Abstract
Bacteria associated with Fucus vesiculosus and Delesseria sanguinea, two macroalgae from the Kiel Fjord were investigated seasonally over two years by cultivation-based methods. A total of 166 bacterial strains were isolated from the macroalgae, affiliated to seven classes of bacteria (Actinobacteria, Bacilli, Alphaproteobacteria, Betaproteobacteria, Gammaproteobacteria, Cytophagia and Flavobacteria). According to 16S rRNA gene sequence similarities they were arranged in 82 phylotypes of > 99.0% sequence identity. Assuming that chemical factors rule the bacteria–macroalga and bacteria–bacteria interactions on algal surfaces, we tested the antibiotic activity of the bacterial isolates not only against a panel of four standard test organisms (Bacillus subtilis, Candida glabrata, Escherichia coli and Staphylococcus lentus) but also four macroalga-associated microorganisms: Algicola bacteriolytica and Pseudoalteromonas elyakovii (macroalgal pathogens), and Bacillus algicola and Formosa algae (strains associated with algal surfaces). Organic extracts of more than 51% of the isolates from the two macroalgae inhibited the growth of at least one of the tested microorganisms. As much as 46% and 45% of the isolates derived from F. vesiculosus and D. sanguinea, respectively, showed antimicrobial activity against the set of macroalga-associated bacteria, compared with 13 and 19% against a standard set of microorganisms. High antibacterial activity against macroalgal pathogens and bacterial competitors support the assumption that complex chemical interactions shape the relationships of bacteria associated with macroalgae and suggest that these bacteria are a rich source of antimicrobial metabolites.
Introduction
Surfaces submerged in the ocean are rapidly covered by biofilms (Harder, Citation2009). The surfaces of marine algae provide especially attractive habitats for epibiotic bacteria (Boyd et al., Citation1999), which are fast colonizers, highly adaptable, and capable of rapid degradation of algal exudates. Therefore they play a key role in the colonization and biofouling of macroalgae (Corre & Prieur, Citation1990). Different species of marine macroalgae in the same habitat support different bacterial communities (Lachnit et al., Citation2009; Nylund et al., Citation2010), while the same macroalgal species, even from different localities, demonstrate high similarities in the composition of associated microbial communities (Staufenberger et al., Citation2008; Lachnit et al., Citation2009). The production of biologically active compounds by the algal host influence the relationships between bacteria and macroalgae (Goecke et al., Citation2010; Persson et al., Citation2011). Recently it was shown that crude extracts of different macroalgae both stimulated and inhibited the growth of certain microorganisms (Goecke et al., Citation2012). Furthermore, the brown alga Fucus vesiculosus seems to mediate bacterial surface colonization by fucoxanthin and other compounds excreted from the thallus (Lachnit et al., Citation2010; Saha et al., Citation2011).
In addition, interactions among associated microbiota and the effects of natural products produced by bacteria thriving at the alga surface are important factors in shaping these communities. Different studies have focused on antifouling and antibiotic activities of macroalga-associated bacteria (Boyd et al., Citation1999; Egan et al., Citation2000; Zheng et al., Citation2005; Dobretsov et al., Citation2006; Kanagasabhapathy et al., Citation2006, Citation2008; Penesyan et al., Citation2009; Wiese et al., Citation2009; Villarreal-Gómez et al., Citation2010; Ismail-Ben Ali et al., Citation2012). These cultivation-based studies have indicated that epibiotic bacteria exhibit a great potential to produce antibiotic compounds. Space and nutrient limitation as well as a highly competitive environment force surface-dwelling microorganisms to evolve particular adaptive responses, as well as antagonistic strategies to prevent colonization or growth of potential competitors (Egan et al., Citation2008). Therefore, the production of antimicrobial chemical defences by associated microorganisms may represent an added level of complexity to the mechanisms bywhich marine algae avoid the deleterious effectsof microbial pathogens (Engel et al., Citation2002). Unfortunately, assessments of the antibacterial activity exhibited by marine organisms in the natural products literature have focused on microbial reference strains from terrestrial origin or of medical relevance (Engel et al., Citation2006; Hughes & Fenical, Citation2011; Goecke et al., 2012), and have rarely included relevant bacteria originating from the natural habitat.
In the present study, for the first time, epiphytic bacteria associated with the red macroalga Delesseria sanguinea were compared with those associated with the co-occurring Fucus vesiculosus. Bacteria from both algae were isolated seasonally and classified by phylogenetic analysis based on 16S rRNA gene sequences. Their potential to shape the microbial communities was investigated by testing theeffect of crude extracts on bacteria associated with the macroalgae and on known algal pathogens as compared with a standard set of bacteria often used in antibiotic test panels.
Table 1. The 42 out of 166 isolates from Fucus vesiculosus (F) and Delesseria sanguinea (D) that could be affiliated to 17 type strains previously described as associated with algae by other authors (see References). The similarity (%) to the closest type strain and the GenBank accession number are given, and the number of isolates.
Table 2. Phylotypes (Px) associated exclusively with Fucus vesiculosus or Delesseria sanguinea, with the closest type strain (in parentheses: for the strain number see Supplementary Information 1). The phylotypes affiliate to seven classes of bacteria according to the 16S rRNA gene sequences. S, phylotype active against the standard set; M, phylotype against macroalga-associated set; – not active; nd, not determined.
Fig 1–4. Phylogenetic relationships of bacteria associated with the two algae and their most closely related type strains (with NCBI accession number). Separate phylogenetic trees are given for sequences belonging to the Bacteroidetes (Fig. 1), Proteobacteria (Fig.2), Bacilli (Fig. 3), and Actinobacteria (Fig. 4). All are neighbour-joining trees based on 16S rRNA gene sequences. Representatives of the phylotypes from this study are shown in bold type. Non-parametric bootstrapping analysis (1000 pseudoreplicates) was conducted and values ≥ 50% are shown. The scale bars indicate the number of substitutions per nucleotide position. Phylotypes with antibiotically active bacteria are indicated by *.
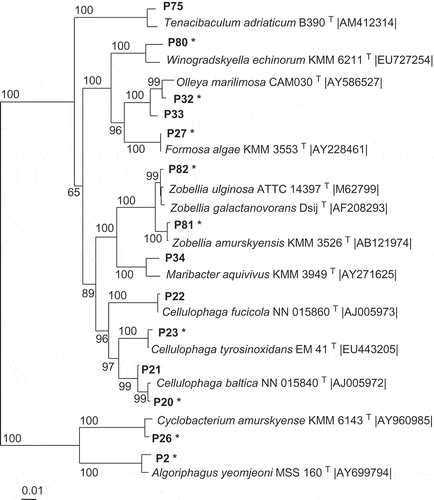
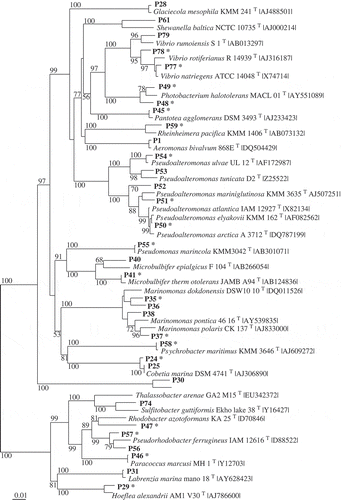
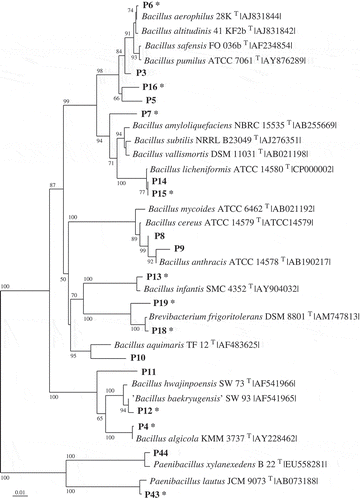
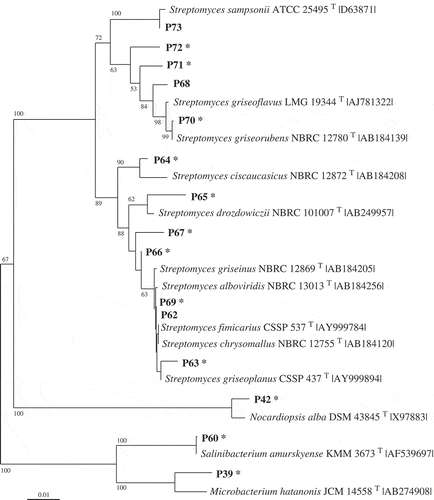
Fig 5. Relative abundance (in percentage) of the different phylotypes belonging to different bacterial classes according to their origin: left, Delesseria sanguinea (D) and right, Fucus vesiculosus (F) in the different seasons (S1: July 2008, W1: January 2009, S2: July 2009, W2: January 2010). The bacterial classes are: Actinobacteria (Ac), Alphaproteobacteria (Al), Bacilli (Ba), Betaproteobacteria (Be), Cytophagia (Cy), Gammaproteobacteria (Ga) and Flavobacteria (Fl).
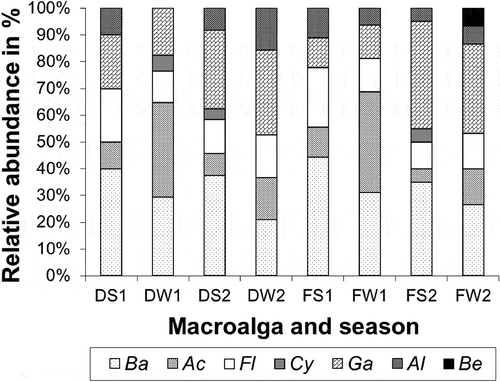
Materials and methods
Sampling of the macroalgae
Samples of the macroalgae were taken from a distinct site of the Strande Beach, in the Kiel Fjord, Western Baltic Sea, Germany (54°25.5′N, 10°12′E). The brown macroalga Fucus vesiculosus (Fucales, Phaeophyceae) and the red macroalga Delesseria sanguinea (Ceramiales, Rhodophyta) were collected at 2–6 m depth in July 2008 (summer I), January 2009 (winter I), July 2009 (summer II) and January 2010 (winter II). The algae were removed carefully from the substratum with a knife and transferred into sterile plastic bags. The samples were stored in the dark at ambient seawater temperature using coolers and processed within 3 hours of collection. In the laboratory, the macroalgae were rinsed three times with sterile Baltic Sea water to remove associated debris and planktonic and loosely attached microorganisms (Penesyan et al., Citation2009). Parts of the macroalgae were fixed in 4% formaldehyde to confirm their taxonomic identification. Algae were identified by examination of their thallus architecture and special morphological characters: fronds, branching, presence of air bladders, midribs, and reproductive structures (Pankow, 1971). Voucher specimens were deposited in the Herbarium of Museo de Historia Natural, Santiago, Chile (code SGO).
Isolation and cultivation of bacteria
The procedure for isolating bacteria associated with the surface of the macroalgae was according to Wiese et al. (Citation2009). Pieces (c. 7 cm) of the macroalgal tissue (one piece per species at each time point) were suspended in sterile Baltic seawater and homogenized using an Ultraturrax T25 (IKA Werke, Staufen Germany). The suspension was plated on five different nutrient media, which had the following composition (all amounts in g l−1): TSB medium (12 g Difco tryptic soy broth, 10 g NaCl, 15 g Bacto agar, pH 7.2 in distilled water); SCA medium (10 g Bacto Trypton, 5 g yeast extract, 20 g NaCl, 1 g MgSO4. xH2O, 6 g Tris HCl, 5 ml glycerol, 15 g Bacto agar, pH 7.5 in distilled water), TM medium (1 g peptone from soymeal, 5 g yeast extract, 15 g Bacto agar, 30 g Tropic Marin Salt in distilled water), GPY medium (0.5 g peptone from soymeal, 0.1 g yeast extract, 15 g Bacto agar, 1 g glucose, pH 7.2 in sterile Baltic Sea water), and HWO medium according to Muscholl-Silberhorn et al. (Citation2008) (0.1 g yeast extract, 15 g Bacto agar, in sterile Baltic Sea water). The plates were incubated at 22°C for 14 days. Colonies were sub-cultivated on individual agar plates by standard procedures. Preservation of the strains was performed according to Wiese et al. (Citation2009).
Table 3. Total number and percentage of phylotypes with antibiotic activity, classified according to their origin, viz. Fucus vesiculosus (a), Delesseria sanguinea (b), and strains shared by both macroalgae (c). In each case information is given about the number of strains that are active against standard test strains (‘Standard set’: Bacillus subtilis, Staphylococcus lentus, Escherichia coli and Candida glabrata), and against the macroalga-associated strains (‘Macroalga-associated set’), the latter being reported as totals and also as ‘surface-associated strains’ (Bacillus algicola, Formosa algae and Algicola bacteriolytica) and ‘macroalgal pathogen strains’ (only Pseudoalteromonas elyakovii). The total active phylotypes represent the number of different phylotypes by algae that inhibited at least one test strain.
b. Delesseria sanguinea
c. Shared by both macroalgae
16S rRNA gene sequence analysis
Genomic DNA of the strains was obtained either by freezing and boiling (−140°C for 30 min, 90°C for 5 min) or by using a Precellys 24 lysis and homogenization device, the PrecellysTM 24-cell mill and corresponding PrecellysTM 24 Kit VK05/VK01 (PEQLAB Biotechnologie, Erlangen, Germany). Samples were subjected to two times 6300 shakes min−1 for 20 s with an intermission of 20 s. After centrifugation for 10 min at 8000 × g, the supernatant was collected and the DNA extract was stored at −20°C. For the 16S rRNA gene amplifications (PCR), almost complete 16S rRNA gene fragments were obtained with the primers 27f and 1492r (Staufenberger et al., Citation2008). Subsequent sequencing was performed at the Institute for Clinical Molecular Biology (University Hospital Schleswig-Holstein, Kiel, Germany), and primers used for sequencing were 534r, 342f, and 790f, as described by Heindl et al. (Citation2010). The 16S rRNA gene sequences obtained in this study were deposited in NCBI GenBank under the accession numbers FR718448, FR775436–FR775439 and FR821090–FR821249.
Phylogenetic analysis
Sequences were edited using CHROMASPRO 1.33 (Technelysium, Cologne, Germany). Results were compared with other sequences in the EMBL prokaryotes database using BLAST, available online at the European Bioinformatics Institute homepage, as well as with the RDP database (Ribosomal Database Project; http://rdp.cme.msu.edu; Cole et al., Citation2009). Type strain relatives of all isolates were determined by comparison of 16S rRNA gene sequences in the EMBL prokaryotes database using BLAST (Altschul et al., Citation1990) and the online database LPSN (http://www.bacterio.net, Euzeby, Citation1997). The similarity matrix of the 16S rRNA gene sequences of all the strains was obtained using Phylogeny Interface Package (PHYLIP 3.9, Felsenstein, Citation1989).
The strains were grouped into phylotypes by sequence similarities of > 99.0%. One representative strain of each phylotype was selected for phylogenetic calculation and tree construction. In addition, all related type strains as well as selected non-type strains, were included in the phylogenetic analysis. Sequences were aligned using the CLUSTAL X program (Thompson et al., Citation1997) and manually corrected if necessary. The phylogenetic analysis was performed with the neighbour-joining (NJ) method (Saitou & Nei, Citation1987) using the Kimura 2-parameter model (Kimura, Citation1983) by the MEGA3.1 software package (Kumar et al., Citation2004). The topology of the trees was evaluated by bootstrap analysis (Felsenstein, Citation1985) based on 1000 resamplings (NJ). NJplot was used to display the phylogenetic trees (Perriere & Gouy,Citation1996).
Preparation of cell extracts
The bacterial strains were plated on the nutrient media (30 ml) that were used for their isolation, and incubated as mentioned above. After 2 weeks, the plates were homogenized with an Ultraturrax T25 and extracted with 20 ml ethylacetate. The obtained extract was concentrated under reduced pressure in a Speedvac RVC 2–33 (Christ, Germany) until completely dry and resuspended in 1 ml methanol. All employed solvents were of analytical reagent grade and obtained from Merck.
Antimicrobial assays
The antimicrobial activity of the extracts (10 µl) of the isolated bacteria was tested against a panel of eight microorganisms comprising standard test strains and macroalga-associated bacteria. The following microorganisms were used. These were (1) Four microorganisms usually tested in standard laboratory tests of antibiotic activity (hereafter ‘standard set’): Escherichia coli DSM 498 (nutrient media: TSB12 = 12 g l−1 TSB in distilled water) as a Gram-negative strain; Bacillus subtilis DSM 347 and Staphylococcus lentus DSM 6672 (both nutrient media: TSB3 = 3 g l−1 TSB in distilled water) as representative Gram-positive bacteria; and the yeast Candida glabrata DSM 6425 (nutrient medium 186/3 = 3 g l−1 yeast extract, 3 g l−1 malt extract, 5 g l−1 peptone from soymeal, 10 g l−1 glucose in distilled water). All four were obtained from the German Collection of Microorganisms and Cell Cultures (DSMZ, Braunschweig, Germany). And (2) four environmental strains that have been identified to be associated with macroalgae in previous studies (hereafter ‘macroalga-associated set’). Two isolates from F. vesiculosus were used, which affiliated to other macroalga-associated bacteria (see Goecke et al., Citation2010) with > 99% 16S rRNA gene sequence similarity, specifically to the Gram-positive Bacillus algicola KMM 3737T (AY228462) and Gram-negative Formosa algae KMM 3553T (AY228461) (isolates AB423f and AB356f, accession numbers FR775437 and FR775436, respectively: see Table S1 in Supplementary Information). The other two environmental strains were two Gram-negative bacterial strains identified as macroalgal pathogens (Sawabe et al., Citation1998, Citation2000): Algicola bacteriolytica ATCC 700679T (causing ‘red spot disease’) and Pseudoalteromonas elyakovii ATCC 700519T (causing ‘spot-wounded fronds’), originally isolated in Japan from diseased beds of Saccharina japonica (formerly Laminaria japonica). Both were purchased from Institute Pasteur CIP (Paris, France), and grown on nutrient medium TM (5 g l−1 yeast extract, 1 g l−1 peptone, and 30 g l−1 tropic marine sea salt in deionized water).
Assay mixtures were prepared by transferring 10 µl aliquots of methanolic solutions of extracts into a sterile 96-well microtitre plate and evaporating the solvent in a vacuum centrifuge (Schneemann et al., Citation2010). 200 μl overnight cultures (i.e. cultures initiated the day before and kept overnight) of each test strain were diluted to an OD = 0.05 determined by spectrophotometry in the corresponding liquid media (see above). Cultures were incubated at 28°C for 24 h under constant shaking at 600 rpm (Centrifuge Heraeus Multifuge 1 L-R Benchtop, Langenselbold, Germany). For evaluation of the cell viability, the reduction of resazurin to resorufin was assessed by measuring the intensity of fluorescence at 560Ex/590Em nm (Collins & Franzblau, Citation1997). The tests were performed in three replicate treatments. The resulting values were compared with those for a positive control (100 µg/well chloramphenicol for the bacteria, cycloheximide for C. glabrata), and respective negative controls wells: blank (extract, no bacteria), solvent (bacteria, no extract) and nutrient medium (no bacteria, no solvent) on the same plate (Schneemann et al., Citation2010).
Results
Isolation and phylogenetic classification of bacteria
Altogether, 166 bacterial strains were isolated from the two macroalgae using samples taken between July 2008 and January 2010 in the Kiel Fjord. Strains with 16S rRNA gene sequences of > 99.0% similarity were considered as one phylotype. Based on the specific level of sequence similarity and supported by the phylogenetic clustering, the most closely related type strains of all isolates were determined (see Table S1 in Online Supplementary Information). The strains affiliated to 82 phylotypes. Fifty-seven phylotypes (97 isolates) were associated with D. sanguinea and 43 phylotypes (69 isolates) with F. vesiculosus. Thirty-nine phylotypes were found associated exclusively with the red algae and 25 exclusively with the brown algae. Only 18 phylotypes were sharedby both macroalgae, of which seven had previously been described as associated with algae (, 2). Using one representative sequence foreach phylotype, a phylogenetic tree was constructed using 16S rRNA gene sequences (Figs 1–4), which was used for detailed analyses. The tree demonstrated that the bacteria isolated from F. vesiculosus and D.sanguinea belonged to seven classes offour bacterial phyla, the Firmicutes (Bacilli), Actinobacteria (Actinobacteria), Proteobacteria (Alphaproteobacteria, Betaproteobacteria, Gammaproteobacteria) and Bacteroidetes (Cytophagia and Flavobacteria) (Fig. 5).
The Bacteroidetes phylum contained 14 phylotypes () affiliating to Algoriphagus, Cellulophaga, Cyclobacterium, Formosa, Maribacter, Olleya, Tenacibaculum, Winogradskyella and Zobellia species.
Among the 34 phylotypes of Proteobacteria phylum, the eight phylotypes of the Alphaproteobacteria () were closely related to Hoeflea, Labrenzia, Paracoccus, Pseudorhodobacter, Sulfitobacter and Thalassobacter species. The 25 phylotypes of Gammaproteobacteria were closely related to species of Aeromonas, Cobetia, Glaciecola, Marinomonas, Microbulbifer, Pantoea, Photobacterium, Pseudoalteromonas, Pseudomonas, Psychrobacter, Rheinheimera, Shewanella and Vibrio species (see Table S1). Only a single phylotype of Betaproteobacteria (gene sequence AB432f) was isolated; this affiliated to a Hydrogenophaga species associated with Fucus vesiculosus ().
The Firmicutes phylum was represented by 19 phylotypes affiliating to the Bacilli (Fig. 3) with most sequences found to cluster close to Bacillus and Paenibacillus species. The Actinobacteria contained 15 phylotypes (Fig. 4) forming three branches, which clustered near Streptomyces, Nocardiopsis, Microbacterium and Salinibacterium species.
Detailed analyses of the culture data revealed astable component present over the seasons (winterand summer) with both macroalgae bearingrepresentatives of Bacilli, Flavobacteria andGammaproteobacteria. In addition, Alphaproteobacteria were constantly found in association with F. vesiculosus (Fig. 5). However, differences were found between the two algae and according to season (). Some phylotypes were present during winter and summer on both algae (e.g. Bacillus amyloliquefaciens, B. licheniformis, B. hwajinpoensis, Cellulophaga baltica and Olleya marilimosa: Table S1), whereas several others were isolated only in summer (e.g. Marinomonas dokdonensis, on both algae), or only in winter (e.g. Zobellia galactanivorans on F. vesiculosus, Microbulbifer thermotolerans on both algae, and Photobacterium halotolerans on D. sanguinea). In total 63 phylotypes were isolated just on a particular macroalga and only at one sampling season (see Table S1).
Antimicrobial activity
Antimicrobial activity was common among the isolates (). Of the total of 166 isolates, 85 (51%) were found to be antibiotic producers and active against at least one of the tested organisms. According to a sequence similarity matrix (> 99.0%) the 166 isolates belonged to 51 (active) phylotypes (62% of all phylotypes) (). The phylotypes thatpresented antimicrobial activity affiliated to thegenera Algoriphagus, Bacillus, Brevibacterium, Cellulophaga, Cobetia, Cyclobacterium, Formosa, Hoeflea, Marinomonas, Microbacterium, Microbulbifer, Nocardiopsis, Paenibacillus, Pantoea, Paracoccus, Photobacterium, Pseudoalteromonas, Pseudomonas, Pseudorhodobacter, Psychrobacter, Rheinheimera, Salinibacterium, Streptomyces, Vibrio and Winodragskyella (, for details see Table S2). Nevertheless, there was large variation in antibiotic activity even between strains belonging to the same phylotype (Table S1). Aparticularly high number of bioactive strainsbelonged to the genera Bacillus, Pseudoalteromonas and Streptomyces (, ).
The proportion of antibiotically active strains isolated from Delesseria sanguinea (53%, 36 phylotypes) was similar to that from Fucus vesiculosus (51%, 26 phylotypes) (,). Although, changes in the occurrence of associated epiphytic bacteria observed between summer and winter could affect the specific capacity to produce bioactive compounds, a final and particular seasonal pattern of bioactivity during the two years was not observed in either Fucus or Delesseria derived strains (Table S3). An important result was that a much higher proportion of isolates (46% of the Fucus vesiculosus and 45% of the Delesseria sanguinea isolates) were active against the macroalga-associated set of bacteria as compared with the standard set of microorganisms (13% and 19% respectively, ). Some of the phylotypes that showed antimicrobial activity were found associated exclusively with one ofthe macroalgae, either Fucus vesiculosus (Formosa algae, Marinomonas dokdonensis, Marinomonas polaris and Pseudomonas marincola) or Delesseria sanguinea (Algoriphagus yeomjeoni, Hoeflea alexandrii, Photobacterium halotolerans, Pseudo alteromonas ulvae and Streptomyces griseorubens, see detail in ), and displayed antimicrobial activity especially against potential competitors (Bacillus algicola and Formosa algae) or algal pathogen strains (Algicola bacteriolytica and Pseudoalteromonas elyakovii). Interestingly, of the 39 phylotypes that were associated exclusively with Delesseria sanguinea and of the 25 from Fucus vesiculosus, 19 and 13 phylotypes respectively exhibited activity against macroalga-associated bacterial strains (). Of the 18 bacterial phylotypes shared by both macroalgae, 72% were active against macroalga-associated bacteria (). As much as 11% of the isolates associated with F. vesiculosus and 14% of those associated with D. sanguinea were able to inhibit the growth of pathogens that affect brown macroalgae (, ).
Discussion
Our investigation on two co-occurring macroalgae Fucus vesiculosus and Delesseria sanguinea from the Kiel Fjord focused on the determination of phylogenetic diversity and antimicrobial activity of cultivable bacteria associated with the two algae. This approach was used because cultivation is essential to provide information on microbial physiology and in particular to prove the production of antibiotic compounds by bacteria.
Phylogenetic analysis using the 16S rRNA gene sequences of all 82 phylotypes demonstrated that the bacteria associated with F. vesiculosus and D. sanguinea were affiliated to four major phyla of the bacterial domain, Actinobacteria, Proteobacteria, Firmicutes and Bacteroidetes (Figs 1, 2). Bacteria belonging to those phyla are usually found in aquatic samples (Lachnit et al., Citation2011) and, as in the present study, Proteobacteria and Bacteroidetes are usually dominant (Cottrell & Kirchman, Citation2000; Longford et al., Citation2007). A prevalence of sequences from these two bacterial groups has been reported both in cultivation-based and molecular studies of phytoplankton (Jasti et al., 2005), green macroalgae (Shiba & Taga, Citation1980; Hengst et al., Citation2010; Barrott et al., Citation2011; Burke et al., Citation2011; Hollants et al., Citation2011), red macroalgae (Ismail-Ben Ali et al., Citation2012) and brown macroalgae including F. vesiculosus (Kong & Chan, Citation1979; Wieseet al., Citation2009; Barrott et al., 2011; Lachnit etal., Citation2011). Hence they emerge as important associates of algaein general (Longford et al., Citation2007; Tujula et al., Citation2010).
Among the 166 isolates, 42 strains were closely related to 17 type strains, which have been shown previously to be associated with algae (), indicating that they are typical species associated with algae. Nevertheless, the fact that some bacteria were found only on one algal species () indicates the presence of regulatory processes that act to selectively attract, favour or eliminate specific phylotypes (Sneed & Pohnert, Citation2011; Goecke et al., 2012). The physiological and biochemical properties of the macroalgae are important factors that determine the composition of microbial communities adhering to their surfaces (Beleneva & Zhukova, Citation2006) and secondary metabolites from the algal host might influence relationships between the bacteria and macroalgae (Goecke et al., Citation2010; Persson et al., Citation2011). In addition, the production of secondary metabolites by the bacteria may contribute to the chemical defence of the alga with which they are associated and such a mechanism has been postulated to act for macroalgae without their own chemical defence mechanism (Armstrong et al., Citation2001). Although this hypothesis needs to be investigated via ecological studies in the field, combined with molecular methods, it is supported by the fact that antimicrobial activity is widespread among alga-associated bacteria (Wiese et al., Citation2009, and see Tables 3 and S2). The proportion of antibiotic active strains isolated from Fucus vesiculosus (51% of the isolates, as many as 60% of the phylotypes) and Delesseria sanguinea (53% of the isolates, as many as 63% of the phylotypes) is remarkably high (, ). Nevertheless, the antibiotic activity profile varied greatly, even between strains belonging to the same phylotype. Different biological activity among different strains of the same species has been observed previously in Pseudoalteromonas and Bacillus strains (see Todorova & Kozhuharova, Citation2010; Vynne et al., Citation2011). Bacteria adapt rapidly to the environment and the production of secondary metabolites relies on many different factors (Bode et al., Citation2002). Loss of antibacterial activity due to cultivation conditions has also been observed by differences in nutrient conditions, storage, etc. (Vynne et al., Citation2011).
Previous studies with Fucus ceranoides have shown that 13% of bacteria (6 out of 45) associated with this alga had antibiotic activity (Lemos et al., Citation1985). In another study, 17% of bacterial isolates (13 out of 77) associated with F. serratus were found to produce antibiotic substances (Boyd et al., 1999). There has been no previous demonstration of antibiotic activity of bacteria associated with any species of Delesseria, though Skovhus et al. (Citation2007) identified several Pseudoalteromonas species associated with D. sanguinea, some of which comprised a bacterial ‘antifouling subgroup’. Particularly interesting is the specific action against possible competitors in the environment and the high portion (45–46%) of bacteria observed to be active against macroalga-associated bacteria (, ), as well as the presence of isolates active against pathogens affecting brown macroalgae (Figs 3a, b). In particular, representatives of Pseudoalteromonas and Bacillus species are efficient producers of antimicrobial compounds and therefore highly successful colonizers of macroalgal surfaces (Egan et al., 2001; Holmström et al., Citation2002; Burgess et al., Citation2003; Kanagasabhapathy et al., Citation2006; Skovhus et al., Citation2007) (Figs 1b, c, and Table S2). Although antimicrobial and antifouling metabolites are considered to target marine microorganisms, thesusceptibility of ecologically relevant bacteria has rarely been studied (Puglisi et al., Citation2007; Kanagasabhapathy et al., Citation2008; Vairappan et al., Citation2010), and this could explain the very much higher percentages of antibiotic activities presented here (as compared with standard-strain based studies).
Our study demonstrated seasonal and host-related variations in occurrence and bioactivity patterns of different phylotypes associated with a brown and a red macroalga living at the same location. Interactions between physicochemical (temperature, season) and biological factors (epibiosis) are the rule rather the exception, because the host and the epiphytes are both affected by the environment (Lobban & Harrison, Citation2000). Recently it has been demonstrated by molecular methods that the composition of the bacterial biofilm on Baltic Sea populations of Fucus vesiculosus varies with the season (Wahl et al., Citation2010; Lachnit et al., Citation2011). The present study also demonstrates the importance of environmental factors on epiphytic microbial communities (Table S1). If we consider the production of antimicrobial chemical defences by associated microorganisms as an added level of complexity to the mechanisms by which marine algae avoid the deleterious effects of microbial pathogens, changes in the community composition of epiphytic bacteria would affect the specific capacity to produce bioactive compounds and therefore also directly and indirectly would affect the associations between macroalgae and bacteria.
According to our results we conclude that:
• | epibiotic bacteria exhibit a great potential to produce antimicrobial metabolites, | ||||
• | there is great variation of epibiotic bacteria even between co-occurring host organisms, | ||||
• | season affects both host and epibiota, and is responsible for modifications on the epibiotic bacterial diversity. |
Supplementary material
Download Zip (64.3 KB)Acknowledgements
This study was financed by the DAAD (German Academic Exchange Service), the CONICYT (Comisión Nacional de Investigación Científica y Tecnológica, Chile), and the Ministry of Science, Economic Affairs and Transport of the State of Schleswig-Holstein (Germany) within the frame of the ‘Future Program of Economy’, which is supported by theEuropean fund for regional development (EFRE). We are grateful to the support given by the Kieler Wirkstoff-Zentrum (KiWiZ) am GEOMAR, Germany. We are grateful to Arlette Erhard, Dr Herwig Heindl andDr Tim Staufenberger for their kind help in methodology.
References
- Akagawa-Matsushita , M. , Matsuo , M. , Koga , Y. and YAMASATo , K . 1992 . Alteromonas atlantica sp. nov. and Alteromonas carrageenovora sp. nov., bacteria that decompose algal polysaccharides . International Journal of Systematic Bacteriology , 42 : 621 – 627 .
- Altschul , S.F. , Gish , W. , Miller , W. , Myers , E.W. and Lipman , D.J . 1990 . Basic local alignment search tool . Journal of Molecular Biology , 215 : 403 – 410 .
- Armstrong , E. , Yan , L. , Boyd , K.G. , Wright , P.C. and Burgess , J.G . 2001 . The symbiotic role of marine microbes on living surfaces . Hydrobiologia , 461 : 37 – 40 .
- Barbeyron , T. , L'haridon , S. , Corre , E. , Kloareg , B. and Potin , P . 2001 . Zobellia galactanovorans gen. nov., sp. nov., a marine species of Flavobacteriaceae isolated from a red alga, and classification of three novel Zobellia species [Cytophaga] uliginosa (ZoBell & Upham 1944) Reichenbach 1989 as Zobellia uliginosa gen. nov., comb . nov. International Journal of Systematic and Evolutionary Microbiology , 51 : 985 – 997 .
- Barrott , K.L. , Rodriguez-Brito , B. , Janouškovec , J. , Marhaver , K.L. , Smith , J.E. , Keeling , P. and Rohwer , F.L . 2011 . Microbial diversity associated with four functional groups of benthic reef algae and the reef-building coral Montastraea annularis . Environmental Microbiology , 13 : 1192 – 1204 .
- Beleneva , I.A. and Zhukova , N.V . 2006 . Bacterial communities of some brown and red algae from Peter the Great Bay, the Sea of Japan . Microbiology (Moscow) , 75 : 410 – 419 .
- Bode , H.B. , Bethe , B. , Höfs , R. and Zeeck , A . 2002 . Big effects from small changes: possible ways to explore nature's chemical diversity . ChemBioChem , 3 : 619 – 627 .
- Boyd , K.G. , Adams , D.R. and Burgess , J.G . 1999 . Antibacterial and repellent activities of marine bacteria associated with algal surfaces . Biofouling , 14 : 227 – 236 .
- Burgess , J.G. , Boyd , K.G. , Armstrong , E. , Jiang , Z. , Yan , L. , Berggren , M. , May , U.T. , Granmo , A. and Adams , D.R . 2003 . The development of a marine natural product-based antifouling paint . Biofouling , 19 : 197 – 205 .
- Burke , C. , Thomas , T. , Lewis , M. , Steinberg , P. and Kjelleberg , S . 2011 . Composition, uniqueness and variability of the epiphytic bacterial community of the green alga Ulva australis . ISME Journal , 5 : 590 – 600 .
- Cole , J.R. , Wang , Q. , Cardenas , E. , Fish , J. , Chai , B. , Farris , R.J. , Klam-Syed-Mohideen , A.S. , Mccarell , D.M. , Marsh , T. , Garrity , G.M. and Tiedje , J.M . 2009 . The Ribosomal Database Project: improved alignments and new tools for rRNA analysis . Nucleic Acids Research , 37 : D141 – 145 . (Web Server issue).
- Collins , L.A. and Franzblau , S.G . 1997 . Microplate alamar blue assay versus BACTEC 460 system for high-throughput screening of compounds against Mycobacterium tuberculosis and Mycobacterium avium . Antimicrobial Agents and Chemotherapy , 42 : 1004 – 1009 .
- Corre , A. and Prieur , D . 1990 . Density and morphology of epiphyte bacteria on the kelp Laminaria digitata . Botanica Marina , 33 : 515 – 523 .
- Cottrell , M.T. and Kirchman , D.L . 2000 . Natural assemblages of marine Proteobacteria and members of the Cytophaga–Flavobacter cluster consuming low- and high-molecular-weight dissolved organic matter . Applied and Environmental Microbiology , 66 : 1692 – 1697 .
- Dobretsov , S. , Dahms , H.U. and Qian , P.Y . 2006 . Inhibition of biofouling by marine microorganisms and their metabolites . Biofouling , 22 : 43 – 54 .
- Egan , S. , Thomas , T. , Holmström , C. and Kjelleberg , S . 2000 . Phylogenetic relationship and antifouling activity of bacterial epiphytes from the marine alga Ulva lactuca . Environmental Microbiology , 2 : 343 – 347 .
- Egan , S. , Holmström , C. and Kjelleberg , S . 2001 . Pseudoalteromonas ulvae sp. nov., a bacterium with antifouling activities isolated from the surface of a marine alga . International Journal of Systematic and Evolutionary Microbiology , 51 : 1499 – 1504 .
- Egan , S. , Thomas , T. and Kjelleberg , S . 2008 . Unlocking the diversity and biotechnological potential of marine surface associated microbial communities . Current Opinion in Microbiology , 11 : 219 – 225 .
- Engel , S. , Jensen , P.R. and Fenical , W . 2002 . Chemical ecology of marine microbial defense . Journal of Chemical Ecology , 28 : 1971 – 1985 .
- Engel , S. , Puglisi , M.P. , Jensen , P.R. and Fenical , W . 2006 . Antimicrobial activities of extracts from tropical Atlantic marine plants against marine pathogens and saprophytes . Marine Biology , 149 : 991 – 1002 .
- Euzeby , J.P . 1997 . List of bacterial names with standing in nomenclature: a folder available on the internet . International Journal of Systematic Bacteriology , 47 : 590 – 592 .
- Felsenstein , J . 1985 . Confidence limits on phylogenies: an approach using the bootstrap . Evolution , 39 : 783 – 791 .
- Felsenstein , J . 1989 . PHYLIP – Phylogeny Interface Package (Version 3.2) . Cladistics , 5 : 164 – 166 .
- Goecke , F. , Labes , A. , Wiese , J. and Imhoff , J.F . 2010 . Chemical interactions between marine macroalgae and bacteria . Marine Ecology Progress Series , 409 : 267 – 300 .
- Goecke , F. , Labes , A. , Wiese , J. and Imhoff , J.F . 2012 . Dual effect of macroalgal extracts on growth of bacteria in Western Baltic Sea . Revista de Biología Marina y Oceanografía , 47 : 75 – 86 .
- Harder , T . 2009 . “ Marine epibiosis: concepts, ecological consequences and host defence ” . In Marine and Industrial Biofouling , Berlin : Springer . (Flemming, H.C. Murthy, P.S. Venkatesan, R. & Cooksey, K. editors), 219–231
- Heindl , H. , Wiese , J. , Thiel , V. and Imhoff , J.F . 2010 . Phylogenetic diversity and antimicrobial activities of bryozoan-associated bacteria isolated from Mediterranean and Baltic Sea habitats . Systematic and Applied Microbiology , 33 : 94 – 104 .
- Hengst , M.B. , Andrade , S. , Gonzalez , B. and Correa , J.A . 2010 . Changes in epiphytic bacterial communities of intertidal seaweeds modulated by host, temporality, and copper enrichment . Microbial Ecology , 60 : 282 – 290 .
- Hollants , J. , Leroux , O. , Leliaert , F. , Decleyre , H. , De Clerck , O. and Willems , A . 2011 . Who is in there? Exploration of endophytic bacteria within the siphonous green seaweed Bryopsis (Bryopsidales, Chlorophyta) . PLOS ONE , 6 : e26458
- Holmström , C. , Egan , S. , Franks , A. , Mccloy , S. and Kjelleberg , S . 2002 . Antifouling activities expressed by marine surface associated Pseudoalteromonas species . FEMS Microbiology Ecology , 41 : 47 – 58 .
- Hughes , C.C. and Fenical , W . 2011 . Antibacterials from the sea . Chemistry – A European Journal , 16 : 12512 – 12525 .
- Ismail-Ben Ali , A. , El Bour , M. , Ktari , L. , Bolhuis , H. , Ahmed , M. , Boudabbous , A. and Stal , L.J . 2012 . Jania rubens-associated bacteria: molecular identification and antimicrobial activity . Journal of Applied Phycology , 24 : 525 – 534 .
- Ivanova , E.P. , Bakunina , I.Y. , Sawabe , T. , Hayashi , K. , Alexeeva , Y.V. , Zhukova , N.V. , Nicolau , D.V. , Zvaygintseva , T.N. and Mikhailov , V.V . 2002 . Two species of culturable bacteria associated with degradation of brown algae Fucus evanescens . Microbial Ecology , 43 : 242 – 249 .
- Ivanova , E.P. , Alexeeva , Y.A. , Zhukova , N.V. , Gorshkova , N.M. , Buljan , V. , Nicolau , D.V. , Mikhailov , V.V. and Christen , R . 2004a . Bacillus algicola sp. nov., a novel filamentous organism isolated from brown alga Fucus evanescens . Systematic and Applied Microbiology , 27 : 301 – 307 .
- Ivanova , E.P. , Alexeeva , Y.V. , Flavier , S. , Wright , J.P. , Zhukova , N.V. , Gorshkova , N.M. , Mikhailov , V.V. , Nicolau , D.V. and Christen , R . 2004b . Formosa algae gen. nov., sp. nov., a novel member of the family Flavobacteriaceae . International Journal of Systematic and Evolutionary Microbiology , 54 : 705 – 711 .
- Jasti , S. , Sieracki , M.E. , Poulton , N.J. , Giewat , M.W. and Rooney-Varga , J.N . 2005 . Phylogenetic diversity and specificity of bacteria closely associated with Alexandrium spp. and other phytoplankton . Applied and Environmental Microbiology , 71 : 3483 – 3494 .
- Johansen , J.E. , Nielsen , P. and Sjøholm , C . 1999 . Description of Cellulophaga baltica gen. nov., sp. nov. and Cellulophaga fucicola gen. nov., sp. nov. and reclassification of [Cytophaga] lytica to Cellulophaga lytica gen. nov., comb . nov. International Journal of Systematic Bacteriology , 49 : 1231 – 1240 .
- Kanagasabhapathy , M. , Sasaki , H. , Haldar , S. , Yamasaki , S. and Nagata , S . 2006 . Antibacterial activities of marine epibiotic bacteria isolated from brown algae of Japan . Annals of Microbiology , 56 : 167 – 173 .
- Kanagasabhapathy , M. , Sasaki , H. and Nagata , S . 2008 . Phylogenetic identification of epibiotic bacteria possessing antimicrobial activities isolated from red algal species of Japan . World Journal of Microbiology and Biotechnology , 24 : 2315 – 2321 .
- Kimura , M . 1983 . The neutral theory of molecular evolution , Cambridge : Cambridge University Press .
- Kong , M.K. and Chan , K.Y . 1979 . A study on the bacterial flora isolated from marine algae . Botanica Marina , 22 : 83 – 97 .
- Kumar , S. , Tamura , K. and Nei , M . 2004 . MEGA3: Integrated software for molecular evolutionary genetics analysis and sequence alignment . Briefings in Bioinformatics , 5 : 150 – 163 .
- Lachnit , T. , Blümel , M. , Imhoff , J.F. and Wahl , M . 2009 . Specific epibacterial communities on macroalgae: phylogeny matters more than habitat . Aquatic Biology , 5 : 181 – 186 .
- Lachnit , T. , Wahl , M. and Harder , T . 2010 . Isolated thallus-associated compounds from the macroalga Fucus vesiculosus mediate bacterial surface colonization in the field similar to that on the natural alga . Biofouling , 26 : 247 – 255 .
- Lachnit , T. , Meske , D. , Wahl , M. , Harder , T. and Schmitz , R . 2011 . Epibacterial community patterns on marine macroalgae are host-specific but temporally variable . Environmental Microbiology , 13 : 655 – 665 .
- Lemos , M.L. , Toranzo , A.E. and Barja , J.L . 1985 . Antibiotic activity of epiphytic bacteria isolated from intertidal seaweeds . Microbial Ecology , 11 : 149 – 163 .
- Lobban , C.S. and Harrison , P.J . 2000 . Seaweed ecology and physiology , Cambridge : Cambridge University Press .
- Longford , S.R. , Tujula , N.A. , Crocetti , G. , Holmes , A.J. , Holmström , C. , Kjelleberg , S. , Steinberg , P.D. and Taylor , M.W . 2007 . Comparisons of diversity of bacterial communities associated with three sessile marine eukaryotes . Aquatic Microbial Ecology , 48 : 217 – 229 .
- Muscholl-Silberhorn , A. , Thiel , V. and Imhoff , J.F . 2008 . Abundance and bioactivity of cultured sponge-associated bacteria from the Mediterranean Sea . Microbial Ecology , 55 : 94 – 106 .
- Nishijima , M. , Takadera , T. , Imamura , N. , Kasai , H. , An , K.D. , Adachi , K. , Nagao , T. , Sano , H. and Yamasato , K . 2009 . Microbulbifer variabilis sp. nov. and Microbulbifer epialgicus sp. nov., isolated from Pacific marine algae, possess a rod–coccus cell cycle in association with the growth phase . International Journal of Systematic and Evolutionary Microbiology , 59 : 1696 – 1707 .
- Nylund , G.M. , Persson , F. , Lindegarth , M. , Cervin , G. , Hermansson , M. and Pavia , H . 2010 . The red alga Bonnemaisonia asparagoides regulates epiphytic bacterial abundance and community composition by chemical defence . FEMS Microbiology Ecology , 71 : 84 – 93 .
- Palacios , L. , Arahal , D.R. , Reguera , B. and Marín , I . 2006 . Hoeflea Alessandri sp. nov., isolated from the toxic dinoflagellate Alexandrium minutum AL1V . International Journal of Systematic and Evolutionary Microbiology , 56 : 1991 – 1995 .
- Pankow , H. 1971 . Algenflora der Ostsee. 1. Benthos , Jena : Gustav Fischer .
- Penesyan , A. , Marshall-Jones , Z. , Holmström , C. , Kjelleberg , S. and Egan , S . 2009 . Antimicrobial activity observed among cultured epiphytic bacteria reflects their potential as source of new drugs . FEMS Microbiology Ecology , 69 : 113 – 124 .
- Perriere , G. and Gouy , M . 1996 . WWW-query: an on-line retrieval system for biological sequence banks . Biochimie , 78 : 364 – 369 .
- Persson , F. , Svensson , R. , Nylund , G.M. , Fredriksson , N.J. , Pavia , H. and Hermansson , M . 2011 . Ecological role of a secondary metabolite for a colonizing bacterial community . Biofouling , 27 : 579 – 588 .
- Puglisi , M.P. , Engel , S. , Jensen , P.R. and Fenical , W . 2007 . Antimicrobial activities of extracts from Indo-Pacific marine plants against marine pathogens and saprophytes . Marine Biology , 150 : 531 – 540 .
- Rao , D. , Webb , J.S. , Holmström , C. , Case , R. , Low , A. , Steinberg , P. and Kjelleberg , S . 2007 . Low densities of epiphytic bacteria from the marine alga Ulva australis inhibits settlement of fouling organisms . Applied and Environmental Microbiology , 73 : 7844 – 7852 .
- Romanenko , L.A. , Zhukova , N.V. , Lysenko , A.M. , Mikhailov , V.V. and Stackebrandt , E . 2003 . Assignment of “Alteromonas marinoglutinosa” NCIMB 1770 to Pseudoalteromonas mariniglutinosa sp. nov., nom. rev., comb . nov. International Journal ofSystematic and Evolutionary Microbiology , 53 : 1105 – 1109 .
- Saha , M. , Rempt , M. , Grosser , K. , Pohnert , G. and Weinberger , F . 2011 . Surface-associated fucoxanthin mediates settlement of bacterial epiphytes on the rockweed Fucus vesiculosus . Biofouling , 27 : 423 – 433 .
- Saitou , N. and Nei , M . 1987 . The neighbor-joining method: a new method for reconstructing phylogenetic trees . Molecular Biology and Evolution , 4 : 406 – 425 .
- Sawabe , T. , Makino , H. , Tatsumi , M. , Nakano , K. , Tajima , K. , Iqbal , M.M. , Yumoto , I. , Ezura , Y. and Christen , R . 1998 . Pseudoalteromonas bacteriolytica sp. nov., a marine bacterium that is the causative agent of red spot disease of Laminaria japonica . International Journal of Systematic Bacteriology , 48 : 769 – 774 .
- Sawabe , T. , Tanaka , R. , Iqbal , M.M. , Tajima , K. , Ezura , Y. , Ivanova , E.P. and Christen , R . 2000 . Assignment of Alteromonas elyakovii KMM162T and five strains isolated from spot wounded fronds of Laminaria japonica to Pseudoalteromonas elyakovii comb. nov. and the extended description of the species . International Journal of Systematic Bacteriology , 50 : 265 – 271 .
- Schneemann , I. , Nagel , K. , Kajahn , I. , Labes , A. , Wiese , J. and Imhoff , J.F . 2010 . Comprehensive investigation of marine Actinobacteria associated with the sponge Halichondria panacea . Applied and Environmental Microbiology , 76 : 3702 – 3714 .
- Shiba , T.O. and Taga , N . 1980 . Heterotrophic bacteria attached to seaweeds . Journal of Experimental Marine Biology and Ecology , 47 : 251 – 258 .
- Skovhus , T.L. , Holmström , C. , Kjelleberg , S. and Dahllöf , I . 2007 . Molecular investigation of the distribution, abundance and diversity of the genus Pseudoalteromonas in marine samples . FEMS Microbiology Ecology , 61 : 348 – 361 .
- Sneed , J.M. and Pohnert , G . 2011 . The green alga Dictyosphaeria ocellata and its organic extracts alter natural bacterial biofilms communities . Biofouling , 27 : 347 – 356 .
- Staufenberger , T. , Thiel , V. , Wiese , J. and Imhoff , J.F . 2008 . Phylogenetic analysis of bacteria associated with Laminaria saccharina . FEMS Microbiology Ecology , 64 : 65 – 77 .
- Thompson , J.D. , Gibson , T.J. , Plewniak , F. , Jeanmougin , F. and Higgins , D.G . 1997 . The ClustalX windows interface: flexible strategies for multiple sequence alignment aided by quality analysis tools . Nucleic Acids Research , 24 : 4876 – 4882 .
- Todorova , S. and Kozhuharova , L . 2010 . Characteristics and antimicrobial activity of Bacillus subtilis strains isolated from soil . World Journal of Microbiology and Biotechnology , 26 : 1207 – 1216 .
- Tujula , N.A. , Crocetti , G.R. , Burke , C. , Thomas , T. , Holmström , C. and Kjelleberg , S . 2010 . Variability and abundance of the epiphytic bacterial community associated with a green marine Ulvacean alga . ISME Journal , 4 : 301 – 311 .
- Vairappan , C.S. , Anangdan , S.P. , Tan , K.L. and Matsunaga , S . 2010 . Role of secondary metabolites as defense chemicals against ice–ice disease bacteria in biofouler at carrageenophyte farms . Journal of Applied Phycology , 22 : 305 – 311 .
- Villarreal-Gómez , L.J. , Soria-Mercado , I.E. , Guerra-Rivas , G. and Ayala-Sánchez , N.E . 2010 . Antibacterial and anticancer activityof seaweeds and bacteria associated with their surface . Revista de Biología Marina y Oceanografía , 45 : 267 – 275 .
- Vynne , N.J. , Mansson , M. , Nielsen , K.F. and Gram , L . 2011 . Bioactivity, chemical profiling, and 16S rRNA-based phylogeny of Pseudoalteromonas strains collected on a global research cruise . Marine Biotechnology , 13 : 1062 – 1073 .
- Wahl , M. , Shahnaz , L. , Dobretsov , S. , Saha , M. , Symanowski , F. , David , K. , Lachnit , T. , Vasel , M. and Weinberger , F . 2010 . Ecology of antifouling resistance in the bladder wrack Fucus vesiculosus: patterns of microfouling and antimicrobial protection . Marine Ecology Progress Series , 411 : 33 – 48 .
- Wiese , J. , Thiel , V. , Nagel , K. , Staufenberger , T. and Imhoff , J.F . 2009 . Diversity of antibiotic active bacteria associated with the brown alga Laminaria saccharina from the Baltic Sea . Marine Biotechnology , 11 : 287 – 300 .
- Zheng , L. , Han , X. , Chen , H. , Lin , W. and Yan , X . 2005 . Marinebacteria associated with marine macroorganisms: the potential antimicrobial resources . Annals of Microbiology , 55 : 119 – 124 .
Supplementary information
The following supplementary material is available for this article, accessible via the Supplementary Content tab on the article's online at http://dx.doi.org/10.1080/09670262.2013.767944.
Supplementary –3: closest relatives of bacterial strains from the two macroalgae, based on 16S rDNA sequences, antibacterial activity of strains, and summary of isolates, respectively.
Alignment of 16S rDNA (fasta format).