscope in both species but very difficult to observe ().
Valve morphometrics differ between H. karadagensis and H. ostrearia (see , and the Materials and methods section for the origins of the different clones). The transverse striae have densities of 31.4 ± 0.2 in 10 µm (mean ± SE, n = 30) in H. karadagensis, but 34.8 ± 0.2 (n = 30) in 10 µm in H. ostrearia, while those of the longitudinal striae are 57.8 ± 0.3 (n = 30) and 53.3 ± 0.2 (n = 30) in 10 µm, respectively. In both cases, the average values of the stria densities in the two species are significantly different (P ≤ 0.001).
Table 1. Morphological data on Haslea karadagensis and H. ostrearia (this study), compared with those on H. ostrearia (or former Navicula ostrearia) and H. pseudostrearia obtained from the literature. When available from the literature, observed extremes are in bold. n.d. = no data.
Biometry of natural populations of H. karadagensis (, different samples collected in the same site on different days in 2008, see Materials and methods) gave an average length of 52.50 ± 0.14 µm and an average width of 8.00 ± 0.03 µm (values are means ± S.E., n = 1148). The longest cell was 84.0 µm long and 8.0 µm wide; the smallest 28.9 µm long by 5.8 µm wide. The widest was 11.2 µm for a length of 58.4 µm, the narrowest 4.5 µm for a length of 33.6 µm. In culture, of 448 cells measured, the largest cell resulting from sexual reproduction was 96.9 µm long, the smallest at the other end of the life cycle, was 18.0 µm long (). Mean values of cell length and width were measured on the different clones maintained in culture for both species, at different times in their life cycle (). Comparing the slopes of the regressions between width and length for both species suggests that width is significantly higher in H. karadagensis than in H. ostrearia for valves ≤50 µm (P < 0.01).
Figs 10, 11. Distribution of lengths and widths in Haslea karadagensis and H. ostrearia. 10. Values measured in natural populations of H. karadagensis collected between April and May 2008 (n = 1148 cells). 11. Relationship between mean values of width and length measured on the different clones of both species maintained in cultures. The equations of the fitting lines are: y = 0.01 x + 8.21 for H. karadagensis (n = 22, R2 = 0.08), y = 0.03 x + 4.28 for H. ostrearia (n = 21, R2 = 0.63). Also included are mean values from available literature for Haslea/Navicula ostrearia from (a) Molisch (Citation1903), (b) Proshkina-Lavrenko (Citation1963), (c) Robert (Citation1973), (d) Massé et al. (Citation2001).
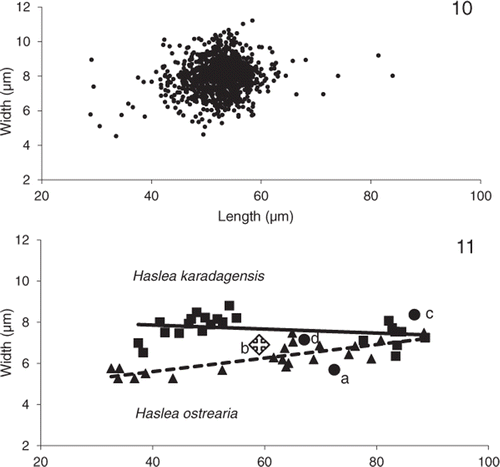
Ultrastructure comparison
show SEM pictures of H. karadagensis, and pictures of both species are available in Supplementary Figures S2–S8 (H. karadagensis) and S9–S13 (H. ostrearia). Figures S2 to S8 correspond to H. karadagensis clone 10607A, with the exception of Fig. S4, which shows deformities of clone 10607C. Supplementary Figs S9–S13 correspond to H. ostrearia clone NCC 148.14c. Ultrastructurally there are no noticeable differences between H. karadagensis and H. ostrearia.
Reproductive behaviour
The reproductive compatibility of the two diatoms producing bluish pigments was also studied. Haslea ostrearia was shown to be heterothallic by Davidovich et al. (Citation2009) and H. karadagensis by Davidovich et al. (Citation2012), who used the same nine clones as those used here. Within each species, crossing experiments between compatible clones under optimal conditions (see Mouget et al., Citation2009) always resulted in auxospore formation, including the positive control cross between the sexually compatible H. ostrearia clones NCC 158.1 and NCC 234. However, in mixtures of the two species, H. karadagensis never bred with H. ostrearia clones of either mating type, and no sexual stages (gametes, zygotes, auxospores, initial cells) were ever observed in mixtures of the two organisms. This suggests prezygotic isolation between the two populations, which can therefore be considered discrete species. Modalities of sexual reproduction in H. karadagensis have also been investigated, and more information about auxospore formation, initial cell size, and cardinal points of the life cycle are described in Davidovich et al. (Citation2012). From all measurements made in this species (both natural samples and cultures in the laboratory) in the course of the life cycle, the ranges of lengths of vegetative cells, gametangia and initial cells (maximal species-specific size) are respectively 18–97 µm (n = 1701), 22–54 µm (n = 148) and 64–97 µm (n = 189).
Molecular genetic differentiation
The DPrbcL1–DPrbcL7 combination allowed a 1545 bp fragment, containing the 1473 bp long rbcL fragment coding for a functional protein of 491 amino acids, to be amplified, and referenced in Genbank as HE663062.1 (H. ostrearia) and HE663063.1 (H. karadagensis). The difference between the two species for the rbcL gene was 2%, calculated using Genedoc. The universal ITS1–ITS4 combination allowed the complete ITS1–5.8 S–ITS2 region to be obtained. However, depending on the species, the length of the fragment was highly variable. For H. ostrearia, fragment length was about 1104 bp (HE663057.1), compared with 1370 bp for H. karadagensis (HE663058.1). The difference for the 5.8S gene (155 bp aligned, identified by comparison with Navicula salinicola sequence GQ330356.1) was 4%. The ITS1 and ITS2 sequence analysis was performed separately using Genedoc, but excluding high polymorphic regions (large indels). It revealed that the new species H. karadagensis (localization: black Sea, HE663058) and H. ostrearia (French Atlantic Coast, HE663057 and HE663059) share 47% (ITS1, 477 bp) and 46% (ITS2, 346 bp) nucleotide identity. The ITS and 5.8S sequences of the two H. ostrearia clones (HE663057 and HE663059) have 99% and 100% identity, respectively.
UV-visible spectrophotometry
UV-visible spectrophotometry showed that the absorption spectra of pigments released into the medium by H. ostrearia and H. karadagensis were similar, exhibiting a main peak in the UV region, and a secondary peak in the red part of the spectrum. However, there were noticeable differences: the marennine UV peak is at 247 nm, but the pigment from H. karadagensis peaked at 260 nm (). Moreover, the visible absorbance peak of marennine occurs at 670 nm, whereas that for the marennine-like pigment of H. karadagensis was at 605 nm (inset ). Pigment concentrations were not calculated because no reliable method is available.
Micro-Raman spectroscopy
To provide molecular vibrational information on pigments, we investigated the living algae in an aqueous environment by confocal Raman spectroscopy. Raman spectra for the parietal chloroplasts, devoid of blue pigment, were identical for both species. Two strong peaks, at 1162 cm−1 and 1527 cm−1 (), are directly attributed to C–C and C = C stretching vibrations, as already observed in diatoms and other organisms (Saito & Tasumi, Citation1983; Wood et al., Citation2005; Hedegaard et al., Citation2006; Heraud et al., Citation2007). Raman spectra of pigments in the apical regions of vegetative cells and gametes of H. ostrearia and H. karadagensis showed two strong peaks, at 1158–1159 cm−1 and 1520–1522 cm−1 (; a summary of the main vibrational wave-numbers is given in ). The positions of these peaks are similar to those observed for the chloroplasts in the two species, suggesting partial light scattering from the chloroplasts during measurement. Raman spectra of H. ostrearia apices and gametes also showed major bands at 1650 and 1462 cm−1, with a broad band at about 1300 cm−1 and other weaker bands (). In contrast, Raman spectra of H. karadagensis apices and gametes () showed an intense band at about 1347 cm−1, two well-resolved bands of medium intensity at 1160 and 1122–1125 cm−1, and several less intense bands.
Fig. 13. Raman spectra at 514.5 nm, recorded using vegetative cells in exponential growth phase or gametes from H. karadagensis (HK) and H. ostrearia (HO). The laser was pointed at the coloured areas (apices of vegetative cells, gametes), or at the chloroplasts (vegetative cells).
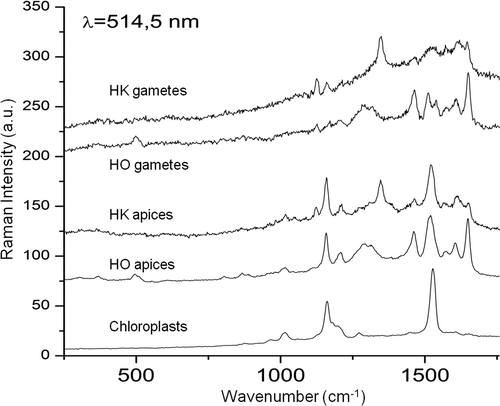
Table 2. Summary of representative vibrational wavenumbers (cm−1) observed by Raman spectroscopy for H. ostrearia apices and gametes, H. karadagensis apices and gametes, and the chloroplasts of both diatoms. The intensity of the signal is indicated as: vs, very strong; s, strong; m, medium; w, weak; vw, very weak. The most intense bands are shown in bold.
Discussion
Morphology, biometry support recognition of a new ‘blue’ diatom
Based on LM and SEM, the new species, H. karadagensis, is morphologically similar to H. ostrearia (Figs , Supplementary Figs S1–S13, ), but it differs from H. ostrearia in valve dimensions and stria densities, in particular referring to published data (Peragallo & Peragallo, Citation1897–1908; Hustedt & Aleem, Citation1951; Massé et al., Citation2001). Both diatoms produce a peculiar bluish pigment, which accumulates at the cell apices and does not occur outside the genus Haslea, supporting a close taxonomic relationship within the same genus. Another diatom, H. pseudostrearia is also very similar to H. ostrearia in its frustule ultrastructure and possesses a similar transverse stria density (34–36 in 10 µm; the longitudinal stria density is rather lower, at 42 in 10 µm: see ), but lacks any blue pigment (Massé et al., Citation2001). Like H. ostrearia and H. pseudostrearia, H. karadagensis lacks a pseudo-stauros and thickened central virgae, which are found in some other diatoms in the genus, such as H. crucigera (Round et al., Citation1990) and H. salstonica (Massé et al., Citation2001). On the other hand, all these species possess two chloroplasts, one along each side of the cell with numerous pyrenoids, features observed in other species of Haslea (Poulin et al., Citation2004; Cox & Williams, Citation2006).
Morphological data ( and Fig. S1) show a higher maximal length and a lower width for valves ≤50 m for H. ostrearia than for H. karadagensis, suggesting that the taxa warrant separation. This is reinforced by data available from the literature (), that compare morphology and cell dimension in blue diatoms identified as Navicula/Haslea ostrearia, including those previously observed in the Black Sea (Proshkina-Lavrenko, Citation1963). Hence the comparison of biometric data strengthens the recognition of two species of diatoms in the same genus that are able to produce blue pigments.
Reproductive and molecular biology support species delimitation
The lack of reproductive compatibility between the two taxa is also consistent with their recognition at the species level, on both the biological species concept and the metapopulation lineage concept (De Queiroz, Citation2007). No signs of sexual reproduction were reported in any of the interbreeding experiments. The 2% difference in the rbcL sequences is similar to the percentage difference recorded between closely related species of the Sellaphora pupula complex (Evans et al., Citation2007). The range of divergence in the conserved 5.8 S gene (4%) is comparable to the 5% observed between two Navicula species (Genbank accessions GQ330358.1 and GQ330356.1: Moniz & Kaczmarska, Citation2010). The main information obtained from sequencing the complete ITS1–5.8 S–ITS2 fragment is a striking size difference in ITS, also observed for reproductively isolated species of the Sellaphora complex (Behnke et al., Citation2004). For all sequenced plasmids, no intra-individual length or composition polymorphisms were observed in the two markers, in contrast to ITS1–5.8 S–ITS2 data in some other diatoms (reviewed in Hamsher et al., Citation2011).
Raman spectroscopy to discriminate diatom blue pigments and species
In order to discriminate the two species of ‘blue’ diatoms, their pigment signatures were first recorded in vitro by spectrophotometry (extracellular form of the pigments), and in vivo by micro-Raman spectroscopy (intracellular form of the pigments). It has been shown from previous studies (Pouvreau et al., Citation2006a ) that the supernatant obtained from cultures of H. ostrearia is composed of an extracellular form of marennine with a molecular weight of 9893 ± 1 Da, which is actively secreted into the medium, plus some of the intracellular form, with a molecular weight of 10751 ± 1 Da, released after cell death. Because Pouvreau et al. (Citation2006a ) demonstrated that pH has a bathochromic effect on the absorption spectrum of marennine, we excluded possible artefacts by controlling the pH of the supernatants from the two species before measurement. At pH 8.0, both absorption peaks, in the UV and visible regions, differed between filtered supernatants from the two diatom cultures. The position of the absorbance peak of H. karadagensis pigment in the visible region differs from that of marennine at the same pH (). Moreover, preliminary experiments using nuclear magnetic resonance (NMR) and matrix-assisted laser desorption ionization time-of-flight (MALDI-TOF) mass spectrometry (our unpublished observations) allow us to hypothesize that the H. karadagensis and H. ostrearia pigments are different molecules belonging to the same chemical family, but whose structure remains unknown.
To extend our study of H. ostrearia and H. karadagensis, we performed the first micro-Raman spectroscopic investigations on their blue pigments. The spectral data reveal a high degree of similarity in the 1000–1700 cm−1 wave-number range, since most of the vibrational bands are present in both spectra. Although the chemical structure of the pigments has not yet been determined, preliminary molecular information can be deduced from Raman spectra. The 1650 cm−1 band of H. ostrearia and H. karadagensis can be assigned to the C=C stretching mode of a phenyl ring, and the 1462 cm−1 band to a C=O or C=N stretching mode (Varsanyi, Citation1974; Johnson et al., Citation1996). Full assignment of the observed bands is difficult but our data reveal that the composition and/or the conformational structure of the pigments in H. ostrearia and H. karadagensis are different. Indeed, the two pigments are easily distinguished, since the most intense Raman spectrum band for the cell apices and gametes of H. karadagensis () is the well-resolved band at 1347–1348 cm−1. Therefore, we have shown that Raman spectroscopy is appropriate for distinguishing between pigments that probably have a complex polycyclic carbide structure containing oxygen and nitrogen (Pouveau et al., Citation2006a ). To help clarify our spectral interpretations, further NMR work is in progress on both pigments.
Concluding remarks
In conclusion, this work demonstrates that the new diatom, H. karadagensis, isolated from the Black Sea, may be considered the second blue pigment-producing diatom after H. ostrearia, which has long been considered the only ‘blue’ diatom, producing marennine. This raises some other questions. Firstly, was the diatom with bluish apices found by Proshkina-Lavrenko (Citation1963) in the Black Sea H. ostrearia or H. karadagensis? The transverse and longitudinal stria densities given were 33–36 and 36 in 10 µm, respectively, and the lengths ranged from 50 µm to 81 µm, with widths of 4.5–7 µm. Based on these data, the diatom seen by Proshkina-Lavrenko could be H. ostrearia, although the longitudinal stria density is below that recorded for this species elsewhere. If so, at least two species of ‘blue’ diatoms, H. ostrearia and H. karadagensis, may occur in the Black Sea. Secondly, the diatom described by Hendey (Citation1964) as H. ostrearia had a different transapical stria density to that commonly attributed to H. ostrearia, closer to H. karadagensis. Lastly, every record of a diatom with blue tips and recorded as H. ostrearia but without in-depth morphological investigation should be reconsidered and, if possible, checked using modern approaches to species determination. With this in mind, various other strains of ‘blue’ diatoms have been obtained from different parts of the world and are currently under investigation in our laboratory.
supplementary material S1
Download Zip (8.3 MB)supplementary material
Download Zip (8.3 MB)supplementary material
Download Zip (8.3 MB)Acknowledgements
We gratefully acknowledge receiving financial help from EGIDE (France) to provide funds for travel and other expenses, and we thank Université du Maine for providing facilities for two of us (N.A.D., O.I.D.). We also thank an anonymous reviewer and the editor for critical comments on the manuscript, and J. M. Ehrman, for SEM imaging of the .
References
- Alverson , AJ . 2008 . Molecular systematics and the diatom species . Protist , 159 : 339 – 353 .
- Barone , R . 1979 . La diatomee bentoniche del porto di Palermo . Giornale Botanica Italiano , 3 : 187 – 295 .
- Behnke , A , Friedl , T , Chepurnov , VA and Mann , DG . 2004 . Reproductive compatibility and rDNA sequence analyses in the Sellaphora pupula species complex (Bacillariophyta) . Journal of Phycology , 40 : 193 – 208 .
- Cox , EJ and Williams , DM . 2006 . Systematics of naviculoid diatoms (Bacillariophyta): a preliminary analysis of protoplasts and frustule characters for family and order level classification . Systematics and Biodiversity , 4 : 385 – 399 .
- Daugbjerg , N and Andersen , RA . 1997 . A molecular phylogeny of the heterokont algae based on analyses of chloroplast encoded rbcL sequence data . Journal of Phycology , 33 : 1031 – 1041 .
- Davidovich , NA , Mouget , J-L and Gaudin , P . 2009 . Heterothallism in the pennate diatom Haslea ostrearia (Bacillariophyta) . European Journal of Phycology , 44 : 251 – 261 .
- Davidovich , NA , Gastineau , R , Gaudin , P , Davidovich , O and Mouget , J-L . 2012 . Sexual reproduction in the newly-described blue diatom Haslea karadagensis . Fottea , 12 : 219 – 229 .
- De Queiroz , K . 2007 . Species concepts and species delimitation . Systematic Biology , 56 : 879 – 886 .
- Doyle , JJ and Doyle , JL . 1990 . Isolation of plant DNA from fresh tissue . Focus , 12 : 13 – 15 .
- Dyer , WTT . 1877 . Greening of oysters . Nature , 16 : 397
- Evans , KM , Wortley , AH and Mann , DG . 2007 . An assessment of potential diatom “barcode” genes (cox1, rbcL, 18 S and ITS rDNA) and their effectiveness in determining relationships in Sellaphora (Bacillariophyta) . Protist , 158 : 349 – 364 .
- Fan , L and Yang , M . 2008 . In-situ resonance Raman spectra of organic pigments in Momo coral . Journal of China University of Geosciences , 19 : 146 – 151 .
- Gaillon , B . 1820 . Des huîtres vertes, et des causes de cette coloration . Journal de physique, de chimie, d’histoire naturelle et des arts , 91 : 222 – 225 .
- Grunow , A . 1877 . New diatoms from Honduras . Monthly Microscopical Journal , 18 : 165 – 186 .
- Hardy , JT . 1973 . Ecology of phytoneuston in a temperate marine lagoon . Limnology and Oceanography , 18 : 525 – 533 .
- Hamsher , SE , Evans , KM , Mann , DG , Poulíčková , A and Saunders , GW . 2011 . Barcoding diatoms: exploring alternatives to COI-5 P . Protist , 162 : 405 – 422 .
- Hedegaard , C , Bardeau , J-F and Chateigner , D . 2006 . Molluscan shell pigments: an in situ resonance Raman study . Journal of Molluscan Studies , 72 : 157 – 162 .
- Hendey , NI . 1964 . An Introductory Account of the Smaller Algae of British Coastal Waters Part V: Bacillariophyceae (Diatoms) HMSO: London
- Hendey , NI . 1974 . The permanganate method for cleaning freshly gathered diatoms . Microscopy , 32 : 423 – 426 .
- Heraud , P , Beardall , J , McNaughton , D and Wood , BR . 2007 . In vivo prediction of the nutrient status of individual microalgal cells using Raman microspectroscopy . FEMS Microbiology Letters , 275 : 24 – 30 .
- Hustedt , F and Aleem , AA . 1951 . Littoral diatoms from the Salstone near Plymouth . Journal of the Marine Biological Association of the United Kingdom , 30 : 177 – 196 .
- Johnson , SA , Bisby , H , Tavender , SM and Parker , AW . 1996 . The free radical site in pea seedling copper amine oxidase probed by resonance Raman spectroscopy and generated by photolysis of caged substrate . FEBS Letters , 380 : 183 – 187 .
- Jolivet , A , Bardeau , J-F , Fablet , R , Paulet , YM and De Pontual , H . 2008 . Understanding otolith biomineralization processes: new insights into microscale spatial distribution of organic and mineral fractions from Raman microspectrometry . Analytical and Bioanalytical Chemistry , 392 : 551 – 560 .
- Jones , HM , Simpson , GE , Stickle , AJ and Mann , DG . 2005 . Life history and systematics of Petroneis (Bacillariophyta) with special reference to British waters . European Journal of Phycology , 40 : 61 – 87 .
- Kennett , DM and Hargraves , PE . 1991 . “ Benthic marine diatoms ” . In Freshwater and Marine Plants of Rhode Island , Edited by: Sheath , RG and Harlin , MM . 127 – 135 . Dubuque : Kendall and Hunt Publishing Company .
- Lankester , ER . 1886 . On green oysters . Quarterly Journal of Microscopical Science , 26 : 71 – 94 .
- Madkour , FA , Dorgham , MM , Hanafi , MH and Holligan , PM . 2007 . Comprehensive hydro-biological observations on the Suez Canal . International Journal of Oceans and Oceanography , 2 : 125 – 137 .
- Massé , M , Rincé , Y , Cox , EJ , Allard , G , Belt , ST and Rowland , SJ . 2001 . Haslea salstonica sp. nov. and Haslea pseudostrearia sp. nov. (Bacillariophyta), two new epibenthic diatoms from the Kingsbridge estuary, United Kingdom . Comptes Rendus de l’Académie des Sciences de Paris, Sciences de la vie/Life Sciences , 324 : 617 – 626 .
- Medlin , LK and Kooistra , WHCF . 2010 . Methods to estimate the diversity in the marine photosynthetic protist community with illustrations from case studies: a review . Diversity , 2 : 973 – 1014 .
- M’harzi , A , Tackx , M , Daro , MH , Kesaulia , I , Caturao , R and Podoor , N . 1998 . Winter distribution of phytoplankton and zooplankton around some sandbanks of the Belgian coastal zone . Journal of Plankton Research , 20 : 2031 – 2052 .
- Mitchell , PH and Barney , RL . 1918 . The occurrence in Virginia of green-gilled oysters similar to those of Marennes . Bulletin of the United States Bureau of Fisheries , 35 : 135 – 149 .
- Molisch , H . 1903 . Notiz über eine blaue Diatomee . Berichte der Deutschen Botanischen Gesellschaft , 21 : 23 – 26 .
- Moniz , MBJ and Kaczmarska , I . 2010 . Barcoding of diatoms: nuclear encoded ITS revisited . Protist , 161 : 7 – 34 .
- Mouget , J-L , Gastineau , R , Davidovich , O , Gaudin , P and Davidovich , NA . 2009 . Light is a key factor in triggering sexual reproduction in the pennate diatom Haslea ostrearia . FEMS Microbiology Ecology , 69 : 194 – 201 .
- Neuville , D and Daste , P . 1978 . Recherches sur le déterminisme de la production de marennine par la Diatomée marine Navicula ostrearia (Gaillon) Bory en culture in vitro . Revue Générale de Botanique , 85 : 255 – 303 .
- Nowak , D , Florek , M , Nowak , J , Kwiatek , W , Lekki , J , Chevallier , P , Hacura , A , Wrzalik , R , Ben-Nissan , B , Van Grieken , R and Kuczumow , A . 2009 . Morphology and the chemical make-up of the inorganic components of black corals . Material Science and Engineering: C , 29 : 1029 – 1038 .
- PERAGALLO, H. & PERAGALLO, M. (1897–1908). Diatomées marines de France et des districts maritimes voisins. J. Tempère, Grez-sur-Loing
- Perkins , RG , Mouget , J-L , Lefebvre , S and Lavaud , J . 2006 . Light response curve methodology and possible implications in the application of chlorophyll fluorescence to benthic diatoms . Marine Biology , 149 : 703 – 712 .
- Petersen , CGJ . 1916 . “Grüne Austern” in Dänemark in 1911–1912 . International Review of Hydrobiology and Hydrography , 7 : 39 – 41 .
- Poulin , M , Massé , G , Belt , ST , Delavault , P , Rousseau , F , Robert , JM and Rowland , SJ . 2004 . Morphological, biochemical and molecular evidence for the transfer of Gyrosigma nipkowii Meister to the genus Haslea (Bacillariophyta) . European Journal of Phycology , 39 : 181 – 195 .
- Pouvreau , JB , Morançais , M , Fleury , F , Rosa , P , Thion , L , Cahingt , B , Zal , F , Fleurence , J and Pondaven , P . 2006a . Preliminary characterisation of the blue-green pigment “marennine” from the tychopelagic diatom Haslea ostrearia (Gaillon/Bory) Simonsen . Journal of Applied Phycology , 18 : 757 – 767 .
- Pouvreau , JB , Morançais , M , Massé , G , Rosa , P , Robert , JM , Fleurence , J and Pondaven , P . 2006b . Purification of the blue-green pigment «marennine» from the marine tychopelagic diatom Haslea ostrearia (Gaillon/Bory) Simonsen . Journal of Applied Phycology , 18 : 769 – 781 .
- Proshkina-Lavrenko , AI . 1963 . Diatomovye vodorosli bentosa Chernogo morya Izdatel'stvo Akademii nauk SSSR, Leningrad
- Ranson , G . 1937 . Le verdissement des huîtres . Sciences , 8 : 13 – 24 .
- Ricard , M . 1987 . “ Diatomophycées ” . In Atlas du phytoplancton marin , Edited by: Sournia , A . Vol. 2 , 1 – 297 . Paris : Editions du Centre National de la Recherche Scientifique .
- Robert , JM . 1973 . La diatomée Navicula ostrearia Bory en Baie de Bourgneuf . Revue des Travaux de l'Institut des Pêches Maritimes (ISTPM) , 37 : 363 – 368 .
- Round , FE , Crawford , RM and Mann , DG . 1990 . The diatoms. Biology and morphology of the genera Cambridge University Press, Cambridge
- Saito , S and Tasumi , M . 1983 . Normal-coordinate analysis of ß-carotene isomers and assignments of the Raman and infrared bands . Journal of Raman Spectroscopy , 14 : 310 – 321 .
- Sauvageau , C . 1906 . A propos de la présence de la diatomée bleue dans la Méditerranée . Travaux des laboratoires (Arcachon) , 6 : 46 – 59 .
- Simonsen , R . 1974 . The diatom plankton of the Indian Ocean expedition of RV “Meteor” . “Meteor” Forschungsergebnisse , Reihe D, 19 : 46 – 49 .
- SNOEIJS, P. & KASPEROVIČIENĖ, J. (editors) (1996). Intercalibration and Distribution of Diatom Species in the Baltic Sea. Volume 4. Opulus Press, Uppsala
- Solazzi , A and Tolomio , C . 1976 . Il fitoplancton primaverile ed estivo lungo le coste settentrionali della Sardegna . Archivio de Oceanografia e Limnologia , 18 : 389 – 409 .
- Spiro , TG . 1974 . Resonance Raman spectroscopy. New structure probe for biological chromophores . Accounts of Chemical Research , 7 : 339 – 344 .
- Sprat , T . 1667 . The history of the generation and ordering of green oysters, commonly called Colchester-oysters . History of the Royal Society, London , : 307 – 319 .
- Sullivan , MJ . 1977 . Structural characteristics of a diatom community epiphytic on Ruppia maritima . Hydrobiologia , 53 : 81 – 86 .
- Tolomio , C . 1978 . Catalogo delle Diatomee e delle Peridinee pin signifactive segnalate nelle acque salmastre italiane . Memorie di Biologia Marina e di Oceanagrafia , 8 : 129 – 150 .
- Trinkler , N , Labonne , M , Marin , A , Jolivet , A , Bohn , M , Poulain , C , Bardeau , J-F and Paillard , C . 2010 . Clam shell repair from the brown ring disease: a study of the organic matrix using Confocal Raman micro-spectrometry and WDS microprobe . Analytical and Bioanalytical Chemistry , 396 : 555 – 567 .
- Varsanyi , G . 1974 . Assignments for vibrational spectra of 700 benzene derivatives John Wiley, New York
- Volkman , JK , Barrett , SM and Dunstan , GA . 1994 . C25 and C30 highly branched isoprenoid alkenes in laboratory cultures of two marine diatoms . Organic Geochemistry , 21 : 407 – 414 .
- White , TJ , Bruns , T , Lee , S and Taylor , J . 1990 . “ Amplification and direct sequencing of fungal ribosomal RNA genes for phylogenetics ” . In PCR Protocols , Edited by: Innis , MA , Gelfand , DH , Sninsky , JJ and White , TJ . 315 – 322 . New York : Academic Press .
- Wood , BR , Heraud , P , Stojkovic , S , Morrison , D , Beardall , J and McNaughton , D . 2005 . A portable Raman acoustic levitation spectroscopic system for the identification and environmental monitoring of algal cells . Analytical Chemistry , 77 : 4955 – 4961 .
- Wu , Q , Nelson , WH , Hargraves , P , Zhang , J , Brown , CW and Seelenbinder , JA . 1998 . Differentiation of algae clones on the basis of resonance Raman spectra excited by visible light . Analytical Chemistry , 70 : 1782 – 1787 .
- Wu , Q , Nelson , WH , Treubig , JM Jr. , Brown , PR , Hargraves , P , Kirs , M , Feld , M , Desari , R , Manoharan , R and Hanlon , EB . 2000 . UV resonance Raman detection and quantitation of domoic acid in phytoplankton . Analytical Chemistry , 72 : 1666 – 1671 .
- Wulff , A , Wängberg , SÅ , Sundbäck , K , Nilsson , C and Underwood , GJC . 2000 . Effects of UVB radiation on a marine microphytobenthic community growing on a sand-substratum under different nutrient conditions . Limnology and Oceanography , 45 : 1144 – 1152 .
Supplementary material
The following supplementary material is available for this article, accessible via the Supplementary Content tab on the article's online page at http://dx.doi.org/10.1080/09670262.2012.741713
Supplementary Fig. S1. Changes in lengths and widths in different clones of Haslea karadagensis and H. ostrearia with the life cycle.
Supplementary Figures S2–S8. Haslea karadagensis, SEM. External and internal views of entire valves (Figs S2, S3), and of central (Figs S5, S6) and apical (Figs S7, S8) raphe endings. Fig. S4: frustule with deformities.
Supplementary Figs S9–S13. Haslea ostrearia, SEM. Internal view of an entire valve (Fig. S9). External and internal views of valve centres (Figs S10, S13), and of apices (Figs S11, S12), respectively.