Abstract
We conducted two temperature experiments to investigate the invasion success of the coarsely branched red algae, Gracilaria vermiculophylla. Our working hypothesis was that the coarsely branched G. vermiculophylla, with well-known broad environmental tolerances, would have physiological traits in-between the typical r and K strategies. A factorial experiment provided light-dependent models of growth rate at six temperatures, with maximum growth of 0.045 day−1 at 20°C. Light-saturated growth and maximum light utilization efficiency both displayed a bell-shaped temperature dependency, with optima at 21°C and 23°C respectively. The minimum light required to maintain growth was low (<1 µmol photons m−2 s−1) for lower temperatures (5–20°C) and increased exponentially to 7 µmol photons m−2 s−1 at 30°C, documenting that G. vermiculophylla has a wide tolerance to low light levels under temperature ranges occurring in the upper littoral zone in the Baltic Sea. A second experiment investigated the metabolic acclimation of G. vermiculophylla to four temperatures, while comparing its physiological responses to those of two native species, Fucus vesiculosus and Ulva lactuca. This experiment showed that the optimum temperature for light-saturated photosynthesis increased for all three species as they became long-term acclimated to higher temperatures. Short-term incubation at high temperature (30°C) was suboptimal for all three algal species when grown at low temperatures (10–15°C) but the algae were unaffected when cultured at higher temperatures (20–25°C). Finally we evaluated the capacity of each of the three species for metabolic homeostasis and found that F. vesiculosus and G. vermiculophylla had an almost identical metabolic performance regardless of acclimation temperature, whereas net photosynthesis of U. lactuca increased significantly with growth temperature. Our results show that G. vermiculophylla shares traits with both the slow-growing, leathery F. vesiculosus (K-strategy) and the fast-growing, sheet-like U. lactuca (r-strategy), by combining relatively high growth rates with a robust metabolic response to changing temperatures. In conclusion, we suggest that having both K and r metabolic traits explains, in part, the invasion success of G. vermiculophylla in temperate estuaries.
Introduction
Gracilaria vermiculophylla is a coarsely branched red algae that originates from the NE Pacific, but has been introduced to the E and W Atlantic and the W Pacific oceans, probably in association with oyster aquaculture (Saunders, Citation2009; Kim et al., Citation2010). In the E Atlantic, G. vermiculophylla was first observed along the French coastline (Mollet et al., Citation1998; Rueness, Citation2005). Following the first sighting in Europe, it has subsequently been found south of France, in Spain, Portugal, Italy and Morocco (Kim et al., Citation2010; Sfriso et al., Citation2010); and also northwards, in the North Sea and Wadden Sea, and penetrating into the Baltic Sea, where it is now present on the west coast of Sweden (Nyberg, Citation2006; Thomsen et al., Citation2007; Nyberg et al., Citation2009), in many of the Danish fjords in the Baltic area (Thomsen et al., Citation2007, 2008), and in the bay of Kiel (Weinberger et al., Citation2008).
The ecological impact of invasive species in marine systems is poorly understood (Ruiz et al., Citation1999; Thomsen et al., Citation2011) and it is possible the recent invasion of G. vermiculophylla into the Baltic Sea may have negative effects on the native flora and fauna. For example, G. vermiculophylla can smother sessile oyster communities (Thomsen & McGlathery, Citation2006) and have negative effects on seagrasses, particularly at high temperatures (Martinez-Luscher & Holmer, Citation2010; Höffle et al., Citation2011). However, other studies suggest that the introduction of G. vermiculophylla into habitats with little substratum suitable for macroalgal attachment, may increase species diversity (Ruesink et al., Citation2006; Nyberg et al., Citation2009; Thomsen et al., Citation2010; Thomsen, Citation2010).
To understand which environmental conditions control survival and growth, and thus the potential geographical distribution of G. vermiculophylla, studies have tested for the effects of stable (Rueness, Citation2005; Nejrup & Pedersen, Citation2012) and fluctuating (Nejrup & Pedersen, Citation2012) salinities, local grazing (Nejrup & Pedersen, Citation2010; Nejrup et al., Citation2012) and nutrients (Tyler & McGlathery, Citation2006; Thomsen & McGlathery, Citation2007; Nejrup & Pedersen, Citation2010; Abreu et al., Citation2011), and tolerances to sediment load and desiccation stress (Thomsen & McGlathery, Citation2007). However, it remains unclear why G. vermiculophylla has an advantage compared to native species of macroalgae in the shallow estuaries of Scandinavia. We hypothesized that the apparent success of G. vermiculophylla in shallow Scandinavian estuaries may, in part, be due to a high tolerance to extremes of, and changes in, light and temperature.
Growth of macroalgae is fundamentally regulated by temperature and light (Lobban & Harrison, Citation1994; Raikar et al., Citation2001; Mendes et al., Citation2012) and knowing a species’ physiological response to these parameters is of vital importance to predict future distributions of non-indigenous macroalgae. Without quantitative data, predictive modelling is impossible (Kearney & Porter, Citation2009). Several studies have quantified the responses of G. vermiculophylla to manipulated levels of light and temperature in controlled laboratory experiments (Yokoya et al., Citation1999; Raikar et al., Citation2001; Rueness, Citation2005; Phooprong et al., Citation2008; Abreu et al., Citation2011; see also for an overview); it should be noted that none of these studies developed predictive regression models for light and temperature responses. The algae used in these experiments were collected from several Japanese, one Portuguese and one French population of G. vermiculophylla and the results indicate that the species has a broad tolerance to many environmental variables. However, the presence of regional ecotypes within Gracilaria species (McLachlan & Bird, Citation1984) makes it less certain how Scandinavian populations of G. vermiculophylla will respond to these environmental variables. Growth in Scandinavian G. vermiculophylla populations has only been measured in field experiments. However, field experiments are characterized by uncontrolled co-varying factors such as day length, grazing rates and nutrient levels, making it difficult to identify temperature and light responses (Weinberger et al., Citation2008; Nejrup & Pedersen, Citation2010). To understand the mechanisms that control growth of G. vermiculophylla in the Baltic region and to be able to generate quantitative and predictive performance models, controlled laboratory experiments that focus on the effects of light and temperature, and also potential interactive effects, are required. Using such data in, for example, spatial ecological modelling, may reveal important patterns relevant to the future distribution of G. vermiculophylla (Kearney & Porter, Citation2009).
Water temperature and light levels vary substantially from winter to summer in shallow Danish estuaries: they range from 0 to 27°C and from 0 to more than 600 µmol photons m−2 s−1 (Staehr & Borum, Citation2011). Tolerance of, and the ability to adapt to, these changing environmental conditions is likely to influence the ecological performance of G. vermiculophylla (and native co-existing algae) and may therefore also confer competitive advantages.
Algae can induce a range of biochemical adaptations to withstand changing light regimes at different water temperatures. At low temperatures, constraints on enzyme activity, membrane fluidity, and light and nutrient uptake reduce metabolic rates (Staehr & Wernberg, Citation2009) and growth (Lapointe et al., Citation1984). As temperature increases, these constraints are gradually reduced, resulting in an increase in metabolic rates and nutrient demand, which also increases the likelihood of nutrient limitation. Beyond the optimum temperature, metabolic rates decline due to limitation in enzyme capacity (Atkin & Tjoelker, Citation2003) and there is reduced production of ATP due to constrained activity of Rubisco activase (Jensen, Citation2000). High temperatures eventually reduce metabolic performance through inactivation or denaturation of proteins. The ability to acclimate to changing temperature varies among species and plant types. For example, fast-growing microalgae can display significant capacity for physiological adjustment within a week (Staehr & Birkeland, Citation2006), compared to seasonal adjustments in slow-growing seagrasses (Staehr & Borum, Citation2011).
We conducted two experiments to test the temperature and light dependency of growth and metabolism in G. vermiculophylla over a wide range of temperature and light combinations. First we tested for interactive effects of light and temperature on somatic growth, using six light levels crossed with six temperatures. We hypothesized that minimum light requirements for growth are low for G. vermiculophylla but that these will increase at high temperatures to balance the enhanced respiratory costs. Second, we investigated in more detail the ability of G. vermiculophylla to acclimate its metabolic rates to increasing short-term incubation temperatures, and measured the associated changes in the tissue concentration of chlorophyll a, nitrogen and carbon. This experiment also included two native species with wide geographical ranges that overlap with that of G. vermiculophylla and that represent different form-functional groups (Littler & Littler, Citation1980) and growth strategies (Pedersen & Borum, Citation1996). These were the slow-growing, thick, leathery alga Fucus vesiculosus and the fast-growing, sheet-forming alga Ulva lactuca, which represent archetypal K and r growth strategies, respectively. We hypothesized that the coarsely branched G. vermiculophylla, with its well-known broad environmental tolerances (e.g. Phooprong et al., Citation2008), would have physiological traits in-between the typical r and K strategies. That is, G. vermiculophylla would have growth rates, and also an ability to adjust optimum temperatures and metabolic rates to different temperature regimes, that are higher than F. vesiculosus but lower than U. lactuca.
Materials and methods
Effect of light and temperature on growth – Experiment I
We first tested for interactive effects of water temperature and light on somatic growth of Gracilaria vermiculophylla. Algae were collected at Holckenhavn Fjord (Fynen, Denmark, 55°17.8′N, 10°46.2′E) in August 2007 (water temperature 16°C) and cultured for 6 weeks at 15°C under a 16 : 8 h light : dark cycle and c. 160 µmol photon m−2 s−1. Algal thalli were exposed to six temperatures (5, 10, 15, 20, 25 or 30°C) fully crossed with six light levels (0, 9, 16, 34, 80 or 225 µmol photons m−2 s−1). A total of 108 algal thalli (N = 3 for each light–temperature combination, each thallus having a blotted wet weight (WW) of 0.848 ± 0.308 g, mean ± sd) were incubated in separate 1.8-l clear plastic containers with ∼ 1.7 l seawater. Light levels were controlled by placing different combinations of spectrally neutral black nylon-net over each 1.8-l container. The treatment receiving 0 µmol photons m−2 s−1 was wrapped in black plastic. The temperature was maintained using circulation pumps in one thermostat-controlled water bath per temperature treatment. Algal thalli were acclimated to each of the 36 combinations of temperatures and light for a minimum of 1 week. Water was diluted from natural seawater (30–35 psu, 1.3 mM total carbon at pH = 7.9) to 20 psu (frequently observed in Holckenhavn Fjord, from where the alga were collected, and a typical salinity for Danish waters in general) with tap water (2.5 mmol total carbon, pH = 8.3). The water was changed twice per week to maintain inorganic carbon levels. Five slow-release fertilizer pellets (PlataCore Depot 6M, Uranium Agrochem, Germany; total N = 14%, total P = 4%, mean weight = 0.14 ± 0.4 g) were added to each container to avoid nutrient limitation, and oxygen levels were maintained by air bubbling through air-stones. Blotted Wet Weight was determined two to three times per week during the 3-week experiment. Relative growth rate (µ) was calculated from:
Effect of temperature on cellular physiology – Experiment II
The effect of temperature acclimation on photosynthetic and respiratory performance was tested on G. vermiculophylla, U. lactuca and F. vesiculosus. Ulva lactuca and F. vesiculosus were collected at Nivå Harbour (Sealand, Denmark, 55°56.4′N, 12°31.6′E) and G. vermiculophylla at Holckenhavn Fjord during summer 2007. Individual algal thalli were incubated in 2.0-l glass containers with ∼ 1.8 l seawater, placed in temperature-controlled water baths. For each species, four replicate experiments were conducted with an initial algal thallus biomass of 0.69 ± 0.20 g WW for F. vesiculosus, 0.99 ± 0.23 g WW for G. vermiculophylla and 3.18 ± 0.84 g WW for U. lactuca (in the form of 3-cm diameter circular disks). Before the experiment, all tissues were cleaned of epiphytes and then placed in a glass container receiving 150 µmol photons m−2 s−1 in a 16 : 8 light : dark cycle in 180 µm filtered seawater (20 psu) with a modified O2 medium containing nitrogen, phosphorus and trace elements (Staehr & Birkeland, Citation2006). The water was changed every week to ensure nutrient-replete conditions.
The temperature was slowly changed (at a maximum of 5°C per day) from 14°C to a final experimental temperature of 10, 15, 20 or 25°C (N = 4). After reaching the final temperature (typically after 2 days), algae were long-term acclimated for 23 days at constant temperature. Wet weight was measured and two-thirds of the water exchanged twice per week. All other experimental parameters were maintained as described above during the 23-day period. Growth during this long-term acclimation was measured as in experiment I. Photosynthesis and respiration were measured during short-term incubations of each thallus at the end of the long-term experiment. Thallus segments (approximately 0.03 g dry weight) were cleaned of epiphytes prior to these short-term incubations in 25-ml glass bottles filled with GFC-filtered (1 µm) seawater. Dry weight was determined by drying thallus segments at 100°C to constant weight, which was typically 24 hours. Segments were small enough to ensure full light exposure and large enough to detect significant changes in dissolved oxygen. Bottles were placed on a rotating wheel and incubated in darkness and at saturating light (410 µmol photons m−2 s−1). A rotating glass bean was added to each bottle to avoid diffusion limitation, and incubation duration was adjusted to keep changes in oxygen saturation below c. 50% within the incubation period. For each series of measurements, three control bottles without algae were incubated in darkness to account for background oxygen consumption. The control bottles were filled successively, so that one bottle was filled at the beginning, one bottle in the middle and one bottle at the end of the bottle-filling procedure for each series. Changes in oxygen concentrations were measured at 10, 20, 25 and 30°C (N = 4). Incubations ran for 45, 60, 90 and 90 min at 30, 25, 15 and 10°C, respectively, to ensure measurable changes in oxygen concentration. After incubation, bottles were kept in the dark at the experimental temperature to stop oxygen production while handling the other bottles. Oxygen concentrations were measured with a Clark-type oxygen microelectrode (OX500, Unisense; Aarhus, Denmark) and recorded using a picoammeter (PA2000, Unisense). In each temperature experiment, the electrode was calibrated to 0 and 100% of air saturation.
Total chlorophyll a was determined spectrophotometrically according to Jeffrey & Humphrey (Citation1975). Organic carbon (C) and nitrogen (N) were measured using a Carlo–Erba EA–1108 CHN analyser (Carlo–Erba, Milano, Italy) according to the manufacturer's recommendations.
Curve fitting
Parameters describing the relationship between growth rate and light level in experiment I were obtained from a nonlinear regression fit of specific growth rate (µ) as a function of the photon flux density (E) according to a saturating exponential model (Webb et al., Citation1974):
The temperature responses of NP max and R thallus (explained below) were fitted to the equations (equation 3) following Johnson et al. (Citation1974), as modified by Staehr & Birkeland (Citation2006). This formula assumes that a single temperature-sensitive enzyme, with active and inactive conformations, controls the fitted metabolic process (for its application to plant photosynthesis, see Santamaria & Van Vierssen, Citation1997):
Statistical analysis
Two-way ANOVA was used to test for the effects of all experimental variables in experiment I and experiment II. In experiment I, we used a 2-way ANOVA to test for the effect of light and temperature on growth. In experiment II, we first used 2-way ANOVA to test for the effects of long-term temperature acclimation, between the three macroalgae species, on the response parameters of growth and the thallus concentration of chlorophyll a, carbon and nitrogen. Then we used 2-way ANOVA to test for interactive effects of long-term acclimation temperature and short-term incubation temperature on net photosynthesis and dark respiration. These analyses were carried out separately for all three species. Tukey's test was used to compare pairs of mean when ANOVA identified significant differences. A Kolmogorov–Smirnoff goodness-of-fit test was used to test for normality and Levene's test was used to test for homogeneity of variances. Data were ln-transformed when necessary to obtain normally distributed data and equal variances. All statistical analyses were carried out using SYSTAT (Systat Software, Chicago, IL, USA).
Results
Effect of light and temperature on growth – Experiment I
The growth rate of G. vermiculophylla increased significantly with light at all temperatures, but there was a strong interaction in which the light dependency of growth differed significantly between experimental temperatures (, ). Growth rates showed a saturating relationship to light for all experimental temperatures (, , all P values <0.01). Growth increased significantly with temperature and light, and the highest growth rate (0.045 ± 0.001 day−1, mean ± SD) was observed for the combination of 20°C and 225 µmol photons day−1 s−1. In the dark treatments there was no net growth or appreciable loss, demonstrating that G. vermiculophylla can maintain almost constant biomass, even at high temperatures, after 3 weeks of darkness. In the 5°C treatment, G. vermiculophylla had a low overall growth rate regardless of the light level (), which was significantly lower than at all other temperatures (Tukey test: P <0.000). The relationship between the maximum specific growth rate and temperature was bell-shaped (), with a fitted optimum of 21°C. A similar unimodal pattern was found for light utilization efficiency (α; ), although this peaked slightly higher, at 23°C (∼0.3 d−1 mol−1 photons m−2). However, the pattern for the light compensation levels (E C; ) was different; E C was low from 5 to 20°C (<1 µmol photons m−2 s−1) but significantly higher at 25°C (4 µmol photons m−2 s−1) and 30°C (7 µmol photons m−2 s−1). Thus, the five-fold increase in E C in the 30°C treatment, compared with the 0 to 20°C treatments, indicated that the increase in respiration exceeds the increase in photosynthesis with increasing temperature.
Fig. 1. Experiment I: Specific growth rates as a function of irradiance of G. vermiculophylla at (A) 5, 10 and 15ºC and (B) 20, 25 and 30ºC. Equations for each curve are shown. See text for explanation of equation 1. Errors are SE, N = 3.
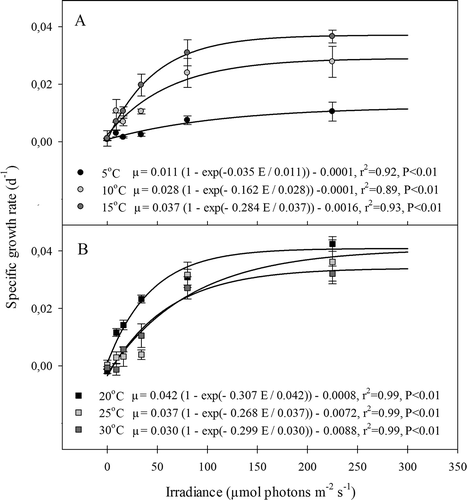
Table 1 Gracilaria vermiculophylla: ANOVA for the effects of temperature and light and interactions between the two factors for experiment I and experiment II. C = organic carbon; N = Nitrogen; C : N = weight ratio of C and N; and Chl a = Chlorophyll a. Bold indicates significant result
Fig. 2 Experiment I: Parameters derived from models of growth rate vs light curves. (A) Light-saturated growth rate (µmax); (B) initial slope of the growth vs. light curve (α) and (C) minimum light demand (EC) to maintain a net positive growth rate. Errors are SE, N = 3. Curves in A and B are fitted using a Gaussian model and C with an exponential growth model. According to the Gaussian curve-fits, optimum temperatures for µmax and α were 21ºC and 23ºC respectively.
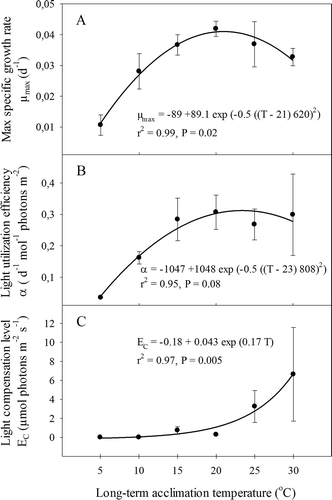
Effect of temperature on growth and cellularphysiology – Experiment II
All alga species showed a unimodal dependency of light-saturated growth on increasing temperature, with optimum temperatures at around 20°C (). Growth increased significantly with temperature from 10°C to 20°C (2-way ANOVA: F = 6.30, P = 0.002) with species having significantly different growth rates (2-way ANOVA: F = 33.10, P = 0.0001) but no interaction effect (2-way ANOVA: F = 1.294, P = 0.285). While G. vermiculophylla and U. lactuca had almost identical growth rates, F. vesiculosus had growth rates half that of the other two species, with the lowest rate found at 25°C (∼ 0.01 day−1).
Fig. 3 Experiment II: Specific growth rates for three macroalgal species in response to four growth temperatures. Errors are SE, N = 4.
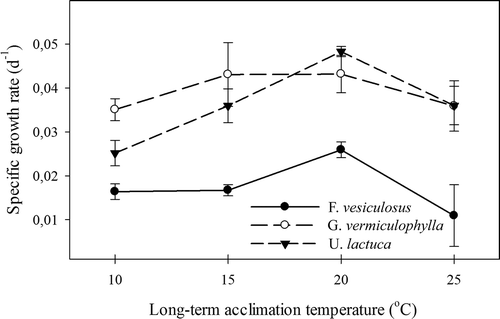
Significant interactions were observed for all cell physiology parameters (C, N, C : N and chlorophyll a: ), indicating that temperature responses varied between the three species. Cellular chlorophyll a was generally highest for F. vesiculosus, lowest for U. lactuca and intermediate for G. vermiculophylla when comparing all temperatures (). The cellular content of nitrogen decreased with increasing temperature for G. vermiculophyllaand U. lactuca but remained relatively constant for F. vesiculosus across temperatures. Cellular carbon content decreased with increasing temperature when comparing all species ( and ). However, we found no significant effect of temperature for the individual species (1-way ANOVA, P > 0.05). Finally, we found a significant interaction between temperature and species (F = 7.004, P <0.001) for the weight ratio of carbon to nitrogen (C : N), which increased with increasing temperature for G. vermiculophylla and U. lactuca (almost identical) but decreased slightly for F. vesiculosus.
Table 2 Experiment II: cellular characteristics of Fucus vesiculosus, Gracilaria vermiculophylla and Ulva lactuca long-term acclimated to four different growth temperatures. Measurements (mean ± SD, N = 4) were made of chlorophyll a (Chl a; µg g−1 DW), organic carbon (C; mg g−1 DW) and nitrogen (N; mg g−1 DW), and the weight ratio of C and N
Temperature dependency and sensitivity of metabolic rates – Experiment II
We found a general tendency for light-saturated photosynthesis (NP max) and dark respiration (R dark) to increase for all algae species as a function of short-term incubation temperature ( and ). The ‘cold’ (i.e. 10 and 15°C) and ‘warm’ (i.e. 20 and 25°C) long-term acclimated algae tended to respond differently to short-term incubations at increasing temperatures (). The effect on NP max and R dark of long-term acclimation temperature and short-term incubation temperature (see Table 3) was significant for all species (P <0.001, ). Furthermore, a significant short-term × long-term temperature interaction effect was observed for all species except with respect to the respiratory response of U. lactuca (F = 0.675, P = 0.727). This interaction effect indicated, with the exception of U. lactuca, that the response of the algae to increasing short-term incubation temperature was significantly affected by the temperature to which the algae had been long-term acclimated. At low short-term incubation temperature (10°C), the photosynthetic rates of species that were long-term acclimated to cold temperatures were generally higher than those that were long-term acclimated to warm temperature. In comparison, at high incubation temperature (30°C) these cold-acclimated algae generally had lower photosynthetic responses than the warm-acclimated algae. Exceptions to this pattern were the unexpectedly high photosynthetic rates of U. lactuca and F. vesiculosus that had been long-term acclimated to 25°C (, ). The suboptimal conditions for cold-acclimated algae at high temperatures were also seen in elevated respiration rates, except in F. vesiculosus (). Warm-acclimated algae generally displayed lower respiration rates than the cold-acclimated ().
Fig. 4 Experiment II: Light-saturated net photosynthesis of short-term incubations to different long-term acclimation temperatures of (A) F. vesiculosus; (B) G. vermiculophylla and (C) U. lactuca. Errors are SE, N = 4.
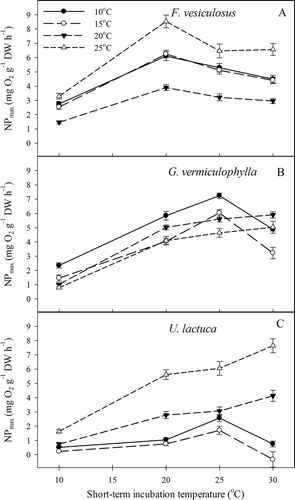
Fig. 5 Experiment II: Dark respiration rates of short-term incubations to different long-term acclimation temperatures of (A) F. vesiculosus; (B) G. vermiculophylla and (C) U. lactuca. Errors are SE, N = 4.
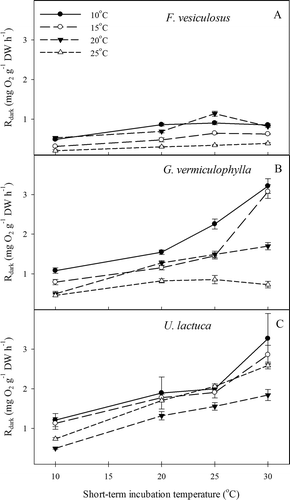
Table 3 Experiment II: parameter estimates from temperature response curves of light-saturated net photosynthesis (NP max) and dark respiration (R dark) of Fucus vesiculosus, Gracilaria vermiculophylla and Ulva lactuca long-term acclimated to different growth temperatures. E a/R gas is the activation energy/gas constant and ΔH/R gas the increase in entropy/gas constant, which are coefficients in the Johnson et al. (Citation1974) temperature response model. Values are mean ± SD (N = 4)
Table 4 Experiment II: 2-way ANOVA for the interaction effects of acclimation temperature and incubation temperature of light-saturated photosynthesis (NP max), dark respiration (R dark) and the metabolic adjustments (Topt NPmax) for three macroalgal species
The optimum temperature for light-saturated net photosynthesis (T opt of NP max) varied significantly between species and between long-term acclimation temperatures (F = 5.620, P <0.001); this interaction occurred because T opt increased for G. vermiculophylla and U. lactuca, but not for F. vesiculosus (). At the highest acclimation temperature (25°C), G. vermiculophylla and U. lactuca had optimum temperatures of 25.7 ± 2.2°C (mean ± SD) to 26.3 ± 3.9°C, respectively, which were not significantly different from each other (Tukey's test, P = 0.502). In comparison, F. vesiculosus had a relatively constant T opt of c. 22°C (), which was significantly lower than for the two other species (Tukey's test, P <0.0001). Thus, while G. vermiculophylla and U. lactuca displayed a strong ability to adjust their optimum temperature for photosynthesis towards the long-term acclimation temperature, metabolic changes for F. vesiculosus were less pronounced.
Fig. 6 Experiment II: Metabolic adjustments for three macroalgae species acclimated to four growth temperatures. (A) Optimum temperature of light-saturated net photosynthesis (NP max), and (B) NP max values at short-term incubation temperatures corresponding to the same long-term acclimation temperatures. Errors are SD, N = 4.
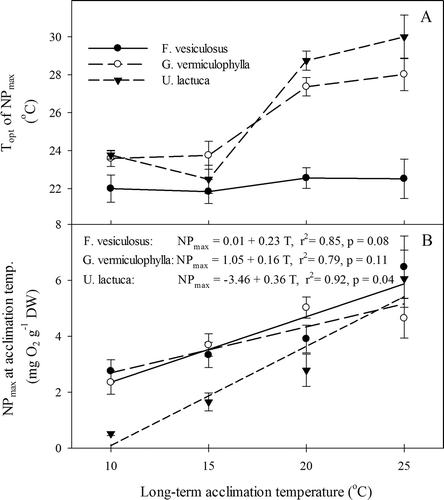
Table 5 Laboratory studies of temperature dependence of somatic growth, light-saturated photosynthesis (NP max) and respiration rates. If the authors worked with both gametophyte and tetrasporophytes, we show data only for tetrasporophytes (Yokoya et al., Citation1999; Abreu et al., Citation2011). E c = the minimum light requirement (µmol photons m−2 s−1). NA = data not available. Studies marked with an asterisk include factorial-designed experiments to test for interaction of temperature with light and/or salinity. Studies marked with # indicate where temperature dependencies were parameterized
To further evaluate the ability to adjust metabolism to prevailing growth temperatures, we compared the rates of light-saturated net photosynthesis between algae that had been long-time acclimated and short-term incubated at the same temperature (i.e. an algae long-term acclimated at 10°C was short-term incubated at 10°C; ). Algae acclimated to a given growth temperature are expected to optimize their cellular physiology (pigmentation, carbon hydrates, enzyme concentration) to the prevailing growth conditions. As a result, full acclimation (metabolic homeostasis) should result in similar net photosynthetic rates across a range of acclimation temperatures (Davison et al., Citation1991). In accordance with this, we would expect the slope of the regression line describing net photosynthetic rates at corresponding long-term acclimation and short-term incubation temperatures to be zero for a species with full ability to acclimate. NP max increased with increasing temperature for all three species () but the slope was significantly different from zero only in U. lactuca; though insignificant, the trend was similar in G. vermiculophylla (P = 0.11) and F. vesiculosus (P = 0.08). Consequently, U. lactuca had much lower photosynthetic rates at lower temperatures than G. vermiculophylla and F. vesiculosus, suggesting a higher degree of metabolic homeostasis in the two latter species.
The sensitivity of metabolism to suboptimal temperatures can be evaluated using the E a/R gas expression, which describes the initial slope of the fitted curve of temperature-dependent photosynthesis. For G. vermiculophylla and F. vesiculosus, E a/R gas ratios for NP max tended to increase with increasing temperature () indicating that the warm-acclimated algae had a weaker photosynthetic response to increasing temperature than cold-acclimated algae. In contrast, U. lactuca showed an opposite change in the E a/R gas ratio, indicating an even stronger response to short-term temperature increases in warm- compared to cold-acclimated algae. To evaluate temperature responses of photosynthesis at supraoptimal temperatures (above T opt) we calculated the ∆H/R gas (enthalpy/gas constant) ratio. This showed a general decrease for all three species with increasing growth temperature, indicating a general rise in NP max rates at supraoptimal temperatures (). In other words, algae acclimated to high temperatures could better tolerate supraoptimal temperatures than cold-acclimated algae. No clear trends in the temperature sensitivity of respiratory metabolism were found for any of the species.
Discussion
The potential success of a non-indigenous species may be predicted by the extent of the species’ native geographical range (Forcella & Wood, Citation1984; Goodwin et al., Citation1998): the larger the native range of the species, the more likely the species is to be able to survive along environmental stress gradients, e.g. along salinity, temperature and light gradients. The native range of Gracilaria vermiculophylla is the SW Pacific Ocean, where physical parameters vary greatly on regional and seasonal scales (Phooprong et al., Citation2008), and we therefore expected it to exhibit high tolerance to environmental stress. Results from our experiments support this idea and indicate a plastic response to a wide range of combinations of light and temperature conditions. Our findings thereby support other physiological studies that have tested how G. vermiculophylla respond to temperature and light stress ().
Importantly, we found many significant interaction effects of temperature and light on growth, metabolism and chlorophyll a content (), implying that the physiological responses of G. vermiculophylla should always be interpreted in the context of explicitly listed temperature and light conditions. A combination of low light and high temperature can limit the ecological performance of temperate macroalgae during summer months, particular at night, in turbid water, and at depth or below algal mats or seagrass canopies (Peckol & Rivers, Citation1996). However, G. vermiculophylla is able to grow in very low light conditions (<1 µmol photons m−2 s−1) and at high temperature (up to at least 25°C; at 30°C, high respiration overtook photosynthesis). The low light levels required to maintain positive biomass production partially explain the high standing biomass in shallow estuaries; here G. vermiculophylla is often found in thick mats (Thomsen et al., Citation2006; Nyberg et al., Citation2009; Nejrup & Pedersen, Citation2010) in which significant self-shading occurs (Krause-Jensen et al., Citation1996; Peckol & Rivers, Citation1996). Our findings suggest that G. vermiculophylla should generally be able to sustain positive growth in shallow Scandinavian estuaries during all seasons (except for the low saline parts (<5 PSU) of the Baltic Sea). This is in contrast to the findings of Weinberger et al. (Citation2008) who performed in situ experiments and found positive growth rates in G. vermiculophylla during the summer (high temperature and high light) but not during spring and autumn, when there was reduced light and temperature. However, biomass loss caused by meso-herbivores and fragmentation (potentially induced by the grazers) in the field experiment may explain this discrepancy. Indeed, Weinberger et al. (Citation2008) recorded higher grazing rates than were reported by Nejrup & Pedersen (Citation2010). Low temperatures and ice cover (i.e. low light) have been proposed to limit the distribution of G. vermiculophylla in the Baltic Sea (Nyberg et al., Citation2009) but in the present experiment, we found positive growth rates at conditions that are relatively similar to those found under ice (1 µmol photons m−2 s−1 and 5°C: Leu et al., Citation2010; Smethie et al., Citation2011). Our results are consistent with the findings of Yokoya et al. (1999, see ), who also found positive growth rates at a temperature of 5°C in culture experiments. This indicates that G. vermiculophylla can survive and even grow a little in cold periods (down to 5°C) if not affected by other stressors. Even though positive growth rates were observed at 5°C, germination is greatly impaired at this temperature (Abreu et al., Citation2011), which could reduce recruitment success and potentially the spread of the species to regions with low summer temperatures. However, G. vermiculophylla is not necessarily dependent on sexual reproduction because, like other Gracilaria species, it can reproduce asexually through fragmentation (e.g. Rueness, Citation2005).
Both G. vermiculophylla and the two native species increased their long-term acclimated photosynthetic rates when exposed to similar short-term incubation temperatures, but with a stronger increase for U. lactuca (), which suggests a less pronounced ability to acclimate net photosynthesis for this species compared with the two slower-growing perennial algae. Similar results for the large brown alga Laminaria saccharina were shown by Davison et al. (Citation1991) and explained by acclimation of Calvin cycle enzyme activities, which enables algae grown at different temperatures to achieve similar rates of light-saturated photosynthesis (Davison & Davison, Citation1987).
The optimum growth temperature of G. vermiculophylla was found to be c. 20°C (experiment I) supporting several other studies (Yokoya et al., Citation1999; Rueness, Citation2005; Abreu et al., Citation2011; ), even though differences in experimental setup can make direct comparisons difficult. However, Yokoya et al. (Citation1999) found somewhat higher growth rates of G. vermiculophylla than those in this study, probably as a result of differences in experimental design (e.g. Yokoya measured growth in small apical fragments that did not self-shade and contained a high biomass proportion of actively growing tissue; such material typically shows high growth rates). Interestingly, Phooprong et al. (Citation2008) found that the optimum temperature of different native Japanese populations of G. vermiculophylla varied with local temperature regimes, suggesting that different ecotypes have different temperature tolerances. The notion of different ecotypes has also been suggested for other Gracilaria species (McLachlan & Bird, Citation1984) and for different invasive G. vermiculophylla strains in Europe (Rueness, Citation2005), and may explain the relatively low growth rates observed here.
Prior to the experiment, we anticipated that growth and photosynthetic rates of the three species in experiment II would be related to their form-functional grouping, i.e. U. lactuca >> G. vermiculophylla > F. vesiculosus (Littler & Littler, Citation1980). Our results partly supported this hypothesis for growth rates, but net photosynthesis was only marginally different between U. lactuca and G. vermiculophylla. This could indicate that U. lactuca, despite frequent water exchanges, experienced nutrient limitation at high temperatures, a notion supported by the tissue nitrogen data which showed decreasing concentration of N with increasing acclimation temperature. Pedersen & Borum (Citation1996) suggested that N-limitation for U. lactuca occurs when cellular concentrations decrease below 2.17% of DW with a subsistence quota of 0.71%, below which growth ceases. Similar levels for F. vesiculosus provide N-limited growth below 1.71% of DW with a subsistence quota of 0.55%. In this study, the content of N varied from 0.66 to 1.24% (at 25 and 10°C respectively) for U. lactuca and 0.89 to 0.99% for F. vesiculosus (no temperature dependency). While we do not have comparative data for G. vermiculophylla, the measured N-content (0.70 to 1.40%, at 25 and 10°C respectively) was lower than the average seasonal values that have been reported, of approximately 2% (Nejrup & Pedersen, Citation2010). These results indicate that despite our efforts to maintain N-replete conditions, all three species could have been N-limited; although this is probably most pronounced for U. lactuca at high temperatures (as this species has by far the highest maximum growth rate and nitrogen demand: Pedersen & Borum, Citation1996). However, reduced tissue nitrogen content amongst algae acclimated to warm conditions does not automatically imply nutrient-limited growth. For example, cold-acclimated plants can have a high N content as a result of high tissue concentrations of enzymes (Raven & Geider, Citation1988), lipid content (Raison et al., Citation1980), and pigment concentration (Falkowski & Laroche, Citation1991) to reduce low temperature stress. Nevertheless, as all three species were grown under identical conditions, direct comparisons of temperature-acclimated metabolism between the species remain possible. Future investigations of temperature acclimatization in respiration and photosynthesis of macroalgae, however, should also evaluate the potential impact of nitrogen limitation, which previously has been shown to influence metabolic performance (Raven & Geider, Citation1988).
Long-term acclimation to 25°C reduced the growth rate of all algal species when compared with rates at 20°C, although this decrease was most pronounced for F. vesiculosus (). In addition, all three species showed optimum growth at 20°C, somewhat higher than reported by Fortes & Lüning (Citation1980) for U. lactuca (10–15°C) and F. vesiculosus (15°C). We discovered that light and temperature have interactive effects on growth (and therefore should be quantified and reported), but Fortes & Lüning did not report light levels for their experiment; a direct comparison is therefore difficult and the reported differences could thus be due to different culture conditions. Furthermore, Nygaard & Dring (Citation2008) reported optimum growth for F. vesiculosus comparable to this study at long-term temperatures down to 15–20°C. Overall these differences in optimum temperatures suggest the presence of regional ecotypes that are adapted to prevailing environmental conditions.
Our laboratory experiments suggest that G. vermiculophylla shares common traits with both the slow-growing, leathery F. vesiculosus, which can be classified as a K-strategist, and the fast-growing, sheet-forming U. lactuca, which can be regarded as an r-strategist (Littler & Littler, Citation1980; Thomsen & McGlathery, Citation2007). When comparing temperature dependencies, G. vermiculophylla had an almost identical, but marginally weaker response to higher temperatures than U. lactuca; this was observed for somatic growth, photosynthesis and respiration. Furthermore, the calculated optimum temperature for net photosynthesis at the temperature at which the algae had long-term acclimated, was more or less the same for the two species. In contrast, the biochemical analysis of thallus content grouped G. vermiculophylla together with F. vesiculosus, being significantly different from that of U. lactuca; this difference was also seen when comparing the species’ efficiency in acclimating to changing temperatures. In conclusion, we suggest that one plausible explanation for the success of G. vermiculophylla in Scandinavian estuaries is, at least in part, the combination of relatively high growth rates with a robust metabolic response to both low and high light levels, and to varying temperatures.
Acknowledgements
MST and PAS are grateful for financial support from Aarhus University research fund (AUFF-2012-FLS-6-33).
References
- Abreu , M.H. , Pereira , R. , Sousa-Pinto , I. and Yarish , C. 2011 . Ecophysiological studies of the non-indigenous species Gracilaria vermiculophylla (Rhodophyta) and its abundance patterns in Ria de Aveiro lagoon, Portugal . European Journal of Phycology , 46 : 453 – 464 .
- Atkin , O.K. and Tjoelker , M.G . 2003 . “ Thermal acclimation and the dynamic response of plant respiration to temperature ” . In Trends in Plant Science Vol. 8 , 343 – 351 .
- Davison , I.R. and Davison , J.O. 1987 . “ The effect of growth temperature on enzyme activities in the brown alga Laminaria saccharina ” . In British Phycological Journal Vol. 22 , 77 – 87 .
- Davison , I.R. , Greene , R.M. and Podolak , E.J. 1991 . Temperature acclimation of respiration and photosynthesis in the brown alga Laminaria saccharina . Marine Biology , 110 : 449 – 454 .
- Falkowski , P.G. and Laroche , J. 1991 . Acclimation to spectral irradiance in algae . Journal of Phycology , 27 : 8 – 14 .
- Forcella , F. and Wood , J.T. 1984 . Colonization potentials of alien weeds are related to their “native” distributions: implications for plant quarantine . Journal of the Australian Institute of Agricultural Science , 50 : 35 – 41 .
- Fortes , M.D. and Lüning , K. 1980 . Growth rates of North Sea macroalgae in relation to temperature, irradiance and photoperiod . Helgoländer Meeresuntersuchungen , 34 : 15 – 29 .
- Goodwin , B.T. , McAllister , A.J. and Fahrig , L. 1998 . Predicting invasiveness of plant species based on biological information . Conservation Biology , 13 : 422 – 426 .
- Höffle , H. , Thomsen , M.S. and Holmer , M. 2011 . High mortality of Zostera marina under high temperature regimes but minor effects of the invasive macroalgae Gracilaria vermiculophylla . Estuarine, Coastal and Shelf Science , 92 : 35 – 46 .
- Jeffrey , S.W. and Humphrey , G.F. 1975 . New spectrophotometric equations for determining chlorophylls a, b, c1 and c2 in higher plants, algae and natural phytoplankton . Biochemie und Physiologie der Pflanzen , 167 : 191 – 194 .
- Jensen , R.G. 2000 . Activation of Rubisco regulates photosynthesis at high temperature and CO2 . Proceedings of the National Academy of Science USA , 97 : 12937 – 12938 .
- Johnson , F.H. , Eyring , H. and Stover , B.J. 1974 . The Theory of Rate Processes in Biology and Medicine , New York : Wiley Interscience .
- Kearney , M. and Porter , W. 2009 . Mechanistic niche modelling: combining physiological and spatial data to predict species' ranges . Ecology Letters , 12 : 334 – 350 .
- Kim , S.Y. , Weinberger , F. and Boo , S.M. 2010 . Genetic data hint at a common donor region for invasive Atlantic and Pacific populations of Gracilaria vermiculophylla (Gracilariales, Rhodophyta) . Journal of Phycology , 46 : 1346 – 1349 .
- Krause-Jensen , D. , McGlathery , K. , Rysgaard , S. and Christensen , P.B. 1996 . Production within dense mats of the filamentous macroalga Chaetomorpha linum in relation to light and nutrient availability . Marine Ecology Progress Series , 134 : 207 – 216 .
- Lapointe , B.E. , Tenore , K.R. and Dawes , C.J. 1984 . Interactions between light and temperature on the physiological ecology of Gracilaria tikvahiae (Gigartinales: Rhodophyta). I. Growth, photosynthesis and respiration . Marine Biology , 80 : 161 – 170 .
- Leu , E. , Wiktor , J. , Søreide , J.E. , Berge , J. and Falk-Petersen , S. 2010 . Increased irradiance reduces food quality of sea ice algae . Marine Ecology Progress Series , 411 : 49 – 60 .
- Littler , M.M. and Littler , D.S. 1980 . The evolution of thallus form and survival strategies in benthic marine macroalgae: field and laboratory tests of a functional form model . American Naturalist , 116 : 25 – 44 .
- Lobban , C.S. and Harrison , P.J. 1994 . Seaweed Ecology and Physiology , Cambridge : Cambridge University Press .
- Martinez-Luscher , J. and Holmer , M. 2010 . Potential effects of the invasive species Gracilaria vermiculophylla on Zostera marina metabolism and survival . Marine Environmental Research , 69 : 345 – 349 .
- Mendes , L.F. , Vale , L.A.S. , Martins , A.P. , Yokoya , N.S. , Marinho-Soriano , E. and Colepicolo , P. 2012 . Influence of temperature, light and nutrients on the growth rates of the macroalga Gracilaria domingensis in synthetic seawater using experimental design . Journal of Applied Phycology , 24 : 1419 – 1426 .
- McLachlan , J. and Bird , C.L. 1984 . Geographical and experimental assessment of the distribution of Gracilaria species (Rhodophyta, Gigartinales) in relation to temperature . Helgoländer Meeresuntersuchungen , 38 : 319 – 334 .
- Mollet , J. , Rahaoui , A. and Lemoine , Y. 1998 . Yield, chemical composition and gel strength of agarocolloids of Gracilaria gracilis, Gracilariopsis longissima and the newly reported Gracilaria cf. Vermiculophylla from Roscoff (Brittany, France) . Journal of Applied Phycology , 10 : 59 – 66 .
- Nejrup , L.B. and Pedersen , M.F. 2010 . Growth and biomass development of the introduced red alga Gracilaria vermiculophylla is unaffected by nutrient limitation and grazing . Aquatic Biology , 10 : 249 – 259 .
- Nejrup , L.B. and Pedersen , M.F. 2012 . The effect of temporal variability in salinity on the invasive red alga Gracilaria vermiculophylla . European Journal of Phycology , 47 : 254 – 263 .
- Nejrup , L.B. , Pedersen , M.F. and Vincent , J. 2012 . Grazer avoidance may explain the red alga Gracilaria vermiculophylla in Scandinavian Waters . Marine Biology , 159 : 1703 – 1712 .
- Nyberg , C.D. 2006 . “ Licenciate thesis ” . In Attributes of non-indigenous seaweeds with special emphasis on Gracilaria vermiculophylla , Göteborg University .
- Nyberg , C.D. , Thomsen , M.S. and Wallentinus , I. 2009 . Flora and fauna associated with the introduced red alga Gracilaria vermiculophylla . European Journal of Phycology , 44 : 395 – 403 .
- Nygaard , C.A. and Dring , M.J. 2008 . Influence of salinity, temperature, dissolved inorganic carbon and nutrient concentration on the photosynthesis and growth of Fucus vesiculosus from the Baltic and Irish Seas . European Journal of Phycology , 43 : 253 – 262 .
- Peckol , P. and Rivers , J.S. 1996 . Contribution by macroalgal mats to primary production of a shallow embayment under high and low nitrogen-loading rates . Estuarine, Coastal and Shelf Science , 43 : 311 – 325 .
- Pedersen , M.F. and Borum , J. 1996 . Nutrient control of algal growth in estuarine waters. Nutrient limitation and the importance of nitrogen requirements and nitrogen storage among phytoplankton and species of macroalgae . Marine Ecology Progress Series , 142 : 261 – 272 .
- Phooprong , S. , Ogawa , H. and Hayashizaki , K. 2008 . Photosynthetic and respiratory responses of Gracilaria vermiculophylla (Ohmi) Papenfuss collected from Kumamoto, Shizuoka and Iwate, Japan . Journal of Applied Phycology , 20 : 743 – 750 .
- Raikar , S.V. , Iima , M. and Fujita , Y. 2001 . Effect of temperature, salinity and light intensity on the growth of Gracilaria spp. (Gracilariales, Rhodophyta) from Japan, Malaysia and India . Indian Journal of Marine Sciences , 30 : 98 – 104 .
- Raison , J.K. , Berry , J.A. , Armond , P.A. and Pike , C.S . 1980 . “ Membrane properties in relation to the adaptation of plants to temperature stress ” . In Adaptation of Plants to Water and Temperature Stress , Edited by: Turner , N.C. and Kramer , P.J. 261 – 273 . John Wiley and Sons, New York .
- Raven , J.A. and Geider , R.J. 1988 . Temperature and algal growth . New Phytologist , 110 : 441 – 461 .
- Rueness , J. 2005 . Life history and molecular sequences of Gracilaria vermiculophylla (Gracilariales, Rhodophyta), a new introduction to European waters . Phycologia , 44 : 120 – 128 .
- Ruesink , J.L. , Feist , B.E. , Harvey , C.J. , Hong , J.S. , Trimble , A.C. and Wisehart , L.M. 2006 . Changes in productivity associated with four introduced species: ecosystem transformation of a ‘pristine’ estuary . Marine Ecology Progress Series , 311 : 203 – 215 .
- Ruiz , G.M. , Fofonoff , P. , Hines , A.H. and Groshol , E.D. 1999 . Non-indigenous species as stressors in estuarine and marine communities: assessing invasion impacts and interactions . Limnology and Oceanography , 44 : 950 – 972 .
- Santamaria , L. and van Vierssen , W. 1997 . Photosynthetic temperature responses of fresh- and brackish-water macrophytes: a review . Aquatic Botany , 58 : 135 – 150 .
- Saunders , G.W. 2009 . Routine DNA barcoding of Canadian Gracilariales (Rhodophyta) reveals the invasive species Gracilaria vermiculophylla in British Columbia . Molecular Ecology Resources , 9 : 140 – 150 .
- Smethie , W.M. Jr. , Chayes , D. , Perry , R. and Schlosser , P. 2011 . A lightweight vertical rosette for deployment in ice-covered waters . Deep Sea Research I , 58 : 460 – 467 .
- Sfriso , A. , Maistro , S. , Andreoli , C. and Moro , I. 2010 . First record of Gracilaria vermiculophylla (Gracilariales, Rhodophyta) in the Po Delta lagoons, Mediterranean Sea (Italy) . Journal of Phycology , 46 : 1024 – 1027 .
- Staehr , P.A. and Birkeland , M. 2006 . Temperature acclimation of two mesophilic microalgae . Phycologia , 45 : 648 – 656 .
- Staehr , P.A. and Borum , J. 2011 . Seasonal acclimation in metabolism reduces light requirements of eelgrass (Zostera marina) . Journal of Experimental Marine Biology and Ecology , 407 : 139 – 146 .
- Staehr , P.A. and Wernberg , T. 2009 . Ocean climate controls the temperature dependency of metabolic rates in kelp . Journal of Phycology , 45 : 91 – 99 .
- Thomsen , M.S. 2010 . Experimental evidence for positive effects of invasive seaweed on native invertebrates via habitat-formation in a seagrass bed . Aquatic Invasions , 5 : 341 – 346 .
- Thomsen , M.S. and McGlathery , K. 2006 . Effects of accumulations of sediments and drift algae on recruitment of sessile organisms associated with oyster reefs . Journal of Experimental Marine Biology and Ecology , 328 : 22 – 34 .
- Thomsen , M.S. and McGlathery , K.J. 2007 . Stress tolerance of the invasive macroalgae Codium fragile and Gracilaria vermiculophylla in a soft-bottom turbid lagoon . Biological Invasions , 9 : 499 – 513 .
- Thomsen , M.S. , McGlathery , K.J. and Tyler , A.C. 2006 . Macroalgal distribution patterns in a shallow, soft-bottom lagoon, with emphasis on the non-native Gracilaria vermiculophylla and Codium fragile . Estuaries and Coasts , 29 : 465 – 473 .
- Thomsen , M.S. , Staerh , P.A. , Nyberg , C.G. , Schwærter , S. , Krause-Jensen , D. and Silliman , B.R . 2007 . Gracilaria vermiculophylla (Ohmi) Papenfuss, 1967 (Rhodophyta, Gracilariaceae) in northern Europe, with emphasis on Danish conditions, and what to expect in the future . Aquatic Invasions , 2 : 83 – 94 .
- Thomsen , M.S. , Wernberg , T. , Stæhr , P.A. , Silliman , B.R. , Josefson , A.B. , Krause-Jensen , D. and Risgaard-Petersen , N . 2008 . Annual changes in abundance of non-indigenous marine benthos on a very large spatial scale . Aquatic Invasions , 3 : 133 – 140 .
- Thomsen , M.S. , Wernberg , T. , Altieri , A. , Tuya , F. , Gulbrandsen , D. , McGlathery , K.J. , Holmer , M. and Silliman , B.R. 2010 . Habitat cascades: the conceptual context and global relevance of facilitation cascades via habitat formation and modification . Integrative and Comparative Biology , 50 : 158 – 175 .
- Thomsen , M.S. , Wernberg , T. , Olden , J.D. , Griffin , J.N. and Silliman , B.R. 2011 . A framework to study the context-dependent impacts of marine invasions . Journal of Experimental Marine Biology and Ecology , 400 : 322 – 327 .
- Tyler , A.C. and McGlathery , K.J. 2006 . Uptake and release of nitrogen by the macroalgae Gracilaria vermiculophylla (Rhodophyta) . Journal of Phycology , 42 : 515 – 525 .
- Webb , W.L. , Newton , M. and Starr , D. 1974 . Carbon dioxide exchange of Alnus rubra: a mathematical model . Oecologia , 17 : 281 – 291 .
- Weinberger , F. , Buchholz , B. , Karez , R. and Wahl , M. 2008 . The invasive red alga Gracilaria vermiculophylla in the Baltic Sea: adaptation to brackish water may compensate for light limitation . Aquatic Biology , 3 : 251 – 264 .
- Yokoya , N.S. , Kakita , H. , Obika , H. and Kitamura , T. 1999 . Effects of environmental factors and plant growth regulators on growth of the red alga Gracilaria vermiculophylla from Shikoku Island, Japan . Hydrobiologia , 399 : 339 – 347 .